- 1Institute of Clinical Physiology, National Research Council (CNR), Pisa, Italy
- 2Department of Clinical and Experimental Medicine, University of Pisa, Pisa, Italy
Primary sclerosing cholangitis is a complex pathological condition, characterized by chronic inflammation and fibrosis of the biliary epithelium. Without proper clinical management, progressive bile ducts and liver damage lead to cirrhosis and, ultimately, to liver failure. The known limited role of current drugs for treating this cholangiopathy has driven researchers to assess alternative therapeutic options. Some herbal remedies and their phytochemicals have shown anti-fibrotic properties in different experimental models of hepatic diseases and, occasionally, in clinical trials in primary sclerosing cholangitis patients; however their mechanism of action is not completely understood. This review briefly examines relevant studies focusing on the potential anti-fibrotic properties of Silybum marianum, Curcuma longa, Salvia miltiorrhiza, and quercetin. Each natural product is individually reviewed and the possible mechanisms of action discussed.
Introduction
Primary Sclerosing Cholangitis (PSC) is a progressive cholestatic liver disease caused by chronic inflammation and fibrosis of the biliary epithelium, resulting in multi-focal bile duct strictures that affect intrahepatic and extrahepatic bile ducts, leading to cirrhosis and eventually hepatic failure (Levy and Lindor, 2006; Takakura et al., 2017). The clinical manifestations and complications related to PSC include abdominal pain, jaundice, infectious cholangitis, pruritus, vitamin deficiencies, metabolic bone disease, portal hypertension, varices, polyps, and malignancies, particularly cholangiocarcinoma (CCA) (Burak et al., 2004; Levy and Lindor, 2006; Razumilava et al., 2011; O'Toole et al., 2012; Franceschet et al., 2016; Karlsen et al., 2017; Takakura et al., 2017; Taghavi et al., 2018; Mertz et al., 2019). The etiology and pathogenesis of PSC remains elusive, although the key role of immune-mediated mechanisms is mostly accredited (Eaton et al., 2013; Tabibian et al., 2018). The exposure to environmental factors could trigger a complex interaction between adaptive and innate immune systems, leading to lymphocyte migration, cholangiocyte damage, and chronic fibrosis. Recently, genome-wide association studies have pointed out a strong correlation between PSC and genes able to regulate immune self-recognition and adaptive immunity (Karlsen et al., 2010; Melum et al., 2011; Ji et al., 2017). A “leaky gut” hypothesis has also been proposed, suggesting that the impairment of intestinal barrier function might lead to microbial translocation into bile (Navaneethan, 2015; Pontecorvi et al., 2016; Sabino et al., 2016; Tabibian et al., 2016; Giordano et al., 2018). PSC-associated cholangiocytes accumulate high level of bacterial lipopolysaccharides in vivo (Sasatomi et al., 1998); thus the impairment of gut-liver axis might trigger hepatobiliary inflammation and immune responses. The lack of unique and well-characterized pathogenesis still makes difficult the development of effective pharmacological therapies, which are actually aimed at treating symptoms and managing complications (Eaton et al., 2013; Lindor et al., 2015; Suri et al., 2019). Hence, liver transplantation remains the treatment of choice for end-stage PSC; however, recurrence occurs in the 20% to 40% of transplanted patients (Visseren and Darwish Murad, 2017).
In PSC, the concentric accumulation of connective tissue around intrahepatic and extrahepatic bile ducts (known as “onion-like” fibrosis) suggests that cholangiocytes play a central role in driving the fibrotic machinery (Eaton et al., 2013; O'Hara et al., 2017; Banales et al., 2019). Cholangiocytes are epithelial cells of the biliary tract that are quiescent in physiological condition. In chronic cholestatic liver disease, including PSC, cholangiocytes play a double role, being target, but also active subjects of the pathological status through multiple molecular processes. In response to liver injury, cholangiocytes acquire a reactive status which is characterized by increased expression of anti-apoptotic genes (Bcl-2) and adhesion molecules, and loss of some epithelial markers such as CK-7, CK-19, or E-cadherin in favor to functional and morphologic markers associated with mesenchymal phenotype (Kalluri and Neilson, 2003; Omenetti et al., 2008; Rygiel et al., 2008; Fabris et al., 2016). Once activated, cholangiocytes release numerous chemokines, cytokines, growth factors, neuroendocrine molecules, and other proinflammatory and fibrogenic mediators that act in autocrine and paracrine manners, activating other cell types (hepatic stellate cells (HSCs), portal fibroblasts, myofibroblasts, and hepatocytes) and recruiting immune cells that promote biliary damage (Lazaridis et al., 2004; Fabris et al., 2016; Fabris et al., 2017; Banales et al., 2019). In vitro and in vivo studies have partially highlighted the importance of cell-cell communication between cholangiocytes and HSCs, which involves multiple signaling pathways related to transforming growth factor-beta 1 (TGF-β1), Smad, hepatocyte nuclear factor 3-β, and integrin αvβ/nuclear factor-kappa B (NFkβ) axis (O'Hara et al., 2013; Kim et al., 2015; McDaniel et al., 2017). Indeed, activated HSCs seem to represent the main contributors to fibrosis in cholestatic liver disease, including PSC (Fickert et al., 2014). Under healthy condition, HSCs exert an equilibrium between extracellular matrix production and degradation; however, following activation especially via TGF-β1, HSCs show a proliferative status, loss of vitamin A and lipid droplets, increased expression of alpha-smooth muscle actin (α-SMA), and synthesis of extracellular matrix components (De Minicis et al., 2007; Mallat and Lotersztajn, 2013). The role of matrix metalloproteinases (MMPs) and tissue inhibitors of metalloproteinases (TIMPs) is well documented in regulating the levels of extracellular matrix (Visse and Nagase, 2003). Interestingly, decreased activity of MMPs is reported in liver fibrosis as a consequence of TIMPs overexpression in activated HSCs (Arthur et al., 1998). Briefly, the fibrotic process requires the involvement of different cells and inflammatory and pro-fibrogenic mediators. It is worth noticing that these mediators are mainly modulated through redox-sensitive reactions (Poli, 2000; Sánchez-Valle et al., 2012; Luangmonkong et al., 2018). Despite the efforts of scientists in testing the efficacy of new and old molecules, the major challenge in PSC remains the chronic progression of hepatobiliary fibrosis towards liver failure.
A wide range of herbal remedies has shown promising effects against hepatic fibrosis either in experimental models or even in preliminary clinical trials (Latief and Ahmad, 2017). In this review, we discuss four plant-derived products that have shown anti-fibrotic properties in multiple mouse models of hepatic fibrosis and in few clinical trials in PSC patients.
Natural Products Active as Hepatic Antifibrotic Agents
A Medline search was performed in order to identify relevant published reports. The key words “herbal,” “phytotherapy,” “phytochemicals,” “bioflavonoids,” “plant extracts” were cross-referenced with “primary sclerosing cholangitis” and “hepatic fibrosis.” Among the many herbal remedies and phytochemicals that exert antifibrotic properties in different hepatic diseases, we focused our attention on four of them. In particular, Silybum marianum and Curcuma longa have been investigated in clinical trials in PSC patients; Salvia miltiorrhiza exerts antifibrotic properties in different chemically-induced liver fibrosis mouse models; and quercetin is a flavonoid presents in silymarin mixture and also it is daily taken with the diet.
Silybum marianum
Silybum marianum, also known as milk thistle, has been used since long time in the management of liver diseases and biliary disorders. Silymarin is a mixture of flavonolignans containing silybins A and B, isosilybins A and B, silychristin, silydianin, and in smaller quantities, flavonols 2.3 dehydrosilybin, quercetin, (+) taxifolin, and kaempferol (Calani et al., 2012). The safety and the efficacy of silymarin have been investigated in PSC patients in an open-label pilot study (Angulo et al., 2008). A significant reduction in ALP and AST occurred following oral treatment with silymarin (140 mg three times daily for one year). No statistically significant changes in serum bilirubin and albumin levels were registered, indicating a possible arrest of PSC progression during silymarin treatment. The immunomodulatory, antioxidant, and antifibrotic effects of this natural mixture have been also investigated in several experimental models of liver fibrosis (Boigk et al., 1997; Tsai et al., 2008; Clichici et al., 2016; Federico et al., 2017; Karimi et al., 2018). In carbon tetrachloride–induced liver fibrosis in rats, silymarin significantly decreased the level of AST, ALT, and ALP in serum, and inhibited the increased expressions of α-SMA in liver tissue (Tsai et al., 2008). The α-SMA is a well-established marker of HSCs activation, and the reduction of this protein levels is related to the inhibition of activated HSCs (Carpino et al., 2005; De Minicis et al., 2007; Mallat and Lotersztajn, 2013). Interestingly, Clichici and colleagues confirmed the effectiveness of silymarin as an antifibrotic agent in carbon tetrachloride–treated mice through the reduction of α-SMA and TGF-β1 expression (Clichici et al., 2015; Clichici et al., 2016). In thioacetamide-induced chronic liver fibrosis, silymarin also demonstrated antifibrotic properties (Chen et al., 2012). The antifibrotic effects were primarily attributed to reduced hepatic levels of TIMP-1/2, whose overexpression is related to HSCs activation, TGF-β1, α-SMA, and collagen I expression (Kara et al., 2008; Chen et al., 2012). These data suggest that silymarin could exert its antifibrotic properties on cholangiocytes/HSCs axis perhaps inhibiting HSCs activation, which represents a key event in PSC development (Fickert et al., 2014). Moreover, Silybum marianum is well known for its antioxidant and anti-inflammatory properties on multiple hepatic disorders via the modulation of various transcription factors (Loguercio et al., 2007; Post-White et al., 2007; Federico et al., 2008; Trappoliere et al., 2009; Loguercio and Festi, 2011; Loguercio et al., 2012; Stiuso et al., 2014).
Salvia miltiorrhiza
Salvia miltiorrhiza is a very popular traditional Chinese remedy widely used to treat different pathological conditions, including cardiovascular diseases, tumors, and cerebrovascular diseases (Yang et al., 2010; Zhou et al., 2011; Wang W.H. et al., 2017; Wang L. et al., 2017). This herbal medicine contains multiple lipophilic compounds and hydrophilic phenolic acids (Zhang et al., 2012; Cai et al., 2016).
Salvia miltiorrhiza has been shown to attenuate liver fibrosis in multiple experimental models (Wasser et al., 1998; Parajuli et al., 2015; Peng et al., 2018). Salvia miltiorrhiza is able to reverse hepatic fibrosis in rats following exposition to carbon tetrachloride, lowering levels of TGF-β1, procollagens I and III (Wasser et al., 1998). As previously reported, TGF-β1 represents the main activator of HSCs, which are responsible of the synthesis of extracellular matrix components (De Minicis et al., 2007; Mallat and Lotersztajn, 2013). A recent study demonstrated that the antifibrotic effect of Salvia miltiorrhiza is correlated with the increased activity of hepatic natural killers (NK) and inhibition of HSCs activation, as confirmed by diminished levels of α-SMA (Peng et al., 2018). Accumulating evidences suggest that NK cells play a pivotal role in controlling liver fibrosis through killing activated HSCs, as reported in both human and animal experiments (Muhanna et al., 2011; Fasbender et al., 2016; Shi et al., 2017). The anti-fibrotic effect of NK cells is suppressed during advanced liver injury, contributing to the progression of liver fibrosis (Jeong et al., 2011). Therefore, the ability of Salvia miltiorrhiza in restoring and promoting the activities of NK cells might represent an important anti-fibrotic mechanism. Most interesting, the immunomodulatory activity of Salvia miltiorrhiza was also demonstrated in BALB/c mice following Listeria monocytogenes infection, as confirmed by the increased number of peripheral monocytes and NK cells (Gao et al., 2012). It is interesting to remind the antifibrotic effects of PF2401-SF, a standardized and purified fraction of Salvia miltiorrhiza, in thioacetamide and carbon tetrachloride-induced liver fibrosis in rats (Parajuli et al., 2013; Parajuli et al., 2015). In these chemically-induced hepatic fibrosis models, Salvia miltiorrhiza seems to exert its action on HSC activation that might be mediated by downregulation of pivotal markers of fibrosis, including α-SMA, collagen I, and TIMP1. It is also well known that Salvia miltiorrhiza protects liver attenuating inflammatory reactions (Xie et al., 2014; Ma et al., 2016) and exerting antioxidant effects (Zhang et al., 1990; Zhao et al., 2006).
Curcuma longa
Curcuma longa (Turmeric) has been used for centuries in both Ayurvedic and Chinese medicine for its anti-inflammatory properties in a wide repertoire of pathological conditions (Pari et al., 2008). Turmeric contains primarily curcumin, a phenolic compound, and three different analogs of curcumin (diferuloylmethane, demethoxycurcumin, and bisdemethoxycurcumin), as well as resins and volatile oils (tumerone, atlantone, and zingiberone) (Jurenka, 2009). More than 50 clinical studies assessed or are currently evaluating the pharmacological effects of curcumin in many different disorders in man. Recently, an open-label pilot study conducted at the Mayo Clinic, was focused on the safety and effectiveness of oral administration (750 mg twice a day for 12 weeks) of BCM-95 CG, a novel bioenhanced preparation of curcumin, in PSC patients (Eaton et al., 2019). Despite the low number of enrolled patients, a large amount of preclinical data suggest that antifibrotic properties of curcumin are relevant in chronic hepatic fibrosis. In particular, Baghdasaryan and colleagues highlighted the ability of curcumin in reducing bile duct injury and biliary fibrosis in Mdr2−/− mice (Baghdasaryan et al., 2010), which are currently used as a murine model for sclerosing cholangitis (Fickert et al., 2004). During cholangiopathies, activated cholangiocytes upregulate the expression of adhesion molecules and proinflammatory mediators responsible of the recruitment of immune cells (Lazaridis et al., 2004; Fabris et al., 2016; Fabris et al., 2017; Banales et al., 2019). In this respect, increased expression of vascular cell adhesion molecule 1 (VCAM-1) by cholangiocytes contributes to the persistence of liver inflammation through the recruitment of monocytes and lymphocytes, and mediating leukocyte adhesion by binding α4β1 integrins (Afford et al., 2014). Interestingly, curcumin is able to reduce VCAM-1 protein levels in Mdr2−/− mice via peroxisome proliferator-activated receptor gamma (PPARγ) signaling, without affecting the expression of stimulatory pro-inflammatory cytokines (Baghdasaryan et al., 2010). These results are consistent with the reduction of bile duct proliferation and biliary fibrosis observed following treatment with PPARγ agonists in bile duct-ligated (BDL) mouse models (Marra et al., 2000; Marra et al., 2005). Thus, the antifibrotic properties of curcumin could be mediated, in part, by the activation of PPARγ in activated cholangiocytes. Other studies have also demonstrated the key role of PPARγ expression in the maintenance of the quiescent HSCs phenotype (Hazra et al., 2004a; Hazra et al., 2004b). Consistently, the depletion of PPARγ together with the increased activation of nuclear factor NF-κB and ERK have been reported in in vitro activated human and rat HSCs (Elsharkawy et al., 1999; Hazra et al., 2004b; Foglia et al., 2019). Curcumin inhibited HSCs activation in vitro through the increased PPARγ expression and stimulation of PPARγ signaling, resulting in the inhibition of NF-κB activity (Xu et al., 2003; Zheng and Chen, 2004; Chen and Zheng, 2008). Furthermore, curcumin suppressed the expression of connective tissue growth factor in activated HSCs in vitro through NF‐κB inhibition, leading to the reduction of extracellular matrix components synthesis, including collagen I (Zheng and Chen, 2006; Chen and Zheng, 2008). Moreover, curcumin inhibits ERK signaling pathway, whose involvement in the activation of HSCs has already been demonstrated (Chen and Zheng, 2008; Foglia et al., 2019). Accumulating evidences indicate curcumin as a compound that possesses anti-inflammatory (Salama et al., 2013; He et al., 2015; Lee et al., 2020) ref) and protective effects towards oxidative associated liver diseases (Reyes-Gordillo et al., 2007; Fu et al., 2008; Salama et al., 2013; Liu et al., 2016; Samarghandian et al., 2017).
Quercetin
Quercetin is a natural flavonoid widely present in many vegetables and fruits belonging to the family of Apiaceae, Brassicaceae, Rosaceae (Anand David et al., 2016; Babaei et al., 2018). It possesses various hepatoprotective properties, including antioxidant, antiviral, anti-inflammatory, anti-proliferative, and antifibrotic effects (Russo et al., 2012; Li et al., 2016; Caddeo et al., 2019; Lesjak et al., 2018; Xu et al., 2019). The positive effect of quercetin on liver fibrosis has been demonstrated both in vitro and in several murine models (Pavanato et al., 2003; Marcolin et al., 2012; Casas-Grajales et al., 2017; Wang R. et al., 2017; Wu et al., 2017; Li et al., 2018). In an in vivo study, Wu and colleagues observed inhibition of HSCs activation and proliferation following treatment with quercetin (Wu et al., 2017). These data are consistent with other in vivo studies that reported the anti-hepatofibrotic properties of quercetin in chemically-induced liver fibrosis mouse models (Pavanato et al., 2003; Casas-Grajales et al., 2017; Wang R. et al., 2017; Li et al., 2018). Quercetin exhibited anti-fibrogenic activity by regulation of HSCs-activation markers (e.g. α-SMA, Collagen I, TIMP-1) (Hernández-Ortega et al., 2012; Wang R. et al., 2017). Indeed, quercetin inhibited the activation of NF-κB in a dose-dependent manner via inhibition of IкBα degradation and decreased the expression of p38 MAPK by inhibiting its phosphorylation. These data are consistent with the well-documented role of p38MAPK/NF-κB axis in inflammation and HSCs activation (Schnabl et al., 2001; Luedde and Schwabe, 2011; Taniguchi and Karin, 2018; Czauderna et al., 2019). Novel evidences into quercetin activity have shown the inhibition of liver fibrosis through the regulation of macrophage activation and function via Notch1 pathway in carbon tetrachloride-treated mice (Li et al., 2018). Macrophage Notch1 expression was increased during liver injury in mice but quercetin treatment reversed this effect. These results are consistent with the role of Notch pathway in macrophage-mediated inflammation (Xu et al., 2015a; Eun and Jeong, 2016; Kimball et al., 2017), including certain hepatic diseases (Geisler and Strazzabosco, 2015; Xu et al., 2015b). Several studies indicate that autophagy may represent a key role for the modulation of numerous signaling pathways related to HSC activation (Thoen et al., 2011; Hernández-Gea et al., 2012). Thoen and colleagues have demonstrated that HSC activation is followed by an increased autophagic flux whose inhibition can in part inhibit HSCs (Thoen et al., 2011; Thoen et al., 2012). Moreover, quercetin was demonstrated to ameliorate liver fibrosis reducing HSCs autophagy through the regulation of the TGF-β1/Smad axis (Wu et al., 2017). It is interesting to note that this signaling pathway is related to the modulation of extracellular matrix gene expression causing fibrosis (Das et al., 2014).
Conclusions
Currently, effective therapies in arresting hepatobiliary fibrosis and restoring proper liver function in PSC are lacking; therefore, alternative therapies, which could help in the clinical management of PSC have been approached. Some herbs and phytochemicals have shown anti-fibrotic properties in different experimental models of liver fibrosis and also in PSC patients. Table 1 is a summary of the literature exploring pharmacological effects and cellular targets of the herbal remedies taken into account in this review. Briefly, silymarin, curcumin, Salvia miltiorrhiza, and quercetin have been demonstrated to inhibit stellate cell activation (Figure 1A). Curcumin has a dual role, affecting both PPARγ and ERK signaling blocking HSC activation. In addition, through PPARy, it also affects cholangiocytes (Figure 1B). The ability of these natural products in acting on cholangiocytes/HSCs axis could represent an encouraging antifibrotic therapeutic opportunity. Moreover, the plant-derived phytoconstituents have antinflammatory and anti-oxidant activity that could impact positively on biliary cholestasis and fibrosis. The evidences supporting the use of herbs and phytochemicals in the management of PSC are insufficient; crude herbs and phytoproducts need to be examined also for their potential toxic effects. Nevertheless, due to the complexity of PSC and the lack of established cures, the roles of Silybum marianum, Curcuma longa, Salvia miltiorrhiza, and quercetin should be better evaluated in properly designed clinical studies. The possible use of these natural compounds alone or in combination with traditional drugs could represent a very promising field for future researches and therapies.
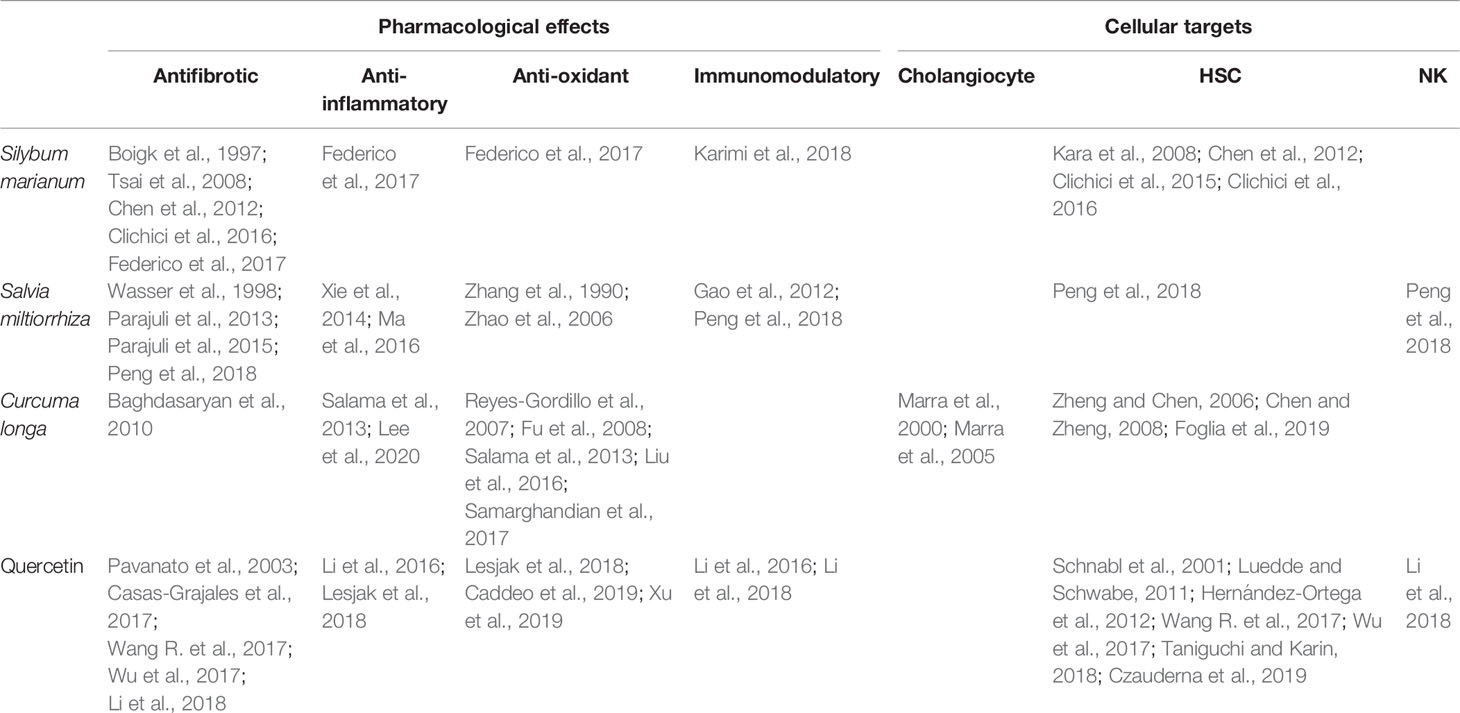
Table 1 Summary of references on pharmacological effects and cellular targets of the herbal remedies Silybum marianum, Salvia miltiorrhiza, Curcuma longa, and quercetin discussed in this review.
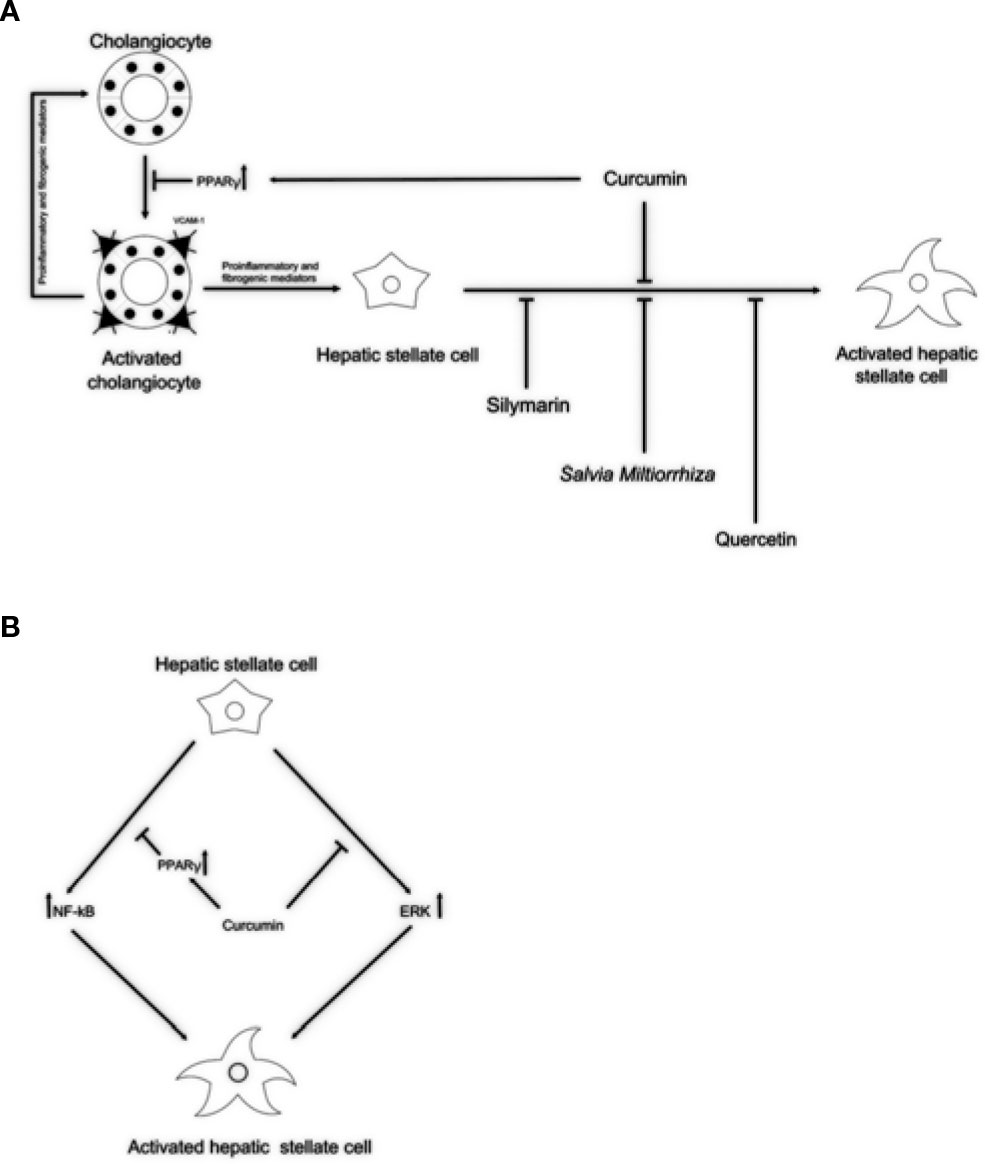
Figure 1 Potential anti-fibrotic properties of natural remedies in cholangiopathies. (A) By activation of PPARγ, curcumin might block reactive cholangiocytes and their inflammatory activation through the reduction of VCAM-1 expression, resulting in decreased attraction of immune cells. Silymarin, Salvia miltiorrhiza, and quercetin might exert its antifibrotic properties acting directly on HSCs activation. (B) In addition, acting as PPARγ activator and by inhibiting the ERK signaling curcumin might block HSCs activation. This dual role of curcumin could explain the antifibrotic properties exerted in experimental models and clinical trials. PPARγ, peroxisome proliferator-activated receptor gamma; VCAM-1, vascular cell adhesion molecule 1; ERK, extracellular signal–regulated kinases; HSCs, hepatic stellate cells.
Author Contributions
All authors contributed to reviewing the current literature and writing of the manuscript and approved the final version of the paper. Conceptualization: EC, MM, SR. Original draft preparation: EC, AC. Final editing: EC, AC, MM, SR.
Funding
The authors gratefully acknowledge the financial support ofAIRCS-Italian Association for Sclerosing Cholangitis Research.
Conflict of Interest
The authors declare that the research was conducted in the absence of any commercial or financial relationships that could be construed as a potential conflict of interest.
Acknowledgments
We are also grateful to Prof. Paola Muti, Department of Oncology, Faculty of Health Sciences, McMaster University, for comments that greatly improved the manuscript.
References
Afford, S. C., Humphreys, E. H., Reid, D. T., Russell, C. L., Banz, V. M., Oo, Y., et al. (2014). Vascular cell adhesion molecule 1 expression by biliary epithelium promotes persistence of inflammation by inhibiting effector T-cell apoptosis. Hepatology 59, 1932–1943. doi: 10.1002/hep.26965
Anand David, A. V., Arulmoli, R., Parasuraman, S. (2016). Overviews of Biological Importance of Quercetin: A Bioactive Flavonoid. Pharmacogn. Rev. 10, 84–89. doi: 10.4103/0973-7847.194044
Angulo, P., Jorgensen, R. A., Kowdley, K. V., Lindor, K. D. (2008). Silymarin in the treatment of patients with primary sclerosing cholangitis: an open-label pilot study. Digestive Dis. Sci. 53, 1716–1720. doi: 10.1007/s10620-007-0052-6
Arthur, M. J., Mann, D. A., Iredale, J. P. (1998). Tissue inhibitors of metalloproteinases, hepatic stellate cells and liver fibrosis. J. Gastroenterol. Hepatol. 13 Suppl, S33–S38. doi: 10.1111/jgh.1998.13.s1.33
Babaei, F., Mirzababaei, M., Nassiri-Asl, M. (2018). Quercetin in Food: Possible Mechanisms of Its Effect on Memory. J. Food Sci. 83, 2280–2287. doi: 10.1111/1750-3841.14317
Baghdasaryan, A., Claudel, T., Kosters, A., Gumhold, J., Silbert, D., Thüringer, A., et al. (2010). Curcumin improves sclerosing cholangitis in Mdr2-/- mice by inhibition of cholangiocyte inflammatory response and portal myofibroblast proliferation. Gut 59, 521–530. doi: 10.1136/gut.2009.186528
Banales, J. M., Huebert, R. C., Karlsen, T., Strazzabosco, M., LaRusso, N. F., Gores, G. J. (2019). Cholangiocyte pathobiology. Nat. Rev. Gastroenterol. Hepatol. 16, 269–281. doi: 10.1038/s41575-019-0125-y
Boigk, G., Stroedter, L., Herbst, H., Waldschmidt, J., Riecken, E. O., Schuppan, D. (1997). Silymarin retards collagen accumulation in early and advanced biliary fibrosis secondary to complete bile duct obliteration in rats. Hepatology 26, 643–649. doi: 10.1002/hep.510260316
Burak, K., Angulo, P., Pasha, T. M., Egan, K., Petz, J., Lindor, K. D. (2004). Incidence and risk factors for cholangiocarcinoma in primary sclerosing cholangitis. Am. J. Gastroenterol. 99, 523–526. doi: 10.1111/j.1572-0241.2004.04067.x
Caddeo, C., Gabriele, M., Fernàndez-Busquets, X., Valenti, D., Fadda, A. M., Pucci, L., et al. (2019). Antioxidant activity of quercetin in Eudragit-coated liposomes for intestinal delivery. Int. J. Pharmaceut. 565, 64–69. doi: 10.1016/j.ijpharm.2019.05.007
Cai, Y., Zhang, W., Chen, Z., Shi, Z., He, C., Chen, M. (2016). Recent insights into the biological activities and drug delivery systems of tanshinones. Int. J. Nanomed. 11, 121–130. doi: 10.2147/IJN.S84035
Calani, L., Brighenti, F., Bruni, R., Del Rio, D. (2012). Absorption and metabolism of milk thistle flavanolignans in humans. Phytomedicine 20, 40–46. doi: 10.1016/j.phymed.2012.09.004
Carpino, G., Morini, S., Ginanni Corradini, S., Franchitto, A., Merli, M., Siciliano, M., et al. (2005). Alpha-SMA expression in hepatic stellate cells and quantitative analysis of hepatic fibrosis in cirrhosis and in recurrent chronic hepatitis after liver transplantation. Digestive Liver Dis. 37, 349–356. doi: 10.1016/j.dld.2004.11.009
Casas-Grajales, S., Vázquez-Flores, L. F., Ramos-Tovar, E., Hernández-Aquino, E., Flores-Beltrán, R. E., Cerda-García-Rojas, C. M., et al. (2017). Quercetin reverses experimental cirrhosis by immunomodulation of the proinflammatory and profibrotic processes. Fundam. Clin. Pharmacol. 31, 610–624. doi: 10.1111/fcp.12315
Chen, A., Zheng, S. (2008). Curcumin inhibits connective tissue growth factor gene expression in activated hepatic stellate cells in vitro by blocking NF-kappaB and ERK signalling. Br. J. Pharmacol. 153, 557–567. doi: 10.1038/sj.bjp.0707542
Chen, I. S., Chen, Y. C., Chou, C. H., Chuang, R. F., Sheen, L. Y., Chiu, C. H. (2012). Hepatoprotection of silymarin against thioacetamide-induced chronic liver fibrosis. J. Sci. Food Agric. 92, 1441–1447. doi: 10.1002/jsfa.4723
Clichici, S., Olteanu, D., Nagy, A. L., Oros, A., Filip, A., Mircea, P. A. (2015). Silymarin inhibits the progression of fibrosis in the early stages of liver injury in CCl4-treated rats. J. Med. Food 18, 290–298. doi: 10.1089/jmf.2013.0179
Clichici, S., Olteanu, D., Filip, A., Nagy, A. L., Oros, A., Mircea, P. A. (2016). Beneficial Effects of Silymarin After the Discontinuation of CCl4-Induced Liver Fibrosis. J. Med. Food 19, 789–797. doi: 10.1089/jmf.2015.0104
Czauderna, C., Castven, D., Mahn, F. L., Marquardt, J. U. (2019). Context-Dependent Role of NF-κB Signaling in Primary Liver Cancer-from Tumor Development to Therapeutic Implications. Cancers (Basel) 11, 8. doi: 10.3390/cancers11081053
Das, F., Bera, A., Ghosh-Choudhury, N., Abboud, H. E., Kasinath, B. S., Choudhury, G. G. (2014). TGFβ-induced deptor suppression recruits mTORC1 and not mTORC2 to enhance collagen I (α2) gene expression. PloS One 9, e109608. doi: 10.1371/journal.pone.0109608
De Minicis, S., Seki, E., Uchinami, H., Kluwe, J., Zhang, Y., Brenner, D. A., et al. (2007). Gene expression profiles during hepatic stellate cell activation in culture and in vivo. Gastroenterology 132, 1937–1946. doi: 10.1053/j.gastro.2007.02.033
Eaton, J. E., Talwalkar, J. A., Lazaridis, K. N., Gores, G. J., Lindor, K. D. (2013). Pathogenesis of primary sclerosing cholangitis and advances in diagnosis and management. Gastroenterology 145, 521–536. doi: 10.1053/j.gastro.2013.06.052
Eaton, J. E., Nelson, K. M., Gossard, A. A., Carey, E. J., Tabibian, J. H., Lindor, K. D., et al. (2019). Efficacy and safety of curcumin in primary sclerosing cholangitis: an open label pilot study. Scandinav. J. Gastroenterol. 54, 633–639. doi: 10.1080/00365521.2019.1611917
Elsharkawy, A. M., Wright, M. C., Hay, R. T., Arthur, M. J., Hughes, T., Bahr, M. J., et al. (1999). Persistent activation of nuclear factor-kappaB in cultured rat hepatic stellate cells involves the induction of potentially novel Rel-like factors and prolonged changes in the expression of IkappaB family proteins. Hepatology 30, 761–769. doi: 10.1002/hep.510300327
Eun, H. S., Jeong, W. I. (2016). Dual Notch signaling in proinflammatory macrophage activation. Hepatology 63, 1381–1383. doi: 10.1002/hep.28386
Fabris, L., Brivio, S., Cadamuro, M., Strazzabosco, M. (2016). Revisiting Epithelial-to-Mesenchymal Transition in Liver Fibrosis: Clues for a Better Understanding of the “Reactive” Biliary Epithelial Phenotype. Stem Cells Int. 2016, 2953727. doi: 10.1155/2016/2953727
Fabris, L., Spirli, C., Cadamuro, M., Fiorotto, R., Strazzabosco, M. (2017). Emerging concepts in biliary repair and fibrosis. Am. J. Physiol. Gastrointestinal Liver Physiol. 313, G102–G116. doi: 10.1152/ajpgi.00452.2016
Fasbender, F., Widera, A., Hengstler, J. G., Watzl, C. (2016). Natural Killer Cells and Liver Fibrosis. Front. Immunol. 7, 19. doi: 10.3389/fimmu.2016.00019
Federico, A., Niosi, M., Vecchio Blanco, C. D., Loguercio, C. (2008). Emerging drugs for non-alcoholic fatty liver disease. Expert Opin. Emerging. Drugs 13, 145–158. doi: 10.1517/14728214.13.1.145
Federico, A., Dallio, M., Loguercio, C. (2017). Silymarin/Silybin and Chronic Liver Disease: A Marriage of Many Years. Molecules 22, 191–207. doi: 10.3390/molecules22020191
Fickert, P., Fuchsbichler, A., Wagner, M., Zollner, G., Kaser, A., Tilg, H., et al. (2004). Regurgitation of bile acids from leaky bile ducts causes sclerosing cholangitis in Mdr2 (Abcb4) knockout mice. Gastroenterology 127, 261–274. doi: 10.1053/j.gastro.2004.04.009
Fickert, P., Pollheimer, M. J., Beuers, U., Lackner, C., Hirschfield, G., Housset, C., et al. (2014). Characterization of animal models for primary sclerosing cholangitis (PSC). J. Hepatol. 60, 1290–1303. doi: 10.1016/j.jhep.2014.02.006
Foglia, B., Cannito, S., Bocca, C., Parola, M., Novo, E. (2019). ERK Pathway in Activated, Myofibroblast-Like, Hepatic Stellate Cells: A Critical Signaling Crossroad Sustaining Liver Fibrosis. Int. J. Mol. Sci. 20, 2700–2722. doi: 10.3390/ijms20112700
Franceschet, I., Cazzagon, N., Del Ross, T., D'Incà, R., Buja, A., Floreani, A. (2016). Primary sclerosing cholangitis associated with inflammatory bowel disease: an observational study in a Southern Europe population focusing on new therapeutic options. Eur. J. Gastroenterol. Hepatol. 28, 508–513. doi: 10.1097/MEG.0000000000000596
Fu, Y., Zheng, S., Lin, J., Ryerse, J., Chen, A. (2008). Curcumin protects the rat liver from CCl4-caused injury and fibrogenesis by attenuating oxidative stress and suppressing inflammation. Mol. Pharmacol. 73, 399–409. doi: 10.1124/mol.107.039818
Gao, D., Mendoza, A., Lu, S., Lawrence, D. A. (2012). Immunomodulatory Effects of Danshen (Salvia miltiorrhiza) in BALB/c Mice. ISRN Inflammation 2012, 954032. doi: 10.5402/2012/954032
Geisler, F., Strazzabosco, M. (2015). Emerging roles of Notch signaling in liver disease. Hepatology 61, 382–392. doi: 10.1002/hep.27268
Giordano, D. M., Pinto, C., Maroni, L., Benedetti, A., Marzioni, M. (2018). Inflammation and the Gut-Liver Axis in the Pathophysiology of Cholangiopathies. Int. J. Mol. Sci. 19, 3003–3021. doi: 10.3390/ijms19103003
Hazra, S., Miyahara, T., Rippe, R. A., Tsukamoto, H. (2004a). PPAR Gamma and Hepatic Stellate Cells. Comp. Hepatol. 3 (Suppl 1), S7. doi: 10.1186/1476-5926-2-S1-S7
Hazra, S., Xiong, S., Wang, J., Rippe, R. A., Krishna, V., Chatterjee, K., et al. (2004b). Peroxisome proliferator-activated receptor gamma induces a phenotypic switch from activated to quiescent hepatic stellate cells. J. Biol. Chem. 279, 11392–11401. doi: 10.1074/jbc.M310284200
He, Y., Yue, Y., Zheng, X., Zhang, K., Chen, S., Du, Z. (2015). Curcumin, inflammation, and chronic diseases: how are they linked? Molecules 20, 9183–9213. doi: 10.3390/molecules20059183
Hernández-Gea, V., Ghiassi-Nejad, Z., Rozenfeld, R., Gordon, R., Fiel, M. I., Yue, Z., et al. (2012). Autophagy releases lipid that promotes fibrogenesis by activated hepatic stellate cells in mice and in human tissues. Gastroenterology 142, 938–946. doi: 10.1053/j.gastro.2011.12.044
Hernández-Ortega, L. D., Alcántar-Díaz, B. E., Ruiz-Corro, L. A., Sandoval-Rodriguez, A., Bueno-Topete, M., Armendariz-Borunda, J., et al. (2012). Quercetin improves hepatic fibrosis reducing hepatic stellate cells and regulating pro-fibrogenic/anti-fibrogenic molecules balance. J. Gastroenterol. Hepatol. 27, 1865–1872. doi: 10.1111/j.1440-1746.2012.07262.x
Jeong, W. I., Park, O., Suh, Y. G., Byun, J. S., Park, S. Y., Choi, E., et al. (2011). Suppression of innate immunity (natural killer cell/interferon-γ) in the advanced stages of liver fibrosis in mice. Hepatology 53, 1342–1351. doi: 10.1002/hep.24190
Ji, S. G., Juran, B. D., Mucha, S., Folseraas, T., Jostins, L., Melum, E., et al. (2017). Genome-wide association study of primary sclerosing cholangitis identifies new risk loci and quantifies the genetic relationship with inflammatory bowel disease. Nat. Genet. 49, 269–273. doi: 10.1038/ng.3745
Jurenka, J. S. (2009). Anti-inflammatory properties of curcumin, a major constituent of Curcuma longa: a review of preclinical and clinical research. Altern. Med. Rev. 14, 277.
Kalluri, R., Neilson, E. G. (2003). Epithelial-mesenchymal transition and its implications for fibrosis. J. Clin. Invest. 112, 1776–1784. doi: 10.1172/JCI20530
Kara, E., Coşkun, T., Kaya, Y., Yumuş, O., Vatansever, S., Var, A. (2008). Effects of silymarin and pentoxifylline on matrix metalloproteinase-1 and -2 expression and apoptosis in experimental hepatic fibrosis. Curr. Ther. Res. Clin. Exp. 69, 488–502. doi: 10.1016/j.curtheres.2008.12.003
Karimi, G., Hassanzadeh-Josan, S., Memar, B., Esmaeili, S. A., Riahi-Zanjani, B. (2018). Immunomodulatory effects of silymarin after subacute exposure to mice: A tiered approach immunotoxicity screening. J. Pharmacopunct. 21, 90–97. doi: 10.3831/KPI.2018.21.011
Karlsen, T. H., Franke, A., Melum, E., Kaser, A., Hov, J. R., Balschun, T., et al. (2010). Genome-wide association analysis in primary sclerosing cholangitis. Gastroenterology 138, 1102–1111. doi: 10.1053/j.gastro.2009.11.046
Karlsen, T. H., Folseraas, T., Thorburn, D., Vesterhus, M. (2017). Primary sclerosing cholangitis - a comprehensive review. J. Hepatol. 67, 1298–1323. doi: 10.1016/j.jhep.2017.07.022
Kim, K. H., Chen, C. C., Alpini, G., Lau, L. F. (2015). CCN1 induces hepatic ductular reaction through integrin αvβ5-mediated activation of NF-κB. J. Clin. Invest. 125, 1886–1900. doi: 10.1172/JCI79327
Kimball, A. S., Joshi, A. D., Boniakowski, A. E., Schaller, M., Chung, J., Allen, R., et al. (2017). Notch Regulates Macrophage-Mediated Inflammation in Diabetic Wound Healing. Front. Immunol. 8, 635. doi: 10.3389/fimmu.2017.00635
Latief, U., Ahmad, R. (2017). Herbal remedies for liver fibrosis: A review on the mode of action of fifty herbs. J. Tradit. Complement. Med. 8, 352–360. doi: 10.1016/j.jtcme.2017.07.002
Lazaridis, K. N., Strazzabosco, M., Larusso, N. F. (2004). The cholangiopathies: disorders of biliary epithelia. Gastroenterology 127, 1565–1577. doi: 10.1053/j.gastro.2004.08.006
Lee, S. Y., Cho, S. S., Li, Y., Bae, C. S., Park, K. M., Park, D. H. (2020). Anti-inflammatory Effect of Curcuma longa and Allium hookeri Co-treatment via NF-κB and COX-2 Pathways. Sci. Rep. 10, 5718. doi: 10.1038/s41598-020-62749-7
Lesjak, M., Beara, I., Simin, N., Pintać, D., Majkić, T., Bekvalac, K., et al. (2018). Antioxidant and anti-inflammatory activities of quercetin and its derivatives. J. Funct. Foods 40, 68–75. doi: 10.1016/j.jff.2017.10.047
Levy, C., Lindor, K. D. (2006). Primary sclerosing cholangitis: epidemiology, natural history, and prognosis. Semin. Liver Dis. 26, 22–30. doi: 10.1055/s-2006-933560
Li, Y., Yao, J., Han, C., Yang, J., Chaudhry, M. T., Wang, S., et al. (2016). Quercetin, Inflammation and Immunity. Nutrients 8, 167. doi: 10.3390/nu8030167
Li, X., Jin, Q., Yao, Q., Xu, B., Li, L., Zhang, S., et al. (2018). The Flavonoid Quercetin Ameliorates Liver Inflammation and Fibrosis by Regulating Hepatic Macrophages Activation and Polarization in Mice. Front. Pharmacol. 9, 72. doi: 10.3389/fphar.2018.00072
Lindor, K. D., Kowdley, K. V., Harrison, M. E., American College of Gastroenterology (2015). ACG Clinical Guideline: Primary Sclerosing Cholangitis. Am. J. Gastroenterol. 110, 646–659. doi: 10.1038/ajg.2015.112
Liu, Z., Dou, W., Zheng, Y., Wen, Q., Qin, M., Wang, X., et al. (2016). Curcumin upregulates Nrf2 nuclear translocation and protects rat hepatic stellate cells against oxidative stress. Mol. Med. Rep. 13, 1717–1724. doi: 10.3892/mmr.2015.4690
Loguercio, C., Festi, D. (2011). Silybin and the liver: from basic research to clinical practice. World J. Gastroenterol. 17, 2288–2301. doi: 10.3748/wjg.v17.i18.2288
Loguercio, C., Federico, A., Trappoliere, M., Tuccillo, C., de Sio, I., Di Leva, A., et al. (2007). The effect of a silybin-vitamin e-phospholipid complex on nonalcoholic fatty liver disease: a pilot study. Digestive Dis. Sci. 52, 2387–2395. doi: 10.1007/s10620-006-9703-2
Loguercio, C., Andreone, P., Brisc, C., Brisc, M. C., Bugianesi, E., Chiaramonte, M., et al. (2012). Silybin combined with phosphatidylcholine and vitamin E in patients with nonalcoholic fatty liver disease: a randomized controlled trial. Free Radical Biol. Med. 52, 1658–1665. doi: 10.1016/j.freeradbiomed.2012.02.008
Luangmonkong, T., Suriguga, S., Mutsaers, H. A. M., Groothuis, G. M. M., Olinga, P., Boersema, M. (2018). Targeting Oxidative Stress for the Treatment of Liver Fibrosis. Rev. Physiol. Biochem. Pharmacol. 175, 71–102. doi: 10.1007/112_2018_10
Luedde, T., Schwabe, R. F. (2011). NF-κB in the liver–linking injury, fibrosis and hepatocellular carcinoma. Nat. Rev. Gastroenterol. Hepatol. 8, 108–118. doi: 10.1038/nrgastro.2010.213
Ma, S., Zhang, D., Lou, H., Sun, L., Ji, J. (2016). Evaluation of the anti-inflammatory activities of tanshinones isolated from Salvia miltiorrhiza var. alba roots in THP-1 macrophages. J. Ethnopharmacol. 188, 193–199. doi: 10.1016/j.jep.2016.05.018
Mallat, A., Lotersztajn, S. (2013). Cellular mechanisms of tissue fibrosis. 5. Novel insights into liver fibrosis. Am. J. Physiol. Cell Physiol. 305, C789–C799. doi: 10.1152/ajpcell.00230.2013
Marcolin, E., San-Miguel, B., Vallejo, D., Tieppo, J., Marroni, N., González-Gallego, J., et al. (2012). Quercetin treatment ameliorates inflammation and fibrosis in mice with nonalcoholic steatohepatitis. J. Nutr. 142, 1821–1828. doi: 10.3945/jn.112.165274
Marra, F., Efsen, E., Romanelli, R. G., Caligiuri, A., Pastacaldi, S., Batignani, G., et al. (2000). Ligands of peroxisome proliferator-activated receptor gamma modulate profibrogenic and proinflammatory actions in hepatic stellate cells. Gastroenterology 119, 466–478. doi: 10.1053/gast.2000.9365
Marra, F., DeFranco, R., Robino, G., Novo, E., Efsen, E., Pastacaldi, S., et al. (2005). Thiazolidinedione treatment inhibits bile duct proliferation and fibrosis in a rat model of chronic cholestasis. World J. Gastroenterol. 11, 4931–4938. doi: 10.3748/wjg.v11.i32.4931
McDaniel, K., Meng, F., Wu, N., Sato, K., Venter, J., Bernuzzi, F., et al. (2017). Forkhead box A2 regulates biliary heterogeneity and senescence during cholestatic liver injury in mice. Hepatology 65, 544–559. doi: 10.1002/hep.28831
Melum, E., Franke, A., Schramm, C., Weismüller, T. J., Gotthardt, D. N., Offner, F. A., et al. (2011). Genome-wide association analysis in primary sclerosing cholangitis identifies two non-HLA susceptibility loci. Nat. Genet. 43, 17–19. doi: 10.1038/ng.728
Mertz, A., Nguyen, N. A., Katsanos, K. H., Kwok, R. M. (2019). Primary sclerosing cholangitis and inflammatory bowel disease comorbidity: an update of the evidence. Ann. Gastroenterol. 32, 124–133. doi: 10.20524/aog.2019.0344
Muhanna, N., Abu Tair, L., Doron, S., Amer, J., Azzeh, M., Mahamid, M., et al. (2011). Amelioration of hepatic fibrosis by NK cell activation. Gut 60, 90–98. doi: 10.1136/gut.2010.211136
Navaneethan, U. (2015). Gut Inflammation in Primary Sclerosing Cholangitis And Autoimmune Sclerosing Cholangitis -Contrasting Pattern of Liver-gut Cross Talk. EBioMedicine 2, 1310–1311. doi: 10.1016/j.ebiom.2015.09.046
O'Hara, S. P., Tabibian, J. H., Splinter, P. L., LaRusso, N. F. (2013). The dynamic biliary epithelia: molecules, pathways, and disease. J. Hepatol. 58, 575–582. doi: 10.1016/j.jhep.2012.10.011
O'Hara, S. P., Karlsen, T. H., LaRusso, N. F. (2017). Cholangiocytes and the environment in primary sclerosing cholangitis: where is the link? Gut 66, 1873–1877. doi: 10.1136/gutjnl-2017-314249
O'Toole, A., Alakkari, A., Keegan, D., Doherty, G., Mulcahy, H., O'Donoghue, D. (2012). Primary sclerosing cholangitis and disease distribution in inflammatory bowel disease. Clin. Gastroenterol. Hepatol. 10, 439–441. doi: 10.1016/j.cgh.2011.11.010
Omenetti, A., Porrello, A., Jung, Y., Yang, L., Popov, Y., Choi, S. S., et al. (2008). Hedgehog signaling regulates epithelial-mesenchymal transition during biliary fibrosis in rodents and humans. J. Clin. Invest. 118, 3331–3342. doi: 10.1172/JCI35875
Parajuli, D. R., Park, E. J., Che, X. H., Jiang, W. Y., Kim, Y. C., Sohn, D. H., et al. (2013). PF2401-SF, standardized fraction of Salvia miltiorrhiza, induces apoptosis of activated hepatic stellate cells in vitro and in vivo. Molecules 18, 2122–2134. doi: 10.3390/molecules18022122
Parajuli, D. R., Zhao, Y. Z., Jin, H., Chi, J. H., Li, S. Y., Kim, Y. C., et al. (2015). Anti-fibrotic effect of PF2401-SF, a standardized fraction of Salvia miltiorrhiza, in thioacetamide-induced experimental rats liver fibrosis. Arch. Pharmacal. Res. 38, 549–555. doi: 10.1007/s12272-014-0425-2
Pari, L., Tewas, D., Eckel, J. (2008). Role of curcumin in health and disease. Arch. Physiol. Biochem. 114, 127–149. doi: 10.1080/13813450802033958
Pavanato, A., Tuñón, M. J., Sánchez-Campos, S., Marroni, C. A., Llesuy, S., González-Gallego, J., et al. (2003). Effects of quercetin on liver damage in rats with carbon tetrachloride-induced cirrhosis. Digestive Dis. Sci. 48, 824–829. doi: 10.1023/a:1022869716643
Peng, Y., Yang, T., Huang, K., Shen, L., Tao, Y., Liu, C. (2018). Salvia Miltiorrhiza Ameliorates Liver Fibrosis by Activating Hepatic Natural Killer Cells in Vivo and in Vitro. Front. Pharmacol. 9, 762. doi: 10.3389/fphar.2018.00762
Poli, G. (2000). Pathogenesis of liver fibrosis: role of oxidative stress. Mol. Aspects Med. 21, 49–98. doi: 10.1016/s0098-2997(00)00004-2
Pontecorvi, V., Carbone, M., Invernizzi, P. (2016). The “gut microbiota” hypothesis in primary sclerosing cholangitis. Ann. Trans. Med. 4, 512. doi: 10.21037/atm.2016.12.43
Post-White, J., Ladas, E. J., Kelly, K. M. (2007). Advances in the use of milk thistle (Silybum marianum). Integr. Cancer Ther. 6, 104–109. doi: 10.1177/1534735407301632
Razumilava, N., Gores, G. J., Lindor, K. D. (2011). Cancer surveillance in patients with primary sclerosing cholangitis. Hepatology 54, 1842–1852. doi: 10.1002/hep.24570
Reyes-Gordillo, K., Segovia, J., Shibayama, M., Vergara, P., Moreno, M. G., Muriel, P. (2007). Curcumin protects against acute liver damage in the rat by inhibiting NF-κB, proinflammatory cytokines production and oxidative stress. Biochim. Biophys. Acta 1770, 989–996. doi: 10.1016/j.bbagen.2007.02.004
Russo, M., Spagnuolo, C., Tedesco, I., Bilotto, S., Russo, G. L. (2012). The flavonoid quercetin in disease prevention and therapy: facts and fancies. Biochem. Pharmacol. 83, 6–15. doi: 10.1016/j.bcp.2011.08.010
Rygiel, K. A., Robertson, H., Marshall, H. L., Pekalski, M., Zhao, L., Booth, T. A., et al. (2008). Epithelial-mesenchymal transition contributes to portal tract fibrogenesis during human chronic liver disease. Lab. Invest. 88, 112–123. doi: 10.1038/labinvest.3700704
Sánchez-Valle, V., Chávez-Tapia, N. C., Uribe, M., Méndez-Sánchez, N. (2012). Role of oxidative stress and molecular changes in liver fibrosis: a review. Curr. Med. Chem. 19, 4850–4860. doi: 10.2174/092986712803341520
Sabino, J., Vieira-Silva, S., Machiels, K., Joossens, M., Falony, G., Ballet, V., et al. (2016). Primary sclerosing cholangitis is characterised by intestinal dysbiosis independent from IBD. Gut 65, 1681–1689. doi: 10.1136/gutjnl-2015-311004
Salama, S. M., Abdulla, M. A., AlRashdi, A. S., Ismail, S., Alkiyumi, S. S., Golbabapour, S. (2013). Hepatoprotective effect of ethanolic extract of Curcuma longa on thioacetamide induced liver cirrhosis in rats. BMC Complement. Altern. Med. 13, 56. doi: 10.1186/1472-6882-13-56
Samarghandian, S., Azimi-Nezhad, M., Farkhondeh, T., Samini, F. (2017). Anti-oxidative effects of curcumin on immobilization-induced oxidative stress in rat brain, liver and kidney. Biomed. Pharmacother. 87, 223–229. doi: 10.1016/j.biopha.2016.12.105
Sasatomi, K., Noguchi, K., Sakisaka, S., Sata, M., Tanikawa, K. (1998). Abnormal accumulation of endotoxin in biliary epithelial cells in primary biliary cirrhosis and primary sclerosing cholangitis. J. Hepatol. 29, 409–416. doi: 10.1016/s0168-8278(98)80058-5
Schnabl, B., Bradham, C. A., Bennett, B. L., Manning, A. M., Stefanovic, B., Brenner, D. A. (2001). TAK1/JNK and p38 have opposite effects on rat hepatic stellate cells. Hepatology 34, 953–963. doi: 10.1053/jhep.2001.28790
Shi, J., Zhao, J., Zhang, X., Cheng, Y., Hu, J., Li, Y., et al. (2017). Activated hepatic stellate cells impair NK cell anti-fibrosis capacity through a TGF-β-dependent emperipolesis in HBV cirrhotic patients. Sci. Rep. 7, 44544. doi: 10.1038/srep44544
Stiuso, P., Scognamiglio, I., Murolo, M., Ferranti, P., De Simone, C., Rizzo, M. R., et al. (2014). Serum oxidative stress markers and lipidomic profile to detect NASH patients responsive to an antioxidant treatment: a pilot study. Oxid. Med. Cell. Longevity 2014, 169216. doi: 10.1155/2014/169216
Suri, J., Patwardhan, V., Bonder, A. (2019). Pharmacologic management of primary sclerosing cholangitis: what's in the pipeline? Expert Rev. Gastroenterol. Hepatol. 13, 723–729. doi: 10.1080/17474124.2019.1636647
Tabibian, J. H., O'Hara, S. P., Trussoni, C. E., Tietz, P. S., Splinter, P. L., Mounajjed, T., et al. (2016). Absence of the intestinal microbiota exacerbates hepatobiliary disease in a murine model of primary sclerosing cholangitis. Hepatology 63, 185–196. doi: 10.1002/hep.27927
Tabibian, J. H., Ali, A. H., Lindor, K. D. (2018). Primary Sclerosing Cholangitis, Part 1: Epidemiology, Etiopathogenesis, Clinical Features, and Treatment. Gastroenterol. Hepatol. (N. Y.) 14, 293–304.
Taghavi, S. A., Eshraghian, A., Niknam, R., Sivandzadeh, G. R., Bagheri Lankarani, K. (2018). Diagnosis of cholangiocarcinoma in primary sclerosing cholangitis. Expert Rev. Gastroenterol. Hepatol. 12, 575–584. doi: 10.1080/17474124.2018.1473761
Takakura, W. R., Tabibian, J. H., Bowlus, C. L. (2017). The evolution of natural history of primary sclerosing cholangitis. Curr. Opin. Gastroenterol. 33, 71–77. doi: 10.1097/MOG.0000000000000333
Taniguchi, K., Karin, M. (2018). NF-κB, inflammation, immunity and cancer: coming of age. Nat. Rev. Immunol. 18, 309–324. doi: 10.1038/nri.2017.142
Thoen, L. F., Guimarães, E. L., Dollé, L., Mannaerts, I., Najimi, M., Sokal, E., et al. (2011). A role for autophagy during hepatic stellate cell activation. J. Hepatol. 55, 1353–1360. doi: 10.1016/j.jhep.2011.07.010
Thoen, L. F., Guimarães, E. L., Grunsven, L. A. (2012). Autophagy: a new player in hepatic stellate cell activation. Autophagy 8, 126–128. doi: 10.4161/auto.8.1.18105
Trappoliere, M., Caligiuri, A., Schmid, M., Bertolani, C., Failli, P., Vizzutti, F., et al. (2009). Silybin, a component of sylimarin, exerts anti-inflammatory and anti-fibrogenic effects on human hepatic stellate cells. J. Hepatol. 50, 1102–1111. doi: 10.1016/j.jhep.2009.02.023
Tsai, J. H., Liu, J. Y., Wu, T. T., Ho, P. C., Huang, C. Y., Shyu, J. C., et al. (2008). Effects of silymarin on the resolution of liver fibrosis induced by carbon tetrachloride in rats. J. Viral Hepatitis 15, 508–514. doi: 10.1111/j.1365-2893.2008.00971.x
Visse, R., Nagase, H. (2003). Matrix metalloproteinases and tissue inhibitors of metalloproteinases: structure, function, and biochemistry. Circ. Res. 92, 827–839. doi: 10.1161/01.RES.0000070112.80711.3D
Visseren, T., Darwish Murad, S. (2017). Recurrence of primary sclerosing cholangitis, primary biliary cholangitis and auto-immune hepatitis after liver transplantation. Best Pract. Res. Clin. Gastroenterol. 31, 187–198. doi: 10.1016/j.bpg.2017.04.004
Wang, W. H., Hsuan, K. Y., Chu, L. Y., Lee, C. Y., Tyan, Y. C., Chen, Z. S., et al. (2017). Anticancer Effects of Salvia miltiorrhiza Alcohol Extract on Oral Squamous Carcinoma Cells. Evidence-Based Complement. Altern. Med. 2017, 5364010. doi: 10.1155/2017/5364010
Wang, L., Ma, R., Liu, C., Liu, H., Zhu, R., Guo, S., et al. (2017). Salvia miltiorrhiza: A Potential Red Light to the Development of Cardiovascular Diseases. Curr. Pharmaceut. Design 23, 1077–1097. doi: 10.2174/1381612822666161010105242
Wang, R., Zhang, H., Wang, Y., Song, F., Yuan, Y. (2017). Inhibitory effects of quercetin on the progression of liver fibrosis through the regulation of NF-кB/IкBα, p38 MAPK, and Bcl-2/Bax signaling. Int. Immunopharmacol. 47, 126–133. doi: 10.1016/j.intimp.2017.03.029
Wasser, S., Ho, J. M., Ang, H. K., Tan, C. E. (1998). Salvia miltiorrhiza reduces experimentally-induced hepatic fibrosis in rats. J. Hepatol. 29, 760–771. doi: 10.1016/s0168-8278(98)80257-2
Wu, L., Zhang, Q., Mo, W., Feng, J., Li, S., Li, J., et al. (2017). Quercetin prevents hepatic fibrosis by inhibiting hepatic stellate cell activation and reducing autophagy via the TGF-β1/Smads and PI3K/Akt pathways. Sci. Rep. 7, 9289. doi: 10.1038/s41598-017-09673-5
Xie, T., Ma, S., Lou, H., Zhu, R., Sun, L. (2014). Two novel abietane norditerpenoids with anti-inflammatory properties from the roots of Salvia miltiorrhiza var. alba. Tetrahedron Lett. 55, 7106–7109. doi: 10.1016/j.tetlet.2014.10.153
Xu, J., Fu, Y., Chen, A. (2003). Activation of peroxisome proliferator-activated receptor-gamma contributes to the inhibitory effects of curcumin on rat hepatic stellate cell growth. Am. J. Physiol. Gastrointestinal Liver Physiol. 285, G20–G30. doi: 10.1152/ajpgi.00474.2002
Xu, J., Chi, F., Tsukamoto, H. (2015a). Notch signaling and M1 macrophage activation in obesity-alcohol synergism. Clinics Res. Hepatol. Gastroenterol. 39 (Suppl 1), S24–S28. doi: 10.1016/j.clinre.2015.05.016
Xu, J., Chi, F., Guo, T., Punj, V., Lee, W. N., French, S. W., et al. (2015b). NOTCH reprograms mitochondrial metabolism for proinflammatory macrophage activation. J. Clin. Invest. 125, 1579–1590. doi: 10.1172/JCI76468
Xu, D., Hu, M. J., Wang, Y. Q., Cui, Y. L. (2019). Antioxidant Activities of Quercetin and Its Complexes for Medicinal Application. Molecules 24, pii: E1123. doi: 10.3390/molecules24061123
Yang, W., Ju, J. H., Jeon, M. J., Han, X., Shin, I. (2010). Danshen (Salvia miltiorrhiza) extract inhibits proliferation of breast cancer cells via modulation of Akt activity and p27 level. Phytother. Res. 24, 198–204. doi: 10.1002/ptr.2945
Zhang, K. Q., Bao, Y., Wu, P., Rosen, R. T., Ho, C. T. (1990). Antioxidative components of tanshen (Salvia miltiorrhiza Bung). J. Agric. Food Chem. 38, 1194–1197. doi: 10.1021/jf00095a007
Zhang, Y., Jiang, P., Ye, M., Kim, S. H., Jiang, C., Lü, J. (2012). Tanshinones: sources, pharmacokinetics and anti-cancer activities. Int. J. Mol. Sci. 13, 13621–13666. doi: 10.3390/ijms131013621
Zhao, G. R., Xiang, Z. J., Ye, T. X., Yuan, Y. J., Guo, Z. X. (2006). Antioxidant activities of Salvia miltiorrhiza and Panax notoginseng. Food Chem. 99, 767–774. doi: 10.1016/j.foodchem.2005.09.002
Zheng, S., Chen, A. (2004). Activation of PPARgamma is required for curcumin to induce apoptosis and to inhibit the expression of extracellular matrix genes in hepatic stellate cells in vitro. Biochem. J. 384, 149–157. doi: 10.1042/BJ20040928
Zheng, S., Chen, A. (2006). Curcumin suppresses the expression of extracellular matrix genes in activated hepatic stellate cells by inhibiting gene expression of connective tissue growth factor. Am. J. Physiol. Gastrointestinal Liver Physiol. 290, G883–G893. doi: 10.1152/ajpgi.00450.2005
Keywords: cholangiocytes, hepatic stellate cells, herbal, phytochemicals, primary sclerosing cholangitis, bioflavonoids
Citation: Ceccherini E, Cecchettini A, Morales MA and Rocchiccioli S (2020) The Potentiality of Herbal Remedies in Primary Sclerosing Cholangitis: From In Vitro to Clinical Studies. Front. Pharmacol. 11:813. doi: 10.3389/fphar.2020.00813
Received: 22 January 2020; Accepted: 19 May 2020;
Published: 10 June 2020.
Edited by:
Ludovico Abenavoli, University of Catanzaro, ItalyReviewed by:
Sreenivasan Sasidharan, University of Science, Malaysia, MalaysiaOksana Zayachkivska, Danylo Halytsky Lviv National Medical University, Ukraine
Copyright © 2020 Ceccherini, Cecchettini, Morales and Rocchiccioli. This is an open-access article distributed under the terms of the Creative Commons Attribution License (CC BY). The use, distribution or reproduction in other forums is permitted, provided the original author(s) and the copyright owner(s) are credited and that the original publication in this journal is cited, in accordance with accepted academic practice. No use, distribution or reproduction is permitted which does not comply with these terms.
*Correspondence: Antonella Cecchettini, YW50b25lbGxhLmNlY2NoZXR0aW5pQHVuaXBpLml0