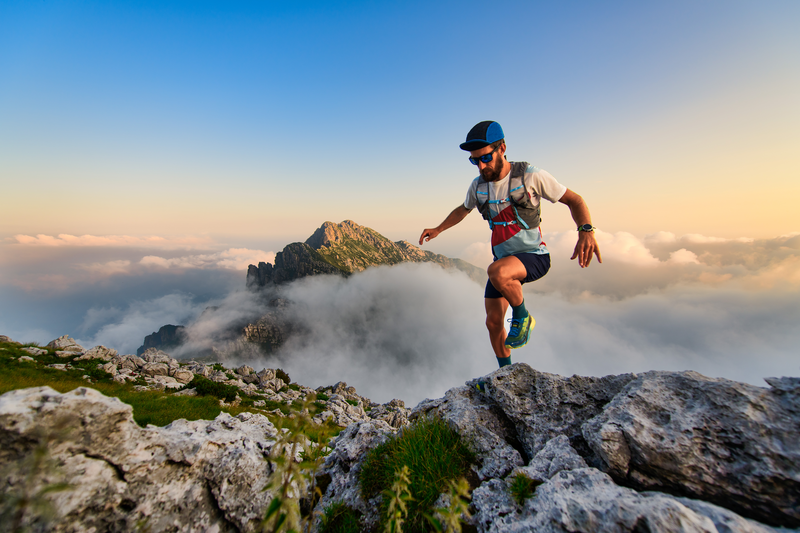
95% of researchers rate our articles as excellent or good
Learn more about the work of our research integrity team to safeguard the quality of each article we publish.
Find out more
ORIGINAL RESEARCH article
Front. Pharmacol. , 05 June 2020
Sec. Cardiovascular and Smooth Muscle Pharmacology
Volume 11 - 2020 | https://doi.org/10.3389/fphar.2020.00821
Previous studies showed that berberine, an alkaloid from Coptis Chinensis Franch, might exert a positive inotropic effect on the heart. However, the underlying mechanisms were unclear. Here, we reported that berberine at 10–20 µM increased the left ventricular (LV) developed pressure and the maximal rate of the pressure rising, and it increased the maximal rate of the pressure descending at 20 µM in Langendorff-perfused isolated rat hearts. These effects diminished with the concentration of berberine increasing to 50 µM. In the concentration range of 50–300 µM, berberine increased the isometric tension of isolated left ventricular muscle (LVM) strips with or without electrical stimulations, and it (30–300 µM) also increased the intracellular Ca2+ level in the isolated LV myocytes. The removal of extracellular Ca2+ hindered the berberine-induced increases in the tension of LVM strips and the intracellular Ca2+ level of LV myocytes. These suggested that berberine might exert its positive inotropic effects via enhancing Ca2+ influx. The blockade of L-type Ca2+ channels (LTCCs) with nifedipine significantly attenuated 300 μM berberine-induced tension increase in LVM strips but not the increase in the intracellular Ca2+ level. Berberine (300 μM) further increased the LVM tension following the treatment with the LTCC opener FPL-64716 (10 μM), indicating an LTCC-independent effect of berberine. Lowering extracellular Na+ attenuated the berberine-induced increases in both the tension of LVM strips and the intracellular Ca2+ level of LV myocytes. In conclusion, berberine might exert a positive inotropic effect on the isolated rat heart by enhancing the Ca2+ influx in LV myocytes; these were extracellular Na+-dependent.
Berberine is an alkaloid present in numerous plants, including Coptis Chinensis Franch, and has been used for the treatment of gastrointestinal infections and diarrhea in Asian countries, particularly in China. The interest in its beneficial effects on significant risk factors of cardiovascular diseases has been growing over the last two decades (Lau et al., 2001; Zeng et al., 2003; Yuan et al., 2006; Affuso et al., 2010; Sola et al., 2014).
Berberine increased the developed force in the isolated left atrium of guinea pigs by enhancing both the force-velocity relationship and the duration of the active state (Shaffer, 1985). The contractile capability of the left ventricle is critical for heart function. As the left ventricle contracts, the pressure rises to a level that is high enough to pump blood into systemic circulation through the aorta (Bombardini, 2005). However, there is no study about the berberine effect on the LV contractility, which is vital for the pumping capability of the heart, and the underlying mechanisms by which berberine affects the left ventricle remains to be determined.
In LV myocytes, the Ca2+ entry through LTCCs triggers Ca2+ release from the sarcoplasmic reticulum into the cytoplasm. These consequently increase the intracellular Ca2+ level and cause LV myocytes shortening, which provides the force for the ejection of blood (Bers, 2002; Eisner et al., 2017; Gilbert et al., 2020). In addition to the Ca2+ influx through LTCCs, Ca2+ also enters the cell through an LTCC-independent pathway (Kiang and Smallridge, 1994; Tang et al., 1998). The Na+/Ca2+ exchanger (NCX) is the dominant transporter mediating Ca2+ efflux (Blaustein and Lederer, 1999; Philipson and Nicoll, 2000; Bers, 2002). The Na+ influx during the early phase of an action potential induces Ca2+ influx via the reverse mode of NCX, which consequently induces the Ca2+ release from the sarcoplasmic reticulum and the elevation of intracellular Ca2+ level (Larbig et al., 2010; Yan et al., 2015).
In this study, we utilized Langendorff-perfused heart recordings, isometric tension recordings, and confocal microscopy techniques to investigate the effects of berberine on the LV contractility and the underlying mechanisms. Our study revealed that berberine elevated the intracellular Ca2+ level of LV myocytes via increasing Ca2+ influx. It consequently increased the LV contractility. These were extracellular Na+-dependent.
We carried out the animal procedures following The Guide for the Care and Use of Laboratory Animals of the National Institutes of Health, and the Institutional Animal Ethics Committee of Southwest University approved all the procedures.
Adult male Sprague-Dawley rats (200–300 g) were purchased from Da Ping Hospital (Chongqing, China, SCXK 2017-0005) and were housed at Southwest University (Chongqing, China, SYXK 2014-0002). All rats were exposed to 12/12 h light/dark cycles at room temperature (24 ± 2°C) and were free to food and water. Rats were euthanized by CO2 inhalation or sodium pentobarbital injection (60 mg/kg body weight, ip), and the hearts were then quickly removed from the thoracic cavity by thoracotomy.
Langendorff-perfused isolated heart recordings were carried out as previously described (Carre et al., 2014; Tilley et al., 2014). Briefly, rats were anesthetized with sodium pentobarbital (60 mg/kg body weight, ip), and the heart was rapidly excised by thoracotomy and then cannulated through the aorta. The isolated heart was mounted on a Langendorff apparatus (DMT MyoHEART 900MH System, Aarhus, Denmark) under a constant perfusion pressure (70 mmHg) and perfused with Krebs-Hensseleit (K-H) buffer saturated with a 95% O2/5% CO2 mixture, yielding a final pH of 7.4 at 37°C. For assessment of the performance of the left ventricle, a balloon connected to a pressure transducer was inserted into the left ventricle. It was used to set the left ventricular end-diastolic pressure (LVEDP) at 5–10 mmHg. After a 30-min stabilization period, berberine was added to the perfusion buffer with accumulative concentrations. Left ventricular systolic pressure (LVSP), LVEDP, LVDP (defined as LVDP = LVSP—LVEDP), the maximal rates of LVDP rising and descending during isovolumetric contraction and relaxation (+dp/dtmax and |−dp/dt|max) were analyzed with LabChart 8.1.5 (ADInstruments, Australia).
Isometric tension recordings in LVM strips were performed as previously described (Uhl et al., 2015). Briefly, the isolated hearts were transferred to the oxygenated K-H buffer containing 30 mM 2,3-butanedione monoxime (pH 7.4) at 4°C. LVM strips of 1–2 mm width, 1–1.5 mm thickness, and 6–8 mm length were prepared and mounted between metal clips attached to an anchoring hook and a force transducer in temperature-controlled (37°C) chambers filled with 10 ml K-H buffer and continuously bubbled with a 95% O2/5% CO2 mixture (DMT 750BTOS Tissue Organ bath system, Aarhus, Denmark). The strips were electrically driven with rectangular pulses (2 ms, 0.1 V, 1 Hz) using a CS4 stimulator (DMT instrument, Denmark). Pre-load was applied until the tension reached 5 mN, and then LVM strips were washed with fresh K-H buffer every 15 min during an equilibration period of 1 h. The contraction of LVM strips was induced by epinephrine (10 μM) or EFS (5 V, 1 Hz). Each berberine-treated strip had its vehicle control strip from the same ventricle.
The LV myocytes of a rat were isolated as described previously (Carre et al., 2014; Roth et al., 2014). Briefly, the isolated hearts were rapidly excised, cannulated through the aorta and retrograde perfused (5 ml/min, 37°C) with oxygenated Ca2+-free Tyrode's buffer using a Langendorff system (LGF-2C, Chengdu instrument, China). The heart was then perfused with nominally Tyrode's buffer containing collagenase II (0.17 mg/ml), collagenase IV (0.10 mg/ml), bovine serum albumin (1.0 mg/ml), and 50 μM CaCl2 until it became soft and spongy. The myocytes were mechanically dispersed and stored in the Kraft-Brühe (K-B) buffer. The concentration of Ca2+ was raised from 0.2 mM to 1.5 mM using a 4-step ladder. The freshly isolated cardiomyocytes were stored at room temperature (25°C) for Ca2+ imaging.
The intracellular Ca2+ levels were monitored using a fluorescent calcium probe Fluo4-AM, as previously described (Chen et al., 2014). Briefly, freshly isolated cardiomyocytes were seeded in a dish coated with laminin and incubated for 30 min at 37°C to adhere to the bottom of the dish. The supernatant was removed, and cells were washed three times with a modified K-H buffer, and then the cells were incubated with 5 μM Fluo4-AM in the dark for 30 min; the Fluo4-AM-containing buffer was then removed, and cells were washed three times with a modified K-H buffer. The LV myocytes were imaged with the Nikon A1+ confocal microscope (Nikon, Tokyo, Japan) equipped with a 20X objective. The fluorescence intensity was detected with a 525 ± 25 nm filter, and the excitation wavelength was 488 nm. All Ca2+-imaging experiments were carried out at room temperature.
Berberine chloride and sodium pentobarbital were purchased from Sigma-Aldrich (St. Louis, MO, the United States), and epinephrine bitartrate was from Adamas (Shanghai, China), and they were dissolved in deionized water. Nifedipine (Sigma-Aldrich, St. Louis, MO, the United States), FPL-64716 (Aladdin, Shanghai, China), and Fluo4-AM (Dojindo, Kyushu, Japan) were dissolved in DMSO, and the final concentration of DMSO in the buffer did not exceed 0.1%. All other chemicals were purchased from Sigma-Aldrich (St. Louis, MO, the United States).
The K-H buffer for Langendorff-perfused rat heart recording was prepared freshly and contained the following (in mM): NaCl 119.0, NaHCO3 25.0, KCl 4.6, D-(+)-glucose 11.0, CaCl2·2H2O 1.5, MgSO4·7H2O 1.6, and KH2PO4 1.2.
The K-H buffer for isometric tension recording experiment contained the following (in mM): NaCl 119.0, NaHCO3 25.0, KCl 4.6, D-(+)-glucose 11.0, CaCl2·2H2O 1.5, MgSO4·7H2O 1.6, KH2PO4 1.2, and sodium pyruvate 1.0.
The Ca2+-free K-H buffer was K-H buffer, excluding CaCl2·2H2O and containing 1 mM ethylene glycol-bis (2-aminoethylether)-N, N, N', N'-tetraacetic acid.
The Cs+-containing Na+-free K-H buffer was prepared freshly and contained the following (in mM): CsCl 119.0, CsHCO3 25.0, KCl 5.8, D-(+)-glucose 11.0, CaCl2·2H2O 1.5, and MgCl2 1.6.
The N-methyl-D-glucamine (NMDG)-containing Na+-free K-H buffer contained (in mM): NMDG 119.0, CsHCO3 25.0, KCl 5.8, D-(+)-glucose 11.0, CaCl2·2H2O 1.5, and MgCl2 1.6.
The pH of all buffers was adjusted to 7.40 with a 95% O2/5% CO2 mixture except those explicitly indicated.
The nominally Ca2+-free Tyrode's buffer for the isolation of LV myocytes contained the following (in mM): NaCl 140.0, KCl 5.4, MgCl2 1.0, D-(+)-glucose 10.0, and 4-(2-hydroxyethyl)-1-piperazinethanesulfonic acid (HEPES) 10.0; pH was adjusted to 7.35 with NaOH.
The K-B buffer for maintaining isolated LV myocytes contained the following (in mM): KOH 80.0, KCl 40.0, L-glutamic acid 50.0, KH2PO4 25.0, taurine 20.0, HEPES 10.0, ethylene glycol-bis (2-aminoethylether)-N, N, N', N'-tetraacetic acid 0.1, and MgSO4 3.0, D-(+)-glucose 10.0; pH was adjusted to 7.40 with KOH.
The modified K-H buffer for Ca2+ imaging experiments contained the following (in mM): NaCl 125.0, KCl 4.0, CaCl2 1.5, MgCl2 1.0, D-(+)-glucose 10.0, HEPES 25.0, and NaH2PO4 1.2; the Ca2+-free modified K-H buffer was the modified K-H buffer excluding CaCl2·2H2O and containing 1 mM ethylene glycol-bis (2-aminoethylether)-N, N, N', N'-tetraacetic acid; the low Na+ modified K-H buffer contained the following (in mM): NaCl 62.5, NMDG 62.5, KCl 4.0, CaCl2 1.5, MgCl2 1.0, D-(+)-glucose 1.0, HEPES 25.0, and NaH2PO4 1.2; pH was adjusted to 7.30 with 12 mM HCl.
All buffers were filtered through a membrane with a pore size of 0.22 µm.
LabChart 8.1.5 (ADInstruments, Australia) was used to analyze the parameters of perfused heart function and developed tension. NIS-Elements 4.3 (Nikon, Japan) and Clampfit 10.5 (Molecular Devices, the United States) was used to analyze the intracellular Ca2+ level. The 5-min recordings before each addition of berberine were analyzed. The parameters were normalized to the recording before the first addition of berberine (taken as 100%) in Langendorff-perfused heart recordings. The isolated LVM strip tension in the 5-min recording before the addition of any compound was taken as the basal level (0%), and the effect of vehicle, berberine, or inhibitors was normalized to the basal level. The average fluorescence intensity in the last 3 min of the 15-min perfusion of vehicle, berberine, or inhibitors was analyzed and normalized to that of the last 3-min recording with the modified K-H buffer (taken as 100%). Statistical analysis was performed with GraphPad Prism 7.02 (GraphPad Software, La Jolla, CA). All values are summarized as means ± SEM; n = the number of cells, LVM strips, and N = the number of rats. Statistical significance was performed with the two-way analysis of variance (ANOVA) with Sidak's multiple comparisons test or Tukey's multiple comparisons test, or the unpaired Student's t-test.
The Langendorff-perfused isolated-heart recordings provide information on heart functions without interference from the sympathetic nervous system (Bell et al., 2011). The isolated hearts were perfused for at least 30 min until they reached a stable state before the addition of berberine in the buffer (Figure 1A). The left ventricular pressure (LVP) wasW continuously recorded for at least 30 min at each concentration of berberine until it reached a stable level, which was defined as the variation of LVP amplitude was less than 10% within 5 min.
Figure 1 Berberine increased the capability of Langendorff-perfused isolated rat hearts for contraction. (A) An original recording showing that the effect of berberine on left ventricular pressure (LVP) of an isolated rat heart. (B–D) Summary data showing the effects of berberine on LVDP, +dp/dtmax and |−dp/dt|max, respectively. The numbers of repeats are as follows: vehicle control group, N = 6; Ber-treated group, N = 6; N is the number of rats. Ber stands for berberine. *p < 0.05, **p < 0.01 vs. vehicle control group; two-way ANOVA with Sidak's multiple comparisons test.
The LVDP, +dp/dtmax and |−dp/dt|max are important parameters used for the evaluation of heart contractility (Jiang et al., 2014; Tilley et al., 2014; Huang et al., 2019). These three parameters increased following the perfusion of berberine (10–20 µM) while they decreased with time in the absence of berberine (Figures 1B–D). Following the perfusion of berberine (10 µM), LVDP, +dp/dtmax, and |−dp/dt|max increased to 129.0 ± 11.4%, 112.3 ± 8.0%, and 110.0 ± 9.3% of the basal levels, respectively; they decreased with time to 84.0 ± 5.8%, 83.1 ± 4.8%, and 81.5 ± 5.8% of the basal levels in the absence of berberine, respectively. The results indicated that berberine could improve the heart function by enhancing contractility of the left ventricle. The LV contractility is crucial for heart function. Next, we investigated the effect of berberine on the contractile properties of LVM strips by using isometric tension recordings.
In the isometric tension recordings, the initial tension of the strips was set to 5 mN, which declined with time; berberine reversed the decline and further increased the tension of LVM strips in a concentration-dependent manner. The isometric tension increased by 76.4 ± 16.7% with the treatment of 100 µM berberine, while it decayed with time by 18.0 ± 4.3% in the absence of berberine (Figure 2). These suggested that berberine can increase the contractile force of LV myocardium.
Figure 2 Berberine increased the tension of freshly isolated left ventricular muscle (LVM) strips. (A, B) Original isometric tension recordings showing that berberine (100 µM) increased the tension of LVM strips. (C) Summary data showing that berberine increased the tension of LVM strips in a concentration-dependent manner. The numbers of repeats are as follows: 30 µM Ber, n = 10, N = 10; 50 µM Ber, n = 7, N = 7; 100 µM and 300 µM Ber, n = 11, N = 11; 200 µM Ber, n = 12, N = 12; n and N are the numbers of LVM strips and rats, respectively. Ber stands for berberine. **p < 0.01, ***p < 0.001 vs. vehicle control group; two-way ANOVA with Sidak's multiple comparisons test.
Epinephrine plays an essential role in the fight-or-flight response by increasing the output of the heart via the activation of β-adrenergic receptors. Under pathophysiological conditions, in the case of heart failure, the excessive activation of β-adrenergic receptors causes sustained elevation of cAMP, induces tachycardia and arrhythmic contractions, and increases cardiac workload (Galindo-Tovar and Kaumann, 2008; Johnson and Antoons, 2018). Epinephrine (10 µM) induced phasic contractions in the isolated LVM strips. In the presence of epinephrine, the tension of LVM strips decreased with time, while the amplitude of the evoked phasic contractions did not change (Figure 3A). Following epinephrine, the subsequent addition of berberine increased the tension and eliminated the evoked phasic contractions in a concentration-dependent manner (Figures 3B, C). Berberine (100 μM) increased the tension by 77.9 ± 14.4%, while it decreased by 23.1 ± 2.8% with time in the absence of berberine (Figure 3). These indicated that berberine could enhance the contractile force and eliminate the epinephrine-evoked phasic contractions in the LVM.
Figure 3 Berberine increased the tension of the freshly isolated left ventricular muscle (LVM) strips pretreated with epinephrine. (A, B) Original isometric tension recordings showing that berberine (100 µM) increased the tension and abolished the epinephrine-evoked phasic contractions in LVM strips. (C) Summary data showing that the effect of berberine on the tension of the LVM strips pretreated with epinephrine. The numbers of repeats are as follows: 30 µM and 50 µM Ber, n = 7, N = 7; 100 µM Ber, n = 8, N = 8; n and N are the numbers of LVM strips and rats, respectively. Ber stands for berberine. ***p < 0.001 vs. vehicle control group; two-way ANOVA with Sidak's multiple comparisons test.
The action potentials generated by the sinoatrial node transmit through the cardiac electrical conduction system to cause myocardial contraction (Nakao et al., 2015). The electrical field stimulations (EFS) induced phasic contractions in LVM strips, but it did not prevent the decline in the tension of LVM strips with time (Figure 4A). The addition of berberine prevented the tension decrease and further increased the tension of EFS-paced LVM strips (Figure 4B). The tension increased by 42.5 ± 21.0% following the addition of 100 μM berberine, but it decreased by 39.7 ± 5.5% with time in the absence of berberine (Figure 4). These data suggested that berberine could improve heart function by increasing the isometric tension of the ventricular muscle. The cardiac contractility relies on the intracellular Ca2+ level of the cardiomyocytes, so we next determined the effect of berberine on the intracellular Ca2+ level of LV myocytes.
Figure 4 Berberine increased the tension of the electrical field stimulation (EFS)-paced left ventricular muscle (LVM) strips. (A, B) Original isometric tension recordings showing that berberine (100 µM) increased the tension of the EFS-paced LVM strips (5 V, 1 Hz). (C) Summary data indicating that berberine increases the tension of the EFS-paced LVM strips in a concentration-dependent manner. The numbers of repeats are as follows: 30 µM Ber, n = 5, N = 5; 50 µM Ber, n = 8, N = 8; 100 µM Ber, n = 6, N = 6; 200 µM Ber and 300 µM Ber, n = 7, N = 7; n and N are the numbers of LVM strips and rats, respectively. Ber stands for berberine. ***p < 0.001 vs. vehicle control group; two-way ANOVA with Sidak's multiple comparisons test.
The increase of the intracellular Ca2+ level is the key to cardiac contractions (Bers, 2002; Eisner et al., 2017). In the range of 30–300 μM, berberine increased the intracellular Ca2+ level of freshly isolated LV myocytes (Figure 5C). The fluorescence intensity of Fluo4-Ca2+ increased to 120.8 ± 21.1% with the treatment of 100 µM berberine, while it decayed with time to 88.83 ± 10.71% in the absence of berberine (Figure 5). These results indicated the berberine might elicit the positive inotropic effect by elevating the intracellular Ca2+ level of LV myocytes.
Figure 5 Berberine concentration-dependently increased the intracellular Ca2+ level of freshly isolated left ventricular (LV) myocytes. (A, B) Original Ca2+ imaging recordings showing that berberine (100 µM) increased the intracellular Ca2+ level of freshly isolated LV myocytes. (C) Summary data showing that berberine concentration-dependently increased the intracellular Ca2+ level. The numbers of repeats are as follows: 30 µM Ber, n = 54, N = 3; 50 µM Ber, n = 65, N = 3; 100 µM Ber, n = 35, N = 3; 300 µM Ber, n = 36, N = 3; n and N are the numbers of LV myocytes and rats, respectively. Ber stands for berberine. *p < 0.05, ***p < 0.001 vs. vehicle control group; two-way ANOVA with Sidak's multiple comparisons test.
Extracellular Ca2+ influx contributes to the increase in the intracellular Ca2+ level of LV myocytes, which induces cardiac contraction (Bers, 2002; Eisner et al., 2017; Gilbert et al., 2020). To investigate the role of Ca2+ influx in the berberine-induced tension increase in LVM strips, we carried out experiments under the Ca2+-free condition (Song et al., 2014). The removal of extracellular Ca2+ significantly attenuated the berberine-induced tension increase in LVM strips (Figure 6E). In the absence of extracellular Ca2+, the tension increased by 17.7 ± 19.8% following the addition of berberine (300 µM) (Figures 6 C, D), while it increased by 121.0 ± 18.3% in the presence of extracellular Ca2+ (1.5 mM) (Figures 6 A, B). It suggested that the berberine-induced tension increase in LVM strips is extracellular Ca2+-dependent.
Figure 6 The removal of extracellular Ca2+ hindered the berberine-induced tension increase in left ventricular muscle (LVM) strips. (A–D) Original isometric tension recordings of the LVM strips treated with vehicle (A), 300 μM Ber (B); vehicle (C) and 300 μM Ber (D) under the Ca2+-free condition, respectively. (E) Summary data showing that the removal of extracellular Ca2+ significantly reduced the ability of berberine to increase the tension of LVM strips. The numbers of repeats are as follows: vehicle control of 300 µM Ber, n = 6, N = 6; 300 µM Ber, n = 6, N = 6; vehicle control of 300 µM Ber in the Ca2+-free Krebs-Hensseleit (K-H) buffer, n = 6, N = 6; 300 µM Ber in the Ca2+-free K-H buffer, n = 7, N = 7; n and N are the numbers of LVM strips and rats, respectively. Ber stands for berberine. ***p < 0.001 vs. vehicle control group; ###p < 0.001 vs. Ber-treated group in the normal K-H buffer; two-way ANOVA with Tukey's multiple comparisons test.
LTCCs are the major Ca2+ channels that allow extracellular Ca2+ influx in LV myocytes (Jaleel et al., 2008). The selective LTCC inhibitor nifedipine (10 µM) can greatly block the channels (Carre et al., 2014). Berberine (300 µM) increased the tension in LVM strips by 121.0 ± 18.3% and 63.6 ± 7.5% in the absence and presence of nifedipine (10 µM), respectively (p < 0.05, Figures 7B, D, E).
Figure 7 Nifedipine attenuated the berberine-induced tension increase in left ventricular muscle (LVM) strips. (A–D) Original isometric tension recordings of the LVM strips treated with vehicle (A), 300 μM Ber (B), 10 μM nifedipine (C), and 300 μM Ber in the presence of 10 μM nifedipine (D), respectively. (E) Summary data showing nifedipine hindered the 300 μM berberine-induced tension increase in LVM strips. The numbers of repeats are as follows: vehicle control of 300 µM Ber, n = 6, N = 6; 300 µM Ber, n = 6, N = 6; 10 μM nifedipine, n = 7, N = 7; 300 µM Ber plus 10 μM nifedipine, n = 5, N = 5; n and N are the numbers of LVM strips and rats, respectively. Ber stands for berberine. ***p < 0.001 vs. vehicle control group; #p < 0.05, vs. 300 μM Ber-treated group; two-way ANOVA with Tukey's multiple comparisons test.
The LTCC opener FPL-64716 enhances Ca2+ influx (Kang et al., 2012). FPL-64716 (10 μM) increased the tension of LVM strips by 105.1 ± 70.8%, and the subsequent addition of berberine induced a further increase by a total of 232.9 ± 56.9% (Figure 8B). It increased by 41.3 ± 29.9% in the vehicle control group (Figure 8A). These results indicated that the positive inotropic effects of berberine were LTCC-independent. To directly investigate the effect of berberine on the Ca2+ influx, we next carried out experiments with isolated LV myocytes under the Ca2+-free condition or in the presence of the LTCC blocker nifedipine.
Figure 8 Berberine increased the tension of the freshly isolated left ventricular muscle (LVM) strips pretreated with FPL-64716. (A, B) Original isometric tension recordings of the LVM strips treated with 10 µM FPL-64716 (A) and 300 μM berberine in the presence of 10 µM FPL-64716 (B). (C) Summary data showing berberine induced an additional increase in the tension of the LVM strips pretreated with FPL-64716. The numbers of repeats are as follows: 10 µM FPL-64716, n = 4, N = 3; 300 µM Ber plus 10 μM FPL-64716, n = 3, N = 3; n and N are the numbers of LVM strips and rats, respectively. Ber stands for berberine. *p < 0.05 vs. 10 µM FPL-64716-treated group; two-tailed unpaired Student's t-test.
Berberine (300 μM) increased the fluorescence intensity of Fluo4-Ca2+ of the isolated LV myocytes to 130.0 ± 2.5% in the extracellular buffer containing 1.5 mM Ca2+ (Figures 9A, B). The removal of extracellular Ca2+ significantly attenuated the berberine-induced fluorescence increase to 106.7 ± 3.3% (Figures 9C–E). These results indicated that the berberine-induced intracellular Ca2+ elevation was extracellular Ca2+-dependent and that berberine might enhance the Ca2+ influx.
Figure 9 The removal of extracellular Ca2+ hindered the berberine-induced increase in the intracellular Ca2+ level of freshly isolated LV myocytes. (A–D) Original Ca2+ imaging recordings of the LV myocytes treated with vehicle (A) and 300 μM berberine (B); vehicle (C) and 300 μM berberine (D) in the Ca2+-free modified Krebs-Hensseleit (K-H) buffer, respectively. (E) Summary data showing that the removal of extracellular Ca2+ significantly hindered the 300 μM berberine-induced increase in the intracellular Ca2+ level of LV myocytes. The numbers of repeats are as follows: vehicle control of 300 µM Ber, n = 57, N = 3; 300 µM Ber, n = 83, N = 3; vehicle control of 300 µM Ber in the Ca2+-free modified K-H buffer, n = 27, N = 3; 300 µM Ber in the Ca2+-free modified K-H buffer, n = 47, N = 3; n and N are the numbers of LV myocytes and rats, respectively. Ber stands for berberine. ***p < 0.001 vs. vehicle control group; ###p < 0.001 vs. Ber-treated group in the normal modified K-H buffer; two-way ANOVA with Tukey's multiple comparisons test.
Next, we investigated the role of LTCCs in the berberine-induced increase in the intracellular Ca2+ level. In the presence of nifedipine, berberine increased the fluorescence intensity of Fluo4-Ca2+ to 130.7 ± 3.2% of the basal level in LV myocytes. It rose to 130.0 ± 2.5% in the absence of nifedipine (Figures 10B, D). These suggested that berberine increases the intracellular Ca2+ concentration also by enhancing the Ca2+ entry via an LTCC-independent pathway. The Na+ is also critical for regulating the excitation-contraction coupling. Next, we determined the role of the extracellular Na+ in the berberine-induced tension increase in the LVM strips.
Figure 10 Nifedipine did not influence the 300 μM berberine-induced increase in the intracellular Ca2+ level of freshly isolated left ventricular (LV) myocytes. (A–D) Original Ca2+ imaging recordings of the LV myocytes treated with vehicle (A), 300 μM Ber (B), 10 μM nifedipine (C) and 300 μM Ber in the presence of 10 μM nifedipine (D). (E) Summary data showing nifedipine did not affect the 300 μM berberine-induced increase in the intracellular Ca2+ level of LV myocytes. The numbers of repeats are as follows: vehicle control of 300 µM Ber, n = 57, N = 4; 300 µM Ber, n = 83, N = 4; 10 μM nifedipine, n = 37, N = 4; 300 µM Ber plus 10 μM nifedipine, n = 46, N = 4; n and N are the numbers of LV myocytes and rats, respectively. Ber stands for berberine. ***p < 0.001 vs. vehicle control group; two-way ANOVA with Tukey's multiple comparisons test.
To determine the role of extracellular Na+ in the berberine-induced tension increase in LVM strips, Cs+, and NMDG were used to replace extracellular Na+, respectively (Xin et al., 2005). The lowering of extracellular Na+ caused a transient increase in the tension of LVM strips (Figures 11B, D). The subsequent addition of berberine induced a smaller increase in the tension of LVM strips in the Na+-free K-H buffer than that in the standard K-H buffer containing 144 mM NaCl. The berberine-induced tension increased with the concentration of Na+ rising in the buffer (Figures 11C, E). These data indicated that the extracellular Na+ is essential for the tension increase in LVM strips caused by berberine.
Figure 11 The berberine-induced tension increase in left ventricular muscle (LVM) strips was extracellular Na+-dependent. (A, B, D) Original isometric tension recordings of the LVM strips treated with 300 μM berberine (A), and 300 μM berberine under the low Na+ condition where NaCl of the Krebs-Hensseleit (K-H) buffer was replaced with CsCl (B) or with NMDG (D). (C, E) Summary data indicating that the 300 µM berberine-induced tension increase has a positive correlation with the concentrations of extracellular NaCl. The numbers of repeats are as follows: experiments with the normal K-H buffer, 300 µM Ber, n = 6, N = 6. Experiments with the K-H buffer in which NaCl was replaced with CsCl: 300 µM Ber in the buffer containing 77 or 10 mM NaCl, n = 5, N = 5; 300 µM Ber in the buffer containing 0 mM NaCl, n = 6, N = 6. Experiments with the K-H buffer in which NaCl was replaced with NMDG: 300 µM Ber in the buffer containing 77 or 0 mM NaCl, n = 6, N = 6; 300 µM Ber in the buffer containing 10 mM NaCl, n = 5, N = 5. n and N are the numbers of LVM strips and rats, respectively. Ber stands for berberine. ***p < 0.001 vs. vehicle control group; #p < 0.05, ##p < 0.01, ###p < 0.001 vs. Ber-treated group in the normal K-H buffer; two-way ANOVA with Tukey's multiple comparisons test.
To further investigate the role of extracellular Na+ in the berberine-induced tension increase in LVM strips, we examined the impact of extracellular Na+ on the berberine-induced rise in the intracellular Ca2+ level of LV myocytes. Berberine (300 μM) raised the fluorescence intensity of Fluo4-Ca2+ to 132.5 ± 18.1% of the basal level in myocytes perfused with the modified K-H buffer containing 125 mM NaCl (Figure 12B). We perfused the myocytes with a modified K-H buffer containing 62.5 mM NaCl and 62.5 mM NMDG. It caused a transient increase in the fluorescence intensity of Fluo4-Ca2+. Following a 30-min equilibration, the subsequent addition of berberine (300 μM) elevated the fluorescence intensity of Fluo4-Ca2+ to 116.2 ± 22.5% of the basal level (Figure 12D). The berberine-induced increase in the fluorescence intensity in LV myocytes was significantly less in the 62.5 mM-NaCl K-H buffer than that in the standard, modified K-H buffer containing 125 mM NaCl (Figure 12E). These data indicated that the elevation in the intracellular Ca2+ level by berberine was extracellular Na+-dependent.
Figure 12 The berberine-induced increase in the intracellular Ca2+ level of left ventricular (LV) myocytes was extracellular Na+-dependent. (A–D) Original Ca2+ imaging recordings of the LV myocytes treated with vehicle (A) and 300 μM Ber (B); vehicle (C) and 300 μM Ber (D) in the modified Krebs-Hensseleit (K-H) buffer containing 62.5 mM NaCl and 62.5 mM NMDG, respectively. (B) Summary data indicating that the berberine-induced increase in the intracellular Ca2+ level in a buffer containing 62.5 mM NaCl was smaller than that in a buffer containing 125 mM NaCl. The numbers of repeats are as follows: vehicle control of 300 µM Ber, n = 42, N = 3; 300 µM Ber, n = 58, N = 3; vehicle control of 300 µM Ber in the K-H buffer containing 62.5 mM NaCl, n = 24, N = 3; 300 µM Ber in the K-H buffer containing 62.5 mM NaCl, n = 21, N = 3; n and N are the numbers of LV myocytes and rats, respectively. Ber stands for berberine. ***p < 0.001 vs. vehicle control group; ###p < 0.001 vs. Ber-treated group in the modified K-H buffer containing 125 mM NaCl; two-way ANOVA with Tukey's multiple comparisons test.
Here we reported that berberine significantly increased the LVDP, +dp/dtmax, and the |−dp/dt|max in Langendorff-perfused isolated rat hearts. Berberine increased the tension of the isolated LVM strips and the intracellular Ca2+ level of isolated LV myocytes. The removal of extracellular Ca2+ or lowering extracellular Na+ reduced the berberine-induced increases in both LVM tension and the Ca2+ levels in LV myocytes. These results suggested that berberine increased the intracellular Ca2+ concentration of LV myocytes dependent on the extracellular Ca2+ and Na+. It consequently exerted its positive inotropic effect on the rat heart.
A clinical study showed that oral administration of berberine (1.2–2.0 g/d) increased LV ejection fraction in patients with chronic congestive heart failure who also took medications of angiotensin-converting enzyme inhibitors, digoxin, diuretics, and nitrates (Zeng et al., 2003). The LV ejection fraction increase in patients with plasma berberine concentrations higher than 0.1 mg/L (~0.3 μM) was more significant than in those with concentrations lower than 0.1 mg/L (Zeng et al., 2003). In the concentration range from 10 to 20 μM, berberine exhibited positive inotropic effects on the isolated rat hearts (Figures 1B–D). The sufficient concentrations in the in vitro study were higher than those observed in the clinical studies (Zeng and Zeng, 1999; Zeng et al., 2003).
Besides, Tan investigated the tissue distribution of berberine and its metabolites in rats with a single oral dose of 200 mg/kg (Tan et al., 2013). The time for berberine to reach its maximal concentrations in the plasma (~76.8 nM) and heart (~2 ng/g) were 1.33 ± 0.29 and 24 h, respectively. Berberrubine, one of the primary active metabolites of berberine, was also found in the heart (Li et al., 2011; Tan et al., 2013). The metabolite of berberine might contribute to the therapeutic effects of berberine as well. The significant decrease in resting systolic and diastolic blood pressures might also be beneficial to the heart failure patients treated with berberine (Zeng et al., 2003).
Berberine increased both the tension of LVM strips and the intracellular Ca2+ level of LV myocytes in the concentration range from 30 or 50 to 300 μM (Figures 2 and 5). The effective concentrations of berberine in these two preparations were higher than those (10–20 μM) in the Langendorff-perfused whole hearts. A previous study also showed that berberine (10–300 μM) increased the developed force in the left atrium of guinea pigs (Shaffer, 1985). These suggested that the impact of berberine on the cardiac function in patients was a systemic phenomenon rather than only via targeting the myocytes. Berberine increased the intracellular Ca2+ level of LV myocytes in a concentration-dependent manner, which reached the maximal level at 300 µM (Figure 5). This response was consistent with the maximal tension increase induced by 300 µM berberine in LVM strips (Figures 2 and 5). Therefore, we selected 300 µM berberine in the final study to determine the underlying mechanism.
The intracellular Ca2+ concentration in LV myocytes is the key for controlling the cardiac contractions, and the Ca2+ influx is critical for raising the intracellular Ca2+ level (Bers, 2002; Eisner et al., 2017; Gilbert et al., 2020). Berberine elevated the tension of LVM strips and the intracellular Ca2+ concentration in the isolated LV myocytes bathed in a buffer containing 1.5 mM Ca2+ (Figures 6B and 9B). The removal of Ca2+ from the buffer attenuated the functions of berberine (Figures 6D and 9D). These suggested that the berberine-induced tension increase in LVM strips is dependent on the extracellular Ca2+, and berberine elevates the intracellular Ca2+ level in LV myocytes by enhancing the Ca2+ influx.
L-type Ca2+ channel (LTCC) is the primary channel for Ca2+ entry in cardiac myocytes. Previous studies showed that berberine decreased or increased LTCC currents in guinea pig ventricular cells (Wang and Zheng, 1997; Xu et al., 1997), but had no effect on the LTCC current in cat ventricular cells (Sanchez-Chapula, 1996). Our results showed that the pretreatment with nifedipine did not affect the berberine-induced increase in the intracellular Ca2+ level of rat LV myocytes (Figure 10). This result indicated that berberine enhanced the Ca2+ entry through pathways not dependent on LTCCs.
The LTCC inhibitor nifedipine significantly attenuated the berberine-induced tension increase in the rat LVM strips (Figure 7). In the presence of the LTCC opener FPL-64716, berberine caused an additional increase in LVM strip tension (Figure 8). Besides, berberine significantly increased the intracellular Ca2+ concentration of LV myocytes bathed in the Ca2+-free buffer (Figure 9D). It indicated the berberine could enhance the Ca2+ release from the intracellular stores, but further research is needed to determine the mechanism. These suggested that berberine might also exert a positive inotropic effect through an LTCC-independent mechanism.
Sodium is also a critical cation regulating the excitation-contraction coupling in cardiomyocytes. The positive inotropic effect of berberine on LV strips bathed in the low Na+ buffer decreased (Figure 11). This phenomenon demonstrated that the berberine-induced tension increase in LVM strips was dependent on the extracellular Na+.
The acutely isolated cardiomyocytes are depolarized, and their intracellular Na+ level is elevated (Yao et al., 1998; Despa et al., 2002). The Na+ influx during the action potential activated reverse NCX and promoted the Ca2+ entry in cardiomyocytes and neurons (Czyz and Kiedrowski, 2002; Larbig et al., 2010; Yan et al., 2015). The removal of extracellular Na+ inhibits the increase in the intracellular Ca2+ level of human epidermoid carcinoma A-431 cells by NaCN (Kiang and Smallridge, 1994). The current study also showed that the reduction in the Na+ concentration in the buffer suppressed the elevation of the intracellular Ca2+ level in LV myocytes by berberine (Figure 12). A previous study showed that berberine increased myocardial contractility and cardiac output via interrelated mechanisms, including the lengthening of ventricular action potential duration, which was partially due to stimulating the reverse mode of NCX (Wang and Zheng, 1997; Lau et al., 2001). The data presented here indicated that berberine increased the tension of LVM strips via a Na+-dependent Ca2+ entry, which might be through activating the reverse mode of NCX.
The current study revealed that berberine elevated the intracellular Ca2+ level in LV myocytes by increasing Ca2+ influx. It consequently increased the LV contractility. These were extracellular Na+-dependent.
All datasets generated for this study are included in the article/supplementary material.
The animal study was reviewed and approved by College of Pharmaceutical Sciences, Southwest University, Chongqing, China.
WX, YW, and JZ contributed to the study design. JZ, YW, and YJ carried out literature research. JZ, YW, JG and YJ performed experiments. WX, JZ, YW and JG contributed to data analysis. WX, JZ, and YW contributed to manuscript preparation and manuscript revision. The first two authors contributed equally to the manuscript.
The authors declare that the research was conducted in the absence of any commercial or financial relationships that could be construed as a potential conflict of interest.
The authors are grateful for the support of the manuscript revision to all the members of our team and Dr. Xian-Min Yu. This work was supported by Funding from Southwest University (Grant number SWU 115086, 104290/22300503) and Administration of Foreign Experts Affairs of China (Grant number BC2018042).
HEPES, 4-(2-hydroxyethyl)-1-piperazinethanesulfonic acid; K-B, Kraft-Brühe; K-H, Krebs-Henseleit; LTCC, L-type Ca2+ channel; LV, left ventricular; LVDP, left ventricular developed pressure; LVEDP, left ventricular end-diastolic pressure; LVM, left ventricular muscle; LVP, left ventricular pressure; LVSP, left ventricular systolic pressure; NMDG, N-Methyl-D-glucamine; NCX, Na+/Ca2+ exchanger.
Affuso, F., Mercurio, V., Fazio, V., Fazio, S. (2010). Cardiovascular and metabolic effects of Berberine. World J. Cardiol. 2 (4), 71–77. doi: 10.4330/wjc.v2.i4.71
Bell, R. M., Mocanu, M. M., Yellon, D. M. (2011). Retrograde heart perfusion: the Langendorff technique of isolated heart perfusion. J. Mol. Cell Cardiol. 50 (6), 940–950. doi: 10.1016/j.yjmcc.2011.02.018
Bers, D. M. (2002). Cardiac excitation-contraction coupling. Nature 415 (6868), 198–205. doi: 10.1038/415198a
Blaustein, M. P., Lederer, W. J. (1999). Sodium/calcium exchange: its physiological implications. Physiol. Rev. 79 (3), 763–854. doi: 10.1152/physrev.1999.79.3.763
Bombardini, T. (2005). Myocardial contractility in the echo lab: molecular, cellular and pathophysiological basis. Cardiovasc. Ultrasound 3, 27. doi: 10.1186/1476-7120-3-27
Carre, G., Carreyre, H., Ouedraogo, M., Becq, F., Bois, P., Thibaudeau, S., et al. (2014). The hypotensive agent dodoneine inhibits L-type Ca2+ current with negative inotropic effect on rat heart. Eur. J. Pharmacol. 728, 119–127. doi: 10.1016/j.ejphar.2014.01.059
Chen, W., Wang, R., Chen, B., Zhong, X., Kong, H., Bai, Y., et al. (2014). The ryanodine receptor store-sensing gate controls Ca2+ waves and Ca2+-triggered arrhythmias. Nat. Med. 20 (2), 184–192. doi: 10.1038/nm.3440
Czyz, A., Kiedrowski, L. (2002). In depolarized and glucose-deprived neurons, Na+ influx reverses plasmalemmal K+-dependent and K+-independent Na+/Ca2+ exchangers and contributes to NMDA excitotoxicity. J. Neurochem. 83 (6), 1321–1328. doi: 10.1046/j.1471-4159.2002.01227.x
Despa, S., Islam, M. A., Pogwizd, S. M., Bers, D. M. (2002). Intracellular [Na+] and Na+ pump rate in rat and rabbit ventricular myocytes. J. Physiol. 539 (Pt 1), 133–143. doi: 10.1113/jphysiol.2001.012940
Eisner, D. A., Caldwell, J. L., Kistamas, K., Trafford, A. W. (2017). Calcium and Excitation-Contraction Coupling in the Heart. Circ. Res. 121 (2), 181–195. doi: 10.1161/CIRCRESAHA.117.310230
Galindo-Tovar, A., Kaumann, A. J. (2008). Phosphodiesterase-4 blunts inotropism and arrhythmias but not sinoatrial tachycardia of (-)-adrenaline mediated through mouse cardiac beta(1)-adrenoceptors. Br. J. Pharmacol. 153 (4), 710–720. doi: 10.1038/sj.bjp.0707631
Gilbert, G., Demydenko, K., Dries, E., Puertas, R. D., Jin, X., Sipido, K., et al. (2020). Calcium Signaling in Cardiomyocyte Function. Cold Spring Harb Perspect. Biol. 12 (3), 1–29. doi: 10.1101/cshperspect.a035428
Huang, H., Xie, M., Gao, L., Zhang, W., Zhu, X., Wang, Y., et al. (2019). Rolipram, a PDE4 Inhibitor, Enhances the Inotropic Effect of Rat Heart by Activating SERCA2a. Front. Pharmacol. 10, 221. doi: 10.3389/fphar.2019.00221
Jaleel, N., Nakayama, H., Chen, X., Kubo, H., MacDonnell, S., Zhang, H., et al. (2008). Ca2+ influx through T- and L-type Ca2+ channels have different effects on myocyte contractility and induce unique cardiac phenotypes. Circ. Res. 103 (10), 1109–1119. doi: 10.1161/CIRCRESAHA.108.185611
Jiang, M., Murias, J. M., Chrones, T., Sims, S. M., Lui, E., Noble, E. G. (2014). American ginseng acutely regulates contractile function of rat heart. Front. Pharmacol. 5, 43. doi: 10.3389/fphar.2014.00043
Johnson, D. M., Antoons, G. (2018). Arrhythmogenic Mechanisms in Heart Failure: Linking beta-Adrenergic Stimulation, Stretch, and Calcium. Front. Physiol. 9, 1453. doi: 10.3389/fphys.2018.01453
Kang, J., Chen, X. L., Ji, J., Lei, Q., Rampe, D. (2012). Ca2+ channel activators reveal differential L-type Ca2+ channel pharmacology between native and stem cell-derived cardiomyocytes. J. Pharmacol. Exp. Ther. 341 (2), 510–517. doi: 10.1124/jpet.112.192609
Kiang, J. G., Smallridge, R. C. (1994). Sodium cyanide increases cytosolic free calcium: evidence for activation of the reversed mode of the Na+/Ca2+ exchanger and Ca2+ mobilization from inositol trisphosphate-insensitive pools. Toxicol. Appl. Pharmacol. 127 (2), 173–181. doi: 10.1006/taap.1994.1151
Larbig, R., Torres, N., Bridge, J. H., Goldhaber, J. I., Philipson, K. D. (2010). Activation of reverse Na+-Ca2+ exchange by the Na+ current augments the cardiac Ca2+ transient: evidence from NCX knockout mice. J. Physiol. 588 (Pt 17), 3267–3276. doi: 10.1113/jphysiol.2010.187708
Lau, C. W., Yao, X. Q., Chen, Z. Y., Ko, W. H., Huang, Y. (2001). Cardiovascular actions of berberine. Cardiovasc. Drug Rev. 19 (3), 234–244. doi: 10.1111/j.1527-3466.2001.tb00068.x
Li, Y., Ren, G., Wang, Y. X., Kong, W. J., Yang, P., Wang, Y. M., et al. (2011). Bioactivities of berberine metabolites after transformation through CYP450 isoenzymes. J. Transl. Med. 9, 62. doi: 10.1186/1479-5876-9-62
Nakao, S., Wakabayashi, S., Nakamura, T. Y. (2015). Stimulus-dependent regulation of nuclear Ca2+ signaling in cardiomyocytes: a role of neuronal calcium sensor-1. PloS One 10 (4), e0125050. doi: 10.1371/journal.pone.0125050
Philipson, K. D., Nicoll, D. A. (2000). Sodium-calcium exchange: a molecular perspective. Annu. Rev. Physiol. 62, 111–133. doi: 10.1146/annurev.physiol.62.1.111
Roth, G. M., Bader, D. M., Pfaltzgraff, E. R. (2014). Isolation and physiological analysis of mouse cardiomyocytes. J. Vis. Exp. (91), e51109. doi: 10.3791/51109
Sanchez-Chapula, J. (1996). Increase in action potential duration and inhibition of the delayed rectifier outward current IK by berberine in cat ventricular myocytes. Br. J. Pharmacol. 117 (7), 1427–1434. doi: 10.1111/j.1476-5381.1996.tb15302.x
Shaffer, J. E. (1985). Inotropic and chronotropic activity of berberine on isolated guinea pig atria. J. Cardiovasc. Pharmacol. 7 (2), 307–315. doi: 10.1097/00005344-198503000-00016
Sola, R., Valls, R. M., Puzo, J., Calabuig, J. R., Brea, A., Pedret, A., et al. (2014). Effects of poly-bioactive compounds on lipid profile and body weight in a moderately hypercholesterolemic population with low cardiovascular disease risk: a multicenter randomized trial. PloS One 9 (8), e101978. doi: 10.1371/journal.pone.0101978
Song, M., Chen, D., Yu, S. P. (2014). The TRPC channel blocker SKF 96365 inhibits glioblastoma cell growth by enhancing reverse mode of the Na+/Ca2+ exchanger and increasing intracellular Ca2+. Br. J. Pharmacol. 171 (14), 3432–3447. doi: 10.1111/bph.12691
Tan, X. S., Ma, J. Y., Feng, R., Ma, C., Chen, W. J., Sun, Y. P., et al. (2013). Tissue distribution of berberine and its metabolites after oral administration in rats. PloS One 8 (10), e77969. doi: 10.1371/journal.pone.0077969
Tang, T., Duffield, R., Ho, A. K. (1998). Effects of Ca2+ channel blockers on Ca2+ loading induced by metabolic inhibition and hyperkalemia in cardiomyocytes. Eur. J. Pharmacol. 360 (2-3), 205–211. doi: 10.1016/s0014-2999(98)00657-8
Tilley, D. G., Zhu, W., Myers, V. D., Barr, L. A., Gao, E., Li, X., et al. (2014). beta-adrenergic receptor-mediated cardiac contractility is inhibited via vasopressin type 1A-receptor-dependent signaling. Circulation 130 (20), 1800–1811. doi: 10.1161/CIRCULATIONAHA.114.010434
Uhl, S., Freichel, M., Mathar, I. (2015). Contractility Measurements on Isolated Papillary Muscles for the Investigation of Cardiac Inotropy in Mice. J. Vis. Exp. (103), e53076. doi: 10.3791/53076
Wang, Y. X., Zheng, Y. M. (1997). Ionic mechanism responsible for prolongation of cardiac action-potential duration by berberine. J. Cardiovasc. Pharmacol. 30 (2), 214–222. doi: 10.1097/00005344-199708000-00010
Xin, W. K., Kwan, C. L., Zhao, X. H., Xu, J., Ellen, R. P., McCulloch, C. A., et al. (2005). A functional interaction of sodium and calcium in the regulation of NMDA receptor activity by remote NMDA receptors. J. Neurosci. 25 (1), 139–148. doi: 10.1523/JNEUROSCI.3791-04.2005
Xu, S. Z., Zhang, Y., Ren, J. Y., Zhou, Z. N. (1997). Effects of berberine of L- and T-type calcium channels in guinea pig ventricular myocytes. Zhongguo Yao Li Xue Bao 18 (6), 515–518.
Yan, Z. Y., Ban, T., Fan, Y., Chen, W. R., Sun, H. L., Chen, H., et al. (2015). Na+-induced Ca2+ influx through reverse mode of Na+-Ca2+ exchanger in mouse ventricular cardiomyocyte. Oncotarget 6 (27), 23272–23280. doi: 10.18632/oncotarget.4878
Yao, A., Su, Z., Nonaka, A., Zubair, I., Lu, L., Philipson, K. D., et al. (1998). Effects of overexpression of the Na+-Ca2+ exchanger on [Ca2+]i transients in murine ventricular myocytes. Circ. Res. 82 (6), 657–665. doi: 10.1161/01.res.82.6.657
Yuan, L., Tu, D., Ye, X., Wu, J. (2006). Hypoglycemic and hypocholesterolemic effects of Coptis chinensis franch inflorescence. Plant Foods Hum. Nutr. 61 (3), 139–144. doi: 10.1007/s11130-006-0023-7
Zeng, X., Zeng, X. (1999). Relationship between the clinical effects of berberine on severe congestive heart failure and its concentration in plasma studied by HPLC. BioMed. Chromatogr. 13 (7), 442–444. doi: 10.1002/(SICI)1099-0801(199911)13:7<442::AID-BMC908>3.0.CO;2-A
Keywords: berberine, heart, positive inotropic effect, Ca2+, Na+
Citation: Zhao J, Wang Y, Gao J, Jing Y and Xin W (2020) Berberine Mediated Positive Inotropic Effects on Rat Hearts via a Ca2+-Dependent Mechanism. Front. Pharmacol. 11:821. doi: 10.3389/fphar.2020.00821
Received: 22 August 2019; Accepted: 19 May 2020;
Published: 05 June 2020.
Edited by:
Martin C. Michel, Johannes Gutenberg University Mainz, GermanyCopyright © 2020 Zhao, Wang, Gao, Jing and Xin. This is an open-access article distributed under the terms of the Creative Commons Attribution License (CC BY). The use, distribution or reproduction in other forums is permitted, provided the original author(s) and the copyright owner(s) are credited and that the original publication in this journal is cited, in accordance with accepted academic practice. No use, distribution or reproduction is permitted which does not comply with these terms.
*Correspondence: Wenkuan Xin, eGlud2Vua3VhbkBzd3UuZWR1LmNu
Disclaimer: All claims expressed in this article are solely those of the authors and do not necessarily represent those of their affiliated organizations, or those of the publisher, the editors and the reviewers. Any product that may be evaluated in this article or claim that may be made by its manufacturer is not guaranteed or endorsed by the publisher.
Research integrity at Frontiers
Learn more about the work of our research integrity team to safeguard the quality of each article we publish.