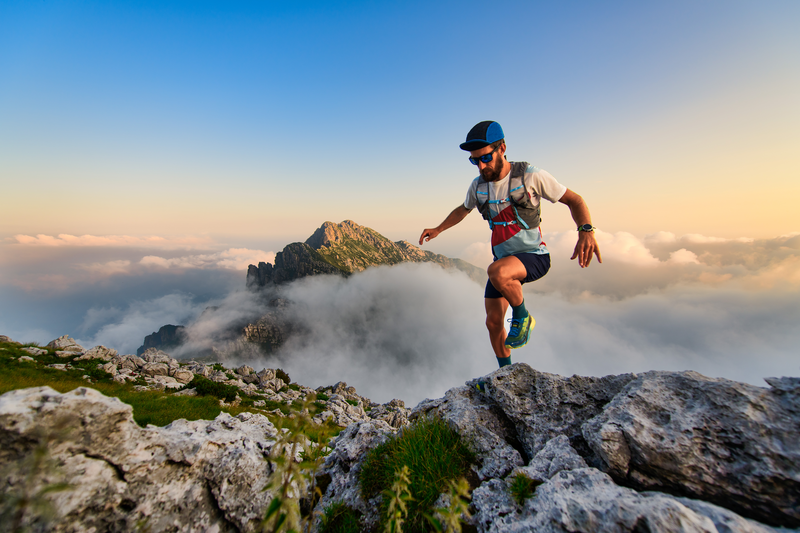
94% of researchers rate our articles as excellent or good
Learn more about the work of our research integrity team to safeguard the quality of each article we publish.
Find out more
ORIGINAL RESEARCH article
Front. Pharmacol. , 10 June 2020
Sec. Pharmacology of Ion Channels and Channelopathies
Volume 11 - 2020 | https://doi.org/10.3389/fphar.2020.00848
This article is part of the Research Topic Ion Channel Signalling in Cancer: From Molecular Mechanisms to Therapeutics View all 17 articles
Increasing evidence indicates that ion channels and transporters cooperate in regulating different aspects of tumor pathophysiology. In cancer cells, H+/HCO3- transporters usually invert the transmembrane pH gradient typically observed in non-neoplastic cells, which is thought to contribute to cancer malignancy. To what extent the pH-regulating transporters are functionally linked to K+ channels, which are central regulators of cell membrane potential (Vm), is unclear. We thus investigated in colorectal cancer cells the implication of the pH-regulating transporters and KV11.1 (also known as hERG1) in the pH modifications stimulated by integrin-dependent cell adhesion. Colorectal cancer cell lines (HCT 116 and HT 29) were seeded onto β1 integrin-dependent substrates, collagen I and fibronectin. This led to a transient cytoplasmic alkalinization, which peaked at 90 min of incubation, lasted approximately 180 min, and was inhibited by antibodies blocking the β1 integrin. The effect was sensitive to amiloride (10 µM) and cariporide (5 µM), suggesting that it was mainly caused by the activity of the Na+/H+ antiporter NHE1. Blocking KV11.1 with E4031 shows that channel activity contributed to modulate the β1 integrin-dependent pHi increase. Interestingly, both NHE1 and KV11.1 modulated the colorectal cancer cell motility triggered by β1 integrin-dependent adhesion. Finally, the β1 integrin subunit, KV11.1 and NHE1 co-immunoprecipitated in colorectal cancer cells seeded onto Collagen I, suggesting the formation of a macromolecular complex following integrin-mediated adhesion. We conclude that the interaction between KV11.1, NHE1, and β1 integrin contributes to regulate colorectal cancer intracellular pH in relation to the tumor microenvironment, suggesting novel pharmacological targets to counteract pro-invasive and, hence, pro-metastatic behavior in colorectal cancer.
Ion channels and transporters are progressively emerging as pivotal modulators of different aspects of cancer cell behavior (Arcangeli et al., 2009; Lastraioli et al., 2015a). Such pleiotropic effects can be traced back to the regulation of either Vm (an effect mainly exerted by K+ channels; Huang and Jan, 2014), or of the concentration and intracellular distribution of specific ion species, such as Ca2+ (Bose et al., 2015) and H+ (Gadsby, 2009), or to the direct modulation of intracellular signaling pathways (Arcangeli et al., 2009; Becchetti et al., 2019). However, the molecular interactions between these mechanisms are poorly understood, and a unified picture of the cancer cell pathophysiology is still missing.
One of the K+ channels most often dysregulated in cancer is KV11.1 (or hERG1), which regulates the resting Vm in excitable cells (Bauer and Schwarz, 2018), as well as in cancers arising from excitable (e.g. neuroblastomas, Crociani et al., 2003) and non-excitable tissues. In particular, KV11.1 modulates intracellular signaling pathways triggered by integrin-mediated adhesion, both in leukemias (Pillozzi and Arcangeli, 2009) and solid cancers such as the colorectal (Crociani et al., 2013), pancreatic (Lastraioli et al., 2015b), gastric (Crociani et al., 2014) and mammary (Becchetti et al., 2017). The underlying mechanism involves the formation of a macromolecular complex between KV11.1 and β1-integrins, which promotes angiogenesis and triggers metastatic spread (Crociani et al., 2013; Becchetti et al., 2017). In pancreatic ductal adenocarcinoma cells, this occurs through the regulation of f-actin dynamics in filopodia (Manoli et al., 2019).
Cancer proliferation and migration are also controlled by intracellular pH, whose regulation is frequently dysregulated in tumors (Webb et al., 2011). Hence, targeting the pH regulating transporters has been suggested as a therapeutic strategy (Persi et al., 2018). Cancer cells generally display a higher activity of the V-type H+-ATPases expressed on the plasmalemma (Sennoune et al., 2004), the Na+/H+ exchanger NHE1 (Stock and Pedersen, 2017), the monocarboxylate transporters (MCTs) (Pinheiro et al., 2012), the Na+/HCO3- cotransporters (NBCs) (Gorbatenko et al., 2014), and the membrane-associated carbonic anhydrases (CAs), such as CA II and CA IX (Mboge et al., 2018). The concerted activity of these proteins generally leads to an inverted transmembrane pH gradient, characterized by alkalization of intracellular pH (pHi) and extracellular acidosis. This is considered a hallmark of cancer metabolism (Sharma et al., 2015), being associated with increased tumor proliferation, invasion, metastasis, and thus higher aggressiveness and resistance to treatment (McCarty and Whitaker, 2010). Both the pHi alkalinization and the extracellular acidosis sustained by the higher activity of H+ transporters in cancer cells promote cell proliferation, escape from apoptosis and metabolic adaptation (Webb et al., 2011). In addition, the inverted pH gradient is involved in the control of cell migration (Webb et al., 2011). An acid extracellular environment favors the formation of invadopodia and activates proteases that degrade the extracellular matrix, hence favoring cancer cell motility and invasiveness (Busco et al., 2010). Conversely, an alkaline pHi stimulates cell motility by promoting cytoskeleton assembly and focal adhesion remodeling (Srivastava et al., 2008; Frantz et al., 2008), and hence is one of the main hallmarks of metastatic tumors (Stock and Schwab, 2009). In this scenario, a pivotal role is exerted by integrin receptors and by the Na+/H+ antiporter NHE1, whose reciprocal regulatory interaction was discovered in the late eighties (Schwartz et al., 1989; Demaurex et al., 1996). In particular, NHE1 is stimulated by cell adhesion, and in turn regulates cell attachment and spreading onto fibronectin (Harguindey et al., 2005; Stock and Schwab, 2006). In migrating cells, the production of a pHi gradient along the axis of movement accompanies the accumulation of NHE1 at the migrating front, which localizes close to integrins (Grinstein et al., 1993; Plopper et al., 1995; Ludwig et al., 2013). The acidic pericellular environment at the leading edge is thought to increase the pHe-dependent avidity of integrins, which facilitates cell-matrix interactions and modulates adhesion strength (Lehenkari and Horton, 1999; Eble and Tuckwell, 2003; Stock et al., 2005; Stock et al., 2008). The corresponding local pHi increase stimulates the focal adhesion dynamics. First, it supports the F-actin severing activity of cofilin (Frantz et al., 2008), which produces free-barbed-end actin in the lamellipodium. Second, it reduces the affinity of talin for actin (Srivastava et al., 2008). It is thus clear that the pH regulating mechanisms are essential determinants of the tumor microenvironment and the cancer cell crosstalk.
Based on the above premises, we investigated whether the pH-regulating transporters are functionally linked to KV11.1 channels, which are strongly dysregulated in cancer cells, and whose activity is tightly related to integrin receptors in modulating cancer cell proliferation and migration (Becchetti et al., 2019). In particular, we studied if pH regulating mechanisms provide a direct link between integrin-mediated hERG1-dependent cell adhesion and the tumor microenvironment. As a model, we used ColoRectal Cancer (CRC) cells, in which knowledge about KV11.1 physiology is particularly extensive.
Unless otherwise indicated, chemicals and drugs were purchased from Sigma-Aldrich (St. Louis, USA).
The human colon carcinoma cell lines HCT 116 and HT 29 were cultured at 37°C and 5% CO2 in air, in Roswell Park Memorial Institute (RPMI) 1640 Medium, with sodium bicarbonate (2 g/L) and 2mmol/L L-glutamine (“culture medium”), supplemented with 10% fetal bovine serum (FBS) (Euroclone, Italy). In all experiments, cells were starved overnight in culture medium without serum (“no-serum medium”) and detached, prior to experiment, with PBS plus 5 mM EDTA.
Extracellular Matrix (ECM) proteins at the final amount per cm2 of surface area shown in brackets: Fibronectin (FN, 5 μg), Collagen-1 [Col-1, 10 μg; produced as reported in Hallowes et al. (1980)], Vitronectin [VN, 0.5 μg; produced as reported in Yatohgo et al. (1988)]. Coating with Polylysine (PL, 0.1 µg) was taken as a control of integrin-independent adhesion, while seeding onto uncoated dishes was our “no-adhesion” control. FN and VN were diluted in PBS, Col-1 in serum-free media, PL in bi-distilled water and plated to cover the entire growth surface, followed by 1 h incubation at either 37°C (for Col-1) or room temperature (for FN, VN and PL). After the coating procedure, incubation with Bovine Serum Albumin (BSA) for 15 min at 37°C was performed to block all the uncovered plastic sites.
To determine pHi, we used 2′,7′-Bis (2-carboxyethyl)-5 (6)-carboxyfluorescein acetoxymethyl esther (BCECF-AM). Cells were starved and detached as described above, and seeded (5 x 104 cells/well) in no-serum medium onto uncoated or coated (see above) 96-well plates (clear bottom 96-well plate, polystyrene, TC-treated, clear flat bottom wells, sterile, w/lid, black; Corning, New York, USA). Cells were then incubated at 37°C in 5% CO2 for different times, in the absence or presence of different drugs (see below). At selected time points, the medium was removed and BCECF-AM (1μM final concentration in loading solution (HBSS 1X plus 0.01% NaHCO3, pH 7)) was added for 30 min at 37°C and 5% CO2. After incubation, cells were washed twice with loading solution at room temperature. For measurement of initial (time 0) pHi, cells were detached, kept in suspension in a 1.5 ml tube and incubated in BCECF-AM-containing solution for 30 min at 37°C. Next, they were washed twice with loading solution, poured in a 96-well plate at 5x104 cells/well at room temperature, and immediately transferred to the microplate reader. Fluorescence intensity was immediately measured with a microplate reader (Infinite 200 PRO, Tecan, Switzerland) set at the following wavelengths: 440–490nm for excitation and 535 nm for emission. A calibration curve was set up using a high K+/Nigericin solution (135 mM KCl, 2 mM K2HPO4, 20 mM HEPES, 1.2 mM CaCl2 and 0.8 mM MgSO4), in a range of pH from 5.0 to 8.5. All pH values were calculated using 490/440nm fluorescence ratio and applying standard curve and linear equations, as detailed in Grant and Acosta, 1997.
The mouse monoclonal anti-β1 integrin antibody BV7 (anti-β1 Ab, kindly gifted by Prof. P. Defilippi, University of Turin, Italy) (Martìn-Padura et al., 1994) was used to block β1-integrins as described in Hofmann et al. (2001). Briefly, cells were seeded onto Col-I in no serum medium, containing anti-β1 Ab (20 μg/ml), for 90 min at 37°C and 5% CO2. Cells (mostly detached) were collected, and pHi mesaurement was performed as above described for time zero condition.
We used the following compounds: 100 µM acetazolamide (CA inhibitor; Parkkila et al., 2000), 10 µM amiloride (inhibitor of NHE1 and epithelial Na+ channel, ENaC; Masereel et al., 2003), 5 µM cariporide (specific NHE1 inhibitor; Hulikova et al., 2013), 30 µM S0859 (NBC inhibitor; Hulikova et al., 2013), and 40 µM E4031 (Tocris, Bristol, UK; KV11.1 blocker; Masi et al., 2005),
Drugs were added to the cells seeded on uncoated or coated surfaces at different time points. Preliminarily, all compounds were tested for their potential cytotoxic effects at all the used concentrations, by measuring cell viability with the trypan blue test (Pillozzi et al., 2018), at 30, 90 and 180 min of incubation. None of the modulators had any cytotoxic effect (Table 1).
Lateral motility was determined using 35 mm dishes and drawing 15 horizontal lines and 3 perpendicular lines on the dish bottom to generate a grid system. Plates were coated with Col-I and 5x105 cells were seeded and allowed to attach for 90 min. Three wounds were drawn following the 3 horizontal lines. Subsequently, the following treatments were performed with drugs diluted in RPMI medium: control, E4031 40 µM, E4031 40 µM + cariporide 5 µM. Then, the distances between cells were measured at each mark point (where the 3 horizontal lines crossed the 15 vertical lines) using a light microscope. The widths measured at time 0 correspond to the W0 parameter. These different 45 points were measured again after 90′. Motility Index (MI) was assessed using the following formula: MI = 1 – Wt/W0, where Wt is the width of the wounds after 90′.
Each treatment was performed in triplicate and the experiments were carried out at least 3 times.
For (co)-immunoprecipitation experiments cells were seeded and incubated on Col-I. Cells were gently collected by mild scraping and resuspended in ice cold PBS. Protein extraction, quantification and total lysate incubation with protein A/G agarose beads (Santa Cruz Biotechnology, Texas, USA) were performed as previously reported (Becchetti et al., 2017). In particular, the composition of the lysis buffer was the following: NP40 (150 mM), NaCl (150 mM), Tris-HCl pH 8 (50 mM), EDTA pH 8 (5 mM), NaF (10 mM), Na4P2O7 (10 mM), Na3VO4 (0.4 mM), and protease inhibitor cocktail (cOmplete Mini-Roche, Germany).
The following antibodies were used at the concentration of 5µg per mg of extracted proteins: LEAF Purified anti-human, Clone TS2/16 (BioLegend, California, USA) to immunoprecipitate the β1-integrin; mAb KV11.1 (MCK Therapeutics, Italy) to immunoprecipitate KV11.1. After overnight incubation, the immuno-complex was captured by adding 30 µl of protein A/G agarose beads for 2 h at 4°C (with rolling agitation). The agarose beads were washed 3 times in ice-cold wash buffer and 3 times in ice cold PBS followed by addition of 2X Laemli buffer (10 µl) and boiled for 5 min at 95°C. Afterwards, SDS-PAGE was performed. After electrophoresis, proteins were transferred onto PVDF membrane (previously activated) in blotting buffer under cold condition for 1 h at 100 V. The PVDF membrane was then blocked with 5% BSA in T-PBS (0.1% tween) solution for 3h at room temperature to cover the unspecific antibody binding sites on the membrane. SDS-PAGE and antibody incubation were performed as previously described (Becchetti et al., 2017). The following antibodies were used: anti β1-integrin, RM-12, polyclonal rabbit antibody, dilution 1:1,000 (Immunological Science, Italy); anti-Kv11.1, C54 polyclonal rabbit antibody, dilution 1:1,000 (DI.V.A.L TOSCANA S.R.L., Italy); anti-NHE1 polyclonal rabbit antibody, dilution 1:500 (Novus Biologicals, Colorado, USA) and anti-tubulin, monoclonal mouse, dilution 1:500 (Santa Cruz Biotechnology, Texas, USA). The following day the membrane was washed with T-PBS (0.1% tween) (15 min x 3 times) and appropriate secondary antibody: (i) conjugated with peroxidase enzyme was dissolved in 5% BSA in T-PBS (0.1% tween) (dilution 1:10.000) for at least 45 min and washed (15 min x 3 times), revealing was performed using ECL solution in dark room (anti-C54 primary antibody) and (ii) for all other primary antibodies, IRDYe 800 CW (LI-COR Biosciences, Nebraska, USA) was dissolved in 5% BSA in T-PBS (0.1% tween) (dilution 1:20.000) for at least 45 min and washed (15 min x 3 times) before membrane scanning using LI-COR Odyssey Scanner (Biosciences, Nebraska, USA).
Data were analyzed with ImageJ and graphs were plotted with OriginPro8. When quantifying variations in KV11.1-β1 integrin interaction, the signal for co-immunoprecipitated protein was first divided by the signal of the protein used for immunoprecipitation and then normalized to the signal of the corresponding protein in the total lysate.
OriginPro8 was used for analysis. Data groups were tested for normality (Shapiro-Wilk test) and variance homogeneity (Welch test). Statistical significance for two sample analysis was carried out with unpaired t-test. Multiple comparisons were carried out by One-way ANOVA, with post-hoc Bonferroni test. A p value ≤ 0.05 was considered statistically significant.
HCT 116 and HT 29 cells were incubated in serum-free medium for different times (0–180 min) onto different ECM substrates: Col-I, FN, and VN. Polylysine or no-coated plastic surfaces were used as integrin-independent or “no-adhesion” controls, respectively. At different time points, pHi was determined by BCECF-AM. Although with slightly different time courses, both cell lines underwent an early pHi increase between 0 and 90 min (Figures 1A, B). At 90 min of incubation, pHi was significantly higher in cells seeded onto Col-I and FN, i.e., two substrates recognized by β1 integrins, which are well expressed in both cell lines (Table 2). Indeed, treatment with a β1 integrin blocking antibody (BV/, Martìn-Padura et al., 1994) (indicated as “anti-β1 Ab” in Figure 1) not only blocked cell adhesion (panel A’ and B’), but also prevented the pHi increase triggered by cell adhesion onto Col-I. In particular, pHi increased from 6.7 at time 0 (i.e., before seeding) to 7.2 at 90 min in cells seeded either onto Col-I or FN. In contrast, pHi remained close to the time 0 value (6.74±0.006 for HCT 116 and 6.66±0.014 for HT 29) in CRC cells seeded onto Col-I and treated with the anti-β1 Ab (insets to Figures 1A, B). The pHi alkalinization was much smaller in cells seeded onto VN (in agreement with the very low expression of β3-integrins in both cell lines, see Table 2), or polylysine or no-coating conditions. Subsequently (i.e., after 90 min of incubation), the pHi observed in cells seeded on Col-I and FN progressively returned to the control value. At 180 min, cells displayed a pHi around 7.0, irrespective of growing conditions (Figures 1A, B). Such values were maintained for at least 24 h (the complete data set is given in Table 3). We conclude that the β1 integrin-mediated adhesion triggers an early and transient pHi alkalinization from 6.7 to 7.2 in CRC cells.
Figure 1 Effect of Collagen I, Fibronectin, and Vitronectin on pHi in HCT 116 and HT 29 cells. The time course of pHi is reported in panel (A) (HCT 116) and in panel (B) (HT 29). Simbol ▪: No coating surface, •: PL coating, ▲: Col-I coating, ▼: FN coating, ♦: VN coating, ○: Col-I anti-β1 Ab. On the right of the panel, 90 min pHi values are reported. Light grey bar: No coating surface, dark grey bar: Col-I, black bar: FN and striped bar: Col-I anti-β1 Ab. Number represent mean ± s.e.m (of three different experiments). *, P < 0.05; **, P < 0.01 and ***, P < 0.001. p value after 30 min of seeding, panel (A): ***P < 0.001: Col-I vs Control: 0.0005, FN vs Control: 0.0005; p value after 90 min of seeding, panel (A): ***P < 0.001: Col-I vs Control: 0.0001, FN vs Control: 0.0001; *P < 0.05: No coat vs Control: 0.01, PL vs Control: 0.01 and VN vs Control: 0.02. p value after 180 min of seeding, panel (A): *P < 0.05: No coat vs Control: 0.01, PL vs Control: 0.01 and VN vs Control: 0.01, Col-I vs Control: 0.01, FN vs Control: 0.01. p value after 30 min of seeding, panel (B) ***P < 0.001: Col-I vs Control: 0.0001 and FN vs Control: 0.0001; p value after 90 min of seeding, panel (B) ***P < 0.001: Col-I vs Control: 0.0001 and FN vs Control: 0.0001; *P < 0.05: No coat vs Control: 0.01, PL vs Control: 0.01 and VN vs Control: 0.01. p value after 180 min of seeding, panel (B) *P < 0.05: No coat vs Control: 0.01, PL vs Control: 0.01, VN vs Control: 0.01, Col-I vs Control: 0.01 and FN vs Control: 0.01. p value after 30 min of seeding, panel (A): ***P < 0.001: Col-I vs No coat: 0.001 and FN vs No coat: 0.001. p value after 90 min of seeding, panel (A): Col-I vs No coat: 0.0001, FN vs No coat: 0.0001; Col-I anti-β1 Ab vs Col-I: 0.0007; Col-I anti-β1 Ab vs FN: 0.0006. p value after 30 min of seeding, panel (B) ***P < 0.001: Col-I vs No coat: 0.001 and FN vs No coat: 0.001. p value after 90 min of seeding, panel (B) Col-I vs No coat: 0.001 and FN vs No coat: 0.001. Col-I anti-β1 Ab vs Col-I: 0.0006; Col-I anti-β1 Ab vs FN: 0.0005. Representative images of cells seeded for 90 min on Col-I, no treated and treated with anti-β1 Ab are reported in panels AI (HCT 116) and BI (HT 29), 100 µm scale bar. The conditions are shown on the top of each picture. Cells seeded on Col-I are elongated and attached, cells seeded on Col-I and treated with anti-β1 Ab are round and detached.
To better determine the mechanism of integrin-dependent pHi increase, we applied blockers of the different pH-regulating transporters expressed in CRC cells (Table 2). In particular, we tested acetazolamide (a wide CA inhibitor), amiloride (an NHE blocker, in particular of NHE1, as well as of ENaC), cariporide (a specific NHE1 inhibitor), and S0859 (an inhibitor of all NBCs). Drugs were used at the concentrations indicated in Materials and Methods, on cells seeded onto Col-I for 90 min, since the beginning of the experiment. Acetazolamide had no effect on pHi of either cell line, whereas both amiloride and cariporide produced a statistically significant decrease of pHi which reached values comparable to those detected in cells before seeding (dotted line in Figure 2). The same effect was produced by cariporide. The treatment with S0859 produced a reduction of pHi, although much lower compared to that obtained with amiloride (Figures 2A, B).
Figure 2 Effect of Acetazolamide, Amiloride, S0859, Cariporide, E4031, and E4031 plus Cariporide on pHi in cells seeded on collagen I, 90 min treatment, in HCT 116 and HT 29 cells. pHi values of HCT 116 are reported in panel (A) and for HT 29 in panel (B). Red line: pHi value at time zero. Number represent mean ± s.e.m (of three different experiments). *, P < 0.05 and ***, P < 0.001. p value panel (A): ***P < 0.001: Control vs Amil: 1.9e^-05, Control vs Carip: 1.9e^-05, Control vs E4031: 0.0001, Control vs E4031+Carip: 1.8e^-05Acet vs Carip: 1.7e^-05; *P < 0.05: Control vs S0859: 0.03, Acet vs S0859: 0.03, Amil vs S0859: 0.02; Carip vs S0859: 0.02; S0859 vs E4031+ Carip: 0.02. p value panel (B): ***P < 0.001: Control vs Amil: 1.5e^-05, Control vs Carip: 1.4e^-05, Acet vs Carip: 1.3e^-05; Control vs E4031: 0.0001 and Control vs E4031+Carip: 1.9e^-05; *P < 0.05: Control vs S0859: 0.04, Acet vs S0859: 0.03; S0859 vs E4031+ Carip: 0.02.
These results suggest that the early alkalinization triggered by β1 integrin-mediated adhesion is mostly sustained by the activity of the Na+/H+ antiporter NHE1, with a lesser contribution of NBC, and scarse involvement of carbonic anhydrases.
We then tested whether KV11.1 was involved in the integrin-dependent pHi alkalinization. To this purpose, cells were treated with the KV11.1 blocker E4031, at 40 µM (Masi et al., 2005). After 90 min of cell adhesion on Col-I, both HCT 116 and HT 29 cells treated with E4031 showed pHi values significantly more acidic compared to the untreated controls (Figures 2A, B). Hence, the activity of KV11.1 appears to control NHE1 activation, after β1 integrin-mediated adhesion. This interpretation was supported by the observation that the combined treatment with E4031 and cariporide had no further effect on the pHi value obtained after NHE1 inhibition by cariporide (Figures 2A, B).
Next, based on the known correlation between pHi and cell motility, we performed experiments of lateral motility on our CRC cell lines, which were seeded onto Col-I for 90 min, and treated with either E4031, or cariporide, or a combination of both. Both cariporide and E4031 produced a statistically significant reduction of the motility index compared to untreated cells (Figures 3A, B). The combined treatment with E4031 and cariporide only slightly increased the inhibitory effects of the single treatments on the motility index of either cell line. We conclude that both KV11.1 and NHE1 are involved in controlling the β1 integrin-dependent cell motility in CRC cells.
Figure 3 Effect of E4031 and E4031 plus Cariporide on motility index in cells seeded on col-I, 90 min treatment. Motility index values are reported for HCT 116 in panel (A) and for HT 29 in panel C. Number represent mean ± s.e.m (of three different experiments). Representative images are reported in panel (B) for HCT 116 and in panel (D) for HT 29, 200 µm scale bar. In figure the statistically significant differences between control and treatments are reported. **, P < 0.01. p value panel (A): **, P < 0.01: Control vs E4031: 0.002 and Control vs E4031+Carip: 0.001. p value panel (C): **, P < 0.01: Control vs E4031: 0.003 and Control vs E4031+Carip: 0.001.
We previously showed that cell adhesion onto β1 integrin-dependent substrates (e.g., FN or Col-I), induces KV11.1 activation, as well as the formation of a macromolecular signaling complex between the channel and β1 integrin on the plasma membrane of HCT 116 cells (Crociani et al., 2013). We thus hypothesized that NHE1 could be also recruited in such complex, which could account for the functional cross-talk between integrin receptors, KV11.1, and NHE1 in CRC cells. Hence, we seeded HCT116 cells on either uncoated or Col-I-coated surfaces for 90 min, and immunoprecipitated the extracted proteins with anti-β1 integrin or anti-KV11.1 antibodies. Blots were then revealed, respectively, with anti-KV11.1 or anti-β1 integrin antibodies, as well as with anti-NHE1 antibodies. We observed that β1-integrin co-immunoprecipitated with both KV11.1 and NHE1 in CRC cells before cell seeding (“pre seeding” in Figure 4), indicating the formation of a β1/Kv11.1/NHE1 complex, whose assembly was further promoted by cell adhesion onto Col-I for 90 min (lanes 3 and 4 in Figure 4). On the contrary, in cells seeded onto uncoated surfaces, only a weak co-immunoprecipitation was observed between β1-integrin and KV11.1, and no association was observed with NHE1. We conclude that cell adhesion onto Col-I stimulates the formation of a macromolecular complex between β1-integrin, KV11.1, and NHE1.
Figure 4 β1-integrin, KV11.1, and NHE1 protein complex. (A) Co-immunoprecipitation of β1 Integrin, Kv 11.1 and NHE1 in HCT 116 cells, seeded on no coating surface and Col-I for 90 min. Densitometric analysis is reported in panel (A). In panel A with “WB” is indicated the protein signal in the co-ip and with “INPUT” the protein signal in the total lysate. Pre-seeding condition is reported as pre, No coating as No coat and Collagen I as Col-I; The immunoprecipitation with anti β1 integrin antibody is indicated as IP β1 and with anti KV11.1 antibody is reported as IP KV11.1. Complex quantification is reported in panel B, black bar: β1-integrin, KV11.1 and NHE1 protein complex and white bar: β1-integrin and KV11.1 protein complex. Number represent mean ± s.e.m (of three different experiments). **, P < 0.01 and ***, P < 0.001. p value panel (B), ***, P < 0.001: β1-integrin/KV11.1/NHE1 complex, Pre vs Col-I IP β1, p: 0.0008; β1-integrin/KV11.1 complex, Pre vs Col-I IP β1, p: 0.00075; β1-integrin/KV11.1/NHE1 complex, Pre vs Col-I IP KV11.1, p: 0.00076; β1-integrin/KV11.1 complex, Pre vs Col-I IP KV11.1 p: 0.00074. β1-integrin/KV11.1/NHE1 complex, No coat vs Col-I IP β1, p: 1.7e^-05; β1-integrin/KV11.1 complex No coat vs Col-I IP β1, p: 0.00072; β1-integrin/KV11.1/NHE1 complex, No coat vs Col-I IP KV11.1, p: 1.9e^-05; β1-integrin/KV11.1 complex, No coat vs Col-I IP KV11.1, p: 0.00073. **, P < 0.01: β1-integrin/KV11.1/NHE1 complex, Pre vs No coat: 0.002; β1-integrin/KV11.1/NHE1 complex vs β1-integrin/KV11.1 complex, No coat IP β1: 0.002. Cropped images of blots are reported.
In the present paper, we provide evidence that, in CRC cells, the β1 integrin-mediated adhesion onto ECM proteins such as Col-I and FN triggers an early and transient pHi alkalinization, from 6.7 to 7.2. The effect is caused by NHE1 activation and is modulated by the activity of the voltage-dependent K+ channel KV11.1. The transporter and the channel appear to cooperate in sustaining the ECM-induced CRC cell motility. Their action is accompanied by the formation of a macromolecular complex between the β1 integrin, KV11.1 and NHE1.
The rapid β1 integrin-dependent pHi alkalinization in CRC cells is similar to the one initially reported in bovine capillary endothelial cells (Schwartz et al., 1991), which is also induced by integrin engagement (mainly β1), and sustained by activation of the Na+/H+ exchanger. In our model, we confirmed the NHE1 involvement by showing that ECM-dependent alkalinization was blocked by cariporide.
Following Schwartz’s seminal observation, the pH regulatory role of NHE1 in normal and cancer cells has been receiving increasing attention (Stock and Pedersen, 2017). In particular, in CRC cells, both H+ extrusion through NHE1 and HCO3- influx through NBCe1 give a significant contribution to pHi regulation. However, while HCO3- influx appears to represent a constitutive element of pHi regulation, the NHE1-mediated H+ efflux may vary, depending on culture conditions, e.g. 2D vs 3D cultures (Hulikova et al., 2011). In CRC cells, we found that the Na+/HCO3- cotransporter, although present, provides only a weak contribution to the integrin-dependent alkalinization in CRC cells. In fact, NHE1 appears to constitute the main molecular device linking the ECM microenvironment to pHi regulation.
Numerous mechanisms leading to NHE1 activation have been described in the various cell types in which the transporter is expressed (Orlowski and Grinstein, 2004). Stimuli such as growth factors, peptide hormones etc., which activate receptor tyrosine kinases and G protein-coupled receptors, enhance NHE1 activity, through the involvement of the mitogen-activated, extracellular signal-related kinase (MEK-ERK)-p90rsk. The latter phosphorylates NHE1, and enables its binding to the multifunctional scaffolding protein 14-3-3, which in turn serves as a hub for the assembly of other signaling molecules which eventually enhance cation exchange (Orlowski and Grinstein, 2004). In this scenario, it is not surprising that integrins exert a stimulatory role on NHE1, as they are known to trigger intracellular signaling pathways that can lead to NHE1 activation (Putney et al., 2002). Integrins, and the tumor microenviroment as a whole, can contribute to trigger a complex signaling pathway which in turn regulates NHE1-dependent motility and invasion in different cancer cells (Cardone et al., 2005). In particular, NHE1 is linked to the actin cytoskeleton and integrates phosphorylation signals arising from kinases which are involved in cytoskeletal reorganization and cell motility. In addition, NHE1 is preferentially localized in pseudopodia, focal adhesion plates, and invadopodia in migrating cells (Paradiso et al., 2004; Patel and Barber, 2005; Clement et al., 2013). In this context, a slight alkalinization mediated by NHE1 was found to regulate the cofilin-mediated actin assembly (Frantz et al., 2008), a central mechanism in cell protrusion. Hence, NHE1 and cofilin respectively act as a pH regulator and a pH sensor, to mediate actin filament assembly.
The most novel result emerging from our data is that the KV11.1 channel is implicated in the pHi alkalinization triggered by integrin-mediated cell adhesion to ECM proteins, and sustained by NHE1 activity. KV11.1 is over-expressed in many cancer types, including CRC (Lastraioli et al., 2004; Lastraioli et al., 2012; Crociani et al., 2013; Iorio et al., 2020). In cancers, β1 integrin-mediated adhesion to FN or Col-I activates KV11.1, and induces the formation of a macromolecular functional complex on the plasma membrane which comprises the channel and the integrin itself. This occurs preferentially when the channel is in the closed conformation, and leads to the activation of signaling pathways, which also involve the scaffold protein 14-3-3, and in turn control different aspects of cancer cell behavior (Becchetti et al., 2017; Becchetti et al., 2019). The recruitment of NHE1 in the KV11.1/β1 integrin complex could give rise to the formation of a signaling hub, facilitating NHE1 activation and hence a localized pHi alkalinization, which in turn could affect the reorganization of actin filaments, presumably regulated by cofilin activation. This agrees with our recent observations in pancreatic ductal adenocarcinoma cells, where KV11.1 regulates cell migration through a reorganization of f-actin in stress fibers and a modulation of filopodia formation and dynamics (Manoli et al., 2019).
The identification of the signaling mechanisms underlying KV11.1 and NHE1 interaction triggered by β1 integrin–mediated adhesion needs further experiments. Nevertheless, the interplay between a K+ channel and the pH regulating transporter NHE1 that we describe in the present paper can be considered of relevance in the context of CRC invasiveness/motility. This aspect is often dependent on a complex interaction between cancer cells and the tumor microenvironment, and in particular with ECM proteins like collagens and fibronectin (Arcangeli, 2011). Finally, targeting the integrin/ion channel/NHE1 molecular hub might represent a therapeutic option to fight cancer invasiveness.
The datasets generated for this study are available on request to the corresponding author.
JI performed the experiments, analyzed the data, prepared the figures, wrote the manuscript. CD performed the experiments and wrote the manuscript. TL performed the experiments. EL helped in the analysis of the data and reviewed the manuscript. GB contributed to experiments and figures. AB reviewed manuscript. AA designed this project and wrote the manuscript.
This work was supported by the Associazione Italiana per la Ricerca sul Cancro (AIRC, Grant N° IG 21510 and Grant N°IG 15627), by the European Union’s Horizon 2020 research and innovation programme under the Marie Skłodowska-Curie grant agreement No 813834, by PRIN 2017 (LIONESS) and by Doctorate Course in Genetics, Oncology and Clinical Medicine (Department of Medical Biotechnologies, University of Siena, Siena, Italy).
The authors declare that the research was conducted in the absence of any commercial or financial relationships that could be construed as a potential conflict of interest.
The authors thank Dr. Massimo D’Amico and Dr. Giulia Petroni for helping with pilot experiments.
The Supplementary Material for this article can be found online at: https://www.frontiersin.org/articles/10.3389/fphar.2020.00848/full#supplementary-material
Arcangeli, A., Crociani, O., Lastraioli, E., Masi, A., Pillozzi, S., Becchetti, A. (2009). Targeting ion channels in cancer: a novel frontier in antineoplastic therapy. Curr. Med. Chem. 16, 66–93. doi: 10.2174/092986709787002835
Arcangeli, A. (2011). Ion channels and transporters in cancer. 3. Ion channels in the tumor cell-microenvironment cross talk. Am. J. Physiol. Cell Physiol. 301, C762–C771. doi: 10.1152/ajpcell.00113.2011
Bauer, C. K., Schwarz, J. R. (2018). Ether-à-go-go K+ channels: effective modulators of neuronal excitability. J. Physiol. 596, 769–783. doi: 10.1113/JP275477
Becchetti, A., Crescioli, S., Zanieri, F., Petroni, G., Mercatelli, R., Coppola, S., et al. (2017). The conformational state of hERG1 channels determines integrin association, downstream signaling, and cancer progression. Sci. Signal. 10, eaaf3236. doi: 10.1126/scisignal.aaf3236
Becchetti, A., Petroni, G., Arcangeli, A. (2019). Ion Channel Conformations Regulate Integrin- Dependent Signaling. Trends Cell Biol. 4, 298–307. doi: 10.1016/j.tcb.2018.12.005
Benoit, Y. D., Larrivée, J. F., Groulx, J. F., Stankova, J., Vachon, P. H., Beaulieu, J. F. (2010). Integrin α8β1 confers anoikis susceptibility to human intestinal epithelial crypt cells. Biochem. Biophys. Res. Commun. 399, 434–439. doi: 10.1016/j.bbrc.2010.07.107
Bose, T., Cieślar-Pobuda, A., Wiechec, E. (2015). Role of ion channels in regulating Ca2+ homeostasis during the interplay between immune and cancer cells. Cell Death Dis. 6, e1648. doi: 10.1038/cddis.2015.23
Boudjadi, S., Carrier, J., Groulx, J., Beaulieu, J. F. (2016). Integrin α1β1 expression is controlled by c-MYC in colorectal cancer cells. Oncogene 35, 1671– 1678. doi: 10.1038/onc.2015.231
Busco, G., Cardone, R. A., Greco, M. R., Bellizzi, A., Colella, M., Antelmi, E., et al. (2010). NHE1 promotes invadopodial ECM proteolysis through acidification of the periinvadopodial space. FASEB J. 24, 3903–3915. doi: 10.1096/fj.09-149518
Cardone, R. A., Casavola, V., Reshkin, S. J. (2005). The role of disturbed pH dynamics and the Na+/H+ exchanger in metastasis. Nat. Rev. Cancer 5, 786–795. doi: 10.1038/nrc1713
Choi, S. H., Kim, M. Y., Yoon, Y. S., Koh, D. I., Kim, M. K., Cho, S. Y., et al. (2019). Hypoxia-induced RelA/p65 derepresses SLC16A3 (MCT4) by downregulating ZBTB7A. Biochim. Biophys. Acta Gene Regul. Mech. 1862, 771–785. doi: 10.1016/j.bbagrm.2019.06.004
Christenheit, A., Heffeter, P., Selzer, E. (2016). A Novel Small-Molecule Integrin Antagonist Inhibits Cells Adhesion Followed By Anoikis in Endothelial Cells - A Comparative Analysis with Cilengitide. Global J. Cancer Ther. 2, 009–018. doi: 10.17352/XXXX-XXXX.000008
Clement, D. L., Mally, S., Stock, C., Lethan, M., Satir, P., Schwab, A., et al. (2013). PDGFRα signaling in the primary cilium regulates NHE1-dependent fibroblast migration via coordinated differential activity of MEK1/2-ERK1/2-p90RSK and AKT signaling pathways. J. Cell Sci. 126, 953–965. doi: 10.1242/jcs.116426
Crociani, O., Guasti, L., Balzi, M., Becchetti, A., Wanke, E., Olivotto, M., et al. (2003). Cell cycle-dependent expression of HERG1 and HERG1B isoforms in tumor cells. J. Biol. Chem. 278, 2947–2955. doi: 10.1074/jbc.M210789200
Crociani, O., Zanieri, F., Pillozzi, S., Lastraioli, E., Stefanini, M., Fiore, A., et al. (2013). hERG1 channels modulate integrin signaling to trigger angiogenesis and tumor progression in colorectal cancer. Sci. Rep. 3, 3308. doi: 10.1038/srep03308
Crociani, O., Lastraioli, E., Boni, L., Pillozzi, S., Romoli, M. R., D’Amico, M., et al. (2014). hERG1 channels regulate VEGF-A secretion in human gastric cancer: clinicopathological correlations and therapeutical implications. Clin. Cancer Res. 20, 1502–1512. doi: 10.1158/1078-0432.CCR-13-2633
Demaurex, N., Downey, G. P., Waddell, T. K., Grinstein, S. (1996). Intracellular pH regulation during spreading of human neutrophils. J. Cell Biol. 133, 1391–1402. doi: 10.1083/jcb.133.6.1391
Eble, J. A., Tuckwell, D. S. (2003). The alpha2beta1 integrin inhibitor rhodocetin binds to the A-domain of the integrin alpha2 subunit proximal to the collagen-binding site. Biochem. J. 376, 77–85. doi: 10.1042/bj20030373
Frantz, C., Barreiro, G., Dominguez, L., Chen, X., Eddy, R., Condeelis, J., et al. (2008). Cofilin is a pH sensor for actin free barbed end formation: role of phosphoinositide binding J. Cell Biol. 183, 865–879. doi: 10.1083/jcb.200804161
Gadsby, D. C. (2009). Ion channels versus ion pumps: the principal difference, in principle. Nat. Rev. Mol. Cell Biol. 10, 344–352. doi: 10.1038/nrm2668
Gorbatenko, A., Olesen, C. W., Boedtkjer, E., Pedersen, S. F. (2014). Regulation and roles of bicarbonate transporters in cancer. Front. Physiol. 5, 130. doi: 10.3389/fphys.2014.00130
Gout, S. P., Jacquier-Sarlin, M. R., Rouard-Talbot, L., Rousselle, P., Block, M. R. (2001). RhoA-dependent switch between alpha2beta1 and alpha3beta1 integrins is induced by laminin-5 during early stage of HT-29 cell differentiation. Mol. Biol. Cell. 12, 3268–3281. doi: 10.1091/mbc.12.10.3268
Grant, R. L., Acosta, D. (1997). Ratiometric measurement of intracellular pH of cultured cells with BCECF in a fluorescence multi-well plate reader. Vitro Cell Dev. Biol. Anim. 33, 256. doi: 10.1007/s11626-997-0044-z
Grinstein, S., Woodside, M., Waddell, T. K., Downey, G. P., J Orlowski, J., Pouyssegur, J., et al. (1993). Focal localization of the NHE-1 isoform of the Na+/H+ antiport: assessment of effects on intracellular pH. EMBO J. 1993. 12, 5209–5218. doi: 10.1002/j.1460-2075.1993.tb06216.x
Hallowes, R. C., Bone, E. J., Jones, W. (1980). A new dimension in the culture in the human breast, in Tissue culture in Medical research, vol. 2. Eds. Richards, R. J., Rajan, K. T. (Pergamon Oxford), 215–244.
Harguindey, S., Orive, G., Luis Pedraz, J., Paradiso, A., Reshkin, S. J. (2005). The role of pH dynamics and the Na+/H+antiporter in the etiopathogenesis and treatment of cancer. Two faces of the some coin — one single nature. Biochim. Biophys. Acta 1756, 1–24. doi: 10.1016/j.bbcan.2005.06.004
Hofmann, G., Bernabei, P. A., Crociani, O., Cherubini, A., Guasti, L., Pillozzi, S., et al. (2001). HERG K+ Channels Activation during β1Integrin-mediated Adhesion to Fibronectin Induces an Up-regulation of αvβ3 Integrin in the Preosteoclastic Leukemia Cell Line FLG 29.1. JBC 276, 4923–4931. doi: 10.1074/jbc.M005682200
Huang, X., Jan, L. Y. (2014). Targeting potassium channels in cancer. J. Cell Biol. 206, 151–162. doi: 10.1083/jcb.201404136
Hulikova, A., Vaughan-Jones, R. D., Swietach, P. (2011). Dual role of CO2/HCO3- buffer in the regulation of intracellular pH of three-dimensional tumor growths. J. Biol. Chem. 286, 13815– 13826. doi: 10.1074/jbc.M111.219899
Hulikova, A., Harris, A. L., Vaughan-Jones, R. D., Swietach, P. (2013). Regulation of intracellular pH in cancer cell lines under normoxia and hypoxia. J. Cell Physiol. 228, 743–752. doi: 10.1002/jcp.24221
Iorio, J., Lastraioli, E., Tofani, L., Petroni, G., Antonuzzo, L., Messerini, L., et al. (2020). hERG1 and HIF-2α Behave as Biomarkers of Positive Response to Bevacizumab in Metastatic Colorectal Cancer Patients. Transl. Oncol. 13, 100740. doi: 10.1016/j.tranon.2020.01.001
Ito, H., Rocha, F. G., Duxbury, M., Zinner, M. J., Ashley, S. W., Whang, E. E. (2004). Fibronectin induces colon cancer MMP-2 activation through a Src- and NfκB-dependent mechanism. J. Surg. Res. 121, 299. doi: 10.1016/j.jss.2004.07.106
Jin, P., Jiang, J., Xie, N., Zhou, L., Huang, Z., Zhang, L., et al. (2019). MCT1 relieves osimertinib-induced CRC suppression by promoting autophagy through the LKB1/AMPK signaling. Cell Death Dis. 10, 615. doi: 10.1038/s41419-019-1844-2
Kim, E. J., Kwon, K. A., Lee, Y. E., Kim, J. H., Kim, S. H., Kim, J. H. (2018). Red Ginseng extract reduces hypoxia-induced epithelial-mesenchymal transition by repressing NF-κB and ERK1/2 pathways in colon cancer. J. GINSENG Res. 42, 288–297. doi: 10.1016/j.jgr.2017.03.008
Lastraioli, E., Guasti, L., Crociani, O., Polvani, S., Hofmann, G., Witchel, H., et al. (2004). herg1 gene and HERG1 protein are overexpressed in colorectal cancers and regulate cell invasion of tumor cells. Cancer Res. 64 (2), 606–611. doi: 10.1158/0008-5472.can-03-2360
Lastraioli, E., Bencini, L., Bianchini, E., Romoli, M. R., Crociani, O., Giommoni, E., et al. (2012). hERG1 Channels and Glut-1 as Independent Prognostic Indicators of Worse Outcome in Stage I and II Colorectal Cancer: A Pilot Study. Transl. Oncol. 5, 105–112. doi: 10.1593/tlo.11250
Lastraioli, E., Perrone, G., Sette, A., Fiore, A., Crociani, O., Manoli, S., et al. (2015a). b. hERG1 channels drive tumour malignancy and may serve as prognostic factor in pancreatic ductal adenocarcinoma. Br. J. Cancer 112, 1076–1087. doi: 10.1038/bjc.2015.28
Lastraioli, E., Iorio, J., Arcangeli, A. (2015b). a. Ion channel expression as promising cancer biomarker. Biochim. Biophys. Acta 1848, 2685–2702. doi: 10.1016/j.bbamem.2014.12.016
Lehenkari, P. P., Horton, M. A. (1999). Single integrin molecule adhesion forces in intact cells measured by atomic force microscopy. Biochem. Biophys. Res. Commun. 259, 645–650. doi: 10.1006/bbrc.1999.0827
Ludwig, F. T., Schwab, A., Stock, C. (2013). The Na+/H+ -exchanger (NHE1) generates pH nanodomains at focal adhesions. J. Cell. Physiol. 228, 1351–1358. doi: 10.1002/jcp.24293
Manoli, S., Coppola, S., Duranti, C., Lulli, M., Magni, L., Kuppalu, N., et al. (2019). The Activity of Kv 11.1 Potassium Channel Modulates F-Actin Organization During Cell Migration of Pancreatic Ductal Adenocarcinoma Cells. Cancers (Basel). 11, 135. doi: 10.3390/cancers11020135
Martìn-Padura, I., Bazzoni, G., Zanetti, A., Bernasconi, S., Elices, M. J., Mantovani, A., et al. (1994). A novel mechanism of colon carcinoma cell adhesion to the endothelium triggered by beta 1 integrin chain. J. Biol. Chem. 269, 6124–6132.
Masereel, B., Pochet, L., Laeckmann, D. (2003). An overview of inhibitors of Na(+)/H(+) exchanger. Eur. J. Med. Chem. 38, 547–554. doi: 10.1016/s0223-5234(03)00100-4
Masi, A., Becchetti, A., Restano-Cassulini, R., Polvani, S., Hofmann, G., Buccoliero, A. M., et al. (2005). hERG1 channels are overexpressed in glioblastoma multiforme and modulate VEGF secretion in glioblastoma cell lines. Br. J. Cancer 93, 781–792. doi: 10.1038/sj.bjc.6602775
Mboge, M. Y., Mahon, B. P., McKenna, R., Frost, S. C. (2018). Carbonic Anhydrases: Role in pH Control and Cancer. Metabolites 8, 19. doi: 10.3390/metabo8010019
McCarty, M. F., Whitaker, J. (2010). Manipulating tumor acidification as a cancer treatment strategy. Altern. Med. Rev. 15, 264–272.
McIntyre, A., Patiar, S., Wigfield, S., Li, J. L., Ledaki, I., Turley, H., et al. (2012). Carbonic anhydrase IX promotes tumor growth and necrosis in vivo and inhibition enhances anti-VEGF therapy. Clin. Cancer Res. 18, 3100–3111. doi: 10.1158/1078-0432.CCR-11-1877
Orlowski, J., Grinstein, S. (2004). Diversity of the mammalian sodium/proton exchanger SLC9 gene family. Pflugers Arch. 447, 549–565. doi: 10.1007/s00424-003-1110-3
Paradiso, A., Cardone, R. A., Bellizzi, A., Bagorda, A., Guerra, L., Tommasino, M., et al. (2004). The Na+–H+ exchanger-1 induces cytoskeletal changes involving reciprocal RhoA and Rac1 signaling, resulting in motility and invasion in MDA-MB-435 cells. Breast Cancer Res. 6, R616–R628. doi: 10.1186/bcr922
Parkkila, S., Rajaniemi, H., Parkkila, A. K., Kivela, J., Waheed, A., Pastorekova, S., et al. (2000). Carbonic anhydrase inhibitor suppresses invasion of renal cancer cells in vitro. Proc. Natl. Acad. Sci. U. S. A. 97, 2220–2224. doi: 10.1073/pnas.040554897
Patel, H., Barber, D. L. (2005). A developmentally regulated Na–H exchanger in Dictyostelium discoideum is necessary for cell polarity during chemotaxis. J. Cell Biol. 169, 321–329. doi: 10.1083/jcb.200412145
Pelillo, C., Bergamo, A., Mollica, H., Bestagno, M., Sava, G. (2015). Colorectal Cancer Metastases Settle in the Hepatic Microenvironment Through α5β1 Integrin. J. Cell Biochem. 116, 2385–2396. doi: 10.1002/jcb.25189
Persi, E., Duran-Frigola, M., Damaghi, M., Roush, W. R., Aloy, P., Cleveland, J. L., LQ., et al. (2018). System analysis of intracellular pH vulnerabilities for cancer therapy. Nat. Commun. 9, 2997. doi: 10.1038/s41467-018-05261-x
Pillozzi, S., Arcangeli, A. (2009). “Physical and Functional Interaction between Integrins and hERG1 Channels in Cancer Cells,” in Integrins and Ion Channels: Molecular Complexes and Signaling. Ed. Becchetti, A., Arcangeli, A (Berlin, Germany: Landes Bioscience and Springer Science+Business Media).
Pillozzi, S., D’Amico, M., Bartoli, G., Gasparoli, L., Petroni, G., Crociani, O., et al. (2018). The combined activation of KCa3.1 and inhibition of Kv11.1/hERG1 currents contribute to overcome Cisplatin resistance in colorectal cancer cells. Br. J. Cancer 118, 200–212. doi: 10.1038/bjc.2017.392
Pinheiro, C., Longatto-Filho, A., Azevedo-Silva, J., Casal, M., Schmitt, F. C., Baltazar, F. (2012). Role of monocarboxylate transporters in human cancers: state of the art. J. Bioenerg. Biomembr. 44, 127. doi: 10.1007/s10863-012-9428-1
Plopper, G. E., McNamee, H. P., Dike, L. E., Bojanowski, K., Ingber, D. E. (1995). Convergence of integrin and growth factor receptor signaling pathways within the focal adhesion complex. Mol. Biol. Cell. 6, 1349–1365. doi: 10.1091/mbc.6.10.1349
Putney, L. K., Denker, S. P., Barber, D. L. (2002). The changing face of the Na+/H+ exchanger, NHE1: structure, regulation, and cellular actions. Annu. Rev. Pharmacol. Toxicol. 42, 527–552. doi: 10.1146/annurev.pharmtox.42.092001.143801
Schmidt, R., Streit, M., Kaiser, R., Herzberg, F., Schirner, M., Schramm, K., et al. (1998). De novo expression of the α5β1-fibronectin receptor in HT29 colon-cancer cells reduces activity of c-src. Increase of c-src activity by attachment on fibronectin. Int. J. Cancer 76, 91–98. doi: 10.1002/(sici)1097-0215(19980330)76:1<91::aid-ijc15>3.0.co;2-j
Schwartz, M. A., Both, G., Lechene, C. (1989). Effect of cell spreading on cytoplasmic pH in normal and transformed fibroblasts. Proc. Natl. Acad. Sci. U. S. A. 86, 4525–4529. doi: 10.1073/pnas.86.12.4525
Schwartz, M. A., Ingber, D. E., Lawrence, M., Springer, T. A., Lechene, C. (1991). Share the Ability to Induce Elevation of Intracellular pH. Exp. Cell Res. 195, 533–535. doi: 10.1016/0014-4827(91)90407-L
Sennoune, S. R., Luo, D., Martinez-Zaguilán, R. (2004). Plasmalemmal vacuolar-type H+-ATPase in cancer biology. Cell Biochem. Biophys. 40, 185. doi: 10.1385/CBB:40:2:18
Sharma, M., Astekar, M., Soi, S., Manjunatha, B. S., Shetty, D. C., Radhakrishnan, R. (2015). pH Gradient Reversal: An Emerging Hallmark of Cancers. Recent Patents Anti-Cancer Drug Discov. 10, 244–258. doi: 10.2174/1574892810666150708110608
Srivastava, J., Barreiro, G., Groscurth, S., Gingras, A. R., Goult, B. T., Critchley, D. R., et al. (2008). Structural model and functional significance of pH-dependent talin–actin binding for focal adhesion remodeling J. PNAS 105, 14436–14441. doi: 10.1073/pnas.0805163105
Stock, C., Pedersen, S. (2017). Roles of pH and the Na+/H+ exchanger NHE1 in cancer: From cell biology and animal models to an emerging translational perspective? Semin. Cancer Biol. 43, 5–16. doi: 10.1016/j.semcancer.2016.12.001
Stock, C., Schwab, A. (2006). Role of the Na/H exchanger NHE1 in cell migration. Acta Physiol. (Oxf) 187, 149–157. doi: 10.1111/j.1748-1716.2006.01543.x
Stock, C., Schwab, A. (2009). Protons make tumor cells move like clockwork. Pflugers Arch. 458, 981–992. doi: 10.1007/s00424-009-0677-8
Stock, C., Gassner, B., Hauck, C. R., Arnold, H., Mally, S., Eble, J. A., et al. (2005). Migration of human melanoma cells depends on extracellular pH and Na+/H+ exchange. J. Physiol. 567, 225–238. doi: 10.1113/jphysiol.2005.088344
Stock, C., Cardone, R. A., Busco, G., Krahling, H., Schwab, A., Reshkin, S. J. (2008). Protons extruded by NHE1: digestive or glue? Eur. J. Cell Biol. 87, 591–599. doi: 10.1016/j.ejcb.2008.01.007
Webb, B. A., Chimenti, M., Jacobson, M. P., Barber, D. L. (2011). Dysregulated pH: a perfect storm for cancer progression. Nat. Rev. Cancer 11, 671–677. doi: 10.1038/nrc3110
Keywords: hERG1, integrins, Collagen I, beta 1 integrin subunit, cariporide, lateral motility
Citation: Iorio J, Duranti C, Lottini T, Lastraioli E, Bagni G, Becchetti A and Arcangeli A (2020) KV11.1 Potassium Channel and the Na+/H+ Antiporter NHE1 Modulate Adhesion-Dependent Intracellular pH in Colorectal Cancer Cells. Front. Pharmacol. 11:848. doi: 10.3389/fphar.2020.00848
Received: 28 February 2020; Accepted: 22 May 2020;
Published: 10 June 2020.
Edited by:
Lin-Hua Jiang, University of Leeds, United KingdomCopyright © 2020 Iorio, Duranti, Lottini, Lastraioli, Bagni, Becchetti and Arcangeli. This is an open-access article distributed under the terms of the Creative Commons Attribution License (CC BY). The use, distribution or reproduction in other forums is permitted, provided the original author(s) and the copyright owner(s) are credited and that the original publication in this journal is cited, in accordance with accepted academic practice. No use, distribution or reproduction is permitted which does not comply with these terms.
*Correspondence: Annarosa Arcangeli, YW5uYXJvc2EuYXJjYW5nZWxpQHVuaWZpLml0
Disclaimer: All claims expressed in this article are solely those of the authors and do not necessarily represent those of their affiliated organizations, or those of the publisher, the editors and the reviewers. Any product that may be evaluated in this article or claim that may be made by its manufacturer is not guaranteed or endorsed by the publisher.
Research integrity at Frontiers
Learn more about the work of our research integrity team to safeguard the quality of each article we publish.