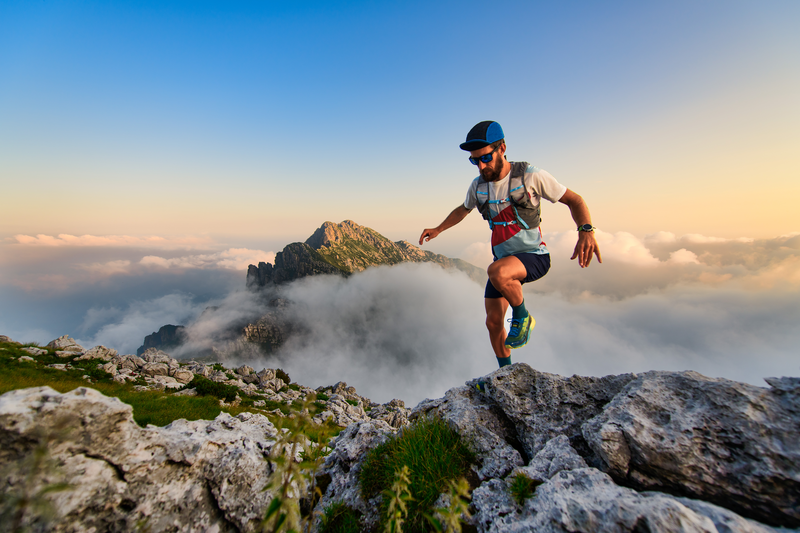
94% of researchers rate our articles as excellent or good
Learn more about the work of our research integrity team to safeguard the quality of each article we publish.
Find out more
REVIEW article
Front. Pharmacol. , 13 March 2020
Sec. Experimental Pharmacology and Drug Discovery
Volume 11 - 2020 | https://doi.org/10.3389/fphar.2020.00222
This article is part of the Research Topic Emerging Mechanisms in Purinergic Signaling: from Cell Biology to Therapeutic Perspectives View all 10 articles
Although often overlooked in our daily lives, saliva performs a host of necessary physiological functions, including lubricating and protecting the oral cavity, facilitating taste sensation and digestion and maintaining tooth enamel. Therefore, salivary gland dysfunction and hyposalivation, often resulting from pathogenesis of the autoimmune disease Sjögren’s syndrome or from radiotherapy of the head and neck region during cancer treatment, severely reduce the quality of life of afflicted patients and can lead to dental caries, periodontitis, digestive disorders, loss of taste and difficulty speaking. Since their initial discovery in the 1970s, P2 purinergic receptors for extracellular nucleotides, including ATP-gated ion channel P2X and G protein-coupled P2Y receptors, have been shown to mediate physiological processes in numerous tissues, including the salivary glands where P2 receptors represent a link between canonical and non-canonical saliva secretion. Additionally, extracellular nucleotides released during periods of cellular stress and inflammation act as a tissue alarmin to coordinate immunological and tissue repair responses through P2 receptor activation. Accordingly, P2 receptors have gained widespread clinical interest with agonists and antagonists either currently undergoing clinical trials or already approved for human use. Here, we review the contributions of P2 receptors to salivary gland function and describe their role in salivary gland dysfunction. We further consider their potential as therapeutic targets to promote physiological saliva flow, prevent salivary gland inflammation and enhance tissue regeneration.
Salivary gland dysfunction and the associated hyposalivation are serious clinical problems that impact millions of people (Atkinson et al., 2005; Qin et al., 2015; Siddiqui and Movsas, 2017). Saliva plays a crucial role in maintaining oral homeostasis by aiding in taste perception and digestion, protecting and lubricating oral tissues, maintaining the integrity of tooth enamel and sustaining the oral microbiome (Dawes et al., 2015). In addition to its physiological roles, saliva contains a plethora of biomarkers and is easy to access allowing clinicians to utilize saliva as a non-invasive diagnostic material to monitor patient health (Chojnowska et al., 2018). Human saliva is increasingly being used to perform screening and risk assessment for systemic diseases, such as HIV, cancer, infections and cardiovascular disorders, demonstrating saliva’s extensive clinical potential (Nunes et al., 2015). Adequate saliva production is essential for maintaining quality of life and salivary gland dysfunction leads to dry mouth, oral bacterial and yeast infections, dental caries and speech problems (Chambers et al., 2004; Meijer et al., 2009).
Hyposalivation and xerostomia (i.e., dry mouth) can present in an iatrogenic manner as side effects of over 400 medications, including antidepressants, antipsychotics, opioids, antihistamines, and others (Furness et al., 2011). Although often transient and reversible, iatrogenic xerostomia contributes to patient non-adherence to medication regimens leaving underlying pathologies untreated. Two common pathophysiological causes of salivary gland dysfunction in humans are Sjögren’s syndrome (SS), an autoimmune disease characterized by xerostomia, autoantibody production and chronic lymphocytic infiltration of the salivary glands (i.e., sialadenitis), and radiotherapy-induced dysfunction where salivary glands sustain collateral damage following γ-radiation to treat head and neck tumors (Pinna et al., 2015; Mariette and Criswell, 2018). In both cases, damage to the salivary parenchyma and the failure to repair saliva-producing salivary acinar epithelium contribute to glandular dysfunction. Current therapies for salivary gland dysfunction are primarily focused on symptom management using muscarinic receptor agonists (i.e., pilocarpine or cevimeline) to stimulate saliva flow from residual salivary epithelium or through the topical use of artificial saliva (Ramos-Casals et al., 2010). While these treatments can provide some relief to patients, they are relatively ineffective because of their transient nature and failure to address the underlying inflammatory and degenerative processes that initiate and sustain glandular tissue damage. Therefore, a better understanding of the pathophysiology of salivary gland dysfunction is crucial to developing novel therapeutic approaches for this serious medical problem.
Purinergic receptors for extracellular nucleosides (i.e., adenosine) or nucleotides (i.e., ATP, ADP, UTP, UDP, and UDP-glucose) mediate numerous physiological processes, including platelet aggregation, neurotransmission, bone remodeling, and inflammatory, and immune responses (Dorsam and Kunapuli, 2004; Orriss et al., 2010; Idzko et al., 2014; Mutafova-Yambolieva and Durnin, 2014; Verkhratsky and Burnstock, 2014). In exocrine tissues, such as salivary gland, lacrimal gland and pancreas, purinergic receptor-mediated ion fluxes and cross-talk with muscarinic receptor signaling have been suggested to modulate secretory function (Novak et al., 2010; Burnstock and Novak, 2012; Hodges and Dartt, 2016). Whereas intracellular nucleotides are well-known for their role in metabolism and enzyme function, it wasn’t until the 1970s that plasma membrane receptors were postulated to respond to extracellular nucleotides, including ATP and ADP, and were suggested to be responsible for non-cholinergic, non-adrenergic neurotransmission (Burnstock et al., 1972; Burnstock, 1976). Under normal conditions, extracellular nucleotides are present at minute concentrations due to the presence of ectonucleotidases (Robson et al., 2006; Zimmermann et al., 2012). However, under pathological conditions nucleotides can accumulate in the extracellular space at abnormally high concentrations, whereupon they activate local purinergic receptors in an autocrine or paracrine manner (Deaglio and Robson, 2011). The purinergic receptor family is subclassified into P1 adenosine receptors (i.e., A1, A2A, A2B, and A3) (Piirainen et al., 2011) or P2 nucleotide receptors. The P2 receptor family is further classified into metabotropic P2Y receptors (i.e., P2Y1,2,4,6,11–14) and ionotropic P2X receptors (i.e., P2X1-7) (Abbracchio et al., 2006; Habermacher et al., 2016).
Pharmacological agonists and antagonists targeting purinergic receptors have gained widespread clinical interest and undergone clinical trials (Burnstock, 2017). P2X7 receptor (P2X7R) antagonists have been previously investigated in phase 2 clinical trials for treatment of inflammatory and autoimmune diseases, including chronic obstructive pulmonary disorder, rheumatoid arthritis and Crohn’s disease (Arulkumaran et al., 2011; Keystone et al., 2012). Recent advances in the development of neuro-permeable P2X7R antagonists have stimulated interest in the use of these compounds to treat neuroinflammatory and neuropsychiatric disorders (Chrovian et al., 2014; Burnstock and Knight, 2018; Bhattacharya and Ceusters, 2019). The P2X3 receptor (P2X3R) contributes to hypersensitivity of lung afferent sensory fibers that mediate cough initiation and phase 2 clinical trials have demonstrated that the P2X3R antagonist gefapixant (AF-219) reduces refractory chronic cough in afflicted patients by 75% (Weigand et al., 2012; Abdulqawi et al., 2015). Follow-up phase 3 clinical trials are currently underway to validate the use of gefapixant for treatment of refractory chronic cough (Muccino and Green, 2019).
Due to its ability to stimulate water transport across epithelial cell membranes following activation of calcium-dependent chloride channels, the P2Y2 receptor (P2Y2R) agonist diquafosol has undergone human clinical trials for the treatment of dry eye disease (DED) and is currently approved for human use in Japan and South Korea under the trade name Diquas (Tauber et al., 2004; Takamura et al., 2012; Koh, 2015). A similar P2Y2R agonist, denufosol, improved lung function relative to placebo in cystic fibrosis patients during phase 2 clinical trials, but failed to achieve its primary endpoints during phase 3 follow-up trials (Accurso et al., 2011). Notably, the FDA-approved anti-coagulant Plavix (clopidogrel), a P2Y12 receptor (P2Y12R) antagonist, was the 2nd most prescribed drug in the world in 2010 and is currently on the World Health Organization’s List of Essential Medicines (Topol and Schork, 2011; Kishore et al., 2018). However, the therapeutic potential of targeting purinergic receptors has not been well-investigated in the context of human salivary dysfunction. In the salivary glands, several purinergic receptors are expressed and upregulated under pathological conditions, including SS (Schrader et al., 2005; Baldini et al., 2013), where their activation mediates inflammatory and immune responses (Baker et al., 2008; Khalafalla M.G. et al., 2017), as well as cell repair mechanisms (El-Sayed et al., 2014). In this review, we summarize the role of purinergic receptors in salivary gland function and highlight their potential as novel therapeutic targets to treat salivary gland dysfunction.
The importance of saliva, as noted above, is clearly exemplified in individuals suffering from salivary gland hypofunction (Chambers et al., 2004; Atkinson et al., 2005; Meijer et al., 2009). In humans, whole unstimulated saliva is formed from the combined secretions of three pairs of major salivary glands, the submandibular (∼65%), parotid (∼20%) and sublingual (∼7%), along with numerous minor glands spread throughout the oral cavity that produce the remainder of saliva (<10%) (Humphrey and Williamson, 2001; de Almeida Pdel et al., 2008; Proctor, 2016). Upon stimulation, the parotid glands contribute the majority of total salivary secretions (Humphrey and Williamson, 2001; de Almeida Pdel et al., 2008; Proctor, 2016). Three basic cell types comprise the salivary glands: acinar epithelial cells that secrete the majority of the water and electrolytes in saliva, ductal cells that modify the electrolyte concentrations in the primary fluid and myoepithelial cells that provide contractile support for acinar cells (Martinez, 1987; Melvin et al., 2005; de Almeida Pdel et al., 2008; Proctor, 2016). Salivary acinar cells are either serous or mucous, whereas ductal cells are classified as intercalated, striated or excretory and the distribution of these cell types is dependent on species and type of gland (Melvin et al., 2005; de Almeida Pdel et al., 2008; Proctor, 2016). Along with the formation and modification of saliva, acinar and ductal cells also secrete important proteins, e.g., amylase and mucins from acinar cells (Boehlke et al., 2015; Frenkel and Ribbeck, 2015), kallikrein from ductal cells (Wong et al., 1983) and growth factors from both cell types (Masahiko et al., 2008), that are integral in maintaining the health of the oral cavity (Proctor, 2016). As shown in Figure 1, saliva formation is initiated in acinar cells by agonist-induced increases in intracellular Ca2+ levels, [Ca2+]i, that induce the opening of apical Ca2+-dependent Cl– channels and basolateral Ca2+-dependent potassium channels, allowing Cl– efflux into the luminal compartment and K+ efflux into the basolateral compartment to maintain membrane potential. The negative electrochemical gradient generated by increased luminal Cl– levels is compensated by the influx of Na+ ions across tight junctions into the lumen leading to Na+Cl– accumulation followed by water movement through water channels, predominately aquaporin-5 (Ma et al., 1999), thus forming saliva in its primary isotonic form. As saliva flows through the salivary gland ducts, electrolyte modification occurs, where Na+ and Cl– ions are exchanged for K+ and HCO ions by ductal cells, creating saliva in its final hypotonic form (Martinez, 1987; Melvin et al., 2005; Lee et al., 2012; Ambudkar, 2014; Proctor, 2016). Several types of Ca2+ mobilizing receptors are expressed on acinar cells (i.e., muscarinic, α-adrenergic, substance P), however, stimulation of the Gq protein-coupled M3 muscarinic receptor (M3R) subtype by acetylcholine is accepted as the main receptor signaling pathway that promotes the increases in [Ca2+]i necessary to enhance fluid secretion. Protein secretion from acinar and ductal cells is predominately mediated by activation of the β-adrenergic receptor (β-AR) and subsequent increases in cAMP (Melvin et al., 2005; Proctor, 2016). In addition to the canonical M3R and β-AR pathways, a mechanism of non-cholinergic, non-adrenergic-mediated salivary flow exists (Ekström et al., 1988; Ekström, 1999; Melvin et al., 2005). Because purinergic receptor activation can result in an increase in [Ca2+]i in salivary gland cells, purinergic receptor-mediated saliva production may contribute to this non-canonical pathway (Turner et al., 1998b; Melvin et al., 2005; Aure et al., 2010; Bhattacharya et al., 2015).
Figure 1. Salivary gland acinar and ductal cells contribute to saliva formation. (A) Activation of type 3 muscarinic receptors (M3R) by acetylcholine (Ach) increases release of calcium from intracellular stores and subsequent opening of the apical Ca2+-dependent chloride channel transmembrane member 16A (TMEM16A; also known as anoctamin-1) and the basolateral Ca2+-dependent potassium channels MaxiK (Kcnma1) and IK1 (Kcnn4), allowing Cl– efflux into the luminal compartment and K+ efflux into the basolateral compartment to maintain membrane potential. The combined actions of the Na+/K+/2 Cl– cotransporter NKCC1, the Na+/H+ exchanger NHE1 and the Cl–/HCO anion exchanger AE2 maintain the pool of intracellular Cl– whereas the Na+/K+ ATPase generates the cellular Na+ and K+ gradients. Sodium influx down the negative electrochemical gradient into the luminal compartment is followed by water through aquaporin 5 (AQP5) water channels generating primary isotonic saliva. Modification of saliva by ductal cells involves exchanging sodium and chloride for potassium and bicarbonate through the combined actions of epithelial Na+ channels (ENaC), cystic fibrosis transmembrane conductance regulator (CFTR) channels, MaxiK channels and perhaps Cl–/HCO exchangers. The resulting hypotonic saliva is then secreted through ducts into the oral cavity. Functional P2X4, P2X7, P2Y1, and P2Y2 receptor expression has been demonstrated in both acinar and ductal cells where they may regulate secretory functions through nucleotide-induced Ca2+ signaling and modulation of membrane ion conductance. Available evidence suggests that P2X7 and P2Y2 receptors exist on both apical and basolateral membranes while P2X4 receptors are restricted to the basolateral compartment and P2Y1 receptor localization is undetermined. Importantly, P2 receptor expression in salivary gland tissue varies depending on species, isolation/culture methods and the presence of inflammatory stimuli, making definitive localization inexact. (B) Acinar (white) and ductal (yellow) cells outlined in a hematoxylin and eosin-stained section of a female C57BL/6 mouse submandibular gland.
In other exocrine tissues, purinergic receptor signaling has been shown to modulate secretory function of acinar and ductal cells through the induction of cellular ion fluxes and cross-talk with cholinergic signaling pathways (Burnstock and Novak, 2012; Hodges and Dartt, 2016). In the pancreas, acinar cells have little functional response to exogenously applied nucleotides (Novak et al., 2002), whereas ductal cells that secrete bicarbonate and isotonic fluid express numerous functional P2X and P2Y receptors (Hede et al., 1999). In response to stimulation by acetylcholine or secretin, pancreatic ductal cell secretion is mediated by the opening of luminal Cl– channels, including Ca2+-activated Cl– channels, as well as basolateral K+ channels to maintain driving force for ion transport (Novak, 2008). Therefore, the finding that extracellular ATP and UTP induce increases in [Ca2+]i and modulate whole cell Cl– and K+ conductance suggests a role for purinergic receptors in secretory regulation of pancreatic ductal cells (Christoffersen et al., 1998; Hede et al., 1999; Zsembery et al., 2000). Furthermore, studies have shown that cholinergic agonists induce ATP release from pancreatic acinar cells (Sorensen and Novak, 2001), as well as parotid and lacrimal gland cell preparations (Novak et al., 2010; Dartt and Hodges, 2011a), further supporting a role for purinergic signaling in the regulation of exocrine secretory function. In rat lacrimal gland acinar cells, extracellular nucleotide-induced protein secretion and [Ca2+]i increases were inhibited by the cholinergic antagonist atropine (Dartt and Hodges, 2011a) whereas in rat parotid acinar cells extracellular nucleotides attenuated acetylcholine-induced [Ca2+]i increases (Jorgensen et al., 1995; Fukushi, 1999). Although the nature of purinergic and cholinergic signaling interaction differs between exocrine tissues, these studies highlight the likely regulatory role of purinergic receptors in exocrine secretory function.
Ten years prior to the initial cloning and identification of P2 receptors, Gallacher (1982) presented the first evidence of P2 receptor activation in salivary glands. His studies demonstrated that ATP evoked a marked increase in membrane conductance, K+ efflux and amylase secretion in the mouse parotid gland, events similar to cholinergic- and adrenergic-mediated saliva secretion (Melvin et al., 2005; Proctor, 2016). McMillian et al. (1987) showed that high extracellular ATP concentrations increased [Ca2+]i in rat parotid acinar cells, the signaling response that promotes saliva production (Melvin et al., 2005). Additional studies by the same group and others determined that the large ATP-induced rise in [Ca2+]i was due to the influx of extracellular Ca2+ through a non-selective cation channel activated by the fully ionized form of ATP (i.e., ATP4–) (Soltoff et al., 1990; Dehaye, 1993; McMillian et al., 1993). The order of agonist potency for channel activation in these studies was determined to be BzATP > ATP > ATPγS = 2MeSATP; thus, the receptor was classified as P2Z, now known as the P2X7 receptor (P2X7R) (Soltoff et al., 1990; Dehaye, 1993; McMillian et al., 1993). Thus, a physiological role for ATP in the Ca2+-dependent formation of saliva was proposed, particularly since ATP was known to be released as a co-transmitter from activated sympathetic and parasympathetic nerve fibers (von Kugelgen et al., 1994; Novak, 2003). During the ensuing years, especially following the cloning, expression and identification of cDNAs for a variety of P2 receptors in the early 1990s (Lustig et al., 1993; Webb et al., 1993; Nguyen et al., 1995; Surprenant et al., 1996), several groups confirmed the expression of P2X7R in salivary gland cells and also identified and functionally characterized the ionotropic P2X4 receptor (P2X4R) and metabotropic P2Y receptors, P2Y1R and P2Y2R, in these cells (Turner et al., 1999).
The P2X7R is a 595 amino acid protein that includes two transmembrane domains, intracellular carboxy and amino termini and a bulky hydrophilic extracellular loop with a cysteine rich region that forms disulfide bridges (McCarthy et al., 2019). It shares 40–50% amino acid homology with the other P2X receptors, but is structurally distinct in that its C-terminal tail extends for an additional 100–200 amino acids (North, 2002; Adinolfi et al., 2005; Sluyter, 2017). The P2X7R is activated by high extracellular ATP (eATP) concentrations (>100 μM) with brief stimulation (10–30 s) causing the depolarization of the plasma membrane due to the opening of a membrane cation channel that promotes the influx of Na+ and Ca2+ and the efflux of K+ (Weisman et al., 1984, 1989; Adinolfi et al., 2005). Sustained P2X7R activation induces the opening of a pore permeable to hydrophilic molecules up to 900 Da, and promotes production of reactive oxygen species (ROS), NLRP3 inflammasome-dependent IL-1β release, extensive plasma membrane blebbing and ultimately cell death (Weisman et al., 1984, 1989; Woods et al., 2012; Di Virgilio et al., 2017; Giuliani et al., 2017; Khalafalla M.G. et al., 2017). The P2X7R is widely expressed in diverse tissues, including hematopoietic cells (Feng et al., 2016), neurons (Miras-Portugal et al., 2017), glia (Stokes et al., 2015; Kaczmarek-Hajek et al., 2018), bone (Agrawal and Gartland, 2015), muscle (Fabbrizio et al., 2019), endothelium (Green et al., 2018), epithelium (Woods et al., 2012), and immune cells (Ferrari et al., 1997). In the exocrine pancreas, P2X7Rs have been shown to be primarily expressed in pancreatic ductal cells where they may contribute to secretory regulation through induction of cation fluxes and interaction with cholinergic signaling (Novak et al., 2010; Burnstock and Novak, 2012). Similarly, in lacrimal glands P2X7Rs mediate [Ca2+]i increases, ERK1/2 activation, protein secretion and modulate both cholinergic and adrenergic receptor signaling pathways (Hodges et al., 2009; Dartt and Hodges, 2011a, b). After its initial characterization in rat parotid acinar cells (McMillian et al., 1987; Gibbons et al., 2001), P2X7R expression and function were reported to promote increases in [Ca2+]i in rat submandibular acinar cells (Lee et al., 1997; Alzola et al., 2001), murine parotid (Li et al., 2003; Reyes et al., 2008; Bhattacharya et al., 2012) and submandibular acinar cells (Nakamoto et al., 2009) and human parotid acinar cells (Brown et al., 2004).
In addition to numerous studies defining its role in mediating inflammatory and immune responses in disease models (Savio et al., 2018; Cao et al., 2019; Zeng et al., 2019), including those pertaining to salivary glands (Woods et al., 2012; Khalafalla M.G. et al., 2017), there is evidence that P2X7Rs regulate salivary secretory function (Nakamoto et al., 2009; Novak et al., 2010; Pochet et al., 2013). Along with its ability to increase [Ca2+]i due to calcium influx, P2X7R activation has been shown to inhibit mobilization of intracellular Ca2+ induced by muscarinic or substance P receptor agonists in rat submandibular acinar cells (Hurley et al., 1993; Metioui et al., 1996) and cholinergic mobilization of [Ca2+]i was significantly increased in parotid acinar cells prepared from P2X7R-null (P2X7R–/–) mice (Novak et al., 2010). The mechanism of this inhibition is still unclear, but it does not appear to be due to interference with binding of the autonomic agonists to their receptors (Hurley et al., 1993). This observation was corroborated in an ex vivo murine submandibular gland (SMG) preparation, where co-stimulation with ATP and muscarinic receptor agonists had an inhibitory effect on the gland’s saliva production (Nakamoto et al., 2009). Further, in glands prepared from P2X7R–/– mice the inhibitory effect of ATP on carbachol-induced saliva secretion was abolished, suggesting an inhibitory role for P2X7Rs in saliva production (Nakamoto et al., 2009). However, in this same study ATP or BzATP alone evoked fluid secretion in a time-dependent manner that was greatly reduced in glands from P2X7R–/– mice, whereas carbachol alone induced similar saliva secretion in wild type and P2X7R–/– glands. Similarly, another study found no significant difference in cholinergic-mediated whole saliva secretion in P2X7R–/– mice compared to wild type (Pochet et al., 2007). In contrast, Novak et al. (2010) found that cholinergic-mediated whole saliva secretion was significantly decreased in P2X7R–/– mice, as compared to wild type mice, and this was particularly evident in male mice. While the reasons for the disparities among these studies are unclear, they may be due to differences in the type of saliva collected (i.e., whole saliva vs. saliva from specific glands), methods of induction of saliva secretion, tissue specificity, sex, or mouse strain.
The P2X7R is also expressed in rat (Lee et al., 1997; Alzola et al., 1998) and mouse salivary ductal cells (Li et al., 2003; Pochet et al., 2007; Nakamoto et al., 2009), suggesting participation in the modification of the electrolyte content of saliva. Studies indicate no difference in [Na+] or [Cl–] in muscarinic agonist-induced whole saliva secreted in wild type compared to P2X7R–/– mice, however the [K+] was elevated in P2X7R–/– mouse whole saliva (Pochet et al., 2007). Since the majority of the K+ in saliva originates from ductal cells, it has been hypothesized that ATP released from acinar cells during exocytosis stimulates ductal P2X7Rs that regulate the activity of K+ channels located on the apical membrane (Liu et al., 1999; Bhattacharya et al., 2015). In addition to K+ modification, activation of P2X7Rs in ductal cells increases phospholipase A2-dependent secretion of arachidonic acid, a precursor of prostaglandin E2 (PGE2), and kallikrein (Alzola et al., 1998) into saliva (Pantano et al., 2019). Interestingly, cell lines of salivary origin exhibit low expression and function of P2X7R, which are enhanced following DNA demethylation (Shin et al., 2015).
Another P2X ionotropic receptor expressed in salivary acinar and ductal cells is the P2X4R (Turner et al., 1998b). Unlike the P2X7R’s requirement for activation by high eATP concentrations, P2X4Rs have nanomolar affinity for ATP (North, 2016; Suurvali et al., 2017) and were initially found to regulate the biphasic response to ATP in rat parotid gland cells (McMillian et al., 1993). The P2X4R is widely expressed in a variety of cell types, e.g., neurons and microglia (Ho et al., 2014), epithelium (Casas-Pruneda et al., 2009), and endothelium (Lv et al., 2015), and P2X4R expression in microglia is notable for the key role it plays in mediating neuropathic pain (Inoue, 2019). Although RT-PCR analysis has identified P2X4R expression in pancreatic acinar and ductal cells (Luo et al., 1999; Novak et al., 2002) and lacrimal gland acinar cells (Hodges et al., 2011; Kamada et al., 2012), its functional role in exocrine tissues remains largely unexplored. Physical interactions between P2X4Rs and P2X7Rs have been demonstrated, although the nature of this interaction remains controversial (Kopp et al., 2019). Some studies suggest that P2X4R and P2X7R subunits form heteromeric channels (Guo et al., 2007; Schneider et al., 2017), while others conclude that P2X4 and P2X7 receptors interact in their respective homotrimeric form (Nicke, 2008; Boumechache et al., 2009; Antonio et al., 2011). Furthermore, P2X4R expression has been localized to lysosomal membranes, whereas P2X7Rs primarily reside at the plasma membrane (Guo et al., 2007; Huang et al., 2014). Nevertheless, studies have also demonstrated functional evidence for P2X4R/P2X7R interactions (Guo et al., 2007; Kawano et al., 2012; Perez-Flores et al., 2015). In salivary epithelium, P2X4Rs modulate P2X7R-mediated ion flow and ethidium bromide dye uptake (Casas-Pruneda et al., 2009), suggesting a functional interaction that regulates physiological processes, including plasma membrane ion channel function and pore formation. Importantly, the interaction between these two purinergic receptors results in a decreased sensitivity to ATP, as compared to the P2X4R or P2X7R alone, suggesting the formation of heteromeric channels with novel functional and pharmacological properties (Casas-Pruneda et al., 2009).
While the contribution of P2X4R activation to physiological saliva production has not been explored, ex vivo murine SMG preparations from P2X7R–/– mice exhibit weak ATP-induced saliva secretion that could be attributed to P2X4R activation (Nakamoto et al., 2009). As seen previously with muscarinic or adrenergic receptor activation (Baldys-Waligorska et al., 1987; Yoshimura and Hiramatsu, 1998; Tanimura et al., 1999; Bruce et al., 2002), co-stimulation of β-adrenergic receptors and P2X7Rs or P2X4Rs enhanced the influx of Ca2+ in mouse parotid acinar cells, as compared to activation of either receptor alone (Bhattacharya et al., 2015). In contrast, studies using human parotid acinar cells found this co-stimulatory effect only between the P2X4R and β-adrenergic receptor (Brown et al., 2004). Taken together, the expression of both P2X7Rs and P2X4Rs in salivary glands supports the idea that they are involved in the interplay between canonical and non-canonical signaling pathways that regulate saliva flow and composition and their involvement is likely dependent on their tissue localization (i.e., basal vs. apical) in polarized acinar and/or ductal epithelial cells (Bhattacharya et al., 2012, 2015).
The metabotropic P2Y1 receptor (P2Y1R), formerly known as the P2T receptor, has been identified and cloned (Webb et al., 1993; Baranska et al., 2017) and has features typical of G protein-coupled receptors, i.e., an extracellular N-terminus and an intracellular C-terminus, seven hydrophobic transmembrane regions, three extracellular loops and three intracellular loops (von Kugelgen and Hoffmann, 2016). The P2Y1R has a distinctive rank order of agonist potencies (i.e., 2-methylthio-ADP > ADP > ATP) and its activation induces canonical Gαq signaling leading to phospholipase C activation and generation of the second messengers inositol 1, 4, 5-trisphosphate (IP3) and diacylglycerol that increase [Ca2+]i and protein kinase C (PKC) activity, respectively (von Kugelgen and Wetter, 2000; Abbracchio et al., 2006; Baranska et al., 2017; von Kugelgen, 2019). Additionally, P2Y1R activation stimulates metalloprotease-dependent transactivation of the epidermal growth factor receptor (EGFR) (Buvinic et al., 2007) and mitogen-activated protein kinase (MAPK) activity through activation of phosphatidylinositol 3-kinase, Src kinase and PKC (Sellers et al., 2001; Baranska et al., 2017). The P2Y1R is widely distributed in mammalian tissues and is involved in many physiological and biochemical responses, such as platelet aggregation (Fabre et al., 1999), pain sensation (Barragan-Iglesias et al., 2015), vasodilation (Zerr et al., 2011), bone remodeling (Orriss et al., 2011), and osmotic volume regulation (Grosche et al., 2013). In exocrine tissues, immunofluorescence and RT-PCR analyses provide evidence of P2Y1R expression in pancreatic ductal cells where P2Y1R agonists also induce [Ca2+]i increases (Luo et al., 1999; Coutinho-Silva et al., 2001). However, the role of P2Y1Rs in exocrine pancreas function has been unexplored. Likewise, P2Y1R expression has been demonstrated in lacrimal acinar cells and myoepithelial cells by RT-PCR, immunofluorescence and measurement of P2Y1R agonist-induced [Ca2+]i increases, but further functional analyses are lacking (Ohtomo et al., 2011). Interestingly, the P2Y1R has been used as a surrogate cell-surface marker for the nuclear protein pancreatic duodenal homeobox 1 (PDX1) to isolate progenitor-like ductal cells from human pancreatic tissues, although no functional role for P2Y1Rs was investigated (Qadir et al., 2018). In contrast, studies on endocrine pancreas function suggest a role for P2Y1Rs in mediating insulin secretion from β cells (Leon et al., 2005; Petit et al., 2009). The P2Y1R is also involved in tissue development, as was first described in chick embryos (Meyer et al., 1999; Meyer et al., 2001) and more recently in the developing brain (Huang et al., 2019). In the developing rat salivary gland, it was observed that acinar cells prepared from immature glands of 1 day-old pups had a robust [Ca2+]i response to P2Y1R agonists, whereas acini prepared from adult rat salivary glands had no response (Park et al., 1997). Interestingly, P2Y1R mRNA expression remained the same at all ages in rats, suggesting that the loss of the P2Y1R-mediated [Ca2+]i response may be due to age-dependent alterations in intracellular G protein coupling (Park et al., 1997). A subsequent study using rat SMG acinar and ductal cell preparations confirmed the age-dependent reduction in P2Y1R-mediated increases in [Ca2+]i and, similarly, found unchanged P2Y1R expression levels at all ages (Baker et al., 2006). This study further demonstrated that P2Y1R-mediated activation of the MAPKs, extracellular signal-regulated kinases 1 and 2 (ERK1/2), was consistent in rats of all ages, indicating that ERK1/2 activation is independent of P2Y1R-mediated changes in [Ca2+]i. Western analysis and assays of GTPγ35S binding to G proteins determined that the age-dependent decrease in P2Y1R activity in rat SMG cells was due to both decreased expression of the 52 kDa Gα14 protein and differential coupling of P2Y1Rs to Gαq/11 with age (Baker et al., 2006). These studies suggest that P2Y1Rs use diverse mechanisms for coupling to multiple G proteins that regulate a variety of physiological responses during development. To date, these findings have not been confirmed in salivary glands of mice, but with the availability of P2Y1R-null mice, it would be of interest to assess the role of this receptor in salivary gland morphology and function during development.
The P2Y2R (formerly known as the P2U receptor), equipotently activated by ATP or UTP (EC50 ∼ 2 μM), is the only other known Gαq-coupled purinergic receptor identified in salivary glands (Turner et al., 1998b, 1999) and has been cloned and functionally characterized in mice and humans (Erb et al., 1993; Lustig et al., 1993; Parr et al., 1994). Similar to the P2Y1R, P2Y2R activation induces canonical Gαq signaling leading to increases in [Ca2+]i and PKC activation, and the P2Y2R is expressed in numerous cell and tissue types, e.g., neurons (Peterson et al., 2013), epithelium (Shishikura et al., 2016; Wu et al., 2017), endothelium (Seye et al., 2003) and immune cells (Idzko et al., 2014; Woods et al., 2018), where it modulates a variety of cellular responses, including neurotransmission (Zhang and Li, 2019), proliferation (Shen et al., 2004), cell migration (Bagchi et al., 2005), cytoskeletal rearrangements (Liao et al., 2007), and ion fluxes (Murakami et al., 2004). The diversity of cellular responses mediated by P2Y2Rs is due, in part, to unique structural features enabling activation of multiple signal transduction pathways. In addition to canonical Gαq signaling (Parr et al., 1994), the P2Y2R contains a motif typically found in extracellular matrix proteins, i.e., an Arg-Gly-Asp (RGD)-sequence, in its first extracellular loop that binds to αvβ3/β5 integrins to activate Go and G12 proteins, enhance MAPK (ERK1/2) phosphorylation and regulate ATP- and UTP-induced cell chemokinesis and chemotaxis (Erb et al., 2001; Bagchi et al., 2005; Wang et al., 2005; Liao et al., 2007). Within the intracellular C-terminus of the P2Y2R, Src-homology-3 (SH3) binding domains (PXXP) enable the P2Y2R to bind and activate the tyrosine kinase Src, enabling nucleotide-induced, Src-dependent transactivation of growth factor receptors and downstream MAPKs that regulate cell proliferation and migration (Liu et al., 2004; Seye et al., 2004). Additionally, interaction of the P2Y2R C-terminus with the actin-binding protein filamin-A contributes to cell migration and Rho GTPase-mediated cytokine release (Yu et al., 2008; Seye et al., 2012). The P2Y2R also mediates the proprotein convertase furin-dependent activation of metalloproteases, i.e., a disintegrin and metalloproteinase 10 and 17 (ADAM10/17), to cleave transmembrane proteins (Camden et al., 2005), thereby releasing EGFR/ERB ligands that promote Src-independent EGFR activation (Ratchford et al., 2010). These diverse P2Y2R signaling pathways have been implicated in a number of pathologies, including Alzheimer’s disease (Ajit et al., 2014), cardiovascular disease (Chen et al., 2017), cancer (Hu et al., 2019), SS (Woods et al., 2018), and hantavirus cardiopulmonary syndrome (Bondu et al., 2018), as well as processes such as wound healing (Jin et al., 2014) and tissue regeneration (El-Sayed et al., 2014).
In exocrine tissues such as the lacrimal gland, RT-PCR and immunohistochemical analyses have identified P2Y2R expression in acinar and ductal cells (Kamada et al., 2012; Tanioka et al., 2014). While no functional response to the P2Y2R agonist UTP was observed in lacrimal acinar cells (Kamada et al., 2012), cultured lacrimal gland myoepithelial cells do exhibit increased [Ca2+]i in response to extracellular UTP suggesting the presence of P2Y2 or P2Y4 receptors (Ohtomo et al., 2011). In the exocrine pancreas, RT-PCR and immunohistochemical analyses indicate that P2Y2Rs are expressed in both pancreatic acini (Novak et al., 2002) and ductal cells (Hede et al., 1999; Luo et al., 1999; Coutinho-Silva et al., 2001), although very few pancreatic acinar cells show functional responses to extracellular ATP or UTP (Novak et al., 2002). In pancreatic ductal cells, P2Y2R-mediated increases in [Ca2+]i altered whole-cell K+ conductance (Hede et al., 1999), likely through modulation of Ca2+-activated K+ channels (Hede et al., 2005), suggesting a role in the regulation of ductal fluid flow and Cl–/HCO levels. Studies with pancreatic ductal cell lines have also shown that the P2Y2R agonists ATP and UTP increase membrane Cl– conductance through the opening of Ca2+-dependent Cl– channels (Galietta et al., 1994; Chan et al., 1996; Zsembery et al., 2000). The ability of P2Y2Rs to induce chloride secretion and subsequent fluid flow across epithelial cell membranes led to investigation of the P2Y2R as a therapeutic target for cystic fibrosis (Weisman et al., 1998; Kellerman et al., 2002; Lazarowski and Boucher, 2009). By stimulating Ca2+-dependent Cl– secretion, topical application of the selective P2Y2R agonist diquafosol has been shown to promote tear secretion and is currently being used to treat DED (Jacobson and Civan, 2016).
In 1991, the P2Y2R was first identified in a cell line of salivary gland origin, human salivary gland (HSG) cells, where it was shown to mediate UTP-induced IP3 production and increases in [Ca2+]i and plasma membrane K+ transport (Yu and Turner, 1991). A subsequent study determined that exposure of HSG cells to UTP potentiated a regulatory volume decrease (RVD) after hypotonic stress, suggesting that activation of P2Y2Rs provides the driving force for net Cl– efflux that enables the cells to rapidly restore their volume (Kim et al., 1996), a response that occurs during salivary secretion (Melvin et al., 2005). In 1998, it was shown that simian virus 40-transformed salivary cell lines from rat SMG and parotid glands (Quissell et al., 1998), unlike HSG cells, were suitable for Ussing chamber studies due to their ability to form polarized cell monolayers (Turner et al., 1998a). Using the polarized rat parotid cell line Par-C10 in a Ussing chamber, transepithelial resistance measurements determined that functional P2Y2R expression was localized to the apical membrane, consistent with its localization in other epithelium (Hwang et al., 1996; Chan et al., 1997; Yang et al., 2009), and its activation by UTP increased an anion ()-dependent change in short-circuit current (Isc) (Chan et al., 1996, 1997; Clarke et al., 1999). Taken together, these results suggest that expression of P2Y2Rs on salivary gland epithelium may contribute to saliva secretion; however, subsequent studies with freshly isolated salivary acinar cells showed little evidence of P2Y2R expression or activity under steady-state conditions (Turner et al., 1997; Ahn et al., 2000; Schrader et al., 2005). Moreover, carbachol-stimulated whole saliva secretion in P2Y2R-null mice (P2Y2R–/–) is unchanged compared to wild type mice (Woods et al., 2018), suggesting that P2Y2Rs do not contribute to overall fluid secretion. Earlier studies demonstrated UTP-induced Cl– fluxes in rat salivary duct cells (Lee et al., 1997; Zeng et al., 1997) with one study suggesting that P2Y2R expression on striated ducts regulates CFTR activity (Ishibashi et al., 2008), thereby possibly modifying the ionic content of saliva.
The contribution of P2 receptors to physiological salivary gland function is predicated on the presence of endogenous agonists (i.e., extracellular nucleotides) in sufficient concentrations to activate their cognate receptors, as is the case when ATP is co-released with neurotransmitters from sympathetic and parasympathetic nerves (von Kugelgen et al., 1994; Novak, 2003). In exocrine tissues such as the pancreas and lacrimal glands, ATP is released in response to stimulation by physiological agonists such as acetylcholine and cholecystokinin-8 (Sorensen and Novak, 2001; Yegutkin et al., 2006; Novak et al., 2010; Dartt and Hodges, 2011a). Additionally, measurable amounts of ATP are present in rat saliva induced by intraperitoneal pilocarpine administration (Ishibashi et al., 2008). However, the concentration of extracellular nucleotides is tightly regulated under physiological conditions and maintained in the low μM range by ectonucleotidases (Pellegatti et al., 2008; Di Virgilio et al., 2018), such as the nucleoside triphosphate diphosphohydrolase ENTPD1 (CD39) and related family members (Deaglio and Robson, 2011; Zimmermann et al., 2012). Using conventional luciferin/luciferase luminescence measurements or cell-based biosensors, the concentration of extracellular ATP released from pancreatic acinar or β cells has been measured at ∼10–25 μM (Hazama et al., 1998; Sorensen and Novak, 2001), although in vivo measurement of absolute extracellular nucleotide concentrations is an active area of research (De Marchi et al., 2020). However, during periods of inflammation or other cellular stresses, such as hypoxia in the tumor microenvironment, extracellular ATP levels have been shown to exceed 100 μM and are likely much higher in the context of the confined pericellular space (Pellegatti et al., 2008; Joo et al., 2014; Di Virgilio et al., 2018; De Marchi et al., 2019). Immune and apoptotic cells release ATP through connexin and pannexin hemichannels during inflammatory responses and uncontrolled release of intracellular ATP pools can also occur during cell necrosis (Eltzschig et al., 2006; Chekeni et al., 2010). Mounting evidence also suggests that connexin 43-mediated ATP release from γ-irradiated cells causes the radiation-induced bystander effect where adjacent, non-irradiated cells exhibit physiological responses mediated by P2 receptors (Tsukimoto et al., 2010; Ohshima et al., 2012; Tsukimoto, 2015; Kojima et al., 2017). Interestingly, the ionotropic P2X7 receptor also has been shown to mediate ATP release (Suadicani et al., 2006; Ohshima et al., 2010), likely through its sustained activation that leads to membrane depolarization and pore formation (Dahlquist et al., 1974; Weisman et al., 1984; Buisman et al., 1988), and P2X7R blockade has been shown to attenuate ionizing radiation (IR)-induced ATP release from salivary acinar cells (Gilman et al., 2019). Recognizing that salivary gland inflammation and radiation exposure, two common sources of salivary gland dysfunction, promote the release of extracellular nucleotides and subsequent P2 receptor activation, defining the role of P2 receptors in salivary gland pathophysiology has been an area of intense interest.
In addition to its role as an ion channel, activation of the P2X7R initiates signaling cascades that produce pro-inflammatory cytokines (e.g., IL-1β, IL-18, IL-6, IL-8, and TNF-α) to enable antigen-presenting cells to initiate innate immune responses (Ferrari et al., 1997; Solini et al., 1999; Mehta et al., 2001; Lister et al., 2007; Shieh et al., 2014). In salivary epithelium, our group has shown that P2X7R activation with ATP or BzATP triggers apoptotic and pro-inflammatory cell responses, including increases in caspase-1 and caspase-3 activity and immune cell infiltration into wild type, but not P2X7R–/–, mouse SMGs (Woods et al., 2012). Also, P2X7R activation in salivary epithelium was found to induce the assembly of the NLRP3 inflammasome multiprotein complex and the subsequent release of IL-1β, a response that was dependent on K+ efflux, production of ROS and functional heat shock protein 90 (Khalafalla M.G. et al., 2017). P2X7R activation also has been shown to mediate the protease-dependent release of α-fodrin (Woods et al., 2012), a putative autoantigen associated with SS (Miyazaki et al., 2005), through a mechanism that requires caspase-3 and calpain enzymatic activities (Hwang et al., 2009b). P2X7R activation induces membrane blebbing, an early indicator of cell apoptosis, in salivary epithelial cells isolated from wild type, but not P2X7R–/–, mice (Woods et al., 2012). The mechanism of P2X7R-mediated membrane blebbing was shown to require sustained elevation of [Ca2+]i, activation of the ROCK I signaling pathway and phosphorylation of myosin light chain, but does not involve caspase-3 activation (Hwang et al., 2009a).
There are increasing lines of evidence that P2X7R-induced pro-inflammatory responses are modulated by the P2X4R as well. In immune cells, P2X4Rs have been shown to modulate P2X7R-induced IL-1β release and dye uptake through interaction with the P2X7R C-terminus and P2X4R antagonism abolished P2X7R-induced Ca2+ influx and IL-1β and IL-18 release (Sakaki et al., 2013). In gingival epithelial cells, P2X7Rs, P2X4Rs and pannexin-1 hemichannels were all required for ATP-induced ROS production, NLRP3 inflammasome activation and IL-1β release (Hung et al., 2013). These cellular mechanisms may also be important in IL-1β release from salivary epithelium, where P2X4Rs have been shown to modulate P2X7R-mediated ion flow and pore formation (Casas-Pruneda et al., 2009).
In rodent salivary glands, P2Y2R expression is negligible under physiological conditions. Interestingly, freshly dispersed salivary epithelial cells significantly upregulated P2Y2R expression and activity as a function of time when placed in culture (Turner et al., 1997; El-Sayed et al., 2014), consistent with a possible role for P2Y2R in the cellular response to stress. P2Y2R upregulation also occurs in the in vivo ductal ligation model of salivary gland inflammation and fibrosis (Ahn et al., 2000) and has been similarly seen in other in vivo models of stress and inflammation, i.e., intestinal inflammation (Grbic et al., 2008), rat vascular neointima formation after balloon angioplasty (Seye et al., 1997), collared rabbit carotid arteries (Seye et al., 2002), glomerulonephritis (Rennert et al., 2018), myocardium of rats with congestive heart failure (Granado et al., 2015) and mouse models of the autoimmune disease SS (Schrader et al., 2005; Woods et al., 2018). IL-1β has been previously shown to induce P2Y2R upregulation (Kong et al., 2009; Peterson et al., 2013), likely through binding of NF-κB p65 to the P2Y2R promoter region that has been demonstrated to mediate inflammation-induced P2Y2R upregulation in human intestinal epithelial cells (Degagne et al., 2009). Taken together, these studies suggest that ATP released from stressed cells during inflammation activates P2X7Rs to induce the release of IL-1β and other cytokines. Subsequent activation of IL-1 receptors by IL-1β in surrounding cells induces P2Y2R upregulation and further downstream responses to ATP and UTP. In this way, the release of a single alarmin (e.g., ATP or UTP) in response to cellular stress can locally modulate a wide range of signaling pathways to fine-tune the tissue response to inflammatory stimuli.
In HSG cells, UTP-induced activation of P2Y2Rs has been shown to regulate localized immune responses and the binding of immune cells through the upregulation of the cell adhesion molecule VCAM-1 via an EGFR-dependent mechanism (Baker et al., 2008). Furthermore, P2Y2R activation has been shown to stimulate the production and secretion of pro-inflammatory lymphotoxin-α (LT-α), a member of the tumor necrosis factor family of cytokines that is required for the development of lymphoid tissues and mediates interactions between immune cells (Shen et al., 2010, 2013), suggesting multiple mechanisms whereby P2Y2Rs regulate localized immune responses relevant to salivary gland inflammation (Seye et al., 2012; Qian et al., 2016; Woods et al., 2018).
A number of autoimmune inflammatory diseases are reported to impact the function of salivary glands, including rheumatoid arthritis (Nagler et al., 2003; Helenius et al., 2005; Zalewska et al., 2011), systemic lupus erythematosus (SLE) (Leite et al., 2015) and diabetes mellitus (Moore et al., 2001). One of the major causes of salivary gland dysfunction is chronic inflammation associated with the autoimmune disease SS, the 2nd most common autoimmune rheumatic disease in the U.S., in which unresolved inflammation of the salivary and lacrimal glands contributes to tissue degeneration and subsequent loss of function (Helmick et al., 2008; Vivino, 2017). Clinical classification criteria for primary SS (pSS) in the absence of other autoimmune diseases include the presence in blood serum of anti-Ro/SSA and anti-La/SSB autoantibodies to their intracellular antigens, increased corneal staining using fluorescein dye (ocular staining score ≥ 5), decreased tear (Schirmer’s test ≤ 1 mm/min) and saliva (≤ 0.1 ml/min) flow rates and the presence of focal lymphocytic sialadenitis (focus score ≥ 1 foci/4 mm2) in minor salivary gland biopsies (Shiboski et al., 2017). During SS pathogenesis, T and B cells (van Woerkom et al., 2005; Daridon et al., 2006), dendritic cells (Ozaki et al., 2010; Zhao et al., 2016), and macrophages (Manoussakis et al., 2007) accumulate in the salivary glands where, along with salivary gland epithelial cells, they produce numerous pro-inflammatory cytokines, including IFN-γ, B cell-activating factor, TNF-α, IL-1β, IL-6 and IL-18, which initiate pro-inflammatory immune responses that ultimately degenerate the salivary glands (Hulkkonen et al., 2001; Willeke et al., 2003; Daridon et al., 2007; Sakai et al., 2008; Nezos et al., 2015). Additionally, SS patients produce high levels of immunoglobulins and autoantibodies besides anti-Ro/SSA and anti-La/SSB (Nardi et al., 2006; Suresh et al., 2015), including anti-α-fodrin (Watanabe et al., 1999; Miyazaki et al., 2005), RF (rheumatoid factor) (Müller et al., 1989; Huo et al., 2010) and other autoantibodies (Ramos-Casals et al., 2006; Shen et al., 2014; Suresh et al., 2015) that have been previously reported to activate intrinsic and extrinsic apoptotic pathways in salivary gland cells (Sisto et al., 2006; Lisi et al., 2007). Furthermore, anti-muscarinic receptor-3 autoantibodies that inhibit saliva production and aquaporin translocation to the plasma membrane (Bacman et al., 1996; Dawson et al., 2006) have been identified in the blood serum of SS patients. Taken together, these data suggest that chronic auto-inflammatory responses along with autoantibody-induced reductions in saliva and tear production and increased salivary acinar cell apoptosis contribute to pSS pathogenesis that ultimately leads to salivary gland dysfunction and fibrosis as well as systemic pathologies (i.e., chronic fatigue, lymphoma development, and secondary autoimmune manifestations).
Previous studies have demonstrated that the expression of P2X7R, caspase-1, IL-1β, IL-18 and components of the NLRP3 inflammasome multiprotein complex are significantly increased in labial salivary gland biopsies from SS patients, which positively correlates with salivary gland focus score (# of mononuclear cell foci/4 mm2 tissue area) (Baldini et al., 2013, 2017). Furthermore, these studies found that when SS patients were stratified based on the presence of anti-Ro/SSA autoantibodies, the increased expression of P2X7R and NLRP3 inflammasome components was even more pronounced in seropositive cohorts compared to seronegative cohorts (Baldini et al., 2013, 2017). Subsequent immunofluorescence analysis indicated that P2X7R expression in SS salivary gland biopsies co-localized with the acinar epithelial cell marker aquaporin 5, rather than immune cell markers, suggesting that P2X7Rs on salivary gland epithelium contribute to SS pathogenesis through a process termed autoimmune epithelitis (Mitsias et al., 2006; Baldini et al., 2017). Additionally, this prospective study of 147 SS patients over ∼5 years found that those who eventually developed mucosa-associated lymphoid tissue non-Hodgkin lymphoma (MALT NHL), a serious complication of SS, had significantly higher labial salivary gland P2X7R expression at the time of SS diagnosis compared to non-lymphoma SS patients, suggesting that P2X7R expression may be a useful biomarker for MALT NHL development (Baldini et al., 2017). In an analysis of P2X7R functional polymorphisms in 114 SS patients and 136 non-SS controls, the frequency of a single nucleotide polymorphism in exon 13 (A1405G, rs2230912) was significantly increased in seropositive SS patients, as compared to control subjects (Lester et al., 2013). As determined by ATP-induced ethidium bromide uptake to detect P2X7R activation in isolated peripheral blood lymphocytes, the P2X7R A1405G polymorphism was found to be a gain-of-function mutation that was suggested to be a risk factor for seropositive SS in the absence of other SS-associated human leukocyte antigen risk alleles. However, this A1405G association failed to be replicated in a larger patient cohort (Lester et al., 2013).
Antagonism of the P2X7R, whose encoding gene is located within a mapped SLE susceptibility region on chromosome 12 (Elliott et al., 2005), has been investigated as a potential treatment for several inflammatory diseases, including SLE (Turner et al., 2007; Taylor et al., 2009), rheumatoid arthritis (Arulkumaran et al., 2011) and chronic obstructive pulmonary disease (Lucattelli et al., 2011). Due to its increased expression in salivary gland biopsies from SS patients (Baldini et al., 2013) and its reported role in the activation of pro-inflammatory responses in salivary epithelium (Woods et al., 2012), the P2X7R has emerged as an appealing therapeutic target to treat SS. Our group reported that in vivo inhibition of P2X7Rs using the competitive antagonist A-438079 significantly reduced sialadenitis and improved carbachol-induced saliva flow in the NOD.H-2h4, CD28–/–, IFNγ–/– murine model of SS-like salivary gland autoimmune exocrinopathy (Khalafalla M.G. et al., 2017). P2X7R antagonism also significantly reduced salivary gland expression of immunoactive molecules known to be upregulated in salivary gland biopsies isolated from SS patients, including IL-1β, ICAM, VCAM, E-selectin, CD80, and CD86 (Tsunawaki et al., 2002; Khalafalla M.G. et al., 2017). Taken together, these studies suggest that the P2X7R represents a promising target for therapeutic intervention in salivary gland inflammation.
Previous studies have demonstrated that the P2Y2R is upregulated in major salivary glands of several mouse models of SS, including NOD.B10 (Schrader et al., 2005), IL-14α transgenic (IL-14αTG) (Woods et al., 2018) and C57BL/6-NOD.Aec1Aec2 mice (unpublished observations). It was recently reported by our group that P2Y2R expression was increased in both SMG epithelium and SMG-infiltrating B cells in aged IL-14αTG mice with SS-like disease and genetic deletion of the P2Y2R attenuated both B and T cell infiltration of the salivary glands (Woods et al., 2018). Additionally, attenuated sialadenitis following P2Y2R deletion correlated with significantly reduced levels of LT-α in salivary gland epithelial cells and infiltrating immune cells, suggesting that P2Y2R-mediated LT-α expression contributes to salivary gland inflammation in IL-14αTG mice (Woods et al., 2018). Interestingly, LT-α levels are increased in the saliva, serum and salivary glands of SS patients, as compared to healthy individuals (Shen et al., 2010; Teos et al., 2015), and blockade of the LT-α receptor has been shown to reduce sialadenitis and improve the secretory function of the salivary gland in the IL-14αTG and NOD mouse models of SS (Gatumu et al., 2009; Shen et al., 2013). Lastly, unpublished observations from our lab indicate that expression of the P2Y2R is increased in salivary gland-infiltrating B cells in NOD.H-2h4, CD28–/–, IFNγ–/– mice, as compared to B cells isolated from salivary glands of C57BL/6 control mice, and intraperitoneal administration of the selective P2Y2R antagonist AR-C118925 significantly attenuates sialadenitis and restores salivary gland function. In summary, these studies highlight the significant contributions of purinergic receptors to salivary gland inflammation and demonstrate their therapeutic potential for the treatment of human pro-inflammatory autoimmune diseases.
Radiation-induced salivary gland dysfunction is a common unintended side effect of radiotherapy in head and neck cancer patients, which causes xerostomia and hyposalivation that affects > 95% of these patients, > 73% of whom continue to suffer from months to years after completion of the radiotherapy (PDQ Supportive and Palliative Care Editorial Board, 2002; Dirix et al., 2006; Jensen et al., 2010; Pinna et al., 2015). Head and neck cancer patients routinely receive fractionated radiation treatment where the tumor region receives high radiation doses while salivary gland sparing techniques attempt to limit the radiation dose to 2 Gy/day (Eisbruch et al., 1999; Grundmann et al., 2009; Pfister et al., 2015). It is estimated that the tolerance dose for a 50% complications rate (TD50) for the parotid and submandibular glands is 28.4 and 39 Gy, respectively (Eisbruch et al., 1999; Li et al., 2007; Murdoch-Kinch et al., 2008). A number of factors including tumor grade, lymph node involvement and location of the tumor create scenarios where salivary gland sparing is not feasible and the tissue is exposed to higher radiation doses. Consequently, chronic hyposalivation and changes in the saliva electrolyte composition occur along with a reduction in pH that leads to alterations in oral microbial flora, increased incidence of dental carries and oral infections and difficulties with swallowing, digestion, and speech (Hu et al., 2013; Pinna et al., 2015).
Several groups have utilized rodent models to demonstrate that acute hyposalivation occurs immediately after IR, before the onset of overt gland damage, which is associated with sustained increases in the [Ca2+]i (Coppes et al., 2005; Liu et al., 2013, 2017; Ambudkar, 2018). In contrast, chronic IR-induced salivary dysfunction results from ROS production, increased caspase-3 activity, disruption of store-operated Ca2+ entry (SOCE), cytoskeletal rearrangements, acinar cell apoptosis, sialadenitis and replacement of normal parenchyma with fibrotic tissue (Coppes et al., 2001; Radfar and Sirois, 2003; Teymoortash et al., 2005; Muhvic-Urek et al., 2006; Avila et al., 2009; Liu et al., 2013, 2017; Wong et al., 2018). One of the early responses to IR is impairment of muscarinic receptor signaling (Coppes et al., 2000, 2005; Konings et al., 2005) required for saliva formation and aquaporin channel activity required for fluid secretion (Takagi et al., 2003). Furthermore, Avila et al. (2009), have demonstrated that radiation also causes a significant reduction in saliva-secreting acinar cells due to p53-dependent apoptosis. Thus, the overall mechanism of radiation-induced salivary gland hypofunction likely involves perturbations in muscarinic receptor signaling, apoptosis of saliva-producing acinar cells and irreversible tissue damage.
The P2X7R is highly expressed in salivary epithelium where its activation induces responses associated with IR-induced hyposalivation, including ROS production, caspase-3 activity, prostaglandin E2 and ATP release, NLRP3 inflammasome activation with IL-1β release and salivary gland cell apoptosis (Woods et al., 2012; Khalafalla M.G. et al., 2017; Gilman et al., 2019). Thus, we recently explored the role of P2X7R activation in γ-radiation-induced hyposalivation. IR exposure induced ATP release from wild type mouse parotid gland epithelial cells (PGECs) that was attenuated by the P2X7R antagonist A-438079 and in PGECs isolated from P2X7R–/– compared to wild type mice (Gilman et al., 2019). Furthermore, systemic administration of A-438079 in γ-irradiated wild type mice conferred significant radioprotection to salivary glands and maintained saliva flow rates similar to non-irradiated mice at 3 and 30 days post-IR. This study also demonstrated that PGE2 is secreted from wild type PGECs following γ-radiation that was reduced in P2X7R–/– PGECs or following A-438079 pretreatment of wild type PGECs (Gilman et al., 2019). Prostaglandins modulate inflammatory responses by altering cytokine production and secretion in macrophages (Ricciotti and Fitzgerald, 2011; Aoki and Narumiya, 2012). The signaling pathway downstream of cyclooxygenase-2 (COX-2), the rate-limiting enzyme that converts arachidonic acid into prostaglandins (Chandrasekharan and Simmons, 2004), has been shown to contribute to the IR-induced bystander effect in other cell types (Zhou et al., 2005; Chai et al., 2013; Kobayashi and Konishi, 2018) and P2X7R activation has been shown to induce arachidonic acid release from rat SMG ductal cells (Alzola et al., 1998). These findings suggest that P2X7R antagonists provide radioprotection by attenuating the damaging tissue response to IR-induced release of alarmins, including ATP and PGE2.
While most current treatments for salivary gland dysfunction target expansion of residual salivary acinar cells to repair damaged tissue, regenerative therapy with stem cells is a novel and promising therapeutic approach to replace damaged salivary glands (Carpenter and Cotroneo, 2010; Lombaert et al., 2017; Ogawa and Tsuji, 2017). Several studies have identified and characterized subsets of endogenous salivary progenitor cells that can be exploited to promote tissue regeneration (Lombaert et al., 2008; Chibly et al., 2014, 2018; Pringle et al., 2016; Emmerson et al., 2018; Weng et al., 2018). The use of modified fibrin hydrogels (Nam et al., 2019a), layered sheets of isolated salivary gland cells released from thermoresponsive culture dishes (Nam et al., 2019b) and salivary organoid cultures generated from embryonic pluripotent stem cells (Tanaka et al., 2018) have been explored as regenerative therapies for damaged salivary glands. Tissue engineering of 3-dimensional (3-D) primary HSG cultures for transplantation into afflicted patients represents another regenerative strategy to restore salivary gland function (Lombaert et al., 2017). Because primary human salivary gland cells undergo loss of cell-specific protein expression and biological function when cultured in a monolayer (Jang et al., 2015), development of 3-D culture strategies using Matrigel (Feng et al., 2009; Maria et al., 2011), collagen-Matrigel (Joraku et al., 2007; Pringle et al., 2016), hyaluronic acid-based hydrogels (Pradhan-Bhatt et al., 2013) and magnetic 3-D levitation (Ferreira et al., 2019) has been explored to maintain salivary gland cell function in culture. Indeed, transplantation of 3-D cultured, primary human salivary gland cells has been shown to ameliorate radiation-induced salivary gland dysfunction in mice (Pringle et al., 2016).
Rodent salivary glands have been shown to possess a high capacity to regenerate following the ligation or obstruction of the main excretory ducts of the gland, where ligated salivary glands initially become inflamed before glandular atrophy occurs through TGF-β-induced fibrosis and Fas ligand-induced epithelial cell apoptosis (Burford-Mason et al., 1993; Ahn et al., 2000; Takahashi et al., 2004, 2005, 2007; Carpenter et al., 2007; Woods et al., 2015). Following de-ligation, residual cells in damaged salivary glands can regenerate the gland through proliferation, migration and self-organization (Takahashi et al., 1998; Man et al., 2001; Kishi et al., 2006; Aure et al., 2015), thereby restoring salivary gland function, i.e., increasing the secretion rate of saliva with a normal ion and protein composition (Scott et al., 1999; Osailan et al., 2006). Concurrent with these glandular changes, functional P2Y2R expression, which is very low under homeostatic conditions, is robustly increased in salivary epithelial cells in response to ductal ligation and P2Y2R expression returns to basal low levels following de-ligation and subsequent recovery of the salivary gland (Ahn et al., 2000; El-Sayed et al., 2014). These findings are in agreement with previous studies demonstrating P2Y2R upregulation in epithelial cells in response to tissue damage and inflammation (Turner et al., 1997; Schrader et al., 2005; Degagne et al., 2009; Woods et al., 2018), suggesting that the P2Y2R is an important component in the repair and regeneration of damaged salivary glands.
Previous studies have demonstrated a role for the P2Y2R in corneal epithelial wound healing by increasing cell migration (Boucher et al., 2010), in liver regeneration by stimulating hepatocyte proliferation (Tackett et al., 2014), in cardiac regeneration by stimulating cardiac progenitor cell proliferation (Khalafalla F.G. et al., 2017) and in intestinal epithelial cell tubulogenesis (Ibuka et al., 2015). Activation of P2Y2Rs in the HSG cell line also induces the transactivation, homodimerization and autophosphorylation of the EGFR, a receptor tyrosine kinase known to be crucial for salivary gland branching morphogenesis and development (Miyazaki et al., 2004; Patel et al., 2006; Mizukoshi et al., 2016). This process in salivary epithelial and endothelial cells involves ADAM10/17-dependent proteolytic cleavage induced by P2Y2R activation that causes the release of cell surface-bound EGFR ligands as well as the Src kinase-dependent transactivation of growth factor receptors through the binding of Src to SH3 binding motifs in the P2Y2R intracellular domain (Liu et al., 2004; Seye et al., 2004; Ratchford et al., 2010). In HSG cells, P2Y2R activation also induces the heterodimerization of EGFR and ErbB3, another member of the EGFR family (Ratchford et al., 2010). ErbB3 has an inactive kinase domain that requires heterodimerization with EGFR to respond to its ligand, neuregulin, which then stimulates the ERK/MAPK signaling pathway to promote cell proliferation, migration, and differentiation (Patel et al., 2006; Ratchford et al., 2010).
Integrins are transmembrane cell surface receptors that interact with extracellular matrix components, including laminin (Nishiuchi et al., 2006), fibronectin (Bharadwaj et al., 2017) and collagen (Tuckwell and Humphries, 1996), intracellular cytoskeletal proteins and other cell surface receptors (Legate et al., 2009) that are crucial components in the salivary gland regeneration process (Wei et al., 2007; El-Sayed et al., 2014). Hence, the bi-directional nature of integrin signaling regulates many physiological processes relevant to salivary gland regeneration, including cell proliferation, polarity, migration, and adhesion (Legate et al., 2009). Through its extracellular RGD domain, the P2Y2R can bind directly to integrins (e.g., αvβ3/5) and allow for nucleotide-induced P2Y2R-mediated activation of integrin signaling pathways, including Rho and Rac GTPase activation that regulate cytoskeletal rearrangements (Erb et al., 2001; Wang et al., 2005). The extracellular ligand for the α5β1 integrin is fibronectin, a well-known mediator of salivary gland morphogenesis (Sakai et al., 2003; Onodera et al., 2010), and we have previously demonstrated that UTP-induced P2Y2R activation also induces α5β1 integrin-mediated migration, aggregation, and self-organization of dispersed salivary epithelial cells into acinar-like spheres (El-Sayed et al., 2014). These spheres resemble native acinar units of the salivary gland, possessing a lumen and organized expression of the tight junction protein ZO-1, and we have shown that the mechanism for P2Y2R-mediated self-organization of salivary gland cells involves the activation of EGFR via the Cdc42 Rho GTPase pathway and subsequent downstream activation of ERK1/2 and JNK signaling pathways (El-Sayed et al., 2014). Thus, these studies suggest a promising role for unique structural motifs in P2Y2Rs that are highly relevant to cell-based regenerative therapy and bioengineering of salivary glands.
Activation of purinergic receptors for extracellular nucleotides in the salivary glands modulates various physiological and pathophysiological functions (Table 1). The ATP-gated ionotropic P2X7 receptor in salivary acinar cells contributes to physiological salivary gland function by modulating muscarinic receptor-induced saliva secretion into the ductal lumen, whereas activation of ductal P2X7Rs modulates ion and protein content of saliva. P2X4R activation also contributes to saliva secretion through the formation of functional homotrimers and P2X4R/P2X7R heterotrimers in salivary gland epithelium, suggesting that P2XRs represent an integration point between canonical and non-canonical signaling pathways that regulate saliva flow and composition. P2Y1Rs also may contribute to salivary gland development through coupling to multiple G proteins resulting in diverse physiological responses. The ability of P2Y2R activation to stimulate increases in [Ca2+]i and Cl– flow across epithelial membranes suggests a role in saliva secretion, however, P2Y2R expression is negligible under normal steady-state conditions. The observed upregulation of P2Y2R expression during tissue stress and in response to P2X7R-induced IL-1β release suggest their significant role in salivary gland pathophysiology. Due to an increase in extracellular nucleotide release during tissue inflammation and dysregulation, nucleotide-induced activation of the interconnected P2X7R-P2Y2R signaling pathways likely modulates multiple immunological and tissue repair functions, including cell migration, growth factor receptor transactivation, integrin signaling, adhesion molecule upregulation, and cytokine release. Thus, P2X7R activation in salivary epithelium and upregulation of the P2Y2R with its unique structural domains likely regulate both salivary gland dysfunction and repair through the stimulation of these important pro-inflammatory processes.
In conclusion, purinergic receptors have emerged as promising therapeutic targets to promote physiological saliva flow, prevent salivary gland inflammation and enhance tissue regeneration required to reverse common causes of salivary gland dysfunction in humans, such as the autoimmune disease SS or the side effect of radiotherapy in head and neck cancer patients. Because purinergic receptors share common agonists and form heteromeric receptors with distinct pharmacologic profiles, unraveling the contribution of intracellular P2 receptor cross-talk to salivary gland dysfunction in animal models and humans will further define their therapeutic value in the treatment of salivary gland disorders. The continued development of high affinity P2R agonists and antagonists and the investigation of their safety and efficacy represent the next steps in the clinical translation of this promising P2 receptor research.
MK, LW, KJ, KF, and JC reviewed literature and drafted the manuscript. MK, LW, KJ, KF, JC, JJ, KL, HG, and GW critically revised, edited, and approved the manuscript.
This work was supported by the National Institute of Dental & Craniofacial Research grants R01DE007389 and R01DE023342 without their involvement in the study design, data collection, data interpretation, or manuscript preparation. This work was also supported by funding from the Faculty of Dentistry at the University of Oslo.
The authors declare that the research was conducted in the absence of any commercial or financial relationships that could be construed as a potential conflict of interest.
Abbracchio, M. P., Burnstock, G., Boeynaems, J. M., Barnard, E. A., Boyer, J. L., Kennedy, C., et al. (2006). International Union of Pharmacology LVIII: update on the P2Y G protein-coupled nucleotide receptors: from molecular mechanisms and pathophysiology to therapy. Pharmacol. Rev. 58, 281–341. doi: 10.1124/pr.58.3.3
Abdulqawi, R., Dockry, R., Holt, K., Layton, G., McCarthy, B. G., Ford, A. P., et al. (2015). P2X3 receptor antagonist (AF-219) in refractory chronic cough: a randomised, double-blind, placebo-controlled phase 2 study. Lancet 385, 1198–1205. doi: 10.1016/S0140-6736(14)61255-1
Accurso, F. J., Moss, R. B., Wilmott, R. W., Anbar, R. D., Schaberg, A. E., Durham, T. A., et al. (2011). Denufosol tetrasodium in patients with cystic fibrosis and normal to mildly impaired lung function. Am. J. Respir. Crit. Care Med. 183, 627–634. doi: 10.1164/rccm.201008-1267OC
Adinolfi, E., Pizzirani, C., Idzko, M., Panther, E., Norgauer, J., Di Virgilio, F., et al. (2005). P2X7 receptor: death or life? Purinergic Signal. 1, 219–227. doi: 10.1007/s11302-005-6322-x
Agrawal, A., and Gartland, A. (2015). P2X7 receptors: role in bone cell formation and function. J. Mol. Endocrinol. 54, R75–R88. doi: 10.1530/JME-14-0226
Ahn, J. S., Camden, J. M., Schrader, A. M., Redman, R. S., and Turner, J. T. (2000). Reversible regulation of P2Y(2) nucleotide receptor expression in the duct-ligated rat submandibular gland. Am. J. Physiol. Cell Physiol. 279, C286–C294.
Ajit, D., Woods, L. T., Camden, J. M., Thebeau, C. N., El-Sayed, F. G., Greeson, G. W., et al. (2014). Loss of P2Y(2) nucleotide receptors enhances early pathology in the TgCRND8 mouse model of Alzheimer’s disease. Mol. Neurobiol. 49, 1031–1042. doi: 10.1007/s12035-013-8577-5
Alzola, E., Chaib, N., Pochet, S., Kabre, E., Marino, A., and Dehaye, J. P. (2001). Modulation by propranolol of the uptake of ethidium bromide by rat submandibular acinar cells exposed to a P2X(7) agonist or to maitotoxin. CellSignal. 13, 465–473. doi: 10.1016/s0898-6568(01)00165-6
Alzola, E., Perez-Etxebarria, A., Kabre, E., Fogarty, D. J., Metioui, M., Chaib, N., et al. (1998). Activation by P2X7 agonists of two phospholipases A2 (PLA2) in ductal cells of rat submandibular gland. Coupling of the calcium-independent PLA2 with kallikrein secretion. J. Biol. Chem. 273, 30208–30217. doi: 10.1074/jbc.273.46.30208
Ambudkar, I. (2018). Calcium signaling defects underlying salivary gland dysfunction. Biochim. Biophys. Acta Mol. Cell Res. 1865, 1771–1777. doi: 10.1016/j.bbamcr.2018.07.002
Ambudkar, I. S. (2014). Ca(2)(+) signaling and regulation of fluid secretion in salivary gland acinar cells. Cell Calcium 55, 297–305. doi: 10.1016/j.ceca.2014.02.009
Antonio, L. S., Stewart, A. P., Xu, X. J., Varanda, W. A., Murrell-Lagnado, R. D., and Edwardson, J. M. (2011). P2X4 receptors interact with both P2X2 and P2X7 receptors in the form of homotrimers. Br. J. Pharmacol. 163, 1069–1077. doi: 10.1111/j.1476-5381.2011.01303.x
Aoki, T., and Narumiya, S. (2012). Prostaglandins and chronic inflammation. Trends Pharmacol. Sci. 33, 304–311. doi: 10.1016/j.tips.2012.02.004
Arulkumaran, N., Unwin, R. J., and Tam, F. W. (2011). A potential therapeutic role for P2X7 receptor (P2X7R) antagonists in the treatment of inflammatory diseases. Expert Opin. Investig. Drugs 20, 897–915. doi: 10.1517/13543784.2011.578068
Atkinson, J. C., Grisius, M., and Massey, W. (2005). Salivary hypofunction and xerostomia: diagnosis and treatment. Dent. Clin. North. Am. 49, 309–326. doi: 10.1016/j.cden.2004.10.002
Aure, M. H., Konieczny, S. F., and Ovitt, C. E. (2015). Salivary gland homeostasis is maintained through acinar cell self-duplication. Dev. Cell 33, 231–237. doi: 10.1016/j.devcel.2015.02.013
Aure, M. H., Roed, A., and Galtung, H. K. (2010). Intracellular Ca2+ responses and cell volume regulation upon cholinergic and purinergic stimulation in an immortalized salivary cell line. Eur. J. Oral Sci. 118, 237–244. doi: 10.1111/j.1600-0722.2010.00738.x
Avila, J. L., Grundmann, O., Burd, R., and Limesand, K. H. (2009). Radiation-induced salivary gland dysfunction results from p53-dependent apoptosis. Int. J. Radiat. Oncol. Biol. Phys. 73, 523–529. doi: 10.1016/j.ijrobp.2008.09.036
Bacman, S., Sterin-Borda, L., José Camusso, J., Arana, R., Hubscher, O., and Borda, E. (1996). Circulating antibodies against rat parotid gland M3 muscarinic receptors in primary Sjögren’s syndrome. Clin. Exp. Immunol. 104, 454–459. doi: 10.1046/j.1365-2249.1996.42748.x
Bagchi, S., Liao, Z., Gonzalez, F. A., Chorna, N. E., Seye, C. I., Weisman, G. A., et al. (2005). The P2Y2 nucleotide receptor interacts with alphav integrins to activate Go and induce cell migration. J. Biol. Chem. 280, 39050–39057. doi: 10.1074/jbc.m504819200
Baker, O. J., Camden, J. M., Ratchford, A. M., Seye, C. I., Erb, L., and Weisman, G. A. (2006). Differential coupling of the P2Y1 receptor to Galpha14 and Galphaq/11 proteins during the development of the rat salivary gland. Arch. Oral. Biol. 51, 359–370. doi: 10.1016/j.archoralbio.2005.10.004
Baker, O. J., Camden, J. M., Rome, D. E., Seye, C. I., and Weisman, G. A. (2008). P2Y2 nucleotide receptor activation up-regulates vascular cell adhesion molecule-1 [corrected] expression and enhances lymphocyte adherence to a human submandibular gland cell line. Mol. Immunol. 45, 65–75. doi: 10.1016/j.molimm.2007.05.009
Baldini, C., Rossi, C., Ferro, F., Santini, E., Seccia, V., Donati, V., et al. (2013). The P2X7 receptor-inflammasome complex has a role in modulating the inflammatory response in primary Sjogren’s syndrome. J. Intern. Med. 274, 480–489. doi: 10.1111/joim.12115
Baldini, C., Santini, E., Rossi, C., Donati, V., and Solini, A. (2017). The P2X7 receptor-NLRP3 inflammasome complex predicts the development of non-Hodgkin’s lymphoma in Sjogren’s syndrome: a prospective, observational, single-centre study. J. Intern. Med. 282, 175–186. doi: 10.1111/joim.12631
Baldys-Waligorska, A., Pour, A., Moriarty, C. M., and Dowd, F. (1987). The effect of calcium and cyclic AMP on amylase release in digitonin-permeabilized parotid gland cells. Biochim. Biophys. Acta 929, 190–196. doi: 10.1016/0167-4889(87)90175-3
Baranska, J., Czajkowski, R., and Pomorski, P. (2017). P2Y1 receptors - properties and functional activities. Adv. Exp. Med. Biol. 1051, 71–89. doi: 10.1007/5584_2017_57
Barragan-Iglesias, P., Mendoza-Garces, L., Pineda-Farias, J. B., Solano-Olivares, V., Rodriguez-Silverio, J., Flores-Murrieta, F. J., et al. (2015). Participation of peripheral P2Y1, P2Y6 and P2Y11 receptors in formalin-induced inflammatory pain in rats. Pharmacol. Biochem. Behav. 128, 23–32. doi: 10.1016/j.pbb.2014.11.001
Bharadwaj, M., Strohmeyer, N., Colo, G. P., Helenius, J., Beerenwinkel, N., Schiller, H. B., et al. (2017). αV-class integrins exert dual roles on α5β1 integrins to strengthen adhesion to fibronectin. Nat. Commun. 8:14348.
Bhattacharya, A., and Ceusters, M. (2019). Targeting neuroinflammation with brain penetrant P2X7 antagonists as novel therapeutics for neuropsychiatric disorders. Neuropsychopharmacology 45, 234–235. doi: 10.1038/s41386-019-0502-9
Bhattacharya, S., Imbery, J. F., Ampem, P. T., and Giovannucci, D. R. (2015). Crosstalk between purinergic receptors and canonical signaling pathways in the mouse salivary gland. Cell Calcium 58, 589–597. doi: 10.1016/j.ceca.2015.09.006
Bhattacharya, S., Verrill, D. S., Carbone, K. M., Brown, S., Yule, D. I., and Giovannucci, D. R. (2012). Distinct contributions by ionotropic purinoceptor subtypes to ATP-evoked calcium signals in mouse parotid acinar cells. J. Physiol. 590, 2721–2737. doi: 10.1113/jphysiol.2012.228148
Boehlke, C., Zierau, O., and Hannig, C. (2015). Salivary amylase - The enzyme of unspecialized euryphagous animals. Arch. Oral. Biol. 60, 1162–1176. doi: 10.1016/j.archoralbio.2015.05.008
Bondu, V., Bitting, C., Poland, V. L., Hanson, J. A., Harkins, M. S., Lathrop, S., et al. (2018). Upregulation of P2Y2R, active uPA, and PAI-1 are essential components of hantavirus cardiopulmonary syndrome. Front. Cell Infect. Microbiol. 8:169. doi: 10.3389/fcimb.2018.00169
Boucher, I., Rich, C., Lee, A., Marcincin, M., and Trinkaus-Randall, V. (2010). The P2Y2 receptor mediates the epithelial injury response and cell migration. Am. J. Physiol. Cell Physiol. 299, C411–C421. doi: 10.1152/ajpcell.00100.2009
Boumechache, M., Masin, M., Edwardson, J. M., Gorecki, D. C., and Murrell-Lagnado, R. (2009). Analysis of assembly and trafficking of native P2X4 and P2X7 receptor complexes in rodent immune cells. J. Biol. Chem. 284, 13446–13454. doi: 10.1074/jbc.M901255200
Brown, D. A., Bruce, J. I., Straub, S. V., and Yule, D. I. (2004). cAMP potentiates ATP-evoked calcium signaling in human parotid acinar cells. J. Biol. Chem. 279, 39485–39494. doi: 10.1074/jbc.m406201200
Bruce, J. I., Shuttleworth, T. J., Giovannucci, D. R., and Yule, D. I. (2002). Phosphorylation of inositol 1,4,5-trisphosphate receptors in parotid acinar cells. A mechanism for the synergistic effects of cAMP on Ca2+ signaling. J. Biol. Chem. 277, 1340–1348. doi: 10.1074/jbc.m106609200
Buisman, H. P., Steinberg, T. H., Fischbarg, J., Silverstein, S. C., Vogelzang, S. A., Ince, C., et al. (1988). Extracellular ATP induces a large nonselective conductance in macrophage plasma membranes. Proc. Natl. Acad. Sci. U.S.A. 85, 7988–7992. doi: 10.1073/pnas.85.21.7988
Burford-Mason, A. P., Cummins, M. M., Brown, D. H., Mackay, A. J., and Dardick, I. (1993). Immunohistochemical analysis of the proliterative capacity of duct and acinar cells during ligation-induced atrophy and subsequent regeneration of rat parotid gland. J. Oral Pathol. Med. 22, 440–446. doi: 10.1111/j.1600-0714.1993.tb00122.x
Burnstock, G. (2017). Purinergic signalling: therapeutic developments. Front. Pharmacol. 8:661. doi: 10.3389/fphar.2017.00661
Burnstock, G., Dumsday, B., and Smythe, A. (1972). Atropine resistant excitation of the urinary bladder: the possibility of transmission via nerves releasing a purine nucleotide. Br. J. Pharmacol. 44, 451–461. doi: 10.1111/j.1476-5381.1972.tb07283.x
Burnstock, G., and Knight, G. E. (2018). The potential of P2X7 receptors as a therapeutic target, including inflammation and tumour progression. Purinergic Signal. 14, 1–18. doi: 10.1007/s11302-017-9593-0
Burnstock, G., and Novak, I. (2012). Purinergic signalling in the pancreas in health and disease. J. Endocrinol. 213, 123–141. doi: 10.1530/JOE-11-0434
Buvinic, S., Bravo-Zehnder, M., Boyer, J. L., Huidobro-Toro, J. P., and Gonzalez, A. (2007). Nucleotide P2Y1 receptor regulates EGF receptor mitogenic signaling and expression in epithelial cells. J. Cell Sci. 120, 4289–4301. doi: 10.1242/jcs.03490
Camden, J. M., Schrader, A. M., Camden, R. E., Gonzalez, F. A., Erb, L., Seye, C. I., et al. (2005). P2Y2 nucleotide receptors enhance alpha-secretase-dependent amyloid precursor protein processing. J. Biol. Chem. 280, 18696–18702. doi: 10.1074/jbc.m500219200
Cao, F., Hu, L. Q., Yao, S. R., Hu, Y., Wang, D. G., Fan, Y. G., et al. (2019). P2X7 receptor: a potential therapeutic target for autoimmune diseases. Autoimmun. Rev. 18, 767–777. doi: 10.1016/j.autrev.2019.06.009
Carpenter, G. H., and Cotroneo, E. (2010). Salivary Gland Regeneration in Salivary Glands. Basel: Karger Publishers, 107–128.
Carpenter, G. H., Osailan, S. M., Correia, P., Paterson, K. P., and Proctor, G. B. (2007). Rat salivary gland ligation causes reversible secretory hypofunction. Acta Physiol. 189, 241–249. doi: 10.1111/j.1365-201x.2006.01662.x
Casas-Pruneda, G., Reyes, J. P., Pérez-Flores, G., Pérez-Cornejo, P., and Arreola, J. (2009). Functional interactions between P2X4 and P2X7 receptors from mouse salivary epithelia. J. Physiol. 587, 2887–2901. doi: 10.1113/jphysiol.2008.167395
Chai, Y., Calaf, G. M., Zhou, H., Ghandhi, S. A., Elliston, C. D., Wen, G., et al. (2013). Radiation induced COX-2 expression and mutagenesis at non-targeted lung tissues of gpt delta transgenic mice. Br. J. Cancer 108, 91–98. doi: 10.1038/bjc.2012.498
Chambers, M. S., Garden, A. S., Kies, M. S., and Martin, J. W. (2004). Radiation-induced xerostomia in patients with head and neck cancer: pathogenesis, impact on quality of life, and management. Head Neck 26, 796–807. doi: 10.1002/hed.20045
Chan, H. C., Cheung, W. T., Leung, P. Y., Wu, L. J., Chew, S. B., Ko, W. H., et al. (1996). Purinergic regulation of anion secretion by cystic fibrosis pancreatic duct cells. Am. J. Physiol. 271, C469–C477.
Chan, H. C., Liu, C. Q., Fong, S. K., Law, S. H., Wu, L. J., So, E., et al. (1997). Regulation of Cl- secretion by extracellular ATP in cultured mouse endometrial epithelium. J. Membr. Biol. 156, 45–52. doi: 10.1007/s002329900186
Chekeni, F. B., Elliott, M. R., Sandilos, J. K., Walk, S. F., Kinchen, J. M., Lazarowski, E. R., et al. (2010). Pannexin 1 channels mediate ‘find-me’ signal release and membrane permeability during apoptosis. Nature 467, 863–867. doi: 10.1038/nature09413
Chen, X., Qian, S., Hoggatt, A., Tang, H., Hacker, T. A., Obukhov, A. G., et al. (2017). Endothelial Cell-Specific Deletion of P2Y2 Receptor Promotes Plaque Stability in Atherosclerosis-Susceptible ApoE-Null Mice. Arterioscler. Thromb. Vasc. Biol. 37, 75–83. doi: 10.1161/ATVBAHA.116.308561
Chibly, A. M., Querin, L., Harris, Z., and Limesand, K. H. (2014). Label-retaining cells in the adult murine salivary glands possess characteristics of adult progenitor cells. PLoS One 9:e107893. doi: 10.1371/journal.pone.0107893
Chibly, A. M., Wong, W. Y., Pier, M., Cheng, H., Mu, Y., Chen, J., et al. (2018). aPKCzeta-dependent Repression of Yap is Necessary for Functional Restoration of Irradiated Salivary Glands with IGF-1. Sci. Rep. 8:6347. doi: 10.1038/s41598-018-24678-4
Chojnowska, S., Baran, T., Wilinska, I., Sienicka, P., Cabaj-Wiater, I., and Knas, M. (2018). Human saliva as a diagnostic material. Adv. Med. Sci. 63, 185–191. doi: 10.1016/j.advms.2017.11.002
Christoffersen, B. C., Hug, M. J., and Novak, I. (1998). Different purinergic receptors lead to intracellular calcium increases in pancreatic ducts. Pflugers. Arch. 436, 33–39. doi: 10.1007/s004240050601
Chrovian, C. C., Rech, J. C., Bhattacharya, A., and Letavic, M. A. (2014). P2X7 antagonists as potential therapeutic agents for the treatment of CNS disorders. Prog. Med. Chem. 53, 65–100. doi: 10.1016/B978-0-444-63380-4.00002-0
Clarke, L. L., Harline, M. C., Otero, M. A., Glover, G. G., Garrad, R. C., Krugh, B., et al. (1999). Desensitization of P2Y2 receptor-activated transepithelial anion secretion. Am. J. Physiol. 276, C777–C787. doi: 10.1152/ajpcell.1999.276.4.C777
Coppes, R. P., Meter, A., Latumalea, S. P., Roffel, A. F., and Kampinga, H. H. (2005). Defects in muscarinic receptor-coupled signal transduction in isolated parotid gland cells after in vivo irradiation: evidence for a non-DNA target of radiation. Br. J. Cancer 92, 539–546. doi: 10.1038/sj.bjc.6602365
Coppes, R. P., Roffel, A. F., Zeilstra, L. J., Vissink, A., and Konings, A. W. (2000). Early radiation effects on muscarinic receptor-induced secretory responsiveness of the parotid gland in the freely moving rat. Radiat. Res. 153, 339–346. doi: 10.1667/0033-7587(2000)153[0339:ereomr]2.0.co;2
Coppes, R. P., Zeilstra, L. J., Kampinga, H. H., and Konings, A. W. (2001). Early to late sparing of radiation damage to the parotid gland by adrenergic and muscarinic receptor agonists. Br. J. Cancer 85, 1055–1063. doi: 10.1054/bjoc.2001.2038
Coutinho-Silva, R., Parsons, M., Robson, T., and Burnstock, G. (2001). Changes in expression of P2 receptors in rat and mouse pancreas during development and ageing. Cell Tissue Res. 306, 373–383. doi: 10.1007/s004410100458
Dahlquist, R., Diamant, B., and Kruger, P. G. (1974). Increased permeability of the rat mast cell membrane to sodium and potassium caused by extracellular ATP and its relationship to histamine release. Int. Arch. Allergy Appl. Immunol. 46, 655–675. doi: 10.1159/000231167
Daridon, C., Devauchelle, V., Hutin, P., Le Berre, R., Martins-Carvalho, C., Bendaoud, B., et al. (2007). Aberrant expression of BAFF by B lymphocytes infiltrating the salivary glands of patients with primary Sjogren’s syndrome. Arthritis Rheum. 56, 1134–1144. doi: 10.1002/art.22458
Daridon, C., Pers, J. O., Devauchelle, V., Martins-Carvalho, C., Hutin, P., Pennec, Y. L., et al. (2006). Identification of transitional type II B cells in the salivary glands of patients with Sjogren’s syndrome. Arthritis Rheum. 54, 2280–2288. doi: 10.1002/art.21936
Dartt, D. A., and Hodges, R. R. (2011a). Cholinergic agonists activate P2X7 receptors to stimulate protein secretion by the rat lacrimal gland. Invest. Ophthalmol. Vis. Sci. 52, 3381–3390. doi: 10.1167/iovs.11-7210
Dartt, D. A., and Hodges, R. R. (2011b). Interaction of alpha1D-adrenergic and P2X(7) receptors in the rat lacrimal gland and the effect on intracellular [Ca2+] and protein secretion. Invest. Ophthalmol. Vis. Sci. 52, 5720–5729. doi: 10.1167/iovs.11-7358
Dawes, C., Pedersen, A. M., Villa, A., Ekstrom, J., Proctor, G. B., Vissink, A., et al. (2015). The functions of human saliva: a review sponsored by the World Workshop on Oral Medicine VI. Arch. Oral. Biol. 60, 863–874. doi: 10.1016/j.archoralbio.2015.03.004
Dawson, L., Stanbury, J., Venn, N., Hasdimir, B., Rogers, S., and Smith, P. (2006). Antimuscarinic antibodies in primary Sjögren’s syndrome reversibly inhibit the mechanism of fluid secretion by human submandibular salivary acinar cells. Arthritis Rheumatism 54, 1165–1173. doi: 10.1002/art.21764
de Almeida Pdel, V., Gregio, A. M., Machado, M. A., De Lima, A. A., and Azevedo, L. R. (2008). Saliva composition and functions: a comprehensive review. J. Contemp. Dent. Pract. 9, 72–80. doi: 10.5005/jcdp-9-3-72
De Marchi, E., Orioli, E., Pegoraro, A., Adinolfi, E., and Di Virgilio, F. (2020). Detection of Extracellular ATP in the Tumor Microenvironment. Using the pmeLUC Biosensor. Methods Mol. Biol. 2041, 183–195. doi: 10.1007/978-1-4939-9717-6_13
De Marchi, E., Orioli, E., Pegoraro, A., Sangaletti, S., Portararo, P., Curti, A., et al. (2019). The P2X7 receptor modulates immune cells infiltration, ectonucleotidases expression and extracellular ATP levels in the tumor microenvironment. Oncogene 38, 3636–3650. doi: 10.1038/s41388-019-0684-y
Deaglio, S., and Robson, S. C. (2011). Ectonucleotidases as regulators of purinergic signaling in thrombosis, inflammation, and immunity. Adv. Pharmacol. 61, 301–332. doi: 10.1016/B978-0-12-385526-8.00010-2
Degagne, E., Grbic, D. M., Dupuis, A. A., Lavoie, E. G., Langlois, C., Jain, N., et al. (2009). P2Y2 receptor transcription is increased by NF-kappa B and stimulates cyclooxygenase-2 expression and PGE2 released by intestinal epithelial cells. J. Immunol. 183, 4521–4529. doi: 10.4049/jimmunol.0803977
Dehaye, J. P. (1993). ATP4- increases the intracellular calcium concentration in rat submandibular glands. Gen. Pharmacol. 24, 1097–1100. doi: 10.1016/0306-3623(93)90355-2
Di Virgilio, F., Dal Ben, D., Sarti, A. C., Giuliani, A. L., and Falzoni, S. (2017). The P2X7 Receptor in Infection and Inflammation. Immunity 47, 15–31. doi: 10.1016/j.immuni.2017.06.020
Di Virgilio, F., Sarti, A. C., Falzoni, S., De Marchi, E., and Adinolfi, E. (2018). Extracellular ATP and P2 purinergic signalling in the tumour microenvironment. Nat. Rev. Cancer 18, 601–618. doi: 10.1038/s41568-018-0037-0
Dirix, P., Nuyts, S., and Van Den Bogaert, W. (2006). Radiation-induced xerostomia in patients with head and neck cancer: a literature review. Cancer 107, 2525–2534. doi: 10.1002/cncr.22302
Dorsam, R. T., and Kunapuli, S. P. (2004). Central role of the P2Y12 receptor in platelet activation. J. Clin. Invest. 113, 340–345. doi: 10.1172/jci20986
Eisbruch, A., Ten Haken, R. K., Kim, H. M., Marsh, L. H., and Ship, J. A. (1999). Dose, volume, and function relationships in parotid salivary glands following conformal and intensity-modulated irradiation of head and neck cancer. Int. J. Radiat. Oncol. Biol. Phys. 45, 577–587. doi: 10.1016/s0360-3016(99)00247-3
Ekström, J. (1999). “Role of nonadrenergic, noncholinergic autonomic transmitters in salivary glandular activities in vivo,” in Neural Mechanisms of Salivary Gland Secretion, eds J. Ekström, and J. R. Garrett, (Basel: Karger Publishers), 94–130. doi: 10.1159/000061124
Ekström, J., Månsson, B., Olgart, L., and Tobin, G. (1988). Non-adrenergic, non-cholinergic salivary secretion in the ferret. Exp. Physiol. 73, 163–173. doi: 10.1113/expphysiol.1988.sp003129
Elliott, J. I., Mcvey, J. H., and Higgins, C. F. (2005). The P2X7 receptor is a candidate product of murine and human lupus susceptibility loci: a hypothesis and comparison of murine allelic products. Arthritis Res. Ther. 7, R468–R475.
El-Sayed, F. G., Camden, J. M., Woods, L. T., Khalafalla, M. G., Petris, M. J., Erb, L., et al. (2014). P2Y2 nucleotide receptor activation enhances the aggregation and self-organization of dispersed salivary epithelial cells. Am. J. Physiol. Cell Physiol. 307, C83–C96. doi: 10.1152/ajpcell.00380.2013
Eltzschig, H. K., Eckle, T., Mager, A., Kuper, N., Karcher, C., Weissmuller, T., et al. (2006). ATP release from activated neutrophils occurs via connexin 43 and modulates adenosine-dependent endothelial cell function. Circ. Res. 99, 1100–1108. doi: 10.1161/01.res.0000250174.31269.70
Emmerson, E., May, A. J., Berthoin, L., Cruz-Pacheco, N., Nathan, S., Mattingly, A. J., et al. (2018). Salivary glands regenerate after radiation injury through SOX2-mediated secretory cell replacement. EMBO Mol. Med. 10:e08051. doi: 10.15252/emmm.201708051
Erb, L., Liu, J., Ockerhausen, J., Kong, Q., Garrad, R. C., Griffin, K., et al. (2001). An RGD sequence in the P2Y(2) receptor interacts with alpha(V)beta(3) integrins and is required for G(o)-mediated signal transduction. J. Cell Biol. 153, 491–501.
Erb, L., Lustig, K. D., Sullivan, D. M., Turner, J. T., and Weisman, G. A. (1993). Functional expression and photoaffinity labeling of a cloned P2U purinergic receptor. Proc. Natl. Acad. Sci. U.S.A. 90, 10449–10453. doi: 10.1073/pnas.90.22.10449
Fabbrizio, P., Apolloni, S., Bianchi, A., Salvatori, I., Valle, C., Lanzuolo, C., et al. (2019). P2X7 activation enhances skeletal muscle metabolism and regeneration in SOD1G93A mouse model of amyotrophic lateral sclerosis. Brain Pathol. doi: 10.1111/bpa.12774 [Epub ahead of print].
Fabre, J. E., Nguyen, M., Latour, A., Keifer, J. A., Audoly, L. P., Coffman, T. M., et al. (1999). Decreased platelet aggregation, increased bleeding time and resistance to thromboembolism in P2Y1-deficient mice. Nat. Med. 5, 1199–1202. doi: 10.1038/13522
Feng, J., Van Der Zwaag, M., Stokman, M. A., Van Os, R., and Coppes, R. P. (2009). Isolation and characterization of human salivary gland cells for stem cell transplantation to reduce radiation-induced hyposalivation. Radiother. Oncol. 92, 466–471. doi: 10.1016/j.radonc.2009.06.023
Feng, W., Yang, F., Wang, R., Yang, X., Wang, L., Chen, C., et al. (2016). High Level P2X7-mediated signaling impairs function of hematopoietic stem/progenitor cells. Stem Cell Rev. Rep. 12, 305–314. doi: 10.1007/s12015-016-9651-y
Ferrari, D., Chiozzi, P., Falzoni, S., Dal Susino, M., Melchiorri, L., Baricordi, O. R., et al. (1997). Extracellular ATP triggers IL-1 beta release by activating the purinergic P2Z receptor of human macrophages. J. Immunol. 159, 1451–1458.
Ferreira, J. N., Hasan, R., Urkasemsin, G., Ng, K. K., Adine, C., Muthumariappan, S., et al. (2019). A magnetic three-dimensional levitated primary cell culture system for the development of secretory salivary gland-like organoids. J. Tissue Eng. Regen. Med. 13, 495–508. doi: 10.1002/term.2809
Frenkel, E. S., and Ribbeck, K. (2015). Salivary mucins in host defense and disease prevention. J. Oral Microbiol. 7:29759. doi: 10.3402/jom.v7.29759
Fukushi, Y. (1999). Heterologous desensitization of muscarinic receptors by P2Z purinoceptors in rat parotid acinar cells. Eur. J. Pharmacol. 364, 55–64. doi: 10.1016/s0014-2999(98)00824-3
Furness, S., Worthington, H. V., Bryan, G., Birchenough, S., and Mcmillan, R. (2011). Interventions for the management of dry mouth: topical therapies. Cochrane Database Syst. Rev. 5:CD008934. doi: 10.1002/14651858.CD008934.pub2
Galietta, L. J., Zegarra-Moran, O., Mastrocola, T., Wohrle, C., Rugolo, M., and Romeo, G. (1994). Activation of Ca(2+)-dependent K+ and Cl- currents by UTP and ATP in CFPAC-1 cells. Pflugers. Arch. 426, 534–541. doi: 10.1007/bf00378531
Gallacher, D. V. (1982). Are there purinergic receptors on parotid acinar cells? Nature 296, 83–86. doi: 10.1038/296083a0
Gatumu, M. K., Skarstein, K., Papandile, A., Browning, J. L., Fava, R. A., and Bolstad, A. I. (2009). Blockade of lymphotoxin-beta receptor signaling reduces aspects of Sjögren’s syndrome in salivary glands of non-obese diabetic mice. Arthritis Res. Ther. 11:R24.
Gibbons, S. J., Washburn, K. B., and Talamo, B. R. (2001). P2X(7) receptors in rat parotid acinar cells: formation of large pores. J. Auton. Pharmacol. 21, 181–190. doi: 10.1046/j.1365-2680.2001.00224.x
Gilman, K. E., Camden, J. M., Klein, R. R., Zhang, Q., Weisman, G. A., and Limesand, K. H. (2019). P2X7 receptor deletion suppresses gamma-radiation-induced hyposalivation. Am. J. Physiol. Regul. Integr. Comp. Physiol. 316, R687–R696. doi: 10.1152/ajpregu.00192.2018
Giuliani, A. L., Sarti, A. C., Falzoni, S., and Di Virgilio, F. (2017). The P2X7 Receptor-Interleukin-1 Liaison. Front. Pharmacol. 8:123. doi: 10.3389/fphar.2017.00123
Granado, M., Amor, S., Montoya, J. J., Monge, L., Fernandez, N., and Garcia-Villalon, A. L. (2015). Altered expression of P2Y2 and P2X7 purinergic receptors in the isolated rat heart mediates ischemia-reperfusion injury. Vascul Pharmacol. 73, 96–103. doi: 10.1016/j.vph.2015.06.003
Grbic, D. M., Degagne, E., Langlois, C., Dupuis, A. A., and Gendron, F. P. (2008). Intestinal inflammation increases the expression of the P2Y6 receptor on epithelial cells and the release of CXC chemokine ligand 8 by UDP. J. Immunol. 180, 2659–2668. doi: 10.4049/jimmunol.180.4.2659
Green, J. P., Souilhol, C., Xanthis, I., Martinez-Campesino, L., Bowden, N. P., Evans, P. C., et al. (2018). Atheroprone flow activates inflammation via endothelial ATP-dependent P2X7-p38 signalling. Cardiovasc. Res. 114, 324–335. doi: 10.1093/cvr/cvx213
Grosche, A., Pannicke, T., Chen, J., Wiedemann, P., Reichenbach, A., and Bringmann, A. (2013). Disruption of endogenous purinergic signaling inhibits vascular endothelial growth factor- and glutamate-induced osmotic volume regulation of Muller glial cells in knockout mice. Ophthalmic Res. 50, 209–214. doi: 10.1159/000354082
Grundmann, O., Mitchell, G. C., and Limesand, K. H. (2009). Sensitivity of salivary glands to radiation: from animal models to therapies. J. Dent. Res. 88, 894–903. doi: 10.1177/0022034509343143
Guo, C., Masin, M., Qureshi, O. S., and Murrell-Lagnado, R. D. (2007). Evidence for functional P2X4/P2X7 heteromeric receptors. Mol. Pharmacol. 72, 1447–1456. doi: 10.1124/mol.107.035980
Habermacher, C., Dunning, K., Chataigneau, T., and Grutter, T. (2016). Molecular structure and function of P2X receptors. Neuropharmacology 104, 18–30. doi: 10.1016/j.neuropharm.2015.07.032
Hazama, A., Hayashi, S., and Okada, Y. (1998). Cell surface measurements of ATP release from single pancreatic beta cells using a novel biosensor technique. Pflugers. Arch. 437, 31–35. doi: 10.1007/s004240050742
Hede, S. E., Amstrup, J., Christoffersen, B. C., and Novak, I. (1999). Purinoceptors evoke different electrophysiological responses in pancreatic ducts. P2Y inhibits K(+) conductance, and P2X stimulates cation conductance. J. Biol. Chem. 274, 31784–31791. doi: 10.1074/jbc.274.45.31784
Hede, S. E., Amstrup, J., Klaerke, D. A., and Novak, I. (2005). P2Y2 and P2Y4 receptors regulate pancreatic Ca(2+)-activated K+ channels differently. Pflugers. Arch. 450, 429–436. doi: 10.1007/s00424-005-1433-3
Helenius, L. M., Meurman, J. H., Helenius, I., Kari, K., Hietanen, J., Suuronen, R., et al. (2005). Oral and salivary parameters in patients with rheumatic diseases. Acta Odontol. Scand. 63, 284–293.
Helmick, C. G., Felson, D. T., Lawrence, R. C., Gabriel, S., Hirsch, R., Kwoh, C. K., et al. (2008). Estimates of the prevalence of arthritis and other rheumatic conditions in the United States. Part I. Arthritis Rheum 58, 15–25. doi: 10.1002/art.23177
Ho, T., Vessey, K. A., and Fletcher, E. L. (2014). Immunolocalization of the P2X4 receptor on neurons and glia in the mammalian retina. Neuroscience 277, 55–71. doi: 10.1016/j.neuroscience.2014.06.055
Hodges, R. R., and Dartt, D. A. (2016). Signaling Pathways of Purinergic Receptors and Their Interactions with Cholinergic and Adrenergic Pathways in the Lacrimal Gland. J. Ocul. Pharmacol. Ther. 32, 490–497. doi: 10.1089/jop.2016.0008
Hodges, R. R., Vrouvlianis, J., Scott, R., and Dartt, D. A. (2011). Identification of P2X(3) and P2X(7) purinergic receptors activated by ATP in rat lacrimal gland. Invest. Ophthalmol. Vis. Sci. 52, 3254–3263. doi: 10.1167/iovs.10-7042
Hodges, R. R., Vrouvlianis, J., Shatos, M. A., and Dartt, D. A. (2009). Characterization of P2X7 purinergic receptors and their function in rat lacrimal gland. Invest. Ophthalmol. Vis. Sci. 50, 5681–5689. doi: 10.1167/iovs.09-3670
Hu, L. P., Zhang, X. X., Jiang, S. H., Tao, L. Y., Li, Q., Zhu, L. L., et al. (2019). Targeting purinergic receptor P2Y2 prevents the growth of pancreatic ductal adenocarcinoma by inhibiting cancer cell glycolysis. Clin. Cancer Res. 25, 1318–1330. doi: 10.1158/1078-0432.CCR-18-2297
Hu, Y. J., Wang, Q., Jiang, Y. T., Ma, R., Xia, W. W., Tang, Z. S., et al. (2013). Characterization of oral bacterial diversity of irradiated patients by high-throughput sequencing. Int. J. Oral. Sci. 5, 21–25. doi: 10.1038/ijos.2013.15
Huang, L., Otrokocsi, L., and Sperlagh, B. (2019). Role of P2 receptors in normal brain development and in neurodevelopmental psychiatric disorders. Brain Res. Bull. 151, 55–64. doi: 10.1016/j.brainresbull.2019.01.030
Huang, P., Zou, Y., Zhong, X. Z., Cao, Q., Zhao, K., Zhu, M. X., et al. (2014). P2X4 forms functional ATP-activated cation channels on lysosomal membranes regulated by luminal pH. J. Biol. Chem. 289, 17658–17667. doi: 10.1074/jbc.M114.552158
Hulkkonen, J., Pertovaara, M., Antonen, J., Pasternack, A., and Hurme, M. (2001). Elevated interleukin-6 plasma levels are regulated by the promoter region polymorphism of the IL6 gene in primary Sjogren’s syndrome and correlate with the clinical manifestations of the disease. Rheumatology 40, 656–661. doi: 10.1093/rheumatology/40.6.656
Humphrey, S. P., and Williamson, R. T. (2001). A review of saliva: normal composition, flow, and function. J. Prosthet. Dent. 85, 162–169. doi: 10.1067/mpr.2001.113778
Hung, S. C., Choi, C. H., Said-Sadier, N., Johnson, L., Atanasova, K. R., Sellami, H., et al. (2013). P2X4 assembles with P2X7 and pannexin-1 in gingival epithelial cells and modulates ATP-induced reactive oxygen species production and inflammasome activation. PLoS One 8:e70210. doi: 10.1371/journal.pone.0070210
Huo, A. P., Lin, K. C., and Chou, C. T. (2010). Predictive and prognostic value of antinuclear antibodies and rheumatoid factor in primary Sjogren’s syndrome. Int. J. Rheum. Dis. 13, 39–47. doi: 10.1111/j.1756-185X.2009.01444.x
Hurley, T. W., Shoemaker, D. D., and Ryan, M. P. (1993). Extracellular ATP prevents the release of stored Ca2+ by autonomic agonists in rat submandibular gland acini. Am. J. Physiol. 265, C1472–C1478.
Hwang, S. M., Koo, N. Y., Choi, S. Y., Chun, G. S., Kim, J. S., and Park, K. (2009a). P2X7 receptor-mediated membrane blebbing in salivary epithelial cells. Korean J. Physiol. Pharmacol. 13, 175–179. doi: 10.4196/kjpp.2009.13.3.175
Hwang, S. M., Li, J., Koo, N. Y., Choi, S. Y., Lee, S. J., Oh, S. B., et al. (2009b). Role of purinergic receptor in alpha fodrin degradation in Par C5 cells. J. Dent. Res. 88, 927–932. doi: 10.1177/0022034509342227
Hwang, T. H., Schwiebert, E. M., and Guggino, W. B. (1996). Apical and basolateral ATP stimulates tracheal epithelial chloride secretion via multiple purinergic receptors. Am. J. Physiol. 270, C1611–C1623.
Ibuka, S., Matsumoto, S., Fujii, S., and Kikuchi, A. (2015). The P2Y(2) receptor promotes Wnt3a- and EGF-induced epithelial tubular formation by IEC6 cells by binding to integrins. J. Cell Sci. 128, 2156–2168. doi: 10.1242/jcs.169060
Idzko, M., Ferrari, D., and Eltzschig, H. K. (2014). Nucleotide signalling during inflammation. Nature 509, 310–317. doi: 10.1038/nature13085
Inoue, K. (2019). Role of the P2X4 receptor in neuropathic pain. Curr. Opin. Pharmacol. 47, 33–39. doi: 10.1016/j.coph.2019.02.001
Ishibashi, K., Okamura, K., and Yamazaki, J. (2008). Involvement of apical P2Y2 receptor-regulated CFTR activity in muscarinic stimulation of Cl(-) reabsorption in rat submandibular gland. Am. J. Physiol. Regul. Integr. Comp. Physiol. 294, R1729–R1736. doi: 10.1152/ajpregu.00758.2007
Jacobson, K. A., and Civan, M. M. (2016). Ocular Purine Receptors as Drug Targets in the Eye. J. Ocul. Pharmacol. Ther. 32, 534–547. doi: 10.1089/jop.2016.0090
Jang, S. I., Ong, H. L., Gallo, A., Liu, X., Illei, G., and Alevizos, I. (2015). Establishment of functional acinar-like cultures from human salivary glands. J. Dent. Res. 94, 304–311. doi: 10.1177/0022034514559251
Jensen, S. B., Pedersen, A. M., Vissink, A., Andersen, E., Brown, C. G., Davies, A. N., et al. (2010). A systematic review of salivary gland hypofunction and xerostomia induced by cancer therapies: prevalence, severity and impact on quality of life. Support Care Cancer 18, 1039–1060. doi: 10.1007/s00520-010-0827-8
Jin, H., Seo, J., Eun, S. Y., Joo, Y. N., Park, S. W., Lee, J. H., et al. (2014). P2Y2 R activation by nucleotides promotes skin wound-healing process. Exp. Dermatol. 23, 480–485. doi: 10.1111/exd.12440
Joo, Y. N., Jin, H., Eun, S. Y., Park, S. W., Chang, K. C., and Kim, H. J. (2014). P2Y2R activation by nucleotides released from the highly metastatic breast cancer cell MDA-MB-231 contributes to pre-metastatic niche formation by mediating lysyl oxidase secretion, collagen crosslinking, and monocyte recruitment. Oncotarget 5, 9322–9334.
Joraku, A., Sullivan, C. A., Yoo, J., and Atala, A. (2007). In-vitro reconstitution of three-dimensional human salivary gland tissue structures. Differentiation 75, 318–324. doi: 10.1111/j.1432-0436.2006.00138.x
Jorgensen, T. D., Gromada, J., Tritsaris, K., Nauntofte, B., and Dissing, S. (1995). Activation of P2z purinoceptors diminishes the muscarinic cholinergic-induced release of inositol 1,4,5-trisphosphate and stored calcium in rat parotid acini. ATP as a co-transmitter in the stimulus-secretion coupling. Biochem. J. 312(Pt 2), 457–464. doi: 10.1042/bj3120457
Kaczmarek-Hajek, K., Zhang, J., Kopp, R., Grosche, A., Rissiek, B., Saul, A., et al. (2018). Re-evaluation of neuronal P2X7 expression using novel mouse models and a P2X7-specific nanobody. eLife 7:36217. doi: 10.7554/eLife.36217
Kamada, Y., Saino, T., Oikawa, M., Kurosaka, D., and Satoh, Y. (2012). P2Y purinoceptors induce changes in intracellular calcium in acinar cells of rat lacrimal glands. Histochem. Cell Biol. 137, 97–106. doi: 10.1007/s00418-011-0885-0
Kawano, A., Tsukimoto, M., Noguchi, T., Hotta, N., Harada, H., Takenouchi, T., et al. (2012). Involvement of P2X4 receptor in P2X7 receptor-dependent cell death of mouse macrophages. Biochem. Biophys. Res. Commun. 419, 374–380. doi: 10.1016/j.bbrc.2012.01.156
Kellerman, D., Evans, R., Mathews, D., and Shaffer, C. (2002). Inhaled P2Y2 receptor agonists as a treatment for patients with Cystic Fibrosis lung disease. Adv. Drug Deliv. Rev 54, 1463–1474. doi: 10.1016/s0169-409x(02)00154-0
Keystone, E. C., Wang, M. M., Layton, M., Hollis, S., Mcinnes, I. B., and Team, D. C. S. (2012). Clinical evaluation of the efficacy of the P2X7 purinergic receptor antagonist AZD9056 on the signs and symptoms of rheumatoid arthritis in patients with active disease despite treatment with methotrexate or sulphasalazine. Ann. Rheum. Dis. 71, 1630–1635. doi: 10.1136/annrheumdis-2011-143578
Khalafalla, F. G., Greene, S., Khan, H., Ilves, K., Monsanto, M. M., Alvarez, R., et al. (2017). P2Y2 nucleotide receptor prompts human cardiac progenitor cell activation by modulating hippo signaling. Circ. Res. 121, 1224–1236. doi: 10.1161/CIRCRESAHA.117.310812
Khalafalla, M. G., Woods, L. T., Camden, J. M., Khan, A. A., Limesand, K. H., Petris, M. J., et al. (2017). P2X7 receptor antagonism prevents IL-1beta release from salivary epithelial cells and reduces inflammation in a mouse model of autoimmune exocrinopathy. J. Biol. Chem. 292, 16626–16637. doi: 10.1074/jbc.M117.790741
Kim, H. D., Bowen, J. W., James-Kracke, M. R., Landon, L. A., Camden, J. M., Burnett, J. E., et al. (1996). Potentiation of regulatory volume decrease by P2U purinoceptors in HSG-PA cells. Am J Physiol 270, C86–C97.
Kishi, T., Takao, T., Fujita, K., and Taniguchi, H. (2006). Clonal proliferation of multipotent stem/progenitor cells in the neonatal and adult salivary glands. Biochem. Biophys. Res. Commun. 340, 544–552. doi: 10.1016/j.bbrc.2005.12.031
Kishore, S. P., Blank, E., Heller, D. J., Patel, A., Peters, A., Price, M., et al. (2018). Modernizing the world health organization list of essential medicines for preventing and controlling cardiovascular diseases. J. Am. Coll. Cardiol. 71, 564–574. doi: 10.1016/j.jacc.2017.11.056
Kobayashi, A., and Konishi, T. (2018). Radiation quality effects alteration in COX-2 pathway to trigger radiation-induced bystander response in A549 lung carcinoma cells. J. Radiat. Res. 59, 754–759. doi: 10.1093/jrr/rry065
Koh, S. (2015). Clinical utility of 3% diquafosol ophthalmic solution in the treatment of dry eyes. Clin. Ophthalmol. 9, 865–872. doi: 10.2147/OPTH.S69486
Kojima, S., Ohshima, Y., Nakatsukasa, H., and Tsukimoto, M. (2017). Role of ATP as a key signaling molecule mediating radiation-induced biological effects. Dose Response 15:1559325817690638. doi: 10.1177/1559325817690638
Kong, Q., Peterson, T. S., Baker, O., Stanley, E., Camden, J., Seye, C. I., et al. (2009). Interleukin-1beta enhances nucleotide-induced and alpha-secretase-dependent amyloid precursor protein processing in rat primary cortical neurons via up-regulation of the P2Y(2) receptor. J. Neurochem. 109, 1300–1310. doi: 10.1111/j.1471-4159.2009.06048.x
Konings, A. W., Coppes, R. P., and Vissink, A. (2005). On the mechanism of salivary gland radiosensitivity. Int. J. Radiat. Oncol. Biol. Phys. 62, 1187–1194. doi: 10.1016/j.ijrobp.2004.12.051
Kopp, R., Krautloher, A., Ramirez-Fernandez, A., and Nicke, A. (2019). P2X7 interactions and signaling - making head or tail of it. Front. Mol. Neurosci. 12:183. doi: 10.3389/fnmol.2019.00183
Lazarowski, E. R., and Boucher, R. C. (2009). Purinergic receptors in airway epithelia. Curr. Opin. Pharmacol. 9, 262–267. doi: 10.1016/j.coph.2009.02.004
Lee, M. G., Ohana, E., Park, H. W., Yang, D., and Muallem, S. (2012). Molecular mechanism of pancreatic and salivary gland fluid and HCO3 secretion. Physiol. Rev. 92, 39–74. doi: 10.1152/physrev.00011.2011
Lee, M. G., Zeng, W., and Muallem, S. (1997). Characterization and localization of P2 receptors in rat submandibular gland acinar and duct cells. J. Biol. Chem. 272, 32951–32955. doi: 10.1074/jbc.272.52.32951
Legate, K. R., Wickstrom, S. A., and Fassler, R. (2009). Genetic and cell biological analysis of integrin outside-in signaling. Genes Dev. 23, 397–418. doi: 10.1101/gad.1758709
Leite, C. A., Galera, M. F., Espinosa, M. M., De Lima, P. R., Fernandes, V., Borges, A. H., et al. (2015). Prevalence of hyposalivation in patients with systemic lupus erythematosus in a brazilian subpopulation. Int. J. Rheumatol. 2015:730285. doi: 10.1155/2015/730285
Leon, C., Freund, M., Latchoumanin, O., Farret, A., Petit, P., Cazenave, J. P., et al. (2005). The P2Y(1) receptor is involved in the maintenance of glucose homeostasis and in insulin secretion in mice. Purinergic Signal. 1, 145–151. doi: 10.1007/s11302-005-6209-x
Lester, S., Stokes, L., Skarratt, K. K., Gu, B. J., Sivils, K. L., Lessard, C. J., et al. (2013). Epistasis with HLA DR3 implicates the P2X7 receptor in the pathogenesis of primary Sjogren’s syndrome. Arthritis Res. Ther. 15:R71. doi: 10.1186/ar4248
Li, Q., Luo, X., Zeng, W., and Muallem, S. (2003). Cell-specific behavior of P2X7 receptors in mouse parotid acinar and duct cells. J. Biol. Chem. 278, 47554–47561. doi: 10.1074/jbc.m308306200
Li, Y., Taylor, J. M., Ten Haken, R. K., and Eisbruch, A. (2007). The impact of dose on parotid salivary recovery in head and neck cancer patients treated with radiation therapy. Int. J. Radiat. Oncol. Biol. Phys. 67, 660–669. doi: 10.1016/j.ijrobp.2006.09.021
Liao, Z., Seye, C. I., Weisman, G. A., and Erb, L. (2007). The P2Y2 nucleotide receptor requires interaction with alpha v integrins to access and activate G12. J. Cell Sci. 120, 1654–1662. doi: 10.1242/jcs.03441
Lisi, S., Sisto, M., Soleti, R., Saponaro, C., Scagliusi, P., D’amore, M., et al. (2007). Fcgamma receptors mediate internalization of anti-Ro and anti-La autoantibodies from Sjogren’s syndrome and apoptosis in human salivary gland cell line A-253. J. Oral Pathol. Med. 36, 511–523. doi: 10.1111/j.1600-0714.2007.00563.x
Lister, M. F., Sharkey, J., Sawatzky, D. A., Hodgkiss, J. P., Davidson, D. J., Rossi, A. G., et al. (2007). The role of the purinergic P2X7 receptor in inflammation. J. Inflamm. 4:5. doi: 10.1186/1476-9255-4-5
Liu, J., Liao, Z., Camden, J., Griffin, K. D., Garrad, R. C., Santiago-Perez, L. I., et al. (2004). Src homology 3 binding sites in the P2Y2 nucleotide receptor interact with Src and regulate activities of Src, proline-rich tyrosine kinase 2, and growth factor receptors. J. Biol. Chem. 279, 8212–8218. doi: 10.1074/jbc.m312230200
Liu, X., Cotrim, A., Teos, L., Zheng, C., Swaim, W., Mitchell, J., et al. (2013). Loss of TRPM2 function protects against irradiation-induced salivary gland dysfunction. Nat. Commun. 4:1515. doi: 10.1038/ncomms2526
Liu, X., Gong, B., De Souza, L. B., Ong, H. L., Subedi, K. P., Cheng, K. T., et al. (2017). Radiation inhibits salivary gland function by promoting STIM1 cleavage by caspase-3 and loss of SOCE through a TRPM2-dependent pathway. Sci. Signal. 10:eaal4064. doi: 10.1126/scisignal.aal4064
Liu, X., Singh, B. B., and Ambudkar, I. S. (1999). ATP-dependent activation of K(Ca) and ROMK-type K(ATP) channels in human submandibular gland ductal cells. J. Biol. Chem. 274, 25121–25129. doi: 10.1074/jbc.274.35.25121
Lombaert, I., Movahednia, M. M., Adine, C., and Ferreira, J. N. (2017). Concise review: salivary gland regeneration: therapeutic approaches from stem cells to tissue organoids. Stem Cells 35, 97–105. doi: 10.1002/stem.2455
Lombaert, I. M., Brunsting, J. F., Wierenga, P. K., Faber, H., Stokman, M. A., Kok, T., et al. (2008). Rescue of salivary gland function after stem cell transplantation in irradiated glands. PLoS One 3:e2063. doi: 10.1371/journal.pone.0002063
Lucattelli, M., Cicko, S., Muller, T., Lommatzsch, M., De Cunto, G., Cardini, S., et al. (2011). P2X7 receptor signaling in the pathogenesis of smoke-induced lung inflammation and emphysema. Am. J. Respir. Cell Mol. Biol. 44, 423–429. doi: 10.1165/rcmb.2010-0038OC
Luo, X., Zheng, W., Yan, M., Lee, M. G., and Muallem, S. (1999). Multiple functional P2X and P2Y receptors in the luminal and basolateral membranes of pancreatic duct cells. Am. J. Physiol. 277, C205–C215. doi: 10.1152/ajpcell.1999.277.2.C205
Lustig, K. D., Shiau, A. K., Brake, A. J., and Julius, D. (1993). Expression cloning of an ATP receptor from mouse neuroblastoma cells. Proc. Natl. Acad. Sci. U.S.A. 90, 5113–5117. doi: 10.1073/pnas.90.11.5113
Lv, Q., Xue, Y., Li, G., Zou, L., Zhang, X., Ying, M., et al. (2015). Beneficial effects of evodiamine on P2X(4)-mediated inflammatory injury of human umbilical vein endothelial cells due to high glucose. Int. Immunopharmacol. 28, 1044–1049. doi: 10.1016/j.intimp.2015.08.020
Ma, T., Song, Y., Gillespie, A., Carlson, E. J., Epstein, C. J., and Verkman, A. S. (1999). Defective secretion of saliva in transgenic mice lacking aquaporin-5 water channels. J. Biol. Chem. 274, 20071–20074. doi: 10.1074/jbc.274.29.20071
Man, Y. G., Ball, W. D., Marchetti, L., and Hand, A. R. (2001). Contributions of intercalated duct cells to the normal parenchyma of submandibular glands of adult rats. Anat. Rec. 263, 202–214. doi: 10.1002/ar.1098
Manoussakis, M. N., Boiu, S., Korkolopoulou, P., Kapsogeorgou, E. K., Kavantzas, N., Ziakas, P., et al. (2007). Rates of infiltration by macrophages and dendritic cells and expression of interleukin-18 and interleukin-12 in the chronic inflammatory lesions of Sjogren’s syndrome: correlation with certain features of immune hyperactivity and factors associated with high risk of lymphoma development. Arthritis Rheum. 56, 3977–3988. doi: 10.1002/art.23073
Maria, O. M., Zeitouni, A., Gologan, O., and Tran, S. D. (2011). Matrigel improves functional properties of primary human salivary gland cells. Tissue Eng. Part A 17, 1229–1238. doi: 10.1089/ten.TEA.2010.0297
Mariette, X., and Criswell, L. A. (2018). Primary Sjogren’s Syndrome. N. Engl. J. Med. 378, 931–939.
Martinez, J. R. (1987). Ion transport and water movement. J. Dent. Res. 66, 638–647. doi: 10.1177/00220345870660s206
Masahiko, M., Sumitomo, S., Shrestha, P., Tanaka, S., Takai, Y., and Shikimori, M. (2008). Multifunctional role of growth factors or biologically active peptides in salivary glands and saliva. Oral Med. Pathol. 12, 115–123. doi: 10.3353/omp.12.115
McCarthy, A. E., Yoshioka, C., and Mansoor, S. E. (2019). Full-Length P2X7 structures reveal how palmitoylation prevents channel desensitization. Cell 179:e613. doi: 10.1016/j.cell.2019.09.017
McMillian, M. K., Soltoff, S. P., Cantley, L. C., Rudel, R., and Talamo, B. R. (1993). Two distinct cytosolic calcium responses to extracellular ATP in rat parotid acinar cells. Br. J. Pharmacol. 108, 453–461. doi: 10.1111/j.1476-5381.1993.tb12825.x
McMillian, M. K., Soltoff, S. P., Cantley, L. C., and Talamo, B. R. (1987). Extracellular ATP elevates intracellular free calcium in rat parotid acinar cells. Biochem. Biophys. Res. Commun. 149, 523–530. doi: 10.1016/0006-291x(87)90399-8
Mehta, V. B., Hart, J., and Wewers, M. D. (2001). ATP-stimulated release of interleukin (IL)-1beta and IL-18 requires priming by lipopolysaccharide and is independent of caspase-1 cleavage. J. Biol. Chem. 276, 3820–3826. doi: 10.1074/jbc.m006814200
Meijer, J. M., Meiners, P. M., Huddleston Slater, J. J., Spijkervet, F. K., Kallenberg, C. G., Vissink, A., et al. (2009). Health-related quality of life, employment and disability in patients with Sjogren’s syndrome. Rheumatology 48, 1077–1082. doi: 10.1093/rheumatology/kep141
Melvin, J. E., Yule, D., Shuttleworth, T., and Begenisich, T. (2005). Regulation of fluid and electrolyte secretion in salivary gland acinar cells. Annu. Rev. Physiol. 67, 445–469. doi: 10.1146/annurev.physiol.67.041703.084745
Metioui, M., Amsallem, H., Alzola, E., Chaib, N., Elyamani, A., Moran, A., et al. (1996). Low affinity purinergic receptor modulates the response of rat submandibular glands to carbachol and substance P. J. Cell. Physiol. 168, 462–475. doi: 10.1002/(sici)1097-4652(199608)168:2<462::aid-jcp25>3.0.co;2-3
Meyer, M. P., Clarke, J. D., Patel, K., Townsend-Nicholson, A., and Burnstock, G. (1999). Selective expression of purinoceptor cP2Y1 suggests a role for nucleotide signalling in development of the chick embryo. Dev. Dyn. 214, 152–158. doi: 10.1002/(sici)1097-0177(199902)214:2<152::aid-aja5>3.0.co;2-l
Meyer, M. P., Swann, K., Burnstock, G., and Clarke, J. D. (2001). The extracellular ATP receptor, cP2Y(1), inhibits cartilage formation in micromass cultures of chick limb mesenchyme. Dev. Dyn. 222, 494–505. doi: 10.1002/dvdy.1196
Miras-Portugal, M. T., Sebastian-Serrano, A., De Diego Garcia, L., and Diaz-Hernandez, M. (2017). Neuronal P2X7 receptor: involvement in neuronal physiology and pathology. J. Neurosci. 37, 7063–7072. doi: 10.1523/JNEUROSCI.3104-16.2017
Mitsias, D. I., Kapsogeorgou, E. K., and Moutsopoulos, H. M. (2006). Sjogren’s syndrome: why autoimmune epithelitis? Oral Dis. 12, 523–532. doi: 10.1111/j.1601-0825.2006.01292.x
Miyazaki, K., Takeda, N., Ishimaru, N., Omotehara, F., Arakaki, R., and Hayashi, Y. (2005). Analysis of in Vivo role of α-fodrin autoantigen in primary sjögren’s syndrome. Am. J. Pathol. 167, 1051–1059. doi: 10.1016/s0002-9440(10)61194-7
Miyazaki, Y., Nakanishi, Y., and Hieda, Y. (2004). Tissue interaction mediated by neuregulin-1 and ErbB receptors regulates epithelial morphogenesis of mouse embryonic submandibular gland. Dev. Dyn. 230, 591–596. doi: 10.1002/dvdy.20078
Mizukoshi, K., Koyama, N., Hayashi, T., Zheng, L., Matsuura, S., and Kashimata, M. (2016). Shh/Ptch and EGF/ErbB cooperatively regulate branching morphogenesis of fetal mouse submandibular glands. Dev. Biol. 412, 278–287. doi: 10.1016/j.ydbio.2016.02.018
Moore, P. A., Guggenheimer, J., Etzel, K. R., Weyant, R. J., and Orchard, T. (2001). Type 1 diabetes mellitus, xerostomia, and salivary flow rates. Oral Surg. Oral Med. Oral Pathol. Oral Radiol. Endod. 92, 281–291. doi: 10.1067/moe.2001.117815
Muccino, D., and Green, S. (2019). Update on the clinical development of gefapixant, a P2X3 receptor antagonist for the treatment of refractory chronic cough. Pulm. Pharmacol. Ther. 56, 75–78. doi: 10.1016/j.pupt.2019.03.006
Muhvic-Urek, M., Bralic, M., Curic, S., Pezelj-Ribaric, S., Borcic, J., and Tomac, J. (2006). Imbalance between apoptosis and proliferation causes late radiation damage of salivary gland in mouse. Physiol. Res. 55, 89–95.
Müller, K., Oxholm, P., Mier-Madsen, M., and Wiik, A. (1989). Circulating IgA- and IgM-rheumatoid factors in patients with primary Sjögren syndrome. Correlation to extraglandular manifestations. Scand. J. Rheumatol. 18, 29–31. doi: 10.3109/03009748909095400
Murakami, T., Fujihara, T., Horibe, Y., and Nakamura, M. (2004). Diquafosol elicits increases in net Cl- transport through P2Y2 receptor stimulation in rabbit conjunctiva. Ophthalmic Res. 36, 89–93. doi: 10.1159/000076887
Murdoch-Kinch, C. A., Kim, H. M., Vineberg, K. A., Ship, J. A., and Eisbruch, A. (2008). Dose-effect relationships for the submandibular salivary glands and implications for their sparing by intensity modulated radiotherapy. Int. J. Radiat. Oncol. Biol. Phys. 72, 373–382. doi: 10.1016/j.ijrobp.2007.12.033
Mutafova-Yambolieva, V. N., and Durnin, L. (2014). The purinergic neurotransmitter revisited: a single substance or multiple players? Pharmacol. Ther. 144, 162–191. doi: 10.1016/j.pharmthera.2014.05.012
Nagler, R. M., Salameh, F., Reznick, A. Z., Livshits, V., and Nahir, A. M. (2003). Salivary gland involvement in rheumatoid arthritis and its relationship to induced oxidative stress. Rheumatology 42, 1234–1241. doi: 10.1093/rheumatology/keg362
Nakamoto, T., Brown, D. A., Catalan, M. A., Gonzalez-Begne, M., Romanenko, V. G., and Melvin, J. E. (2009). Purinergic P2X7 receptors mediate ATP-induced saliva secretion by the mouse submandibular gland. J. Biol. Chem. 284, 4815–4822. doi: 10.1074/jbc.M808597200
Nam, K., Dean, S. M., Brown, C. T., Smith, R. J. Jr., Lei, P., Andreadis, S. T., et al. (2019a). Synergistic effects of laminin-1 peptides, VEGF and FGF9 on salivary gland regeneration. Acta Biomater. 91, 186–194. doi: 10.1016/j.actbio.2019.04.049
Nam, K., Kim, K., Dean, S. M., Brown, C. T., Davis, R. S., Okano, T., et al. (2019b). Using cell sheets to regenerate mouse submandibular glands. NPJ Regen. Med. 4:16. doi: 10.1038/s41536-019-0078-3
Nardi, N., Brito-Zeron, P., Ramos-Casals, M., Aguilo, S., Cervera, R., Ingelmo, M., et al. (2006). Circulating auto-antibodies against nuclear and non-nuclear antigens in primary Sjogren’s syndrome: prevalence and clinical significance in 335 patients. Clin. Rheumatol. 25, 341–346. doi: 10.1007/s10067-005-0059-3
Nezos, A., Gravani, F., Tassidou, A., Kapsogeorgou, E. K., Voulgarelis, M., Koutsilieris, M., et al. (2015). Type I and II interferon signatures in Sjogren’s syndrome pathogenesis: contributions in distinct clinical phenotypes and Sjogren’s related lymphomagenesis. J. Autoimmun. 63, 47–58. doi: 10.1016/j.jaut.2015.07.002
Nguyen, T., Erb, L., Weisman, G. A., Marchese, A., Heng, H. H., Garrad, R. C., et al. (1995). Cloning, expression, and chromosomal localization of the human uridine nucleotide receptor gene. J. Biol. Chem. 270, 30845–30848. doi: 10.1074/jbc.270.52.30845
Nicke, A. (2008). Homotrimeric complexes are the dominant assembly state of native P2X7 subunits. Biochem. Biophys. Res. Commun. 377, 803–808. doi: 10.1016/j.bbrc.2008.10.042
Nishiuchi, R., Takagi, J., Hayashi, M., Ido, H., Yagi, Y., Sanzen, N., et al. (2006). Ligand-binding specificities of laminin-binding integrins: a comprehensive survey of laminin–integrin interactions using recombinant α3β1, α6β1, α7β1 and α6β4 integrins. Matrix Biol. 25, 189–197. doi: 10.1016/j.matbio.2005.12.001
North, R. A. (2002). Molecular physiology of P2X receptors. Physiol. Rev. 82, 1013–1067. doi: 10.1152/physrev.00015.2002
Novak, I. (2003). ATP as a signaling molecule: the exocrine focus. News Physiol. Sci. 18, 12–17. doi: 10.1152/nips.01409.2002
Novak, I. (2008). Purinergic receptors in the endocrine and exocrine pancreas. Purinergic Signal. 4, 237–253. doi: 10.1007/s11302-007-9087-6
Novak, I., Jans, I. M., and Wohlfahrt, L. (2010). Effect of P2X(7) receptor knockout on exocrine secretion of pancreas, salivary glands and lacrimal glands. J. Physiol. 588, 3615–3627. doi: 10.1113/jphysiol.2010.190017
Novak, I., Nitschke, R., and Amstrup, J. (2002). Purinergic receptors have different effects in rat exocrine pancreas. Calcium signals monitored by fura-2 using confocal microscopy. Cell Physiol. Biochem. 12, 83–92. doi: 10.1159/000063784
Nunes, L. A., Mussavira, S., and Bindhu, O. S. (2015). Clinical and diagnostic utility of saliva as a non-invasive diagnostic fluid: a systematic review. Biochem. Med. 25, 177–192. doi: 10.11613/BM.2015.018
Ogawa, M., and Tsuji, T. (2017). “Functional salivary gland regeneration by organ replacement therapy,” in Salivary Gland Development and Regeneration, ed. S. Cha, (Berlin: Springer), 193–203. doi: 10.1007/978-3-319-43513-8_10
Ohshima, Y., Tsukimoto, M., Harada, H., and Kojima, S. (2012). Involvement of connexin43 hemichannel in ATP release after gamma-irradiation. J. Radiat. Res. 53, 551–557. doi: 10.1093/jrr/rrs014
Ohshima, Y., Tsukimoto, M., Takenouchi, T., Harada, H., Suzuki, A., Sato, M., et al. (2010). gamma-Irradiation induces P2X(7) receptor-dependent ATP release from B16 melanoma cells. Biochim. Biophys. Acta 1800, 40–46. doi: 10.1016/j.bbagen.2009.10.008
Ohtomo, K., Shatos, M. A., Vrouvlianis, J., Li, D., Hodges, R. R., and Dartt, D. A. (2011). Increase of intracellular Ca2+ by purinergic receptors in cultured rat lacrimal gland myoepithelial cells. Invest. Ophthalmol. Vis. Sci. 52, 9503–9515. doi: 10.1167/iovs.11-7809
Onodera, T., Sakai, T., Hsu, J. C., Matsumoto, K., Chiorini, J. A., and Yamada, K. M. (2010). Btbd7 regulates epithelial cell dynamics and branching morphogenesis. Science 329, 562–565. doi: 10.1126/science.1191880
Orriss, I., Syberg, S., Wang, N., Robaye, B., Gartland, A., Jorgensen, N., et al. (2011). Bone phenotypes of P2 receptor knockout mice. Front. Biosci. 3:1038–1046. doi: 10.2741/s208
Orriss, I. R., Burnstock, G., and Arnett, T. R. (2010). Purinergic signalling and bone remodelling. Curr. Opin. Pharmacol. 10, 322–330. doi: 10.1016/j.coph.2010.01.003
Osailan, S. M., Proctor, G. B., Carpenter, G. H., Paterson, K. L., and Mcgurk, M. (2006). Recovery of rat submandibular salivary gland function following removal of obstruction: a sialometrical and sialochemical study. Int. J. Exp. Pathol. 87, 411–423. doi: 10.1111/j.1365-2613.2006.00500.x
Ozaki, Y., Ito, T., Son, Y., Amuro, H., Shimamoto, K., Sugimoto, H., et al. (2010). Decrease of blood dendritic cells and increase of tissue-infiltrating dendritic cells are involved in the induction of Sjogren’s syndrome but not in the maintenance. Clin. Exp. Immunol. 159, 315–326. doi: 10.1111/j.1365-2249.2009.04071.x
Pantano, E., Marchi, S., Biagini, M., Di Fede, M., Nardi Dei, V., Rossi Paccani, S., et al. (2019). NHBA is processed by kallikrein from human saliva. PLoS One 14:e0203234. doi: 10.1371/journal.pone.0203234
Park, M. K., Garrad, R. C., Weisman, G. A., and Turner, J. T. (1997). Changes in P2Y1 nucleotide receptor activity during the development of rat salivary glands. Am. J. Physiol. 272, C1388–C1393.
Parr, C. E., Sullivan, D. M., Paradiso, A. M., Lazarowski, E. R., Burch, L. H., Olsen, J. C., et al. (1994). Cloning and expression of a human P2U nucleotide receptor, a target for cystic fibrosis pharmacotherapy. Proc. Natl. Acad. Sci. U.S.A. 91, 3275–3279. doi: 10.1073/pnas.91.8.3275
Patel, V. N., Rebustini, I. T., and Hoffman, M. P. (2006). Salivary gland branching morphogenesis. Differentiation 74, 349–364. doi: 10.1111/j.1432-0436.2006.00088.x
PDQ Supportive and Palliative Care Editorial Board (2002). “Oral Complications of Chemotherapy and Head/Neck Radiation (PDQ(R)): health professional version,” in PDQ Cancer Information Summaries (Bethesda, MD: National Cancer Institute).
Pellegatti, P., Raffaghello, L., Bianchi, G., Piccardi, F., Pistoia, V., and Di Virgilio, F. (2008). Increased level of extracellular ATP at tumor sites: in vivo imaging with plasma membrane luciferase. PLoS One 3:e2599. doi: 10.1371/journal.pone.0002599
Perez-Flores, G., Levesque, S. A., Pacheco, J., Vaca, L., Lacroix, S., Perez-Cornejo, P., et al. (2015). The P2X7/P2X4 interaction shapes the purinergic response in murine macrophages. Biochem. Biophys. Res. Commun. 467, 484–490. doi: 10.1016/j.bbrc.2015.10.025
Peterson, T. S., Thebeau, C. N., Ajit, D., Camden, J. M., Woods, L. T., Wood, W. G., et al. (2013). Up-regulation and activation of the P2Y(2) nucleotide receptor mediate neurite extension in IL-1beta-treated mouse primary cortical neurons. J. Neurochem. 125, 885–896. doi: 10.1111/jnc.12252
Petit, P., Lajoix, A. D., and Gross, R. (2009). P2 purinergic signalling in the pancreatic beta-cell: control of insulin secretion and pharmacology. Eur. J. Pharm. Sci. 37, 67–75. doi: 10.1016/j.ejps.2009.01.007
Pfister, D. G., Spencer, S., Brizel, D. M., Burtness, B., Busse, P. M., Caudell, J. J., et al. (2015). Head and Neck Cancers, Version 1.2015. J. Natl. Compr. Canc. Netw. 13, 847–855.
Piirainen, H., Ashok, Y., Nanekar, R. T., and Jaakola, V. P. (2011). Structural features of adenosine receptors: from crystal to function. Biochim. Biophys. Acta 1808, 1233–1244. doi: 10.1016/j.bbamem.2010.05.021
Pinna, R., Campus, G., Cumbo, E., Mura, I., and Milia, E. (2015). Xerostomia induced by radiotherapy: an overview of the physiopathology, clinical evidence, and management of the oral damage. Ther Clin Risk Manag. 11, 171–188. doi: 10.2147/TCRM.S70652
Pochet, S., Garcia-Marcos, M., Seil, M., Otto, A., Marino, A., and Dehaye, J. P. (2007). Contribution of two ionotropic purinergic receptors to ATP responses in submandibular gland ductal cells. Cell. Signal. 19, 2155–2164. doi: 10.1016/j.cellsig.2007.06.012
Pochet, S., Seil, M., El Ouaaliti, M., and Dehaye, J. P. (2013). [P2X4 or P2X7: which of these two receptors is the best target to promote salivation?]. Med. Sci. 29, 509–514. doi: 10.1051/medsci/2013295014
Pradhan-Bhatt, S., Harrington, D. A., Duncan, R. L., Jia, X., Witt, R. L., and Farach-Carson, M. C. (2013). Implantable three-dimensional salivary spheroid assemblies demonstrate fluid and protein secretory responses to neurotransmitters. Tissue Eng. Part A 19, 1610–1620. doi: 10.1089/ten.TEA.2012.0301
Pringle, S., Maimets, M., Van Der Zwaag, M., Stokman, M. A., Van Gosliga, D., Zwart, E., et al. (2016). Human Salivary Gland Stem Cells Functionally Restore Radiation Damaged Salivary Glands. Stem Cells 34, 640–652. doi: 10.1002/stem.2278
Qadir, M. M. F., Alvarez-Cubela, S., Klein, D., Lanzoni, G., Garcia-Santana, C., Montalvo, A., et al. (2018). P2RY1/ALK3-expressing cells within the adult human exocrine pancreas Are BMP-7 expandable and exhibit progenitor-like characteristics. Cell Rep. 22, 2408–2420. doi: 10.1016/j.celrep.2018.02.006
Qian, S., Hoggatt, A., Jones-Hall, Y. L., Ware, C. F., Herring, P., and Seye, C. I. (2016). Deletion of P2Y2 receptor reveals a role for lymphotoxin-alpha in fatty streak formation. Vascul. Pharmacol. 85, 11–20. doi: 10.1016/j.vph.2016.06.001
Qin, B., Wang, J., Yang, Z., Yang, M., Ma, N., Huang, F., et al. (2015). Epidemiology of primary Sjogren’s syndrome: a systematic review and meta-analysis. Ann. Rheum. Dis. 74, 1983–1989.
Quissell, D. O., Turner, J. T., and Redman, R. S. (1998). Development and characterization of immortalized rat parotid and submandibular acinar cell lines. Eur. J. Morphol. 36, 50–54.
Radfar, L., and Sirois, D. A. (2003). Structural and functional injury in minipig salivary glands following fractionated exposure to 70 Gy of ionizing radiation: an animal model for human radiation-induced salivary gland injury. Oral Surg. Oral Med. Oral Pathol. Oral Radiol. Endod. 96, 267–274. doi: 10.1016/s1079-2104(03)00369-x
Ramos-Casals, M., Nardi, N., Brito-Zeron, P., Aguilo, S., Gil, V., Delgado, G., et al. (2006). Atypical autoantibodies in patients with primary Sjogren syndrome: clinical characteristics and follow-up of 82 cases. Semin. Arthritis Rheum. 35, 312–321. doi: 10.1016/j.semarthrit.2005.12.004
Ramos-Casals, M., Tzioufas, A. G., Stone, J. H., Siso, A., and Bosch, X. (2010). Treatment of primary Sjogren syndrome: a systematic review. JAMA 304, 452–460. doi: 10.1001/jama.2010.1014
Ratchford, A. M., Baker, O. J., Camden, J. M., Rikka, S., Petris, M. J., Seye, C. I., et al. (2010). P2Y2 nucleotide receptors mediate metalloprotease-dependent phosphorylation of epidermal growth factor receptor and ErbB3 in human salivary gland cells. J. Biol. Chem. 285, 7545–7555. doi: 10.1074/jbc.M109.078170
Rennert, L., Zschiedrich, S., Sandner, L., Hartleben, B., Cicko, S., Ayata, C. K., et al. (2018). P2Y2R signaling is involved in the onset of glomerulonephritis. Front. Immunol. 9:1589. doi: 10.3389/fimmu.2018.01589
Reyes, J. P., Perez-Cornejo, P., Hernandez-Carballo, C. Y., Srivastava, A., Romanenko, V. G., Gonzalez-Begne, M., et al. (2008). Na+ modulates anion permeation and block of P2X7 receptors from mouse parotid glands. J. Membr. Biol. 223, 73–85. doi: 10.1007/s00232-008-9115-7
Ricciotti, E., and Fitzgerald, G. A. (2011). Prostaglandins and inflammation. Arterioscler. Thromb. Vasc. Biol. 31, 986–1000. doi: 10.1161/ATVBAHA.110.207449
Robson, S. C., Sevigny, J., and Zimmermann, H. (2006). The E-NTPDase family of ectonucleotidases: structure function relationships and pathophysiological significance. Purinergic Signal. 2, 409–430. doi: 10.1007/s11302-006-9003-5
Sakai, A., Sugawara, Y., Kuroishi, T., Sasano, T., and Sugawara, S. (2008). Identification of IL-18 and Th17 cells in salivary glands of patients with Sjogren’s syndrome, and amplification of IL-17-mediated secretion of inflammatory cytokines from salivary gland cells by IL-18. J. Immunol. 181, 2898–2906. doi: 10.4049/jimmunol.181.4.2898
Sakai, T., Larsen, M., and Yamada, K. M. (2003). Fibronectin requirement in branching morphogenesis. Nature 423, 876–881. doi: 10.1038/nature01712
Sakaki, H., Fujiwaki, T., Tsukimoto, M., Kawano, A., Harada, H., and Kojima, S. (2013). P2X4 receptor regulates P2X7 receptor-dependent IL-1beta and IL-18 release in mouse bone marrow-derived dendritic cells. Biochem. Biophys. Res. Commun. 432, 406–411. doi: 10.1016/j.bbrc.2013.01.135
Savio, L. E. B., De Andrade Mello, P., Da Silva, C. G., and Coutinho-Silva, R. (2018). The P2X7 receptor in inflammatory diseases: angel or demon? Front. Pharmacol. 9:52. doi: 10.3389/fphar.2018.00052
Schneider, M., Prudic, K., Pippel, A., Klapperstuck, M., Braam, U., Muller, C. E., et al. (2017). Interaction of purinergic P2X4 and P2X7 receptor subunits. Front. Pharmacol. 8:860. doi: 10.3389/fphar.2017.00860
Schrader, A. M., Camden, J. M., and Weisman, G. A. (2005). P2Y2 nucleotide receptor up-regulation in submandibular gland cells from the NOD.B10 mouse model of Sjogren’s syndrome. Arch. Oral. Biol. 50, 533–540. doi: 10.1016/j.archoralbio.2004.11.005
Scott, J., Liu, P., and Smith, P. M. (1999). Morphological and functional characteristics of acinar atrophy and recovery in the duct-ligated parotid gland of the rat. J. Dent. Res. 78, 1711–1719. doi: 10.1177/00220345990780110801
Sellers, L. A., Simon, J., Lundahl, T. S., Cousens, D. J., Humphrey, P. P., and Barnard, E. A. (2001). Adenosine nucleotides acting at the human P2Y1 receptor stimulate mitogen-activated protein kinases and induce apoptosis. J. Biol. Chem. 276, 16379–16390. doi: 10.1074/jbc.m006617200
Seye, C. I., Agca, Y., Agca, C., and Derbigny, W. (2012). P2Y2 receptor-mediated lymphotoxin-alpha secretion regulates intercellular cell adhesion molecule-1 expression in vascular smooth muscle cells. J. Biol. Chem. 287, 10535–10543. doi: 10.1074/jbc.M111.313189
Seye, C. I., Gadeau, A. P., Daret, D., Dupuch, F., Alzieu, P., Capron, L., et al. (1997). Overexpression of P2Y2 purinoceptor in intimal lesions of the rat aorta. Arterioscler. Thromb. Vasc. Biol. 17, 3602–3610. doi: 10.1161/01.atv.17.12.3602
Seye, C. I., Kong, Q., Erb, L., Garrad, R. C., Krugh, B., Wang, M., et al. (2002). Functional P2Y2 nucleotide receptors mediate uridine 5’-triphosphate-induced intimal hyperplasia in collared rabbit carotid arteries. Circulation 106, 2720–2726. doi: 10.1161/01.cir.0000038111.00518.35
Seye, C. I., Yu, N., Gonzalez, F. A., Erb, L., and Weisman, G. A. (2004). The P2Y2 nucleotide receptor mediates vascular cell adhesion molecule-1 expression through interaction with VEGF receptor-2 (KDR/Flk-1). J. Biol. Chem. 279, 35679–35686. doi: 10.1074/jbc.m401799200
Seye, C. I., Yu, N., Jain, R., Kong, Q., Minor, T., Newton, J., et al. (2003). The P2Y2 nucleotide receptor mediates UTP-induced vascular cell adhesion molecule-1 expression in coronary artery endothelial cells. J. Biol. Chem. 278, 24960–24965. doi: 10.1074/jbc.m301439200
Shen, J., Seye, C. I., Wang, M., Weisman, G. A., Wilden, P. A., and Sturek, M. (2004). Cloning, up-regulation, and mitogenic role of porcine P2Y2 receptor in coronary artery smooth muscle cells. Mol. Pharmacol. 66, 1265–1274. doi: 10.1124/mol.104.002642
Shen, L., Kapsogeorgou, E. K., Yu, M., Suresh, L., Malyavantham, K., Tzioufas, A. G., et al. (2014). Evaluation of salivary gland protein 1 antibodies in patients with primary and secondary Sjogren’s syndrome. Clin. Immunol. 155, 42–46. doi: 10.1016/j.clim.2014.08.009
Shen, L., Suresh, L., Malyavantham, K., Kowal, P., Xuan, J., Lindemann, M. J., et al. (2013). Different stages of primary Sjögren’s syndrome involving lymphotoxin and type 1 IFN. J. Immunol. 191, 608–613. doi: 10.4049/jimmunol.1203440
Shen, L., Suresh, L., Wu, J., Xuan, J., Li, H., Zhang, C., et al. (2010). A role for lymphotoxin in primary Sjögren’s disease. J. Immunol. 185, 6355–6363.
Shiboski, C. H., Shiboski, S. C., Seror, R., Criswell, L. A., Labetoulle, M., Lietman, T. M., et al. (2017). 2016 American College of Rheumatology/European League Against Rheumatism classification criteria for primary Sjogren’s syndrome: a consensus and data-driven methodology involving three international patient cohorts. Ann. Rheum. Dis. 76, 9–16. doi: 10.1136/annrheumdis-2016-210571
Shieh, C. H., Heinrich, A., Serchov, T., Van Calker, D., and Biber, K. (2014). P2X7-dependent, but differentially regulated release of IL-6, CCL2, and TNF-alpha in cultured mouse microglia. Glia 62, 592–607. doi: 10.1002/glia.22628
Shin, Y. H., Kim, M., Kim, N., Choi, S. K., Namkoong, E., Choi, S. Y., et al. (2015). Epigenetic alteration of the purinergic type 7 receptor in salivary epithelial cells. Biochem. Biophys. Res. Commun. 466, 704–710. doi: 10.1016/j.bbrc.2015.09.095
Shishikura, Y., Koarai, A., Aizawa, H., Yamaya, M., Sugiura, H., Watanabe, M., et al. (2016). Extracellular ATP is involved in dsRNA-induced MUC5AC production via P2Y2R in human airway epithelium. Respir. Res. 17:121.
Siddiqui, F., and Movsas, B. (2017). Management of Radiation Toxicity in Head and Neck Cancers. Semin. Radiat. Oncol. 27, 340–349. doi: 10.1016/j.semradonc.2017.04.008
Sisto, M., Lisi, S., Castellana, D., Scagliusi, P., D’amore, M., Caprio, S., et al. (2006). Autoantibodies from Sjögren’s syndrome induce activation of both the intrinsic and extrinsic apoptotic pathways in human salivary gland cell line A-253. J. Autoimmun. 27, 38–49. doi: 10.1016/j.jaut.2006.05.001
Solini, A., Chiozzi, P., Morelli, A., Fellin, R., and Di Virgilio, F. (1999). Human primary fibroblasts in vitro express a purinergic P2X7 receptor coupled to ion fluxes, microvesicle formation and IL-6 release. J. Cell Sci. 112(Pt 3), 297–305.
Soltoff, S. P., McMillian, M. K., Lechleiter, J. D., Cantley, L. C., and Talamo, B. R. (1990). Elevation of [Ca2+]i and the activation of ion channels and fluxes by extracellular ATP and phospholipase C-linked agonists in rat parotid acinar cells. Ann. N. Y. Acad. Sci. 603, 76–90. doi: 10.1111/j.1749-6632.1990.tb37663.x
Sorensen, C. E., and Novak, I. (2001). Visualization of ATP release in pancreatic acini in response to cholinergic stimulus. Use of fluorescent probes and confocal microscopy. J. Biol. Chem. 276, 32925–32932. doi: 10.1074/jbc.m103313200
Stokes, L., Spencer, S. J., and Jenkins, T. A. (2015). Understanding the role of P2X7 in affective disorders-are glial cells the major players? Front. Cell Neurosci. 9:258. doi: 10.3389/fncel.2015.00258
Suadicani, S. O., Brosnan, C. F., and Scemes, E. (2006). P2X7 receptors mediate ATP release and amplification of astrocytic intercellular Ca2+ signaling. J. Neurosci. 26, 1378–1385. doi: 10.1523/jneurosci.3902-05.2006
Suresh, L., Malyavantham, K., Shen, L., and Ambrus, J. L. Jr. (2015). Investigation of novel autoantibodies in Sjogren’s syndrome utilizing Sera from the Sjogren’s international collaborative clinical alliance cohort. BMC Ophthalmol. 15:38. doi: 10.1186/s12886-015-0023-1
Surprenant, A., Rassendren, F., Kawashima, E., North, R. A., and Buell, G. (1996). The cytolytic P2Z receptor for extracellular ATP identified as a P2X receptor (P2X7). Science 272, 735–738. doi: 10.1126/science.272.5262.735
Suurvali, J., Boudinot, P., Kanellopoulos, J., and Ruutel Boudinot, S. (2017). P2X4: a fast and sensitive purinergic receptor. Biomed. J. 40, 245–256. doi: 10.1016/j.bj.2017.06.010
Tackett, B. C., Sun, H., Mei, Y., Maynard, J. P., Cheruvu, S., Mani, A., et al. (2014). P2Y2 purinergic receptor activation is essential for efficient hepatocyte proliferation in response to partial hepatectomy. Am. J. Physiol. Gastrointest. Liver Physiol. 307, G1073–G1087. doi: 10.1152/ajpgi.00092.2014
Takagi, K., Yamaguchi, K., Sakurai, T., Asari, T., Hashimoto, K., and Terakawa, S. (2003). Secretion of saliva in X-irradiated rat submandibular glands. Radiat. Res. 159, 351–360. doi: 10.1667/0033-7587(2003)159[0351:sosixi]2.0.co;2
Takahashi, S., Gobe, G. C., Yoshimura, Y., Kohgo, T., Yamamoto, T., and Wakita, M. (2007). Participation of the Fas and Fas ligand systems in apoptosis during atrophy of the rat submandibular glands. Int. J. Exp. Pathol. 88, 9–17. doi: 10.1111/j.1365-2613.2006.00511.x
Takahashi, S., Nakamura, S., Domon, T., Yamamoto, T., and Wakita, M. (2005). Active participation of apoptosis and mitosis in sublingual gland regeneration of the rat following release from duct ligation. J. Mol. Histol. 36, 199–205. doi: 10.1007/s10735-005-1764-6
Takahashi, S., Schoch, E., and Walker, N. I. (1998). Origin of acinar cell regeneration after atrophy of the rat parotid induced by duct obstruction. Int. J. Exp. Pathol. 79, 293–301. doi: 10.1046/j.1365-2613.1998.710405.x
Takahashi, S., Shinzato, K., Nakamura, S., Domon, T., Yamamoto, T., and Wakita, M. (2004). Cell death and cell proliferation in the regeneration of atrophied rat submandibular glands after duct ligation. J. Oral Pathol. Med. 33, 23–29. doi: 10.1111/j.1600-0714.2004.00191.x
Takamura, E., Tsubota, K., Watanabe, H., and Ohashi, Y., and Diquafosol Ophthalmic Solution Phase 3 Study Group, (2012). A randomised, double-masked comparison study of diquafosol versus sodium hyaluronate ophthalmic solutions in dry eye patients. Br. J. Ophthalmol. 96, 1310–1315. doi: 10.1136/bjophthalmol-2011-301448
Tanaka, J., Ogawa, M., Hojo, H., Kawashima, Y., Mabuchi, Y., Hata, K., et al. (2018). Generation of orthotopically functional salivary gland from embryonic stem cells. Nat. Commun. 9:4216. doi: 10.1038/s41467-018-06469-7
Tanimura, A., Nezu, A., Tojyo, Y., and Matsumoto, Y. (1999). Isoproterenol potentiates alpha-adrenergic and muscarinic receptor-mediated Ca2+ response in rat parotid cells. Am. J. Physiol. 276, C1282–C1287. doi: 10.1152/ajpcell.1999.276.6.C1282
Tanioka, H., Kuriki, Y., Sakamoto, A., Katsuta, O., Kawazu, K., and Nakamura, M. (2014). Expression of the P2Y(2) receptor on the rat ocular surface during a 1-year rearing period. Jpn. J. Ophthalmol. 58, 515–521. doi: 10.1007/s10384-014-0342-4
Tauber, J., Davitt, W. F., Bokosky, J. E., Nichols, K. K., Yerxa, B. R., Schaberg, A. E., et al. (2004). Double-masked, placebo-controlled safety and efficacy trial of diquafosol tetrasodium (INS365) ophthalmic solution for the treatment of dry eye. Cornea 23, 784–792. doi: 10.1097/01.ico.0000133993.14768.a9
Taylor, S. R., Turner, C. M., Elliott, J. I., Mcdaid, J., Hewitt, R., Smith, J., et al. (2009). P2X7 deficiency attenuates renal injury in experimental glomerulonephritis. J. Am. Soc. Nephrol. 20, 1275–1281. doi: 10.1681/ASN.2008060559
Teos, L. Y., Zhang, Y., Cotrim, A. P., Swaim, W., Won, J. H., Ambrus, J., et al. (2015). IP3R deficit underlies loss of salivary fluid secretion in Sjögren’s Syndrome. Sci. Rep. 5:13953.
Teymoortash, A., Simolka, N., Schrader, C., Tiemann, M., and Werner, J. A. (2005). Lymphocyte subsets in irradiation-induced sialadenitis of the submandibular gland. Histopathology 47, 493–500. doi: 10.1111/j.1365-2559.2005.02256.x
Topol, E. J., and Schork, N. J. (2011). Catapulting clopidogrel pharmacogenomics forward. Nat. Med. 17, 40–41. doi: 10.1038/nm0111-40
Tsukimoto, M. (2015). Purinergic signaling is a novel mechanism of the cellular response to ionizing radiation. Biol. Pharm. Bull. 38, 951–959. doi: 10.1248/bpb.b15-00062
Tsukimoto, M., Homma, T., Ohshima, Y., and Kojima, S. (2010). Involvement of purinergic signaling in cellular response to gamma radiation. Radiat. Res. 173, 298–309. doi: 10.1667/RR1732.1
Tsunawaki, S., Nakamura, S., Ohyama, Y., Sasaki, M., Ikebe-Hiroki, A., Hiraki, A., et al. (2002). Possible function of salivary gland epithelial cells as nonprofessional antigen-presenting cells in the development of Sjögren’s syndrome. J. Rheumatol. 29, 1884–1896.
Tuckwell, D., and Humphries, M. (1996). “Integrin–collagen binding,” in Seminars in Cell & Developmental Biology, ed. J., Davey, (Amsterdem: Elsevier), 649–657. doi: 10.1006/scdb.1996.0079
Turner, C. M., Tam, F. W., Lai, P. C., Tarzi, R. M., Burnstock, G., Pusey, C. D., et al. (2007). Increased expression of the pro-apoptotic ATP-sensitive P2X7 receptor in experimental and human glomerulonephritis. Nephrol. Dial. Transplant. 22, 386–395. doi: 10.1093/ndt/gfl589
Turner, J. T., Landon, L. A., Gibbons, S. J., and Talamo, B. R. (1999). Salivary gland P2 nucleotide receptors. Crit. Rev. Oral Biol. Med. 10, 210–224. doi: 10.1177/10454411990100020701
Turner, J. T., Redman, R. S., Camden, J. M., Landon, L. A., and Quissell, D. O. (1998a). A rat parotid gland cell line, Par-C10, exhibits neurotransmitter-regulated transepithelial anion secretion. Am. J. Physiol. 275, C367–C374. doi: 10.1152/ajpcell.1998.275.2.C367
Turner, J. T., Weisman, G. A., Landon, L. A., Park, M., and Camden, J. M. (1998b). Salivary gland nucleotide receptors: evidence for functional expression of both P2X and P2Y subtypes. Eur. J. Morphol. 36, 170–175.
Turner, J. T., Weisman, G. A., and Camden, J. M. (1997). Upregulation of P2Y2 nucleotide receptors in rat salivary gland cells during short-term culture. Am. J. Physiol. 273, C1100–C1107.
van Woerkom, J. M., Kruize, A. A., Wenting-Van Wijk, M. J., Knol, E., Bihari, I. C., Jacobs, J. W., et al. (2005). Salivary gland and peripheral blood T helper 1 and 2 cell activity in Sjogren’s syndrome compared with non-Sjogren’s sicca syndrome. Ann. Rheum. Dis. 64, 1474–1479. doi: 10.1136/ard.2004.031781
Verkhratsky, A., and Burnstock, G. (2014). Biology of purinergic signalling: its ancient evolutionary roots, its omnipresence and its multiple functional significance. Bioessays 36, 697–705. doi: 10.1002/bies.201400024
Vivino, F. B. (2017). Sjogren’s syndrome: clinical aspects. Clin. Immunol. 182, 48–54. doi: 10.1016/j.clim.2017.04.005
von Kugelgen, I. (2019). Pharmacology of P2Y receptors. Brain Res. Bull. 151, 12–24. doi: 10.1016/j.brainresbull.2019.03.010
von Kugelgen, I., and Hoffmann, K. (2016). Pharmacology and structure of P2Y receptors. Neuropharmacology 104, 50–61. doi: 10.1016/j.neuropharm.2015.10.030
von Kugelgen, I., Kurz, K., Bultmann, R., Driessen, B., and Starke, K. (1994). Presynaptic modulation of the release of the co-transmitters noradrenaline and ATP. Fundam. Clin. Pharmacol. 8, 207–213. doi: 10.1111/j.1472-8206.1994.tb00800.x
von Kugelgen, I., and Wetter, A. (2000). Molecular pharmacology of P2Y-receptors. Naunyn Schmiedebergs Arch. Pharmacol. 362, 310–323. doi: 10.1007/s002100000310
Wang, M., Kong, Q., Gonzalez, F. A., Sun, G., Erb, L., Seye, C., et al. (2005). P2Y2 nucleotide receptor interaction with αv integrin mediates astrocyte migration. J. Neurochem. 95, 630–640. doi: 10.1111/j.1471-4159.2005.03408.x
Watanabe, T., Tsuchida, T., Kanda, N., Mori, K., Hayashi, Y., and Tamaki, K. (1999). Anti-alpha-fodrin antibodies in Sjogren syndrome and lupus erythematosus. Arch. Dermatol. 135, 535–539.
Webb, T. E., Simon, J., Krishek, B. J., Bateson, A. N., Smart, T. G., King, B. F., et al. (1993). Cloning and functional expression of a brain G-protein-coupled ATP receptor. FEBS Lett. 324, 219–225. doi: 10.1016/0014-5793(93)81397-i
Wei, C., Larsen, M., Hoffman, M. P., and Yamada, K. M. (2007). Self-organization and branching morphogenesis of primary salivary epithelial cells. Tissue Eng 13, 721–735. doi: 10.1089/ten.2006.0123
Weigand, L. A., Ford, A. P., and Undem, B. J. (2012). A role for ATP in bronchoconstriction-induced activation of guinea pig vagal intrapulmonary C-fibres. J. Physiol. 590, 4109–4120. doi: 10.1113/jphysiol.2012.233460
Weisman, G. A., De, B. K., Friedberg, I., Pritchard, R. S., and Heppel, L. A. (1984). Cellular responses to external ATP which precede an increase in nucleotide permeability in transformed cells. J. Cell. Physiol. 119, 211–219. doi: 10.1002/jcp.1041190211
Weisman, G. A., De, B. K., and Pritchard, R. S. (1989). Ionic dependence of the extracellular ATP-induced permeabilization of transformed mouse fibroblasts: role of plasma membrane activities that regulate cell volume. J. Cell. Physiol. 138, 375–383. doi: 10.1002/jcp.1041380221
Weisman, G. A., Garrad, R. C., Erb, L. J., Otero, M., Gonzalez, F. A., and Clarke, L. L. (1998). Structure and function of P2Y2 nucleotide receptors in cystic fibrosis (CF) epithelium. Adv. Exp. Med. Biol. 431, 417–424. doi: 10.1007/978-1-4615-5381-6_82
Weng, P. L., Aure, M. H., Maruyama, T., and Ovitt, C. E. (2018). Limited regeneration of adult salivary glands after severe injury involves cellular plasticity. Cell Rep. 24:e1463. doi: 10.1016/j.celrep.2018.07.016
Willeke, P., Schotte, H., Schluter, B., Erren, M., Becker, H., Dyong, A., et al. (2003). Interleukin 1beta and tumour necrosis factor alpha secreting cells are increased in the peripheral blood of patients with primary Sjogren’s syndrome. Ann. Rheum. Dis. 62, 359–362.
Wong, R. S., Madapallimattam, G., and Bennick, A. (1983). The role of glandular kallikrein in the formation of a salivary proline-rich protein A by cleavage of a single bond in salivary protein C. Biochem. J. 211, 35–44. doi: 10.1042/bj2110035
Wong, W. Y., Pier, M., and Limesand, K. H. (2018). Persistent disruption of lateral junctional complexes and actin cytoskeleton in parotid salivary glands following radiation treatment. Am. J. Physiol. Regul. Integr. Comp. Physiol. 315, R656–R667. doi: 10.1152/ajpregu.00388.2017
Woods, L. T., Camden, J. M., Batek, J. M., Petris, M. J., Erb, L., and Weisman, G. A. (2012). P2X7 receptor activation induces inflammatory responses in salivary gland epithelium. Am. J. Physiol. Cell Physiol. 303, C790–C801. doi: 10.1152/ajpcell.00072.2012
Woods, L. T., Camden, J. M., El-Sayed, F. G., Khalafalla, M. G., Petris, M. J., Erb, L., et al. (2015). Increased Expression of TGF-beta signaling components in a mouse model of fibrosis induced by submandibular gland duct ligation. PLoS One 10:e0123641. doi: 10.1371/journal.pone.0123641
Woods, L. T., Camden, J. M., Khalafalla, M. G., Petris, M. J., Erb, L., Ambrus, J. L., et al. (2018). P2Y2 R deletion ameliorates sialadenitis in IL-14alpha-transgenic mice. Oral Dis. 24, 761–771. doi: 10.1111/odi.12823
Wu, L., Oshima, T., Fukui, H., Watari, J., and Miwa, H. (2017). Adenosine triphosphate induces P2Y2 activation and interleukin-8 release in human esophageal epithelial cells. J. Gastroenterol. Hepatol. 32, 1341–1347. doi: 10.1111/jgh.13672
Yang, C., Su, L., Wang, Y., and Liu, L. (2009). UTP regulation of ion transport in alveolar epithelial cells involves distinct mechanisms. Am. J. Physiol. Lung. Cell Mol. Physiol. 297, L439–L454. doi: 10.1152/ajplung.90268.2008
Yegutkin, G. G., Samburski, S. S., Jalkanen, S., and Novak, I. (2006). ATP-consuming and ATP-generating enzymes secreted by pancreas. J. Biol. Chem. 281, 29441–29447. doi: 10.1074/jbc.m602480200
Yoshimura, K., and Hiramatsu, Y. (1998). Characteristics of amylase secretion induced by various secretagogues examined in perifused rat parotid acinar cells. Eur. J. Morphol. 36, 198–202.
Yu, H. X., and Turner, J. T. (1991). Functional studies in the human submandibular duct cell line, HSG-PA, suggest a second salivary gland receptor subtype for nucleotides. J. Pharmacol. Exp. Ther. 259, 1344–1350.
Yu, N., Erb, L., Shivaji, R., Weisman, G. A., and Seye, C. I. (2008). Binding of the P2Y2 nucleotide receptor to filamin A regulates migration of vascular smooth muscle cells. Circ. Res. 102, 581–588. doi: 10.1161/CIRCRESAHA.107.162271
Zalewska, A., Waszkiewicz, N., Szajda, S. D., and Waszkiel, D. (2011). Impact of salivary flow and lysozyme content and output on the oral health of rheumatoid arthritis patients. Postepy Hig. Med. Dosw. 65, 40–45. doi: 10.5604/17322693.932232
Zeng, D., Yao, P., and Zhao, H. (2019). P2X7, a critical regulator and potential target for bone and joint diseases. J. Cell. Physiol. 234, 2095–2103. doi: 10.1002/jcp.27544
Zeng, W., Lee, M. G., and Muallem, S. (1997). Membrane-specific regulation of Cl- channels by purinergic receptors in rat submandibular gland acinar and duct cells. J. Biol. Chem. 272, 32956–32965. doi: 10.1074/jbc.272.52.32956
Zerr, M., Hechler, B., Freund, M., Magnenat, S., Lanois, I., Cazenave, J. P., et al. (2011). Major contribution of the P2Y(1)receptor in purinergic regulation of TNFalpha-induced vascular inflammation. Circulation 123, 2404–2413. doi: 10.1161/CIRCULATIONAHA.110.002139
Zhang, X., and Li, G. (2019). P2Y receptors in neuropathic pain. Pharmacol. Biochem. Behav. 5:172788. doi: 10.1016/j.pbb.2019.172788
Zhao, J., Kubo, S., Nakayamada, S., Shimajiri, S., Zhang, X., Yamaoka, K., et al. (2016). Association of plasmacytoid dendritic cells with B cell infiltration in minor salivary glands in patients with Sjogren’s syndrome. Mod. Rheumatol. 26, 716–724. doi: 10.3109/14397595.2015.1129694
Zhou, H., Ivanov, V. N., Gillespie, J., Geard, C. R., Amundson, S. A., Brenner, D. J., et al. (2005). Mechanism of radiation-induced bystander effect: role of the cyclooxygenase-2 signaling pathway. Proc. Natl. Acad. Sci. U.S.A. 102, 14641–14646. doi: 10.1073/pnas.0505473102
Zimmermann, H., Zebisch, M., and Strater, N. (2012). Cellular function and molecular structure of ecto-nucleotidases. Purinergic Signal. 8, 437–502. doi: 10.1007/s11302-012-9309-4
Keywords: purinergic receptors, saliva, salivary gland dysfunction, Sjögren’s syndrome, extracellular nucleotides, head and neck cancer
Citation: Khalafalla MG, Woods LT, Jasmer KJ, Forti KM, Camden JM, Jensen JL, Limesand KH, Galtung HK and Weisman GA (2020) P2 Receptors as Therapeutic Targets in the Salivary Gland: From Physiology to Dysfunction. Front. Pharmacol. 11:222. doi: 10.3389/fphar.2020.00222
Received: 25 November 2019; Accepted: 18 February 2020;
Published: 13 March 2020.
Edited by:
Rosa Gomez-Villafuertes, Complutense University of Madrid, SpainReviewed by:
Ivana Novak, University of Copenhagen, DenmarkCopyright © 2020 Khalafalla, Woods, Jasmer, Forti, Camden, Jensen, Limesand, Galtung and Weisman. This is an open-access article distributed under the terms of the Creative Commons Attribution License (CC BY). The use, distribution or reproduction in other forums is permitted, provided the original author(s) and the copyright owner(s) are credited and that the original publication in this journal is cited, in accordance with accepted academic practice. No use, distribution or reproduction is permitted which does not comply with these terms.
*Correspondence: Gary A. Weisman, d2Vpc21hbmdAbWlzc291cmkuZWR1
Disclaimer: All claims expressed in this article are solely those of the authors and do not necessarily represent those of their affiliated organizations, or those of the publisher, the editors and the reviewers. Any product that may be evaluated in this article or claim that may be made by its manufacturer is not guaranteed or endorsed by the publisher.
Research integrity at Frontiers
Learn more about the work of our research integrity team to safeguard the quality of each article we publish.