- 1Department of Basic Veterinary Medicine, College of Animal Science and Veterinary Medicine, Jilin University, Changchun, China
- 2Department of Food Quality and Safety, College of Food Science and Engineering, Jilin University, Changchun, China
α-Cyperone, extracted from Cyperus rotundus, has been reported to inhibit microglia-mediated neuroinflammation. Oxidative stress and apoptosis play crucial roles in the course of Parkinson’s disease (PD). PD is a common neurodegenerative disease characterized by selective death of dopaminergic neurons. This study was designed to investigate the neuroprotective effects of α-cyperone against hydrogen peroxide (H2O2)-induced oxidative stress and apoptosis in dopaminergic neuronal SH-SY5Y cells. Neurotoxicity was assessed by MTT assay and the measurement of lactic dehydrogenase (LDH) release. The level of reactive oxygen species (ROS) was measured by dichlorodihydrofluorescin diacetate (DCFH-DA) staining. The apoptosis of SH-SY5Y cells was evaluated by annexin-V-FITC staining. The translocation of NF-E2-related factor 2 (Nrf2) was determined by western blot and immunofluorescence staining. Western blot analysis was conducted to determine the expression level of cleaved-caspase-3, the pro-apoptotic factor Bax, and the anti-apoptotic factor, Bcl-2. The results showed that α-cyperone substantially decreased H2O2-induced death, release of LDH, and the production of ROS in SH-SY5Y cells. In addition, we found that α-cyperone attenuated H2O2-induced cellular apoptosis. Moreover, α-cyperone remarkably reduced the expression of cleaved-caspase-3 and Bax, and upregulated Bcl-2. Furthermore, α-cyperone enhanced the nuclear translocation of Nrf2. Pretreatment with brusatol (BT, an Nrf2 inhibitor) attenuated α-cyperone-mediated suppression of ROS, cleaved-caspase-3, and Bax, as well as α-cyperone-induced Bcl-2 upregulation in H2O2-treated SH-SY5Y cells. α-cyperone neuroprotection required Nrf2 activation. In conclusion, α-cyperone attenuated H2O2-induced oxidative stress and apoptosis in SH-SY5Y cells via the activation of Nrf2, suggesting the potential of this compound in the prevention and treatment of PD.
Introduction
Parkinson’s disease (PD) is the second most common age-associated neurodegenerative disorder, affecting about 2% of subjects older than 60 years and more than 5 million people worldwide (Olanow et al., 2009). PD is mainly characterized by a progressive degeneration of dopaminergic neurons in the substantia nigra pars compacta region of the midbrain (Obeso et al., 2010). Its most common features include resting tremor, rigidity, and bradykinesia (Coelho and Ferreira, 2012). Previous studies have reported that age, genetic, and environmental factors are major determinants in PD pathogenesis. Moreover, the role of oxidative stress and mitochondrial dysfunction is increasingly recognized. For example, it has been demonstrated that oxidative stress and mitochondrial dysfunction can cause neuronal damage and degeneration in PD pathogenesis (Henchcliffe and Beal, 2008; Hauser and Hastings, 2013; Subramaniam and Chesselet, 2013). Therefore, inhibition of these events is a potential strategy to protect dopaminergic neurons.
Oxidative stress is a damaging response resulting from an imbalance between the generation of oxygen-derived radicals and the organism’s antioxidant potential, and implies excessive production of reactive oxygen species (ROS) (Schieber and Chandel, 2014). Excessive ROS production and accumulation damage the structure of cell membranes and the biological functions of lipids, proteins and DNA, and ultimately cause the initiation of cell apoptosis (Ma, 2012). Additionally, hydrogen peroxide (H2O2)-induced ROS production and release can cause a series of oxidative stress responses, which in turn lead to mitochondrial dysfunction, cell damage, and death (Cheng et al., 2010). Apoptosis induced by mitochondrial dysfunction is regulated by the anti-apoptotic protein, Bcl-2, and the pro-apoptotic protein Bax (D’orsi et al., 2017). Caspase-3, a key component of the apoptotic machinery, is activated to generate cleaved-caspase-3 leading to cell death (Porter and Janicke, 1999). Therefore, suppression of cleaved-caspase-3 and Bax, or Bcl-2 upregulation, may prevent neuronal apoptosis due to oxidative stress.
α-Cyperone, extracted from Cyperus rotundus, has been demonstrated to weaken the inflammatory response by microtubule destabilization in the brain (Azimi et al., 2016). Sutalangka et al. has reported Cyperus rotundus has neuroprotective and cognitive-enhancing effects in AF64A-treated rats (Sutalangka and Wattanathorn, 2017). We previously showed that α-cyperone exerts neuroprotective effects by inhibiting microglia-mediated neuroinflammation (Huang et al., 2018). The SH-SY5Y neuroblastoma cell line has been previously used as a cellular model of PD (Xie et al., 2010). H2O2 is commonly used to reproduce oxidative stress in vitro. It has been reported that oxidative stress plays a crucial role in PD (Jenner, 2003; Puspita et al., 2017). Some natural products were reported to prevent oxidative stress in H2O2-treated SH-SY5Y cells (Alvarino et al., 2017; De Oliveira et al., 2017; De Oliveira et al., 2018). However, the effects of α-cyperone in H2O2-treated SH-SY5Y cells have not been explored. Here, we investigated whether α-cyperone prevented oxidative stress-induced apoptosis of SH-SY5Y cells, and addressed the underlying mechanisms.
Materials and Methods
Materials
3-(3,4-Dimethylthiazole-2-yl)-2,5-diphenyltetrazoliumbromide (MTT), brusatol (BT, an Nrf2 inhibitor), and dimethylsulfoxide (DMSO) were obtained from Sigma Aldrich (St Louis, MO, USA). The Bicinchoninic acid protein H2O2 assay (BCA) kit, RIPA lysis buffer, ROS detection kit, and LDH detection kit were obtained from Beyotime Biotechnology (Shanghai, China). α-Cyperone (> 98% purity; Yuan ye Biotech, Shanghai, China) was dissolved in DMSO and freshly diluted to the final concentration of 0.05% DMSO. The Annexin V-FITC/PI Apoptosis Detection Kit was obtained from Solarbio (Beijing Solarbio Science & Technology, Beijing, China). Phosphate buffered saline (PBS), Fetal bovine serum (FBS) and Dulbecco’s modified Eagle’s medium (DMEM) were obtained from Gibco (Grand Island, NY, United States). Primary antibodies against Nrf2, Bax, Bcl-2, cleaved-caspase-3, PCNA, and β-actin were obtained from Proteintech Group (Wuhan China). Secondary goat anti-rabbit or goat anti-mouse antibodies were obtained from Santa Cruz, CA, USA.
Cell culture
The SH-SY5Y cells were obtained from the Cell Culture Center at the Institute of Basic Medical Sciences, Chinese Academy of Medical Sciences (Peking, China). The cell lines were seeded on 25 cm2 cell culture flasks and maintained in DMEM containing 10% FBS, at 37°C in a 5% CO2 incubator. When cells were approximately at 80% confluence, they were subcultured by trypsinization (0.05%, w/v) and seeded into 6-well or 96-well plates. SH-SY5Y cells were cultured for five days with 10 μM retinoic acid (RA) in DMEM plus 1% FBS, 2 mM glutamine and the necessary antibiotics, followed by another five days of culture in serum-free DMEM with BDNF (brain-derived neurotrophic factor, 50 ng/mL), glutamine (2 mM), and antibiotics (P/S). After culture in serum-free DMEM for 4 h, the cells were pretreated with α-cyperone (15 μM or 30 μM) for 2 h and then co-treated with H2O2 (200 µM) for 24 h. To investigate whether the neuroprotective effect of α-cyperone was associated with the activation of Nrf2, SH-SY5Y cells were pretreated with brusatol (BT, an Nrf2 inhibitor dissolved in 0.05% DMSO) for 4 h, co-treated with α-cyperone (30 μM) for 2 h, and then post-treated with H2O2 for 24 h. Control cells were cultured in medium containing 0.05% DMSO.
MTT Assay
SH-SY5Y cells (1 × 104 cells/well) were seeded and cultured into a 96-well plate for 12 h. Cells were supplied with fresh serum-free DMEM for 4 h, pretreated with α-cyperone for 2 h, and then co-treated with H2O2 for 24 h. The cell viability was determined by MTT assay according to the manufacturer’s instructions. First, MTT (0.5 mg/mL) was transferred to the cell cultures, and the cells were incubated for an additional 4 h. The supernatant was removed, DMSO (150 μL/well) was added, and the plates were shaken for 15 min. The absorbance was measured using an absorbance reader (iMark, BioRad, USA) with a testing wavelength of 570 nm and a reference wavelength of 630 nm.
LDH Assay
The release of LDH in the medium was determined by an LDH assay kit in accordance with the supplier’s instructions. In brief, α-cyperone was transferred into the medium for 2 h before co-treatment with H2O2. After stimulation with H2O2 for 24 h, the culture medium (120 μL/well) was collected and transferred to a new 96-well plate. The reaction solution (60 μL/well) was added, and the plate was shaken for 30 min. The release of LDH was evaluated by measuring the absorbance at 490 nm and 600 nm using an absorbance reader (iMark, BioRad, USA).
Measurement of Intracellular ROS
Intracellular ROS was measured with a ROS detection kit according to the manufacturer’s instructions. Briefly, SH-SY5Y cells were seeded into 96-well plates (2 × 104 cells/well) for 12 h, and then incubated with serum-free DMEM for 4 h. The cells were pretreated with α-cyperone for 2 h, and then incubated with 50 µM of DCFH-DA for 30 min prior to stimulation with H2O2 for 10 min to induce ROS generation. The cells were washed twice with PBS (100 μL/well), incubated with PBS (100 μL/well), and then analyzed by a multi-detection reader at excitation and emission wavelengths of 485 and 535 nm, respectively.
Apoptosis Assay
SH-SY5Y cells apoptosis were assessed by Annexin V-FITC/PI Apoptosis Detection Kit. Briefly, cells (1 × 106 cells/well) were seeded in 6-well plates for 12 h and then pretreated with α-cyperone for 2 h followed by co-treatment with H2O2 for an additional 24 h. Cells were mildly washed twice with ice-cold PBS, collected, and centrifuged at 1500 rpm for 5 min at 4 °C. Next, cells were harvested, and the percentage of apoptotic cells was assessed by flow cytometry. Gated cells were separated into four quadrants: early apoptotic cells (Annexin positive/PI negative), necrotic cells (Annexin negative/PI positive), late apoptotic cells (Annexin positive/PI positive), and viable cells (Annexin negative/PI negative). The regions LR, UL, UR, and LL represent early apoptotic, necrotic, late apoptotic, and live cells, respectively.
Western Blot
After treatment with α-cyperone or H2O2, the cells were harvested and lysed in RIPA lysis buffer containing phenyl-methylsulfonyl fluoride (PMSF). The total protein concentration was evaluated by using a BCA kit with bovine serum albumin as a standard. Equal amounts of proteins (30 µg/well) were separated by a 12% SDS-polyacrylamide gel, and then transferred to polyvinylidene difluoride membranes (PVDF: Millipore, Bedford, MA). The membrane was blocked with 5% (w/v) non-fat milk for 2 h at 25°C. The membrane was incubated with the primary and then with the secondary antibody. The membranes were visualized by the ECL western blot detection system according to the manufacturer’s instruction. The band intensities were quantified using Image J gel analysis software. All experiments were performed in triplicate.
Immunofluorescence Assay
SH-SY5Y cells were seeded on poly-L-lysine-coated coverslips in 24-well plates for 12 h, and then treated with α-cyperone (30 μM) for 2 h. To analyze the intracellular distribution of Nrf2, the cells were harvested and fixed with Immunol Staining Fix Solution for 10 min. After three washes with PBS, the cells were permeabilized for 10 min, incubated with 5% normal goat serum for 2 h, incubated with the anti-Nrf2 antibody (1:200) overnight, and then washed with PBS. After incubation with the secondary antibody for 1 h, the cells were stained with DAPI. The coverslips were washed with PBS and then mounted onto slides with fluorescent mounting medium. Representative images were chose from 9 fields of view per treatment group.
Nrf2 Silencing by siRNA Transfection
An Nrf2-specific siRNA was used to knock down Nrf2 expression. SH-SY5Y cells (1 × 105 cells/well) were grown in 6-well plates. After treatment with RA and BDNF, the cells were allowed to reach 50%-60% confluence. For each transfection, 80 pmol of Nrf2 siRNA duplex were diluted into 100 μL of siRNA transfection medium (serum-free Opti-MEM). In a separate tube, 4 μL of the transfection reagent, LipofectamineTM 3000, were diluted into 100 μL of siRNA transfection medium (serum-free Opti-MEM). The dilutions were mixed gently and incubated for 20 min at 25°C. Next, 200 μL of the siRNA/Lipofectamine complex were added to cell culture medium serum-free DMEM (2 mL/well). After siRNA transfection for 12 h, the transfected cells were exposed to α-cyperone. The sequences targeting Nrf2 were as follows: sense, 5′ GAA UUA CAG UGU CUU AAU A 3′; antisense, 5′ UAU UAA GAC ACU GUA AUU C 3′.
Statistical Analysis
The data were presented as the means ± S.E.M and analyzed with SPSS 19.0 (IBM). The comparisons between the different experimental groups were evaluated by one-way ANOVA, whereas multiple comparisons were performed using the LSD method. P values < 0.05 were considered statistically significant.
Results
Effect of α-Cyperone on H2O2-Induced Neurotoxicity in SH-SY5Y Cells
To verify possible protective effects of α-cyperone against H2O2-induced neurotoxicity in SH-SY5Y cells, MTT and LDH assays were performed. In brief, SH-SY5Y cells were pretreated with α-cyperone (15 or 30 μM) for 2 h and then incubated with H2O2 (200 μM) for 24 h. We found that 30 μM α-cyperone had no toxic effect on SH-SY5Y cells (Figure 2A). However, the cell viability was remarkably reduced in H2O2-treated cells compared to controls (Figure 2A). The pretreatment with α-cyperone increased the viability of H2O2-treated SH-SY5Y cells (Figure 2A). The release of LDH, a cytosolic enzyme, is a commonly used indicator of decreased cell integrity. Whereas cell treatment with H2O2 increased LDH release (Figure 1B), pretreatment with α-cyperone markedly attenuated this effect (Figure 2B). Taken together, these results revealed that α-cyperone exerted a neuroprotective effect against H2O2-induced neurotoxicity in SH-SY5Y cells.
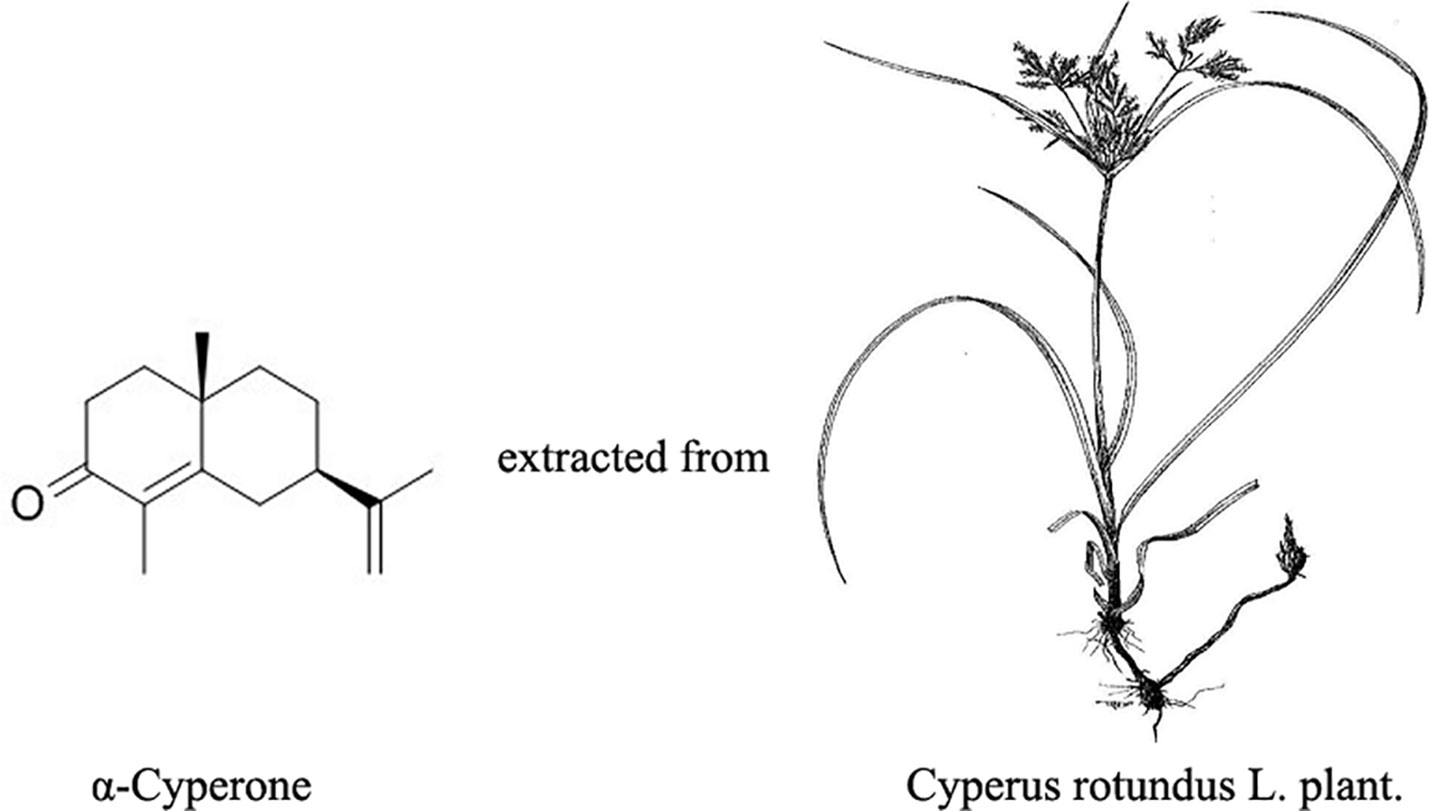
Figure 1 Structure of α-cyperone and Cyperus rotundus L. plant. Cyperus rotundus L. Traditional uses have been summarized and reported (Pirzada et al., 2015; Kamala et al., 2018).
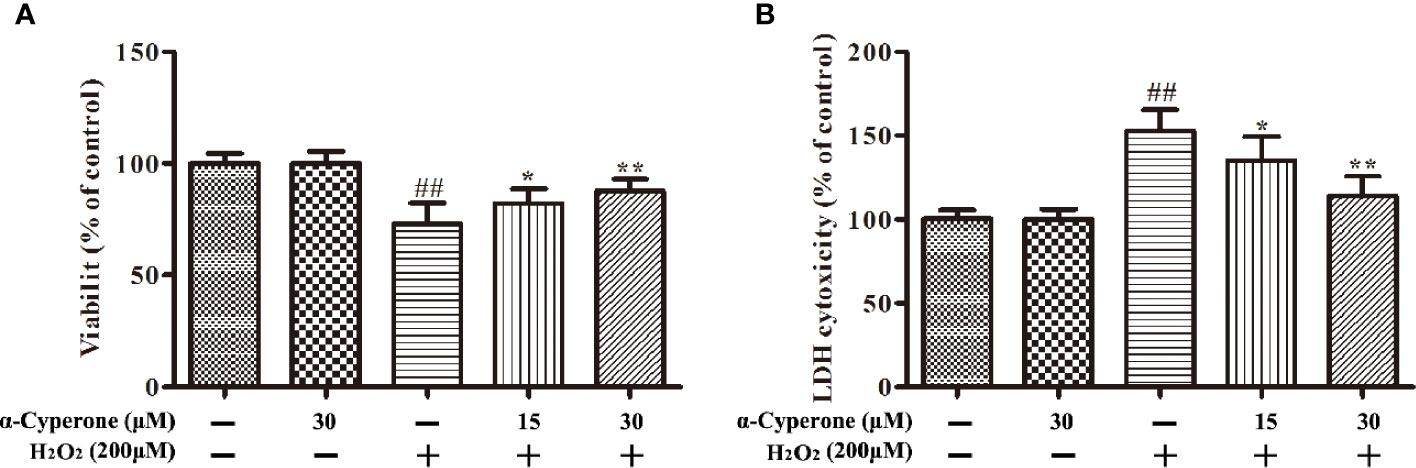
Figure 2 Effect of α-cyperone on H2O2-induced neurotoxicity in SH-SY5Y cells. After being pretreated with α-cyperone (15 or 30 μM) for 2 h, SH-SY5Y cells were stimulated with H2O2 (200 μM) for 24 h. (A) Cell viability was measured by MTT assay. (B) The release of LDH was assessed by LDH assay. All experiments were repeated at least three times and similar results were obtained. Data are presented as the mean ± SE, (n = 5 samples per group). ##p < 0.01 vs. the control group, *p < 0.05 and **p < 0.01 vs. the H2O2-treated group.
Effect of α-Cyperone on H2O2-Induced ROS Production in SH-SY5Y Cells
Mitochondria are the major source of ROS in mammalian cells, and excessive ROS production causes oxidative stress and mitochondrial dysfunction, resulting in cell apoptosis. The production of ROS in SH-SY5Y cells was measured using a DCFH-DA fluorescence assay. We found that 30 μM α-cyperone alone did not affect ROS production (Figure 3). Treatment with H2O2 markedly increased the intracellular level of ROS in SH-SY5Y cells (Figure 3). However, pretreatment with α-cyperone dramatically prevented ROS production (Figure 3), suggesting that the compound protected dopaminergic neurons against oxidative stress damage.
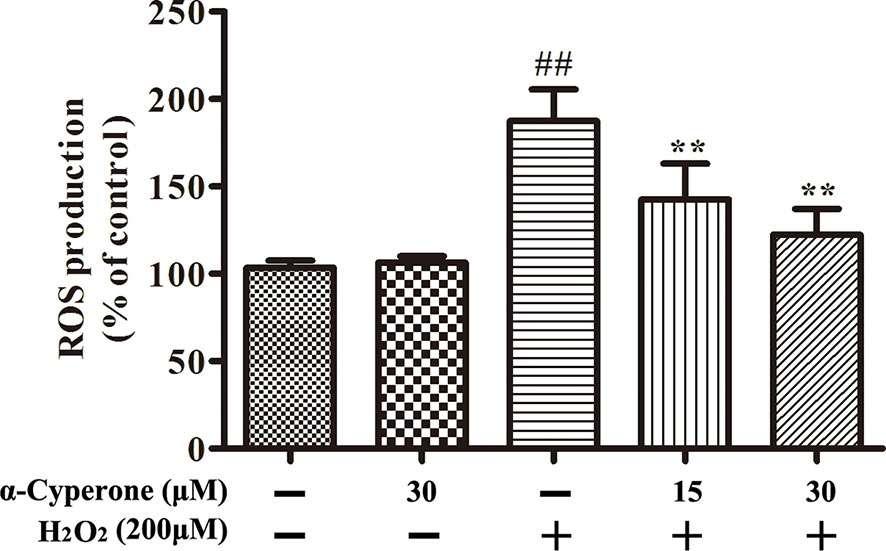
Figure 3 Effect of α-cyperone on H2O2-induced ROS in SH-SY5Y cells. After treatment with α-cyperone (15 or 30 μM) for 2 h, SH-SY5Y cells were incubated with 50 µM of DCFH-DA for 30 min prior to stimulation with H2O2 (200 μM) for 10 min. The production of ROS was determined by an ROS detection kit. All experiments were repeated at least three times and similar results were obtained. Data are presented as the mean ± SE, (n= 5 samples per group). ##p < 0.01 vs. the control group, **p < 0.01 vs. the H2O2-treated group.
Effect of α-Cyperone on H2O2-Induced Cell Apoptosis
To assess the effect of α-cyperone on H2O2-induced apoptosis in SH-SY5Y cells, the apoptotic rate was measured by flow cytometry. We found that 30 μM α-cyperone alone did not affect the apoptotic rate of SH-SY5Y cells (Figure 4B). After treatment with H2O2 for 24 h, the apoptotic rate of SH-SY5Y cells increased to approximately 50% (Figure 4C). However, pretreatment with α-cyperone clearly reduced the H2O2-induced effect (Figures 4D–F), indicating that α-cyperone inhibited H2O2-induced cell apoptosis. Moreover, whereas the fraction of annexin-negative/PI-positive necrotic cells was about 6.39% after 24 h of incubation with H2O2, pretreatment with 30 μM α-cyperone brought this proportion to about 4.47%.
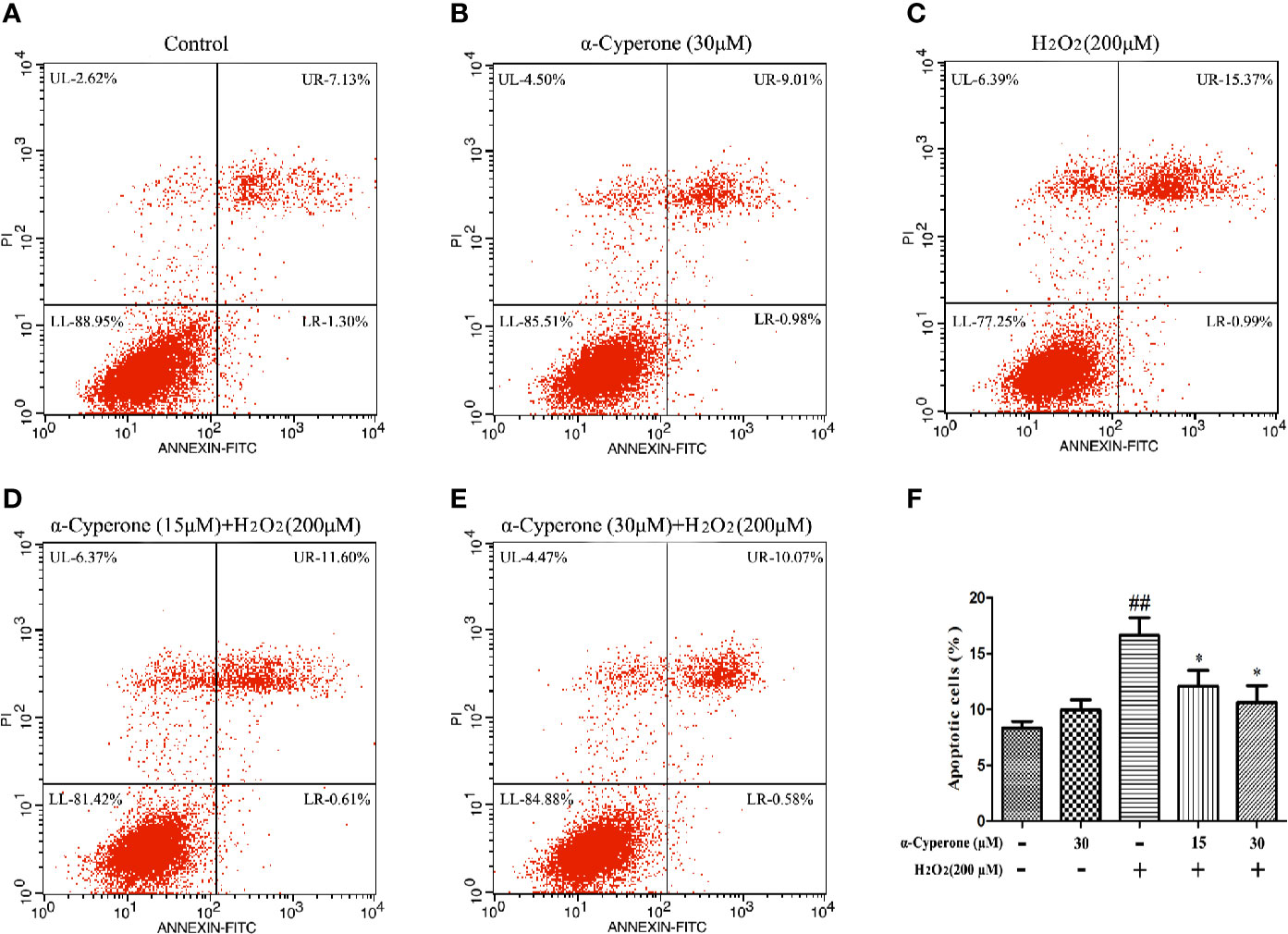
Figure 4 Effect of α-cyperone on H2O2-induced cell apoptosis. After being pretreated with α-cyperone (15 or 30 μM) for 2 h, SH-SY5Y cells were stimulated with H2O2 (200 μM) for 24 h. (A–F) The apoptosis were assessed by an Annexin V-FITC/PI Apoptosis Detection Kit. All experiments were repeated at least three times and similar results were obtained. Data are presented as the mean ± SE, (n= 5 samples per group). ##p < 0.01 vs. the control group, *p < 0.05 vs. the H2O2-treated group.
Effect of α-Cyperone on H2O2-Induced Changes in Bax, Bcl-2, and Cleaved-Caspase-3 Expression
To further explore the effects of α-cyperone on H2O2-induced apoptosis, we analyzed the expression level of Bax, Bcl-2, and cleaved-caspase-3 by western blot. The level of Bax and Bcl-2 expression may be indicative of mitochondrial dysfunction, affecting cell apoptosis. We found that H2O2 upregulated the pro-apoptotic protein, Bax, while pretreatment with α-cyperone attenuated this effect in SH-SY5Y cells (Figures 5A, B). Moreover, 30 μM α-cyperone alone enhanced the expression level of the anti-apoptotic protein, Bcl-2. Notably, while 200 μM H2O2 downregulated Bcl-2 expression, cell pretreatment with α-cyperone reduced the latter effect (Figure 5C). The expression level of cleaved-caspase-3 protein is a marker of cell apoptosis. We found that α-cyperone suppressed cleaved-caspase-3 expression in H2O2-treated SH-SY5Y cells (Figure 5D). Taken together, our results suggested that α-cyperone prevented the apoptosis of dopaminergic neurons due to mitochondrial dysfunction.
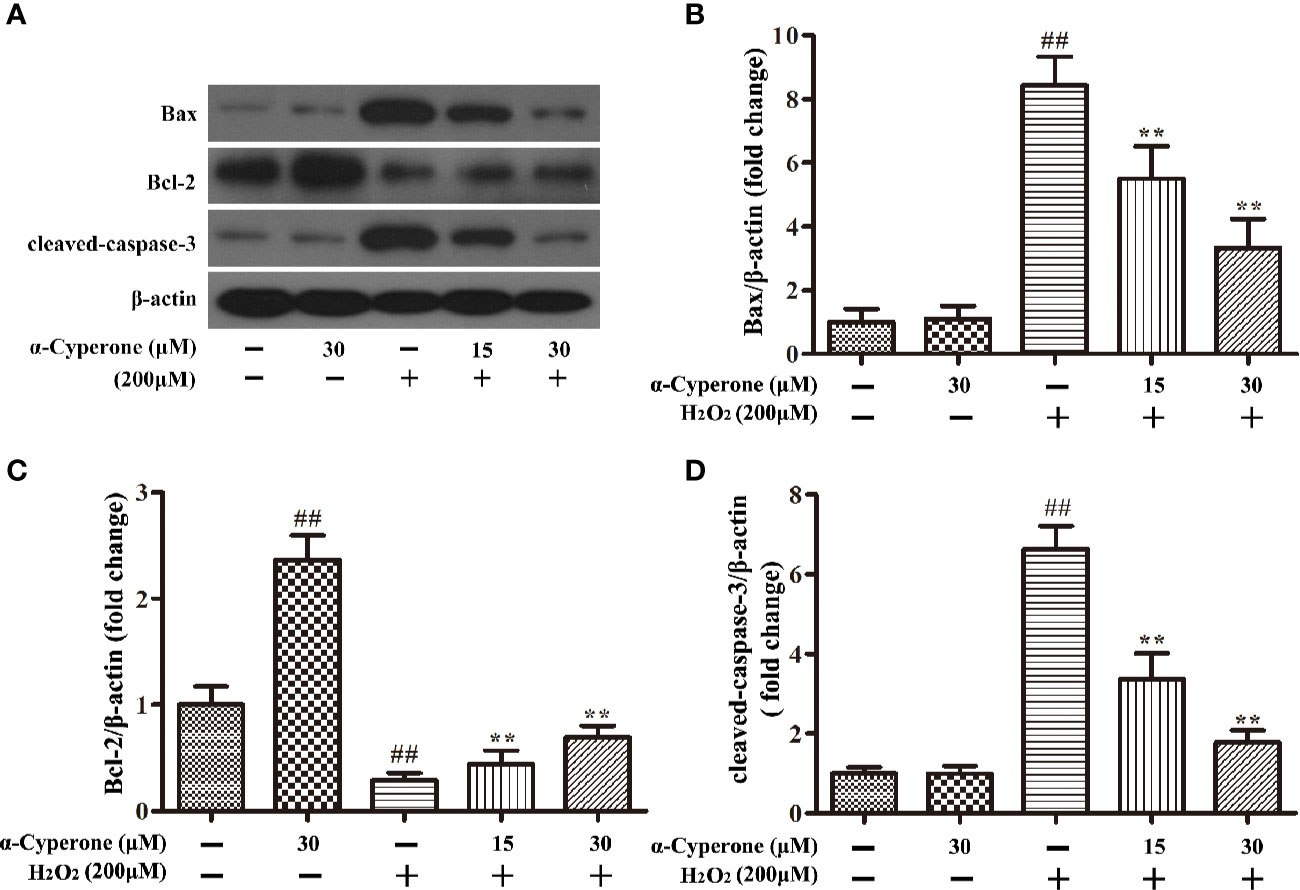
Figure 5 Effect of α-cyperone on Bax, Bcl-2, and cleaved-caspase-3 expression in SH-SY5Y cells pre-challenged with H2O2. After being pretreated with α-cyperone (15 or 30 μM) for 2 h, SH-SY5Y cells were stimulated with H2O2 (200 μM) for 24 h. (A–D) The expression levels of Bax, Bcl-2, and cleaved-caspase-3 were determined by western blot. All experiments were repeated at least three times and similar results were obtained. Data are presented as the mean ± SE, (n= 5 samples per group). ##p < 0.01 vs. the control group, **p < 0.01 vs. the H2O2-treated group.
Effect of α-Cyperone on the Nuclear Translocation of Nrf2 in SH-SY5Y Cells
Nrf2 is a transcription factor regulating basal and inducible transcription of genes encoding factors protective against various oxidative stresses. Nrf2 activation causes its nuclear translocation, leading to the activation of antioxidant or detoxifying genes. To assess the effect of α-cyperone or H2O2 on the activation of Nrf2, we used western blot and immunofluorescence assay to measure Nrf2 nuclear translocation in SH-SY5Y cells. We observed that 30 μM α-cyperone enhanced the nuclear translocation of Nrf2 over a period of 6 h (Figure 6A). However, the effect of α-cyperone on Nrf2 translocation was already detectable after 2 h. And we also found that 30 μM of α-cyperone observably activated the nuclear translocation of Nrf2 for 2 h (Figures 6B, D). In addition, 200 μM H2O2 had no effect on Nrf2 activation in SH-SY5Y cells, as assessed by both western blot and immunofluorescence (Figures 6A, C, E). Overall, these results suggested that α-cyperone promoted the nuclear translocation of Nrf2 in SH-SY5Y cells.
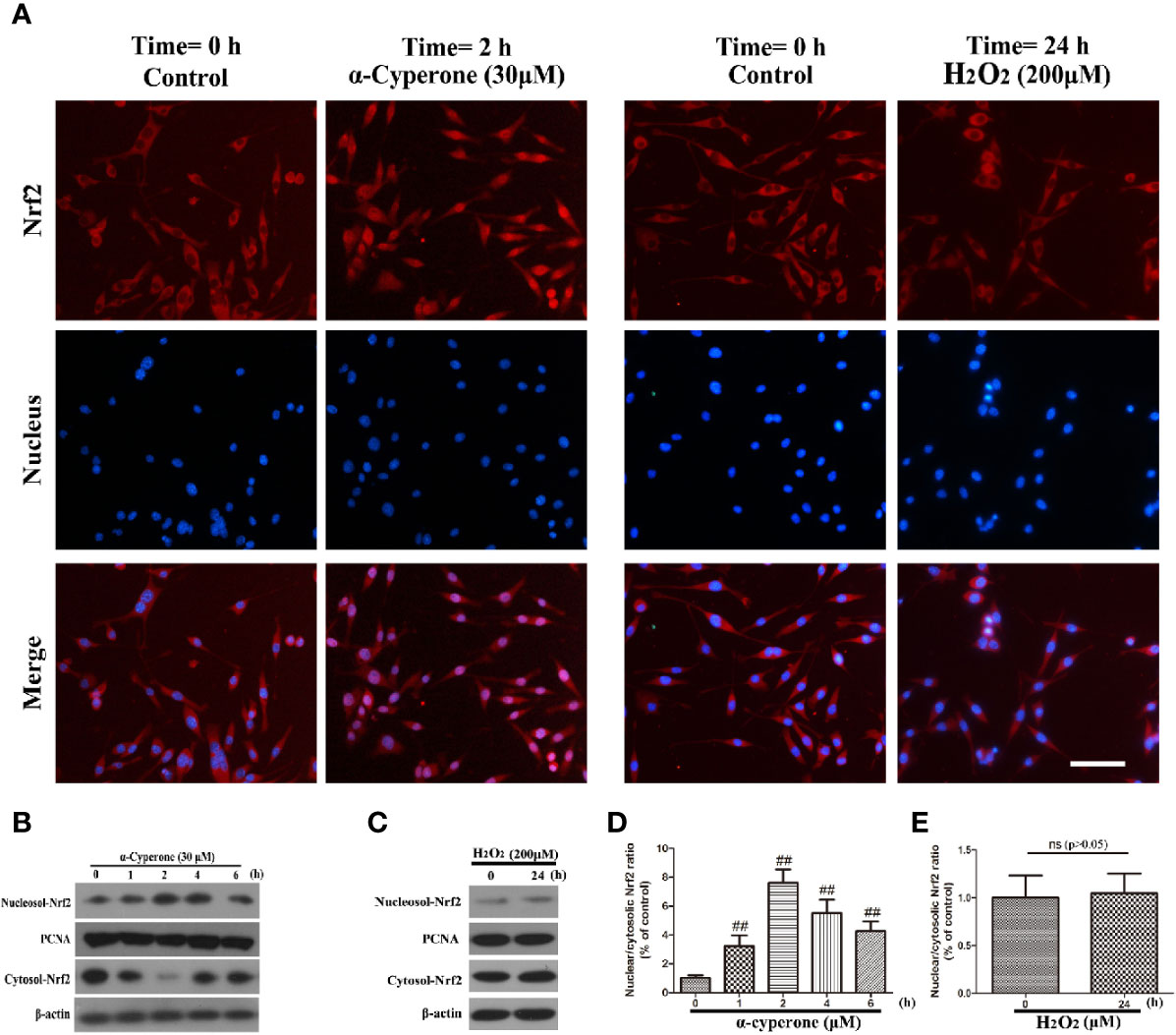
Figure 6 Effects of α-cyperone and H2O2 on the nuclear translocation of Nrf2 in SH-SY5Y cells. (A) SH-SY5Y cells were treated with α-cyperone (30 μM) for 2 h or H2O2 (200 μM) for 24 h, and the nuclear translocation of Nrf2 was determined by immunofluorescence assay. (B–E) After incubation with α-cyperone (30 μM) for various time periods (1-6 h) or with H2O2 (200 μM) for 24 h, the nuclear and cytosolic Nrf2 levels were measured by western blot. All experiments were repeated at least three times and similar results were obtained. Representative photomicrographs of Nrf2 are shown. The scale bar represents 100 μ. Data are presented as the mean ± SE, (n= 5 samples per group). ##p < 0.01 vs. the control group.
α-Cyperone Effects on ROS Production, as Well as on the Expression of Bax, Cleaved-Caspase-3, and Bcl-2, Depend on Nrf2 Activation
To explore whether α-cyperone effects on oxidative stress, mitochondrial dysfunction, and apoptosis were dependent on Nrf2 activation, we pretreated SH-SY5Y cells with BT (an inhibitor of Nrf2) or knocked down Nrf2 expression by specific siRNAs. Western botting results showed that both conditions dramatically attenuated the inhibitory effect of α-cyperone on ROS production, as well as on Bax and cleaved-caspase-3 expression (Figures 7A–C, E). Moreover, the inhibition of Nrf2 activation reduced the expression of the anti-apoptotic protein, Bcl-2 (Figure 7D). MTT, LDH, and ROS assays revealed the absence of cytotoxicity only in cells cultured in DMEM, control cells, and cells transfected with Nrf2-siRNA (not shown). These results indicated that α-cyperone inhibited oxidative stress, mitochondrial dysfunction, and apoptosis in H2O2-treated SH-SY5Y cells via the activation of Nrf2.
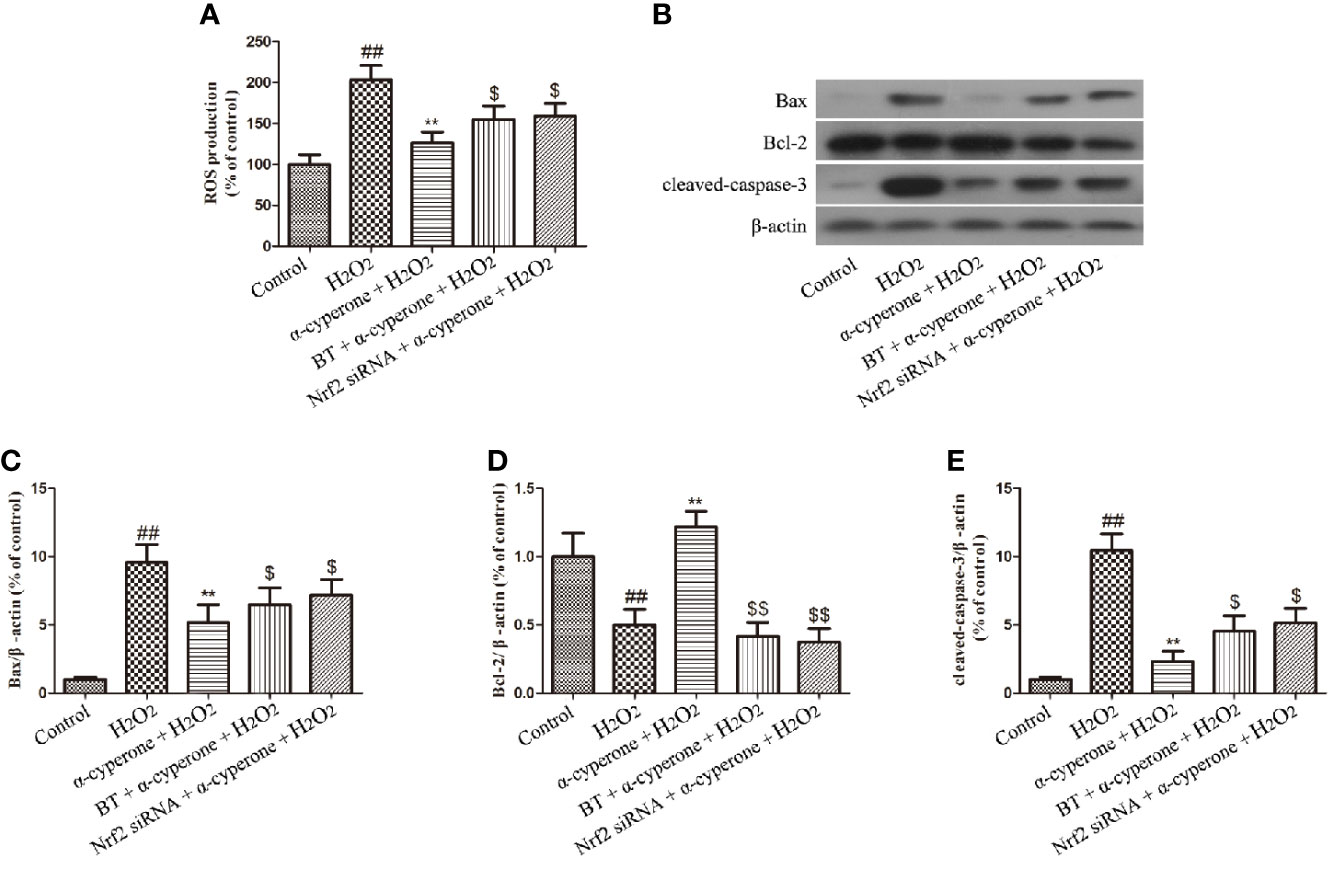
Figure 7 Role of Nrf2 in the protective effects of α-cyperone against H2O2-induced oxidative stress, mitochondrial dysfunction, and apoptosis. After cell pretreatment with the Nrf2 inhibitor, BT (250 nM), for 4 h or with Nrf2 siRNA for 12 h, SH-SY5Y cells were treated with α-cyperone (30 μM) for 2 h. (A) Then, the cells were incubated with 50 µM of DCFH-DA for 30 min prior to stimulation with H2O2 (200 µM) for 10 min. The production of ROS was determined by ROS detection kits. (B-E) The cells were also stimulated with H2O2 (200 µM) for 24 h, and the expression levels of Bax, Bcl-2, and cleaved-caspase-3 were measured by western blot. All experiments were repeated at least three times and similar results were obtained. Data are presented as the mean ± SE, (n= 5 samples per group). ##p < 0.01 vs. the control group, **p < 0.01 vs. the H2O2-treated group, $p < 0.05 and $$p < 0.01 vs. the α-cyperone + H2O2 group.
Discussion
In the present study, we reported the neuroprotective effect of α-cyperone in an in vitro model of PD based on the exposure of SH-SY5Y human neuroblastoma cells to H2O2. This cells have many characteristics of human dopaminergic neuron and, therefore, are commonly employed to in vitro mimic PD alterations. Accumulating evidence has demonstrated that oxidative stress, associated with mitochondrial dysfunction, contributes to the development of PD (Dias et al., 2013; Mullin and Schapira, 2013; Yan et al., 2013; Kong et al., 2014). Our results revealed that α-cyperone reduced the excessive production of ROS, and attenuated mitochondrial dysfunction and cellular apoptosis in H2O2-induced SH-SY5Y cells. Pretreatment with BT (an inhibitor of Nrf2) attenuated the neuroprotective effects of α-cyperone, suggesting that α-cyperone-mediated protection of dopaminergic neurons from oxidative stress-induced apoptosis, as well as from mitochondrial dysfunction, occurred via the activation of Nrf2.
We previously reported that α-cyperone inhibits neuroinflammation in activated-microglia. Moreover, it has been reported that α-cyperone inhibits LPS-induced COX-2 expression and PGE2 production through the negative regulation of NF-κB signaling in RAW 264.7 cells (Jung et al., 2013). To further explore whether α-cyperone protected dopaminergic neurons against oxidative stress-induced damage, we investigated the effect of α-cyperone in H2O2-treated SH-SY5Y cells. We confirmed the neuroprotective effect of α-cyperone against H2O2-induced neurotoxicity by both MTT and LDH assay. Cell pretreatment with H2O2 is known to cause the excessive release of ROS, which initiates DNA and mitochondrial damage, resulting in cell apoptosis (Dai et al., 2014; Ding et al., 2016; Fu et al., 2016). Accumulating evidence has shown that ROS production is associated with oxidative stress and mitochondrial anomalies (Nesi et al., 2017; Sundqvist et al., 2017; Franco-Iborra et al., 2018). We found that α-cyperone reduced the production of ROS in H2O2-treated SH-SY5Y cells. It has been demonstrated that cell apoptosis caused by oxidative stress may be related to various diseases (Cervellati et al., 2014; Paradies et al., 2014; Wu et al., 2014; Li and Hu, 2015). In the present study, α-cyperone notably suppressed the apoptosis of SH-SY5Y cells. To explore this phenomenon, we investigated the effect of α-cyperone on mitochondrial dysfunction. We found that α-cyperone upregulated Bcl-2 and downregulated Bax in H2O2-treated SH-SY5Y cells, suggesting that α-cyperone suppressed mitochondrial dysfunction. Sanga et al. have proved that the inhibition of cleaved-caspase-3 production protects a SH-SY5Y against toxic injury (Sang et al., 2018). In addition, Wu et al. have reported that carnosic acid protects against 6-hydroxydopamine-induced neurotoxicity in in vivo and in vitro models of PD, by inducing antioxidative enzymes and inhibiting the production of cleaved-caspase-3 (Wu et al., 2015). We demonstrated that α-cyperone effectively inhibited the expression of cleaved-caspase-3 in H2O2-induced SH-SY5Y cells. These results suggested that α-cyperone may protect dopaminergic neurons against mitochondrial dysfunction induced by oxidative stress.
The rhizome of C. rotundus has been reported to exert anti-apoptotic and anxiolytic activity in SH-SY5Y cells (Awad et al., 2003; Hemanth Kumar et al., 2013). Moreover, Hu et al. have demonstrated that the essential oil from C. rotundus rhizomes, containing α-cyperone (38.46%), possesses an excellent antioxidant activity, protects against DNA damage in human neuroblastoma SH-SY5Y cells (Hu et al., 2017). We found that α-cyperone promoted the nuclear translocation of Nrf2 in SH-SY5Y cells. Nrf2 is a potent transcriptional activator, controlling the transcription of many cytoprotective genes in response to oxidative stress (Maruyama et al., 2011; Qaisiya et al., 2014). Under normal conditions, Nrf2 is present in the cell cytoplasm and maintains the basal levels of cytoprotective enzymes. Upon activation, Nrf2 translocates to the nucleus and upregulates various cytoprotective enzymes (Baird and Dinkova-Kostova, 2011). Several studies have reported Nrf2-dependent neuroprotective effects of natural products in SH-SY5Y cells (Lou et al., 2014; Jin et al., 2015; Kwon et al., 2015; De Oliveira et al., 2016). We found that cell pretreatment with BT (an inhibitor of Nrf2) attenuated α-cyperone-induced neuroprotection.
ROS may be a critical mediator of H2O2 neurotoxicity. Mitochondria, endoplasmic reticulum, α-synuclein, and dopamine may contribute to oxidative stress in dopaminergic neurons. Our results showed that BT or Nrf2 silencing attenuated α-cyperone effects on cleaved caspase-3 expression in H2O2-treated SH-SY5Y cells (Figure 7E). However, α-cyperone neuroprotection may be associated with other signaling pathways and need be further explored. PD models based on Nrf2 knockout mouse will be a suitable tool to in-depth characterize α-cyperone neuroprotection and its therapeutic potential in the context of PD.
In conclusion, our results showed that α-cyperone exerted neuroprotective effects against H2O2-induced cell apoptosis in SH-SY5Y cells. In addition, α-cyperone inhibited excessive ROS production and mitochondrial dysfunction. Moreover, α-cyperone downregulated the expression of Bax and cleaved-caspase-3, and upregulated Bcl-2 expression. Finally, Nrf2 inhibition attenuated α-cyperone-induced neuroprotection. The neuroprotective actions of α-cyperone are summarized in Figure 8. Taken together, these results suggest that α-cyperone is a potential candidate for the prevention of apoptosis in dopaminergic neurons.
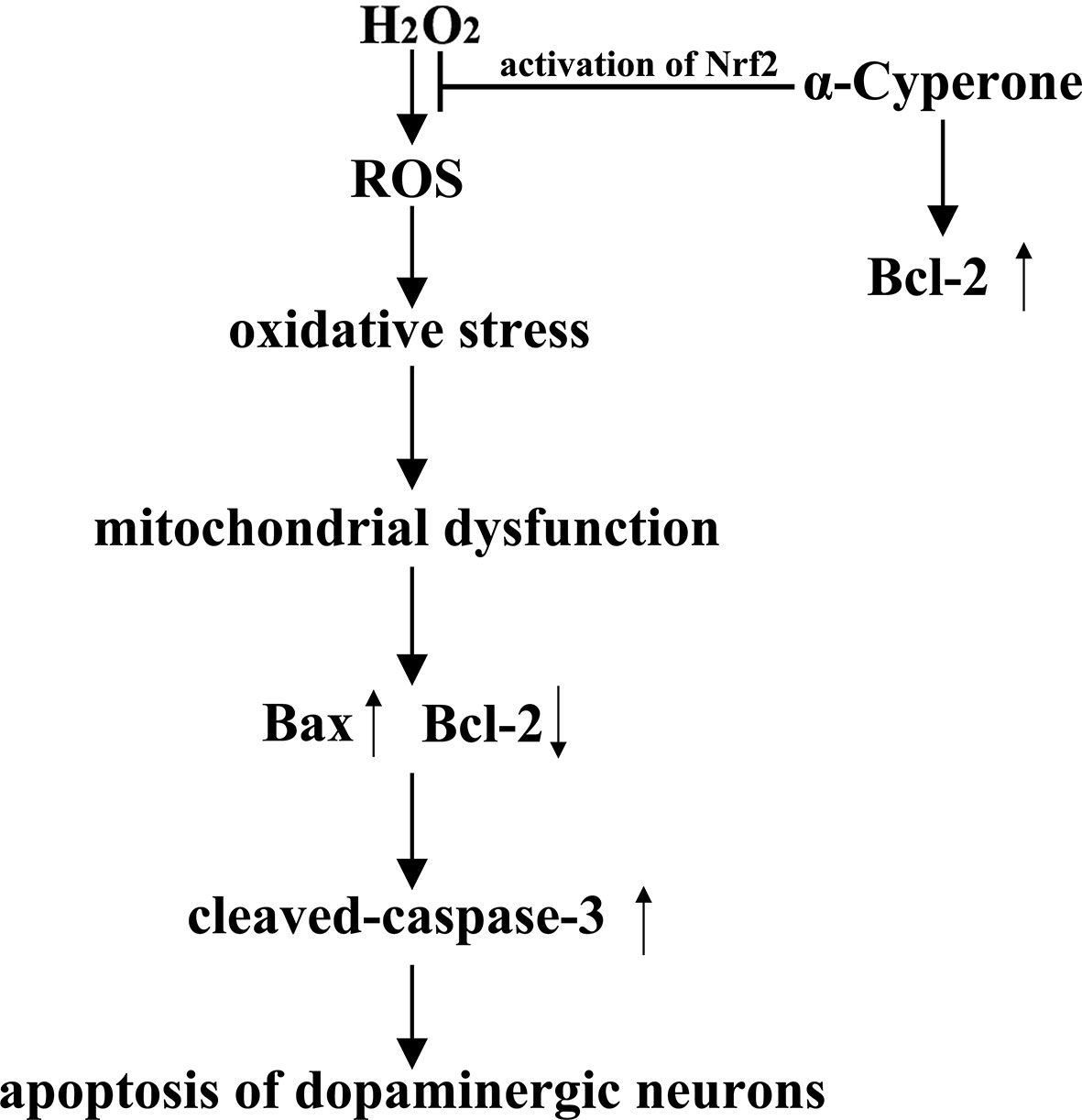
Figure 8 Proposed schematic mechanisms of α-cyperone neuroprotection in an in vitro PD model. α-cyperone attenuates H2O2-induced oxidative stress and apoptosis in SH-SY5Y cells via the activation of Nrf2.
Data Availability Statement
The datasets generated for this study are available on request to the corresponding author.
Author Contributions
JL and DL conceived and designed the study. BH and SF performed the experiments, analyzed the data, and wrote the paper. YL, DH, XR, XY, TM, JD, XG, and YZ collected the samples and information. All authors reviewed the manuscript. In addition, all authors have read and approved the manuscript.
Funding
This work was funded by National Nature Science Foundation of China (project No. 31772547, 31672509), Jilin Scientific and Technological Development Program (project No. 20170623029 TC, 20170623083-04TC), JLU Science and Technology Innovative Research Team.
Conflict of Interest
The authors declare that the research was conducted in the absence of any commercial or financial relationships that could be construed as a potential conflict of interest.
References
Alvarino, R., Alonso, E., Tribalat, M. A., Gegunde, S., Thomas, O. P., Botana, L. M. (2017). Evaluation of the Protective Effects of Sarains on H2O2-Induced Mitochondrial Dysfunction and Oxidative Stress in SH-SY5Y Neuroblastoma Cells. Neurotox Res. 32, 368–380. doi: 10.1007/s12640-017-9748-3
Awad, R., Arnason, J. T., Trudeau, V., Bergeron, C., Budzinski, J. W., Foster, B. C., et al. (2003). Phytochemical and biological analysis of skullcap (Scutellaria lateriflora L.): a medicinal plant with anxiolytic properties. Phytomedicine 10, 640–649. doi: 10.1078/0944-7113-00374
Azimi, A., Ghaffari, S. M., Riazi, G. H., Arab, S. S., Tavakol, M. M., Pooyan, S. (2016). alpha-Cyperone of Cyperus rotundus is an effective candidate for reduction of inflammation by destabilization of microtubule fibers in brain. J. Ethnopharmacol. 194, 219–227. doi: 10.1016/j.jep.2016.06.058
Baird, L., Dinkova-Kostova, A. T. (2011). The cytoprotective role of the Keap1-Nrf2 pathway. Arch. Toxicol. 85, 241–272. doi: 10.1007/s00204-011-0674-5
Cervellati, F., Cervellati, C., Romani, A., Cremonini, E., Sticozzi, C., Belmonte, G., et al. (2014). Hypoxia induces cell damage via oxidative stress in retinal epithelial cells. Free Radic. Res. 48, 303–312. doi: 10.3109/10715762.2013.867484
Cheng, W. Y., Tong, H., Miller, E. W., Chang, C. J., Remington, J., Zucker, R. M., et al. (2010). An integrated imaging approach to the study of oxidative stress generation by mitochondrial dysfunction in living cells. Environ. Health Perspect. 118, 902–908. doi: 10.1289/ehp.0901811
Coelho, M., Ferreira, J. J. (2012). Late-stage Parkinson disease. Nat. Rev. Neurol. 8, 435–442. doi: 10.1038/nrneurol.2012.126
D’orsi, B., Mateyka, J., Prehn, J. H. M. (2017). Control of mitochondrial physiology and cell death by the Bcl-2 family proteins Bax and Bok. Neurochem. Int. 109, 162–170. doi: 10.1016/j.neuint.2017.03.010
Dai, S. H., Chen, T., Wang, Y. H., Zhu, J., Luo, P., Rao, W., et al. (2014). Sirt3 protects cortical neurons against oxidative stress via regulating mitochondrial Ca2+ and mitochondrial biogenesis. Int. J. Mol. Sci. 15, 14591–14609. doi: 10.3390/ijms150814591
De Oliveira, M. R., Peres, A., Ferreira, G. C., Schuck, P. F., Bosco, S. M. (2016). Carnosic Acid Affords Mitochondrial Protection in Chlorpyrifos-Treated Sh-Sy5y Cells. Neurotox Res. 30, 367–379. doi: 10.1007/s12640-016-9620-x
De Oliveira, M. R., Brasil, F. B., Andrade, C. M. B. (2017). Naringenin Attenuates H2O2-Induced Mitochondrial Dysfunction by an Nrf2-Dependent Mechanism in SH-SY5Y Cells. Neurochem. Res. 42, 3341–3350. doi: 10.1007/s11064-017-2376-8
De Oliveira, M. R., Da Costa Ferreira, G., Brasil, F. B., Peres, A. (2018). Pinocembrin Suppresses H2O2-Induced Mitochondrial Dysfunction by a Mechanism Dependent on the Nrf2/HO-1 Axis in SH-SY5Y Cells. Mol. Neurobiol. 55, 989–1003. doi: 10.1007/s12035-016-0380-7
Dias, V., Junn, E., Mouradian, M. M. (2013). The role of oxidative stress in Parkinson’s disease. J. Parkinsons Dis. 3, 461–491. doi: 10.3233/JPD-130230
Ding, X., Wang, D., Li, L., Ma, H. (2016). Dehydroepiandrosterone ameliorates H2O2-induced Leydig cells oxidation damage and apoptosis through inhibition of ROS production and activation of PI3K/Akt pathways. Int. J. Biochem. Cell Biol. 70, 126–139. doi: 10.1016/j.biocel.2015.11.018
Franco-Iborra, S., Vila, M., Perier, C. (2018). Mitochondrial Quality Control in Neurodegenerative Diseases: Focus on Parkinson’s Disease and Huntington’s Disease. Front. Neurosci. 12, 342. doi: 10.3389/fnins.2018.00342
Fu, X. Y., Yang, M. F., Cao, M. Z., Li, D. W., Yang, X. Y., Sun, J. Y., et al. (2016). Strategy to Suppress Oxidative Damage-Induced Neurotoxicity in PC12 Cells by Curcumin: the Role of ROS-Mediated DNA Damage and the MAPK and AKT Pathways. Mol. Neurobiol. 53, 369–378. doi: 10.1007/s12035-014-9021-1
Hauser, D. N., Hastings, T. G. (2013). Mitochondrial dysfunction and oxidative stress in Parkinson’s disease and monogenic parkinsonism. Neurobiol. Dis. 51, 35–42. doi: 10.1016/j.nbd.2012.10.011
Hemanth Kumar, K., Tamatam, A., Pal, A., Khanum, F. (2013). Neuroprotective effects of Cyperus rotundus on SIN-1 induced nitric oxide generation and protein nitration: ameliorative effect against apoptosis mediated neuronal cell damage. Neurotoxicology 34, 150–159. doi: 10.1016/j.neuro.2012.11.002
Henchcliffe, C., Beal, M. F. (2008). Mitochondrial biology and oxidative stress in Parkinson disease pathogenesis. Nat. Clin. Pract. Neurol. 4, 600–609. doi: 10.1038/ncpneuro0924
Hu, Q. P., Cao, X. M., Hao, D. L., Zhang, L. L. (2017). Chemical Composition, Antioxidant, DNA Damage Protective, Cytotoxic and Antibacterial Activities of Cyperus rotundus Rhizomes Essential Oil against Foodborne Pathogens. Sci. Rep. 7, 45231. doi: 10.1038/srep45231
Huang, B., He, D., Chen, G., Ran, X., Guo, W., Kan, X., et al. (2018). alpha-Cyperone inhibits LPS-induced inflammation in BV-2 cells through activation of Akt/Nrf2/HO-1 and suppression of the NF-kappaB pathway. Food Funct. 9, 2735–2743. doi: 10.1039/C8FO00057C
Jenner, P. (2003). Oxidative stress in Parkinson’s disease. Ann. Of Neurol. 53, S26–S36. doi: 10.1002/ana.10483
Jin, X., Liu, Q., Jia, L., Li, M., Wang, X. (2015). Pinocembrin attenuates 6-OHDA-induced neuronal cell death through Nrf2/ARE pathway in SH-SY5Y cells. Cell Mol. Neurobiol. 35, 323–333. doi: 10.1007/s10571-014-0128-8
Jung, S. H., Kim, S. J., Jun, B. G., Lee, K. T., Hong, S. P., Oh, M. S., et al. (2013). alpha-Cyperone, isolated from the rhizomes of Cyperus rotundus, inhibits LPS-induced COX-2 expression and PGE2 production through the negative regulation of NFkappaB signalling in RAW 264.7 cells. J. Ethnopharmacol. 147, 208–214. doi: 10.1016/j.jep.2013.02.034
Kamala, A., Middha, S. K., Karigar, C. S. (2018). Plants in traditional medicine with special reference to Cyperus rotundus L.: a review. 3 Biotech. 8, 309. doi: 10.1007/s13205-018-1328-6
Kong, Y., Trabucco, S. E., Zhang, H. (2014). Oxidative stress, mitochondrial dysfunction and the mitochondria theory of aging. Interdiscip. Top. Gerontol. 39, 86–107. doi: 10.1159/000358901
Kwon, S. H., Ma, S. X., Hwang, J. Y., Lee, S. Y., Jang, C. G. (2015). Involvement of the Nrf2/HO-1 signaling pathway in sulfuretin-induced protection against amyloid beta25-35 neurotoxicity. Neuroscience 304, 14–28. doi: 10.1016/j.neuroscience.2015.07.030
Li, L., Hu, G. K. (2015). Pink1 protects cortical neurons from thapsigargin-induced oxidative stress and neuronal apoptosis. Biosci. Rep. 35 (1). doi: 10.1042/BSR20140104
Lou, H., Jing, X., Wei, X., Shi, H., Ren, D., Zhang, X. (2014). Naringenin protects against 6-OHDA-induced neurotoxicity via activation of the Nrf2/ARE signaling pathway. Neuropharmacology 79, 380–388. doi: 10.1016/j.neuropharm.2013.11.026
Ma, Z. A. (2012). The role of peroxidation of mitochondrial membrane phospholipids in pancreatic beta -cell failure. Curr. Diabetes Rev. 8, 69–75. doi: 10.2174/157339912798829232
Maruyama, A., Nishikawa, K., Kawatani, Y., Mimura, J., Hosoya, T., Harada, N., et al. (2011). The novel Nrf2-interacting factor KAP1 regulates susceptibility to oxidative stress by promoting the Nrf2-mediated cytoprotective response. Biochem. J. 436, 387–397. doi: 10.1042/BJ20101748
Mullin, S., Schapira, A. (2013). alpha-Synuclein and mitochondrial dysfunction in Parkinson’s disease. Mol. Neurobiol. 47, 587–597. doi: 10.1007/s12035-013-8394-x
Nesi, G., Sestito, S., Digiacomo, M., Rapposelli, S. (2017). Oxidative Stress, Mitochondrial Abnormalities and Proteins Deposition: Multitarget Approaches in Alzheimer’s Disease. Curr. Top. Med. Chem. 17, 3062–3079. doi: 10.2174/1568026617666170607114232
Obeso, J. A., Rodriguez-Oroz, M. C., Goetz, C. G., Marin, C., Kordower, J. H., Rodriguez, M., et al. (2010). Missing pieces in the Parkinson’s disease puzzle. Nat. Med. 16, 653–661. doi: 10.1038/nm.2165
Olanow, C. W., Stern, M. B., Sethi, K. (2009). The scientific and clinical basis for the treatment of Parkinson disease. Neurology 72, S1–136. doi: 10.1212/WNL.0b013e3181a1d44c
Paradies, G., Paradies, V., Ruggiero, F. M., Petrosillo, G. (2014). Oxidative stress, cardiolipin and mitochondrial dysfunction in nonalcoholic fatty liver disease. World J. Gastroenterol. 20, 14205–14218. doi: 10.3748/wjg.v20.i39.14205
Pirzada, A. M., Ali, H. H., Naeem, M., Latif, M., Bukhari, A. H., Tanveer, A. (2015). Cyperus rotundus L.: Traditional uses, phytochemistry, and pharmacological activities. J. Ethnopharmacol. 174, 540–560. doi: 10.1016/j.jep.2015.08.012
Porter, A. G., Janicke, R. U. (1999). Emerging roles of caspase-3 in apoptosis. Cell Death Differ. 6, 99–104. doi: 10.1038/sj.cdd.4400476
Puspita, L., Chung, S. Y., Shim, J. W. (2017). Oxidative stress and cellular pathologies in Parkinson’s disease. Mol. Brain 10, 53. doi: 10.1186/s13041-017-0340-9
Qaisiya, M., Coda Zabetta, C. D., Bellarosa, C., Tiribelli, C. (2014). Bilirubin mediated oxidative stress involves antioxidant response activation via Nrf2 pathway. Cell Signal 26, 512–520. doi: 10.1016/j.cellsig.2013.11.029
Sang, Q., Liu, X., Wang, L., Qi, L., Sun, W., Wang, W., et al. (2018). Curcumin Protects an SH-SY5Y Cell Model of Parkinson’s Disease Against Toxic Injury by Regulating HSP90. Cell Physiol. Biochem. 51, 681–691. doi: 10.1159/000495326
Schieber, M., Chandel, N. S. (2014). ROS function in redox signaling and oxidative stress. Curr. Biol. 24, R453–R462. doi: 10.1016/j.cub.2014.03.034
Subramaniam, S. R., Chesselet, M. F. (2013). Mitochondrial dysfunction and oxidative stress in Parkinson’s disease. Prog. Neurobiol. 106–107, 17–32. doi: 10.1016/j.pneurobio.2013.04.004
Sundqvist, M., Christenson, K., Bjornsdottir, H., Osla, V., Karlsson, A., Dahlgren, C., et al. (2017). Elevated Mitochondrial Reactive Oxygen Species and Cellular Redox Imbalance in Human NADPH-Oxidase-Deficient Phagocytes. Front. Immunol. 8, 1828. doi: 10.3389/fimmu.2017.01828
Sutalangka, C., Wattanathorn, J. (2017). Neuroprotective and cognitive-enhancing effects of the combined extract of Cyperus rotundus and Zingiber officinale. BMC Complement Altern. Med. 17, 135. doi: 10.1186/s12906-017-1632-4
Wu, Y. T., Wu, S. B., Wei, Y. H. (2014). Metabolic reprogramming of human cells in response to oxidative stress: implications in the pathophysiology and therapy of mitochondrial diseases. Curr. Pharm. Des. 20, 5510–5526. doi: 10.2174/1381612820666140306103401
Wu, C. R., Tsai, C. W., Chang, S. W., Lin, C. Y., Huang, L. C., Tsai, C. W. (2015). Carnosic acid protects against 6-hydroxydopamine-induced neurotoxicity in in vivo and in vitro model of Parkinson’s disease: involvement of antioxidative enzymes induction. Chem. Biol. Interact. 225, 40–46. doi: 10.1016/j.cbi.2014.11.011
Xie, H. R., Hu, L. S., Li, G. Y. (2010). SH-SY5Y human neuroblastoma cell line: in vitro cell model of dopaminergic neurons in Parkinson’s disease. Chin Med. J. (Engl) 123, 1086–1092.
Keywords: α-cyperone, oxidative stress, apoptosis, ROS, Nrf2
Citation: Huang B, Liu J, Fu S, Zhang Y, Li Y, He D, Ran X, Yan X, Du J, Meng T, Gao X and Liu D (2020) α-Cyperone Attenuates H2O2-Induced Oxidative Stress and Apoptosis in SH-SY5Y Cells via Activation of Nrf2. Front. Pharmacol. 11:281. doi: 10.3389/fphar.2020.00281
Received: 20 February 2019; Accepted: 27 February 2020;
Published: 08 April 2020.
Edited by:
Carlos Alberto Manssour Fraga, Federal University of Rio de Janeiro, BrazilReviewed by:
Juan Andrés Parga, University of Santiago de Compostela, SpainVíctor López, Universidad San Jorge, Spain
Copyright © 2020 Huang, Liu, Fu, Zhang, Li, He, Ran, Yan, Du, Meng, Gao and Liu. This is an open-access article distributed under the terms of the Creative Commons Attribution License (CC BY). The use, distribution or reproduction in other forums is permitted, provided the original author(s) and the copyright owner(s) are credited and that the original publication in this journal is cited, in accordance with accepted academic practice. No use, distribution or reproduction is permitted which does not comply with these terms.
*Correspondence: Dianfeng Liu, ccldf@163.com
†These authors have contributed equally to this work