- 1College of Pharmaceutical Science, Zhejiang Chinese Medical University, Hangzhou, China
- 2Affiliated Secondary Hospital, Zhejiang Chinese Medical University, Hangzhou, China
Herbal medicine is a major part of traditional Chinese medicine (TCM), which is evolved as a system of medical practice from ancient China. The use of herbal medicine is mainly based on practice and theories and concepts rooted in ancient philosophy. In the era of evidence-based medicine, it is essential to accurately evaluate herbal remedy with standard/modern medical practice approaches. Tetradium ruticarpum (A. Juss.) Hartley (TR), a medicinal plant with diversify bioactive components, has been broadly used to treat pain and gastrointestinal disorders in TCM. However, TR has also been reported to have potential toxicity by long-term use or excessive doses, though the associated compounds are yet to be identified. TR is usually processed, and/or combined with other herbs in TCM formulas in order to achieve a synergistic effect or reduce its toxicity. Since processing or polyherbal formulation of TR may lead to changes in its chemical composition and contents, quality, efficacy and toxicity, comparison of TR samples before and after processing, as well as its combination with other medicines, would provide useful knowledge of bioactive compounds, efficacy and toxicity of this valuable medicinal plant. Here we reviewed the recent studies about the phytochemistry, pharmacokinetic behaviors and toxicity of TR under various processing or polyherbal formulation conditions, which would expand our understanding of mechanisms of TR’s efficacy and toxicity and be valuable for quality control in industrial manufacturing, future medicinal research, and safety and rational use of TR in TCM.
Introduction
Traditional Chinese medicine (TCM) has been practiced in China for more than 2,500 years and herbal medicine (HM) is an indispensable part of it. HMs are plant tissues characterized by complex compositions with scant evidence on their efficacy, mode of action, and adverse reactions and the usage of HMs is mainly based on experience. In TCM practice, raw plants usually processed or prescribed with other herbs to achieve better medical efficacy (Cai et al., 2019; Jiang et al., 2019; Tian Q. et al., 2019). Therefore, accurate evaluation of the efficacy, toxicity, and rational use of HM with modern chemical and clinical approaches is critical for the development of TCM in the current era of evidence-based medicine.
Processing is a traditional method of herbal preparations. All raw medicinal plants need to be cut, cleaned, steamed, or fried with other adjuvants before being used in TCM (Cai, 2008). Meanwhile, polyherbal formulation, or namely compatibility, is a common TCM clinical practice, where poly-herbs combined to have synergistic or balanced effects (Ekor, 2014; Ji et al., 2019; Shi et al., 2019; Wu et al., 2019). Processing or compatibility may lead to altered quality, efficacy, or safety of HM (Zhou et al., 2017). Hence, analyzing the changes of HMs before and after processing and compatibility is important for their proper and safety use. Tetradium ruticarpum (A. Juss.) Hartley (known hereafter as “TR”), namely Evodiae Fructus or Wu zhu yu in Chinese, is a small shrub native to temperate and tropical regions of Asia (Editorial Committee of Flora of China, 2008) and its dried nearly-ripe fruit is a frequently used HM (Figure 1). TR is used to treat headaches, abdominal colic, epigastric distension, dysentery, dysmenorrhea, and post-partum hemorrhage (Commission of Chinese Pharmacopeia, 2015), but it may have minor toxicity on excessive usage including liver toxicity in rodents, as well as vision disorders and hair loss in humans (Cai et al., 2006; Cai et al., 2014; Yin et al., 2015). Since various processing and polyherbal formulations of TR are being used clinically, TR may thus serve as a potential representative to study the chemical knowledge base of efficacy and toxicity via processing and compatibility approach on HMs.
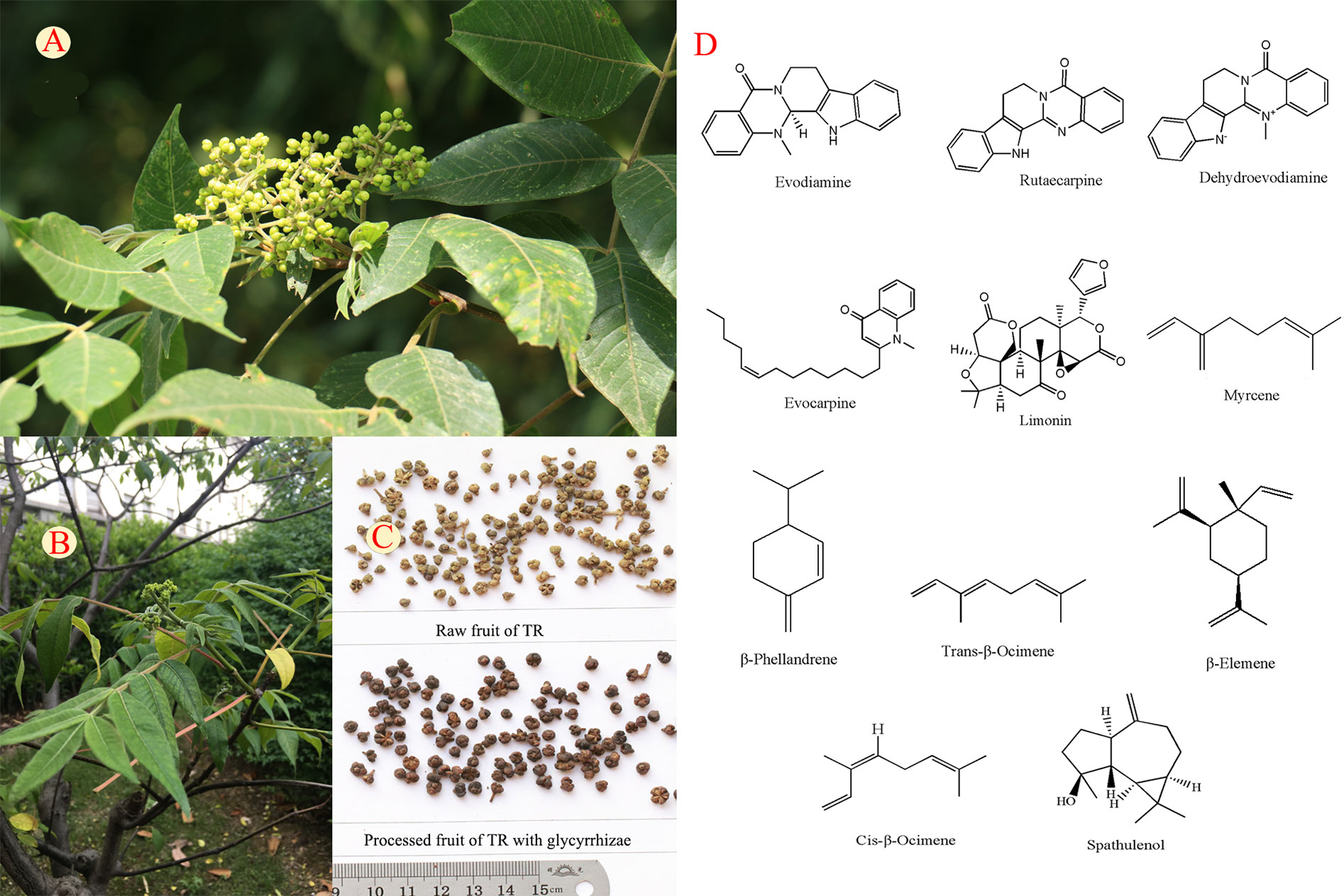
Figure 1 The fruit (A), whole plant (B), crude and processed products (C), and structures of typical components (D) of Tetradium ruticarpum (A. Juss.) Hartley.
Pharmacokinetics, which are usually accompanied with processing and combination of HMs, can provide clues on the modification of therapeutic effects of medicinal herbs. Moreover, the phytochemistry and toxicity of processed TR and its compatibility with other HMs can facilitate the identification of the relationships between compounds, efficacy, and toxicity. In this review, recent studies about the phytochemistry, pharmacokinetics, and toxicity of TR are summarized and discussed.
Processing and Polyherbal Formulations
Processing and polyherbal formulation are the common practices in TCM. Accompanied with physical and/or chemical reactions, these procedures are believed to improve effects, reduce toxicity, or obtain synergistic or balanced effects. Several processing and compatibility have been reported in the use of TR. According to the 2015 Edition of Chinese Pharmacopoeia, stir-frying of TR with licorice water extract is the “standard” processing method, other methods include washing with hot or cold water as well as “stir-baking” with ginger juice, vinegar, salt, rice wine, black soybean water extract, or Coptidis Rhizoma water extract have been reported in ancient medical books and local practices. In the following sections, treatment with licorice, salt, etc. means stir-frying of TR with those compounds. Different processing methods may change the effects of TR (Xiao et al., 2017; Pei, 2018). In addition, TR is prescribed clinically with other herbs for treating various diseases, such as with Angelica sinensis Radix for blood deficiency, as well as mixed with Pinellia Rhizome and Coptidis Rhizoma for persistent vomiting. The composition and therapeutic effects of typical polyherbal formulations and TR preparations are summarized in Supplementary Table S1.
Phytochemistry
In TCM practice, HM’s property is the basis of clinical diagnosis and treatment. As the fruit of TR is pungent and bitter, processing and compatibility will decrease its pungent aroma and bitter taste. The pungent smelling may derive from volatile oils, while the bitter taste may come from limonoids. Thus, TR’s property is closely related with its phytochemistry, which are connected with its processing or combined using application.
More than 100 compounds have been isolated or identified from the fruits of TR. Alkaloids, limonoids, volatile oils, carboxylic acids, and flavonoids are the major compounds and their typical structures are shown in Figure 1. Alkaloids, limonoids and some essential oils have been shown to account for anti-tumor, anti-inflammation, analgesic, and antimicrobial activities of TR (Wang X. et al., 2013; Yang, X. et al., 2013; Gavaraskar et al., 2015). The quantification changes of these components are shown in Table 1 and the supporting identification evidences are provided in Supplementary Figure S1. The impacts of processing and polyherbal prescribing of TR on the major ingredients are summarized in the following sections.
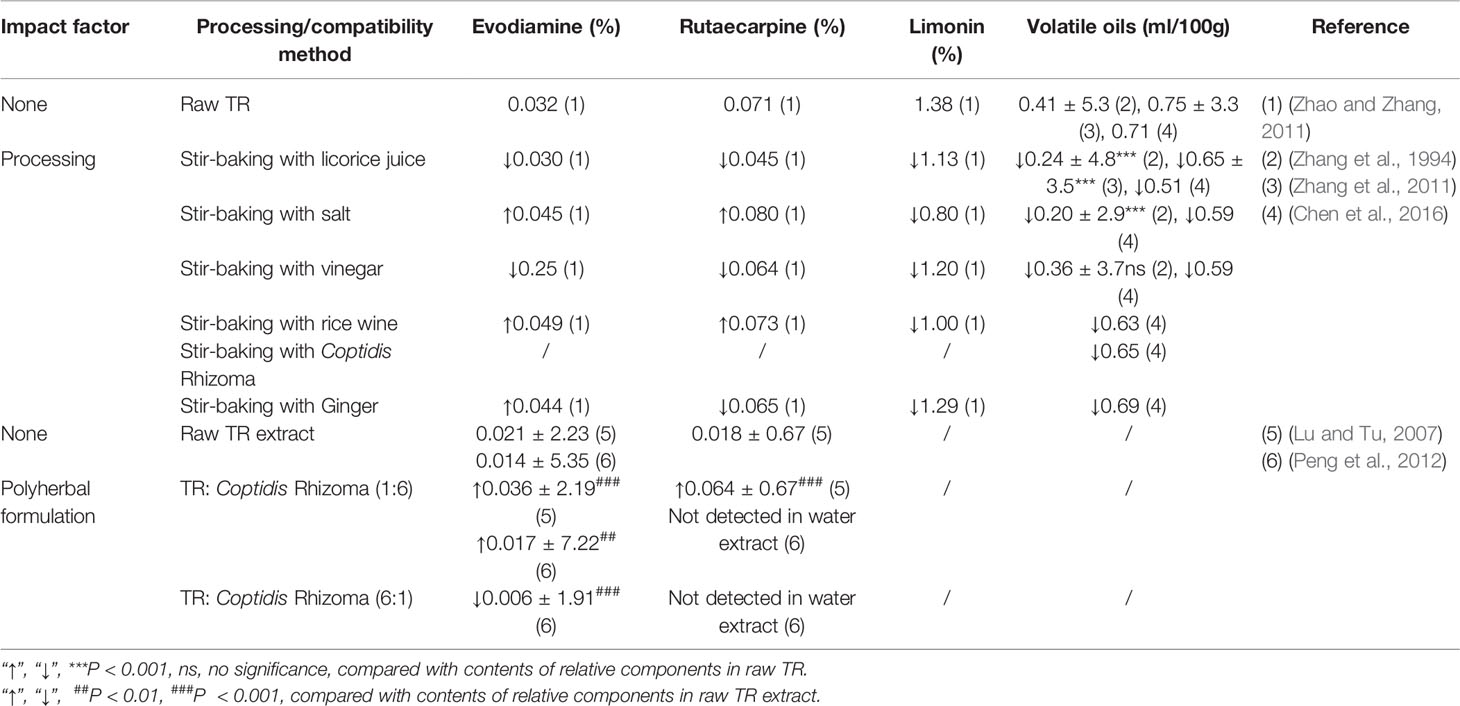
Table 1 Contents of evodiamine, rutaecarpine, limonin, and volatile oils in Tetradium ruticarpum before and after processing or polyherbal formulation.
Alkaloids
Alkaloids in TR are mainly composed of indole-type and quinolone-type ones. Evodiamine, rutaecarpine, and dehydroevodiamine are indole-type alkaloids, while evocarpine has the basic structure of quinolones. Some TR alkaloids have anti-inflammatory, anti-nociceptive, antibacterial, and antimalarial activities (Wang and Liang, 2004). For instance, evodiamine has been demonstrated to have multiple anti-tumor activities, while rutaecarpine offers protection against cardiovascular diseases (Yang J. et al., 2013; Yang F. et al., 2017; Tian KM. et al., 2019).
Alkaloid content varies depending on where TR was been harvested, as well as the processing procedures employed. Stir-baking with salt and wine will increase the concentrations of most of the alkaloids, while stir-baking with licorice or vinegar decrease the main alkaloids. Evodiamine and rutaecarpine could be used as two examples to study the effects of stir-baking processing on the content of alkaloids. These two compounds usually achieve higher quantity if stir-baking using rice wine or salt. When processing with ginger, the content of evodiamine increases while rutaecarpine declines (Zhao and Zhang, 2011; Zhang et al., 2019).
Alkaloid contents of TR was also affected by polyherbal formulations. The compatibility of Coptidis Rhizoma and TR has been evaluated. With a TR: Coptidis Rhizoma ratio of 1:6 in a formulation, the solubility of the main alkaloids from TR increases (and even enjoys better solubility than a TR-only decoction) while the contents of main components in Coptidis Rhizoma decreases (Lu and Tu, 2007; Liu et al., 2010; Peng et al., 2012; Wang L. et al., 2013).
Limonoids
Limonoids are characterized by the basic structure of furanolactones (Figure 1). Since the typical structure consists of four six-membered rings and a furan ring, limonoids are classified as tetranortriterpene compounds. Limonin is a typical limonoid ingredient in TR, which has been reported to have antibacterial, antiviral, anti-tumor, and anti-obesity activities (Ono et al., 2011; Yang X. et al., 2013; Tundis et al., 2014; Zhao et al., 2019). In addition, limonin counteracts the analgesic effects of TR (Xu et al., 2019). Limonin content generally decreases after processing (Zhao and Zhang, 2011; Ma et al., 2016).
Volatile Oils
The major volatile components in TR are terpenoids and their derivatives such as olefins, aromatic, and heterocyclic aromatic compounds. Volatile oils from TR exert analgesic, anti-inflammatory, and antibacterial effects (Huang and Sun, 2012). Most types of processing or compatible procedures can decrease volatile oil contents. A recent study showed that the volatile compounds in TR before and after processing by licorice was 0.75 and 0.65%, respectively (Zhang et al., 2011). Processing with ginger can lead to retention of most essential oils, whereas processing with vinegar and salt result in substantial loss of them (Zhang et al., 1994; Chen et al., 2016). Processing can also change the components and proportions of volatile oils. Specifically, levels of myrcene, β-phellandrene, and trans-ocimene are increased, whereas those of β-elemene and cis-ocimene are decreased, after processing using licorice (Zhang et al., 2011). TR prepared with Coptidis Rhizoma leads to reductions in the contents of β-phellandrene and myrcene, whereas the spathulenol level increases (Lu et al., 2003; Chen et al., 2016).
Pharmacokinetics
Beside the chemical part explanation, the alternation of pharmacokinetic properties may be the other elucidation of TR’s changing efficacy or toxicity after processing or compatibility.
Pharmacokinetic Parameters
Pharmacokinetic studies have mainly focused on alkaloids of TR. The concentration-time curves of evodiamine or rutaecarpine have been fitted to a one-compartment model (Luan et al., 2006; Shyr et al., 2006; Wu et al., 2007). The maximum plasma concentration (Cmax), half-life (t1/2), and area under the curve (AUC) for evodiamine at 500 mg/kg given orally to rats have been reported to be 49 ± 19 ng/ml, 138 ± 16.9 min, and 4.18 ± 0.74 min·μg/ml, respectively. The oral bioavailability is ~0.1% in free-moving rats (Shyr et al., 2006). The Cmax of rutaecarpine has been reported to be 2.4 ± 3.0 ng/ml after oral administration of 40 mg/kg to rats for 18 days. Levels of rutaecarpine and evodiamine detected in vivo from TR extracts are much higher (22.8 ± 4.4 and 78.8 ± 23.5 ng/ml, respectively) than that of rutaecarpine alone and evodiamine alone given to rats at similar concentrations (Ding et al., 2008; Xu et al., 2012).
Processing with licorice can prolong the elimination time of alkaloid compounds in rats. The t1/2 of evodiamine and rutaecarpine in processed TR given at 0.4 g/kg has been reported to be 146.57 ± 38.38 and 167.10 ± 15.82 min, respectively (Bao et al., 2011). Both in vitro and in vivo studies revealed that Coptidis Rhizoma combined with TR leads to reduction in exposure to alkaloids from Coptidis Rhizoma. In the in vitro experiments with monolayers of Caco-2 cells under and semi-physiologic conditions, the dissolution of eight components from TR increased with the increasing proportions of Coptidis Rhizoma, whereas the dissolution of main alkaloids from TR increased along with the components of Coptidis Rhizoma decreased (Deng, 2008; Yang Y. et al., 2017). Pretreatment with TR in rats suggests that its mechanism of action involves induction of hepatic expression of uridine 5′-diphospho-glucuronosyltransferase (UGT)1A1 (Ma et al., 2011; Yan et al., 2011). Mechanism involved may be the increasing efflux of the main bioactive alkaloids of Coptidis Rhizoma when prescribed with TR. Since few reports have focused on the processing factors on the pharmacokinetic parameters of TR, its relationship with changes in efficacy and toxicity is yet to be explored.
Distribution
In vivo studies revealed that TR processed with licorice exhibits different distribution behavior when compared with the raw TR, for the evodiamine concentration increased dramatically compared with that of the pre-processed product (Zhao, 2011). Compared with administration of Coptidis Rhizoma alone, combined administration with TR led to greater distribution of main alkaloids of Coptidis Rhizoma in the liver and less in the lungs (Liang et al., 2016). Although the findings are interesting, the mechanism of the distribution still needs further exploration.
Metabolism
TR extracts may inhibit erythromycin activity in human liver microsomes and regulate N-demethylation levels under the presence of nicotinamide adenine dinucleotide phosphate (NADPH) via cytochrome P450 3A4 (CYP3A4). Rutaecarpine and limonin are the main causes of decline in residual CYP3A4 activity. Iwata et al. (2005) reported that the half-maximal inhibitory concentration (IC50) of rutaecarpine was > 100 and 1.4 μM before and 20 min after preincubation, respectively, and the IC50 of limonin was 23.5 and 1.8 μM, respectively. A competitive inhibitor of CYP3A4, ketoconazole, can attenuate inhibitory activity of limonin. CYP3A4 is one type of CYP isoform present not only in the liver, but also in the small intestine of humans. Therefore, the fruit of TR could induce drug interactions via CYP3A4 in the small intestine, thereby demonstrating a potential for alteration of efficacy before and after processing. Besides, according to studies using rat liver microsomes, hepatic UGT1A1 is believed to induce decreased exposure to the alkaloids of Coptidis Rhizoma, with the activity and expression of UGT1A1 being identified after TR pretreatment for 2 weeks (Ma et al., 2011). This action could explain why the dissolution of Coptidis Rhizoma declined after combination with TR. In another study, rutaecarpine was given consecutively to mice for 7 days (10, 20, and 30 mg/kg, p.o.). The transcription expressions of CYP450 genes Cyp1a2, 2b10, 2e1, 3a11, and 4a10 were induced, as were the genes encoding hepatic transporters and phase-2 enzymes, which could provide clues of herb-drug interactions (Zhu et al., 2013).
Pharmacology
TR is a high-frequently prescribed medicinal plant in oriental history for anti-nociceptive and gastrointestinal disorders (Yu et al., 2000; Liu et al., 2003; Yu et al., 2006), anti-cancer (Pan et al., 2012; Park et al., 2018), anti-Alzheimer's disease (Park et al., 1996; Wang et al., 2001), anti-obesity (Rebhun et al., 2015; Nie et al., 2016), cardiovascular effects (Seya et al., 2016), and anti-thrombotic effect (Sheu et al., 2000). However, pharmacological studies of TR are still in its infant stage. So far, only a few studies considered the effects of TR before and after processing (Supplementary Table S2). Salt and vinegar stir-baking products of TR demonstrated better analgesic activities, while licorice product show better anti-inflammatory effect (Deng and Li, 1999). Moreover, some limitations exist in early pharmacological studies, as results commonly presented in the format of original data without calculating the relative inhibition of these pharmacological activities. Furthermore, statistic analysis were mainly conducted by student’s t-test, which may not be suitable for comparisons of more than two groups. In addition, negative controls (TR before processing or polyherbal formulation) were missing in many earlier studies. Symmetrical and critical pharmacological evaluation of TR are necessary in future studies.
Toxicity
Although HMs have long been used in history, the toxicological information and toxicity assessment of these herbal products is quite scare (Ekor, 2014). Similarly, the toxic components, mode of toxicity, prevention, and diagnose method of TR’s potential toxicity is still to be extensively investigated.
Toxicity of TR’s product before and after processing has only been reported in several studies. Volatile oils from raw and processed TR were given to mice for acute-toxicity comparisons. The median lethal dose (LD50) of essential oils from raw TR was 2.82 [95% confidence interval (CI) 2.48–3.21] ml/kg and the LD50 of processed group was 2.91 (95% CI is 2.49–3.41) ml/kg; the volatile oil content decreased by 13.33% whereas LD50 increased by 19.15% after processing (Zhang et al., 2011). The toxic damage in rats caused by 35 days of administration of volatile oils of TR recovered essentially after 20 days (Yin et al., 2015). In vitro and in vivo toxicity experiments have also been conducted for raw, licorice-, and salt-processed TR. The processing procedure reduced the toxicity in mice and the L02 cell model, but salt processing proved to be much safer (Zhang et al., 2018). In another study, the hepatotoxicity of TR was time- and dose-correlated with increases in levels of alanine transaminase, aspartate transaminase, lactate dehydrogenase, alkaline phosphatase, and the liver index (Zhang et al., 2017; Zhang et al., 2018).
TR extracts were also compared for their toxicity. The most tolerance dosages of whole, water and ethanol extracts of TR have been reported to be 15.6, 80, and 70.6 g·kg/day, respectively; the water extract and volatile oil from TR had analgesic activity and the effect was dose-dependent, with the safety scope of the water extract being larger than that of the volatile oil (Huang and Sun, 2012). Hepatocyte swelling, cytoplasmic-sparing neodymium, nuclear swelling, focal, or unicellular necrosis were observed in a few areas, accompanied by infiltration of inflammatory cells (Zhou et al., 2011). Genetic toxicity was not observed in the ethanol extract of TR in the micronucleus assay of bone-marrow cells in mice, whereas rutaecarpine and limonin showed some genotoxicity in vitro for the Ames test and CHL chromosome aberration assay (Xia et al., 2014).
Possible toxic components were speculated by several researchers. Li et al. (2017) claimed that a 50% ethanol extract was responsible for the hepatotoxicity of TR. Identification of this fraction demonstrated that nine indole alkaloids, 10 quinolone alkaloids, five triterpenoids, three flavonoids, one other alkaloid, and one organic acid were present, whereas 21 components absorbed in blood were detected. However, a study with L02 cells resulted in completely different conclusion (Wang et al., 2017).They postulated that isomers of caffeoyl gluconic acid could be the hepatotoxic substance. There is still plenty of work to be done toward TR’s toxicity, to find out the exact toxic ingredients, to clarify the toxic mechanisms, to support the quality standard for industrial manufacturing, and to facilitate rational and safety use.
Conclusions and Future Perspectives
Herbal remedies has played, and will continue to play, an irreplaceable role in the TCM development. Processing and polyherbal formulation of HMs are common TCM clinical practices, which usually accompanied with changings of quality, efficacy, and toxicity. TR is widely used in East Asia for its analgesic, anti-inflammatory, and gastrointestinal protective effect, with various processing and compatibility practices. TR is also proved to have minor toxicity. The phytochemistry, pharmacokinetics, efficacy, and toxicity of TR have been studied under various processing or combination conditions. All the recent studies are valuable for proper processing, novel lead compound design and rational use of TR in TCM, as well as study its effective and toxic mechanisms.
Nevertheless, some knowledge gaps are yet to be filled. First, the chemical investigation in vitro and in vivo is the knowledge base for pharmacological and toxicological study, by processing or multi-herb combination, changing composition, content, or pharmacokinetic properties of these components from TR are highly connected with its efficacy or toxicity alterations. Besides, bioactive/toxic screening and separation may provide a better view on its effective or toxic ingredients, thus bringing scientific support and improvement of the current quality standard of TR and its related products, which will also bring a new insight of bioactive lead compound design. Second, the impact of processing or compatibility of TR on the toxicity evaluation and toxic mechanism is not well understood. Whether TR’s toxicity attributes to its toxic constituents, toxic metabolites, or the toxic syndromes will only observed in allergic patients, should be fully investigated. Third, safety assessments concerned with HMs in recent times are highly needed, how to prevent or diagnostic their potential toxicity is becoming more vital. Biomarker investigation technology is now emerging as a powerful tool, which may indicate the toxicity of TR at different time courses are possible solutions for safety assessment, and may serve as guarantees for prevention, diagnosis, and treatment of potential toxic HM clinical application.
Author Contributions
Q-YSha, X-NS and HH collated documents and wrote the manuscript. L-PQ and GC contributed significantly to outline and revise the manuscript. Q-YZ helped with the phytochemistry section and manuscript revision. K-HL provided photograph of crude and processed TR. Q-YSho and H-YF helped with the consulting of pharmacokinetic and toxicity sections, respectively. MH helped with summarizing the table on compatibility application.
Funding
This study was supported by the National Natural Science Foundation of China (81703707); Traditional Chinese Medicine Research Project, Zhejiang Province of China (2019ZA038); China Scholarship Council (201908330362).
Conflict of Interest
The authors declare that the research was conducted in the absence of any commercial or financial relationships that could be construed as a potential conflict of interest.
Supplementary material
The Supplementary Material for this article can be found online at: https://www.frontiersin.org/articles/10.3389/fphar.2020.00133/full#supplementary-material
References
Bao, T. D., Li, Y. J., Weng, X. G., Yang, Q., Zhang, Y. F., Dong, Y., et al. (2011). Studies on pharmacokinetics of evodiamine and rutaecarpine in rats plasma after oral administration extracts of Euodiae Fructus. China J. Chin Mater Med. 36, 3519–3522. doi: 10.4268/cjcmm20112429
Cai, Q., Wei, J., Zhao, W., Shi, S., Zhang, Y., Wei, R., et al. (2014). Toxicity of Evodiae fructus on rat liver mitochondria: the role of oxidative stress and mitochondrial permeability transition. Molecules 19, 21168–21182. doi: 10.3390/molecules191221168
Cai, H., Xu, Y., Xie, L., Duan, Y., Zhou, J., Liu, J., et al. (2019). Investigation on spectrum-effect correlation between constituents absorbed into blood and bioactivities of Baizhu Shaoyao San before and after processing on ulcerative colitis rats by UHPLC/Q-TOF-MS/MS coupled with gray correlation analysis. Molecules 24, 940–965. doi: 10.3390/molecules24050940
Cai, X. Y., Meng, N., Yang, B. (2006). Analysis of one case of excessive intoxication caused by evodia officinalis. Beijing J. Tradit. Chin. Med. 25, 171–172. doi: 10.3969/j.issn.1674-1307.2006.03.024
Chen, J., Gao, Y., Tan, P., Wang, L., Zhang, X. L., Zhang, Y. F., et al. (2016). Analysis on volatile oil constituents in euodiae fructus and processed products by GC-MS. Chin. J. Inf. TCM. 23, 91–95. doi: 10.3969/j.issn.1005-5304.2016.12.022
Commission of Chinese Pharmacopeia (2015). Pharmacopeia of the People's Republic of China (2015 dition) Part I, 2015 ed. (People’s Medical Publishing House).
Deng, X. Y., Li, Q. (1999). Effect of different process on pharmacological actions of Fructus Evodiae. Chin Trad. Patent Med. 21, 236–238.
Deng, Y. T. (2008). The changes of dissolution and pharmacokinetics of the main components of coptis-evodia herb couple. 32. (Shenyang: Shenyang Univ. Pharm).
Ding, J. S., Gao, R., Li, D., Peng, J., Ran, L. L., Li, Y. J. (2008). Solid dispersion of rutaecarpine improved its antihypertensive effect in spontaneously hypertensive rats. Biopharm. Drug Dispos. 29, 495–500. doi: 10.1002/bdd.634
Ekor, M. (2014). The growing use of herbal medicines: issues relating to adverse reactions and challenges in monitoring safety. Front. Pharmacol. 4, 177. doi: 10.3389/fphar.2013.00177
Gavaraskar, K., Dhulap, S., Hirwani, R. R. (2015). Therapeutic and cosmetic applications of Evodiamine and its derivatives–A patent review. Fitoterapia 106, 22–35. doi: 10.1016/j.fitote.2015.07.019
Huang, W., Sun, R. (2012). Study on analgestic effect and safety scope of different components of Evodia Fructus. Chin J. Pharmacovigilance. 9, 397–400. doi: 10.3969/j.issn.1672-8629.2012.07.004
Iwata, H., Tezuka, Y., Kadota, S., Hiratsuka, A., Watabe, T. (2005). Mechanism-based inactivation of human liver microsomal CYP3A4 by rutaecarpine and limonin from Evodia fruit extract. Drug Metab. Pharmacokinet. 20, 34–45. doi: 10.2133/dmpk.20.34
Ji, S., He, D. D., Su, Z. Y., Du, Y., Wang, Y. J., Gao, S. K., et al. (2019). P450 enzymes-based metabolic interactions between monarch drugs and the other constituent herbs: a strategy to explore compatibility mechanism of Sangju-Yin. Phytomedicine 58, 152866. doi: 10.1016/j.phymed.2019.152866
Jiang, H., Yang, L., Xing, X., Yan, M., Guo, X., Yang, B., et al. (2019). Chemometrics coupled with UPLC-MS/MS for simultaneous analysis of markers in the raw and processed Fructus Xanthii, and application to optimization of processing method by BBD design. Phytomedicine 57, 191–202. doi: 10.1016/j.phymed.2018.12.020
Lee, S. K., Lee, J., Lee, E.-S., Jahng, Y., Kim, D.-H., Jeong, T. C. (2004). Characterization of in vitro metabolites of rutaecarpine in rat liver microsomes using liquid chromatography/tandem mass spectrometry. Rapid Commun. Mass Spectromet. 18, 1073–1080. doi: 10.1002/rcm.1448
Li, W. L., Sun, X. M., Chen, C., Liu, Y., Song, H., Ding, X. X., et al. (2017). Analysis of Evodia rutaecarpa hepatotoxic part and absorbed components in rat serum based on UPLC-TOF-MS. J. Chin. Mass. Spectro. Soc 38, 282–293. doi: 10.7538/zpxb.youxian.2016.0065
Liang, R. F., Zhang, F., Li, G. S., Shi, L. F. (2016). Effect of Euodiae Fructus on tissue distribution of active components from Coptidis Rhizoma in rats. Chin J. Exp. Trad. Med. Formulae. 22, 89–93. doi: 10.3390/molecules22081264
Liu, B. L., Dai, Y. Y., Tang, N., Zhang, F. L., Wang, C. (2003). Anti-inflammatory activity of chloroform extract of Evodia rutaecarpa on experimental colitis in mice. Pharmacol. Clin. Chin Mater Med. 19, 16–19. doi: 10.3969/j.issn.1001-859X.2003.06.009
Liu, F. J., Sun, D. M., Lu, J. H., Xu, A. L. (2010). The content of alkaloid of coptis chinensis was determined by TLC scanning method. Chin. Mat. Med. 32, 75–79. doi: 10.3969/j.issn.1001-1528.2010.01.022
Lu, J. H., Tu, Y. S. (2007). HPLC determination of content of evodiamine and rutaecarpine before and after combining application of Coptidis Rhizoma and Euodiae Fructus. Chin. Trad. Patent Med. 29, 311–312. doi: 10.3969/j.issn.1001-1528.2007.02.058
Lu, J. H., Ning, D. S., Tu, Y. S., Jiang, B., Zeng, Y. E. (2003). Assay of volatile oil from herb couple of Rhizoma Coptidis and Fructus Evodiae by GC/MS. J. Guangzhou Univ. TCM. 20, 312–315. doi: 10.3969/j.issn.1007-3213.2003.04.017
Luan, L. J., Qiu, G. L., Cheng, Y. Y. (2006). Study on pharmacokinetics of evodiamine and rutaecarpine in rabbit. Chin Pharm. J. 41, 48–50. doi: 10.3321/j.issn.1001-2494.2006.01.015
Ma, B. L., Yao, M. K., Han, X. H., Ma, Y. M., Wu, J. S., Wang, C. H. (2011). Influences of Fructus evodiae pretreatment on the pharmacokinetics of Rhizoma coptidis alkaloids. J. Ethnopharmacol. 137, 1395–1401. doi: 10.1016/j.jep.2011.08.002
Ma, Q. Q., Xu, W. Q., Zhang, X. Y. (2016). Effects of different processing methods on fat-soluble constituents of Evodia Fructus. Chem. Manage. 101. doi: 10.3969/j.issn.1008-4800.2016.35.075
Nie, X. Q., Chen, H. H., Zhang, J. Y., Zhang, Y. J., Yang, J. W., Pan, H. J., et al. (2016). Rutaecarpine ameliorates hyperlipidemia and hyperglycemia in fat-fed, streptozotocin-treated rats via regulating the IRS-1/PI3K/Akt and AMPK/ACC2 signaling pathways. Acta Pharmacol. Sin. 37, 483–496. doi: 10.1038/aps.2015.167
Ono, E., Inoue, J., Hashidume, T., Shimizu, M., Sato, R. (2011). Anti-obesity and anti-hyperglycemic effects of the dietary citrus limonoid nomilin in mice fed a high-fat diet. Biochem. Biophys. Res. Commun. 410, 677–681. doi: 10.1016/j.bbrc.2011.06.055
Pan, X. B., Hartley, J. M., Hartley, J. A., White, K. N., Wang, Z. T., Bligh, S. W. A. (2012). Evodiamine, a dual catalytic inhibitor of type I and II topoisomerases, exhibits enhanced inhibition against camptothecin resistant cells. Phytomedicine 19, 618–624. doi: 10.1016/j.phymed.2012.02.003
Park, C. H., Kim, S. H., Choi, W., Lee, Y. J., Kim, J. S., Kang, S. S., et al. (1996). Novel anticholinesterase and antiamnesic activities of dehydroevodiamine, a constituent of Evodia rutaecarpa. Planta Med. 62, 405–409. doi: 10.1055/s-2006-957926
Park, E., Lee, M. Y., Seo, C. S., Jang, J. H., Kim, Y. U., Shin, H. K. (2018). Ethanol Extract of Evodia rutaecarpa Attenuates Cell Growth through Caspase-Dependent Apoptosis in Benign Prostatic Hyperplasia-1 Cells. Nutrients 10, 11. doi: 10.3390/nu10040523
Pei, X. J. (2018). Study on clinical dose-effect relationship and application characteristics of evodia rutaecarpa (Beijing: University of Chinese Medicine).
Peng, Q. X., Cai, H. B., Shi, Y., Peng, J. L., Mo, Z. X. (2012). The content differences of main alkaloids after compatible application of Rhizoma Coptidis and Fructus Evodiae. Chin. Med. Mat. 35, 742–744. doi: 10.13863/j.issn1001-4454.2012.05.045
Rebhun, J. F., Roloff, S. J., Velliquette, R. A., Missler, S. R. (2015). Identification of evodiamine as the bioactive compound in evodia (Evodia rutaecarpa Benth.) fruit extract that activates human peroxisome proliferator-activated receptor gamma (PPARgamma). Fitoterapia 101, 57–63. doi: 10.1016/j.fitote.2014.12.009
Ren, W., Li, Y., Zuo, R., Wang, H.-J., Si, N., Zhao, H.-Y., et al. (2014). Species-related difference between limonin and obacunone among five liver microsomes and zebrafish using ultra-high-performance liquid chromatography coupled with a LTQ-Orbitrap mass spectrometer. Rapid Commun. Mass Spectromet. 28, 2292–2300. doi: 10.1002/rcm.7026
Seya, K., Furukawa, K. I., Chiyoya, M., Yu, Z. Q., Kikuchi, H., Daitoku, K., et al. (2016). 1-Methyl-2-undecyl-4(1H)-quinolone, a derivative of quinolone alkaloid evocarpine, attenuates high phosphate-induced calcification of human aortic valve interstitial cells by inhibiting phosphate cotransporter PiT-1. J. Pharmacol. Sci. 131, 51–57. doi: 10.1016/j.jphs.2016.04.013
Sheu, J. R., Hung, W. C., Wu, C. H., Lee, Y. M., Yen, M. H. (2000). Antithrombotic effect of rutaecarpine, an alkaloid isolated from evodia rutaecarpa, on platelet plug formation in in vivo experiments. Br. J. Haematol. 110, 110–115. doi: 10.1046/j.1365-2141.2000.01953.x
Shi, X. Q., Yue, S. J., Tang, Y. P., Chen, Y. Y., Zhou, G. S., Zhang, J., et al. (2019). A network pharmacology approach to investigate the blood enriching mechanism of Danggui buxue Decoction. J. Ethnopharmacol. 235, 227–242. doi: 10.1016/j.jep.2019.01.027
Shyr, M. H., Lin, L. C., Lin, T. Y., Tsai, T. H. (2006). Determination and pharmacokinetics of evodiamine in the plasma and feces of conscious rats. Anal. Chim. Acta 558, 16–21. doi: 10.1016/j.aca.2005.11.045
Tian, K. M., Li, J. J., Xu, S. W. (2019). Rutaecarpine: a promising cardiovascular protective alkaloid from Evodia rutaecarpa (Wu Zhu Yu). Pharmacol. Res. 141, 541–550. doi: 10.1016/j.phrs.2018.12.019
Tian, Q., Liu, F., Xu, Z., Liu, H., Yin, H., Sun, Z., et al. (2019). Evaluation of the chemical consistency of Yin-Chen-Hao-Tang prepared by combined and separated decoction methods using high-performance liquid chromatography and quadrupole time-of-flight mass spectrometry coupled with multivariate statistical analysis. J. Sep. Sci. 42, 1664–1675. doi: 10.1002/jssc.201800961
Tundis, R., Loizzo, M. R., Menichini, F. (2014). An overview on chemical aspects and potential health benefits of limonoids and their derivatives. Crit. Rev. Food Sci. Nutr. 54, 225–250. doi: 10.1080/10408398.2011.581400
Wang, Q. Z., Liang, J. Y. (2004). Survey on chemical constituents and physiological activities of Evodia Forst plants. Chin Trad. Herb. Drug 35, U007–U010. doi: 10.3321/j.issn:0253-2670.2004.08.050
Wang, H. H., Chou, C. J., Liao, J. F., Chen, C. F. (2001). Dehydroevodiamine attenuates beta-amyloid peptide-induced amnesia in mice. Eur. J. Pharmacol. 413, 221–225. doi: 10.1016/S0014-2999(00)00913-4
Wang, L. Y., Tang, Y. P., Liu, X., Ge, Y. H., Li, W. X. (2013). Research on Chinese medicine pairs (VI) - Coptidis Rhizoma-Evodiae Fructus. China J. Chin. Mat. Med. 38, 4214–4219. doi: 10.4268/cjcmm20132406
Wang, X. X., Zan, K., Shi, S. P., Zeng, K. W., Jiang, Y., Guan, Y., et al. (2013). Quinolone alkaloids with antibacterial and cytotoxic activities from the fruits of Evodia rutaecarpa. Fitoterapia 89, 1–7. doi: 10.1016/j.fitote.2013.04.007
Wang, L., Dou, L. W., Guo, W., Li, X. Y., Yang, Q., Wu, X. W., et al. (2017). Toxic Q-marker identification based on conventional application of traditional Chinese medicine: taking Evodiae Fructus as an example. Chin Tradit. Herb. Drug 48, 1159–1166. doi: 10.7501/j.issn.0253-2670.2017.06.017
Wu, H., Ding, J. S., Jiang, X. H. (2007). Pharamcokinetics of rutaecarpine in Beagle dogs. West Chin J. Pharm. Sci. 22, 408–410. doi: 10.3969/j.issn.1006-0103.2007.04.017
Wu, J. X., Zheng, H., Yao, X., Liu, X. W., Zhu, H. J., Yin, C. L., et al. (2019). Comparative analysis of the compatibility effects of Danggui-Sini Decoction on a blood stasis syndrome rat model using untargeted metabolomics. J. Chromatogr. B. Analyt. Technol. Biomed. Life Sci. 1105, 164–175. doi: 10.1016/j.jchromb.2018.12.017
Xia, Q. Y., Liu, Y. P., Yang, R. F., Liu, Q. Q., Zhuo, Y. Q., Li, H. X. (2014). Studies on the genetic toxicity of Evodia rutaecarpa and its main components. World Chin Med. 9, 145–150. doi: 10.3969/j.issn.1673-7202.2014.02.005
Xiao, Y., Duan, J. F., Liu, Y., Li, H., Dou, Z. Y. (2017). Historic Successive Changes of Euodiae Fructus in Processing Methods and Functions. Chin. J. Exp. Tradit. Med. Formulae. 23, 223–228. doi: 10.13422/j.cnki.syfjx.2017030223
Xu, S., Peng, J., Li, Y., He, L., Chen, F., Zhang, J., et al. (2012). Pharmacokinetic comparisons of rutaecarpine and evodiamine after oral administration of Wu-Chu-Yu extracts with different purities to rats. J. Ethnopharmacol. 139, 395–400. doi: 10.1016/j.jep.2011.11.023
Xu, Y. S., Qiu, F., Wu, S., He, R., Gong, M. X., Wang, Z. M. (2019). UPLC-MS/MS determination of the active components from Wuzhuyu Decoction in plasma and brain tissues of nitroglycerin-induced migraine rats. China J. Chin. Mat. Med. 1–17. doi: 10.19540/j.cnki.cjcmm.20190624.502
Yan, R., Wang, Y., Shen, W., Liu, Y., Di, X. (2011). Comparative pharmacokinetics of dehydroevodiamine and coptisine in rat plasma after oral administration of single herbs and Zuojinwan prescription. Fitoterapia 82, 1152–1159. doi: 10.1016/j.fitote.2011.07.012
Yan, R., Wang, Y., Shen, W., Zhao, X., Zhang, X. (2012). Relative determination of dehydroevodiamine in rat plasma by LC–MS and study on its pharmacokinetics. J. Chromatogr. Sci. 50, 582–585. doi: 10.1093/chromsci/bms050
Yang, J., Cai, X. T., Lu, W. G., Hu, C. P., Xu, X. J., Yu, Q., et al. (2013). Evodiamine inhibits STAT3 signaling by inducing phosphatase shatterproof 1 in hepatocellular carcinoma cells. Cancer Lett. 328, 243–251. doi: 10.1016/j.canlet.2012.09.019
Yang, X. B., Qian, P., Yang, X. W., Liu, J. X., Gong, N. B., Lv, Y. (2013). Limonoid constituents of Euodia rutaecarpa var. bodinieri and their inhibition on NO production in lipopolysaccharide-activated RAW264.7 macrophages. J. Asian Nat. Prod. Res. 15, 1130–1138. doi: 10.1080/10286020.2013.817392
Yang, S. S., Tian, M., Yuan, L., Deng, H. Y., Wang, L., Li, A. Z., et al. (2016). Analysis of E. rutaecarpa Alkaloids Constituents In Vitro and In Vivo by UPLC-Q-TOF-MS Combined with Diagnostic Fragment. J. Anal. Methods Chem. 2016, 1–10. doi: 10.1155/2016/3182796
Yang, F., Shi, L., Liang, T., Ji, L., Zhang, G., Shen, Y., et al. (2017). Anti-tumor effect of evodiamine by inducing Akt-mediated apoptosis in hepatocellular carcinoma. Biochem. Biophys. Res. Commun. 485, 54–61. doi: 10.1016/j.bbrc.2017.02.017
Yang, Y. F., Zhou, Q. L., Yang, X. W. (2017). Elucidation of compatibility interactions of traditional chinese medicines: in vitro absorptions across Caco-2 Monolayer of coptidis rhizoma and euodiae fructus in zuojin and fanzuojin formulas as a case. Phytother. Res. 31, 1220–1229. doi: 10.1002/ptr.5843
Yin, L. S., Lv, L. L., Gong, Y. S., Li, X. Y., Huang, W., SUn, R. (2015). Study on chronic toxicity of volatile oil from Evodia Fructus in Rats. Chin J. Pharmacovigilance. 12, 20–25. doi: 10.3969/j.issn.1672-8629.2015.01.0020
Yu, L. L., Liao, J. F., Chen, C. F. (2000). Anti-diarrheal effect of water extract of Evodiae fructus in mice. J. Ethnopharmacol. 73, 39–45. doi: 10.1016/S0378-8741(00)00267-1
Yu, X., Wu, D. Z., Yuan, J. Y., Zhang, R. R., Hu, Z. B. (2006). Gastroprotective effect of Fructus Evodiae water extract on ethanol-induced gastric lesions in rats. Am. J. Chin. Med. 34, 1027–1035. doi: 10.1142/S0192415X06004491
Zhang, T., Zhang, S. C., Wei, L. X. (1994). Medica Analysis and comparison of the essential oil from Evodia rutaecarpa (Juss.) Benth before and after processing by GC-MS. Chin. Mat. Med. 19, 341–343.
Zhang, X. F., Gao, N. N., Liu, Y. H., Du, H., Yang, L., Li, F. (2011). A Study on the composition and toxicity changes of essential oil of Evodia rutaecarpa (Juss.) Benth. Before and after processing. Pharm. J. Chin. PLA. 27, 229–232. doi: 10.3969/j.issn.1008-9926.2011.03.014
Zhang, S. R., Liu, S. L., Zhong, Z. G., Zeng, C. H., Wu, C. Y., Huang, Y. C. (2017). Study on hepatotoxicity of different processed products of Evodia rutaecarpa (Juss.) benth on L02 human normal liver cells in vitro. Chin Arch. TCM. 35, 2664–2667. doi: 10.13193/j.issn.1673-7717.2017.10.051
Zhang, S. R., Liu, S. L., Zhong, Z. G., Chen, B., Wei, H. (2018). The relationship of Dose-time-toxicity of different processed products of Evodia rutaecarpa (Juss.) Benth on hepatotoxicity in mice. Lishizhen Med. Mater. Med. Res. 29, 881–884. doi: CNKI:SUN:SZGY.0.2018-04-037
Zhang, C. P., Gong, Q. F., Yu, H., Wen, R., Yan, L. P. (2019). Study on processing technology of stir-fried Euodiae Fructus with water decoction of Coptidis Rhizoma of Zhangbang. Chin. Tradit. Herb. Drugs 50, 3065–3070. doi: 10.7501/j.issn.0253-2670.2019.13.011
Zhao, J. M., Zhang, Z. Q. (2011). Effect of different processing methods on three components in Evodia rutaecarpa. Chin. J. Exp. Tradit. Med. Formulae. 17, 60–62. doi: 10.3969/j.issn.1005-9903.2011.07.020
Zhao, W., Wu, M., Cui, L., Du, W. (2019). Limonin attenuates the stemness of cervical carcinoma cells by promoting YAP nuclear-cytoplasmic translocation. Food Chem. Toxicol. 125, 621–628. doi: 10.1016/j.fct.2019.02.011
Zhao, J. M. (2011). Study on quality, pharmacokinetics and tissue distribution of Evodia Fructus. 50–51, (Shenyang: Liaoning Univ. TCM).
Zhou, Q., Zhang, Q., Jin, R. M. (2011). Time-effect and Dose-effect of Evodia rutaecarpa on Hepatotoxicity in Mice. Chin J. Exper Trad. Med. Formulae 17, 232–235. doi: CNKI:11-3495/R.20110303.1352.014
Zhou, L., Xu, J. D., Zhou, S. S., Shen, H., Mao, Q., Kong, M., et al. (2017). Chemomics-based marker compounds mining and mimetic processing for exploring chemical mechanisms in traditional processing of herbal medicines, a continuous study on Rehmanniae Radix. J. Chromatogr. A. 1530, 232–240. doi: 10.1016/j.chroma.2017.11.036
Keywords: Tetradium ruticarpum, processing, polyherbal formulation, compatibility, phytochemistry, pharmacokinetics, toxicity, pharmacology
Citation: Shan Q-y, Sang X-n, Hui H, Shou Q-y, Fu H-y, Hao M, Liu K-h, Zhang Q-y, Cao G and Qin L-p (2020) Processing and Polyherbal Formulation of Tetradium ruticarpum (A. Juss.) Hartley: Phytochemistry, Pharmacokinetics, and Toxicity. Front. Pharmacol. 11:133. doi: 10.3389/fphar.2020.00133
Received: 06 September 2019; Accepted: 31 January 2020;
Published: 06 March 2020.
Edited by:
Cheorl-Ho Kim, Sungkyunkwan University, South KoreaReviewed by:
Francis-Alfred Unuagbe Attah, University of Ilorin, NigeriaWei Peng, Chengdu University of Traditional Chinese Medicine, China
Copyright © 2020 Shan, Sang, Hui, Shou, Fu, Hao, Liu, Zhang, Cao and Qin. This is an open-access article distributed under the terms of the Creative Commons Attribution License (CC BY). The use, distribution or reproduction in other forums is permitted, provided the original author(s) and the copyright owner(s) are credited and that the original publication in this journal is cited, in accordance with accepted academic practice. No use, distribution or reproduction is permitted which does not comply with these terms.
*Correspondence: Qiao-yan Zhang, zqy1965@163.com; Gang Cao, caogang33@163.com; Lu-ping Qin, lpqin@zcmu.edu.cn
†These authors share first authorship