- 1MTA-SZTE Research Group of Cardiovascular Pharmacology, Hungarian Academy of Sciences, Szeged, Hungary
- 2Department of Pharmacology and Pharmacotherapy, Faculty of Medicine, University of Szeged, Szeged, Hungary
- 3Institute of Biomedical Engineering, Karlsruhe Institute of Technology (KIT), Karlsruhe, Germany
- 4Orion Pharma, Espoo, Finland
Background and Purpose: The exact mechanism of spontaneous pacemaking is not fully understood. Recent results suggest tight cooperation between intracellular Ca2+ handling and sarcolemmal ion channels. An important player of this crosstalk is the Na+/Ca2+ exchanger (NCX), however, direct pharmacological evidence was unavailable so far because of the lack of a selective inhibitor. We investigated the role of the NCX current in pacemaking and analyzed the functional consequences of the If-NCX coupling by applying the novel selective NCX inhibitor ORM-10962 on the sinus node (SAN).
Experimental Approach: Currents were measured by patch-clamp, Ca2+-transients were monitored by fluorescent optical method in rabbit SAN cells. Action potentials (AP) were recorded from rabbit SAN tissue preparations. Mechanistic computational data were obtained using the Yaniv et al. SAN model.
Key Results: ORM-10962 (ORM) marginally reduced the SAN pacemaking cycle length with a marked increase in the diastolic Ca2+ level as well as the transient amplitude. The bradycardic effect of NCX inhibition was augmented when the funny-current (If) was previously inhibited and vice versa, the effect of If was augmented when the Ca2+ handling was suppressed.
Conclusion and Implications: We confirmed the contribution of the NCX current to cardiac pacemaking using a novel NCX inhibitor. Our experimental and modeling data support a close cooperation between If and NCX providing an important functional consequence: these currents together establish a strong depolarization capacity providing important safety factor for stable pacemaking. Thus, after individual inhibition of If or NCX, excessive bradycardia or instability cannot be expected because each of these currents may compensate for the reduction of the other providing safe and rhythmic SAN pacemaking.
Introduction
Computational modeling as well as experimental results established previously that the normal pacemaker function is not only regulated by the hyperpolarization-activated funny current (If) (DiFrancesco, 1981) but is also regulated by the intracellular Ca2+ handling (Lakatta and DiFrancesco, 2009; Yaniv et al., 2013a; Yaniv et al., 2015; Sirenko et al., 2016). Lakatta and co-workers suggested that the sinus node (SAN) cells operate by a rhythmic clock-like oscillator system where the sarcoplasmic reticulum serves as a Ca2+-clock, which rhythmically discharges diastolic local Ca2+ releases (LCRs), and activates the forward (inward) Na+/Ca2+ exchanger (NCX) current to accelerate the diastolic depolarization and facilitates the membrane-clock (M-clock) (Yaniv et al., 2015). Recent experimental results further suggest that these clocks work tightly coupled since the M-clock regulates the Ca2+ influx and efflux while the NCX also regulates the diastolic depolarization forming a coupled-clock system. Therefore, NCX may have crucial importance in the clock-like oscillator system since the NCX-mediated inward current is directly translated to membrane potential changes via the operation of forward mode of the exchanger. This hypothesis was repeatedly challenged and the pivotal role of Ca2+ clock was questioned by other authors (Noble et al., 2010; Himeno et al., 2011; DiFrancesco and Noble, 2012).
As early as 1983, Irishawa and Morad showed in elegant experiments that full inhibition of If current by caesium did not significantly influence SAN spontaneous activity arguing for mechanisms other than If (Noma et al., 1983). On the other hand, other studies suggest a fundamental role of the exchanger in normal automaticity. A low-sodium bath solution inhibited spontaneous action potentials (AP) firing in guinea-pig SAN cells via suppressing normal function of NCX (Sanders et al., 2006). Other studies reported that depletion of SR store by application of ryanodine markedly disturbed the normal pacemaker activity in rabbit SAN cells (Bogdanov et al., 2001). Mouse genetic models revealed that partial atrial NCX1 knock out (≈90%) caused severe bradycardia and other rhythm disorders (Herrmann et al., 2013), while complete atrial NCX knock-out completely suppressed the atrial depolarization exerting ventricular escape rhythm on the ECG (Groenke et al., 2013). The application of KB-R7943, a non-selective NCX inhibitor, also suppressed spontaneous beating in guinea-pig SAN cells (Sanders et al., 2006) however it has also marked effect on the Ca2+-currents. The supposed crucial role of NCX in the normal pacemaker function of SAN could not be directly investigated experimentally so far due to the lack of a selective NCX inhibitor. Recently, two novel NCX inhibitors were synthesized: ORM-10103 and ORM-10962, both showing improved selectivity without influencing ICaL function (Jost et al., 2013; Kohajda et al., 2016; Oravecz et al., 2017).
In this study we confirmed the contributing role of NCX to spontaneous pacemaking by its direct pharmacological inhibition via the novel, selective inhibitor ORM-10962. Our data suggest that a strong crosstalk between If and NCX also exists in multicellular level, which was described and discussed by the Lakatta group earlier in single cell level (Yaniv et al., 2015). In addition, however, extending these earlier findings, we show that the effect of individual If and NCX inhibition is minimal whereas a combined inhibition acts synergistically, providing an important safety margin for secure spontaneous activity of the SAN.
Materials and Methods
Ethical Statement
All experiments were conducted in compliance with the Guide for the Care and Use of Laboratory Animals (USA NIH publication No 85-23, revised 1996) and conformed to Directive 2010/63/EU of the European Parliament. The protocols were approved by the Review Board of the Department of Animal Health and Food Control of the Ministry of Agriculture and Rural Development, Hungary (XIII./1211/2012).
Animals
The measurements were performed in right atrial tissue obtained from young New-Zealand white rabbits from both genders weighing 2.0–2.5 kg.
Voltage-Clamp Measurements
Cell Preparations
For measuring If pacemaker current, we isolated single cells from the SAN region of rabbit heart by enzymatic dissociation. The animals were sacrificed by concussion after receiving 400 IU/kg heparin intravenously. The chest was opened and the heart was quickly removed and placed into cold (4°C) solution with the following composition (mM): NaCl 135, KCl 4.7, KH2PO4 1.2, MgSO4 1.2, 4-(2-hydroxyethyl)-1-piperazineethanesulfonic acid (HEPES) 10, NaHCO3 4.4, glucose 10, CaCl2 1.8, (pH 7.2 with NaOH). The heart was mounted on a modified, 60 cm high Langendorff column and perfused with oxygenated and prewarmed (37°C) solution mentioned above. After washing out of blood (3–5 min) the heart was perfused with nominally Ca-free solution until the heart stopped beating (approx. 3–4 min). The digestion was performed by perfusion with the same solution supplemented with 1.8 mg/ml (260 U/ml) collagenase (type II, Worthington). After 10–12 min, the heart was removed from the cannula. The right atrium was cut and the crista terminalis and SAN region were excised and cut into small strips. Strips were placed into enzyme free solution containing 1 mM CaCl2 and equilibrated at 37°C for 10 min. After 10 min with gentle agitation, the cells were separated by filtering through a nylon mesh. Sedimentation was used for harvesting cells. The supernatant was removed and replaced by HEPES-buffered Tyrode’s solution. The cells were stored at room temperature in the Tyrode’s solution.
Measurement of Pacemaker Current (Funny Current)
For the measurement of the If current, the method of Verkerk et al. (2009) was adapted and applied. The current was recorded in HEPES-buffered Tyrode’s solution while the composition of the pipette solution was the following (in mM): KOH 110, KCl 40, K2ATP 5, MgCl2 5, EGTA 5, HEPES 10, and GTP 0.1 (pH was adjusted to 7.2 by aspartic acid). The current was activated by hyperpolarizing voltage pulses to −120 mV from a holding potential of −30 mV. The pacemaker current was identified as ivabradine (IVA) sensitive current. The experiments were performed at 37°C.
Fluorescent Optical Measurements
Isolated, spontaneously beating SAN cells were used for measurements. Ca2+ transients were measured by Fluo-4 AM fluorescent dye. Isolated cells were loaded with 5 µM dye for 20 min in room temperature in dark. Loaded cells were mounted in a low volume imaging chamber (RC47FSLP, Warner Instruments) and continuously superfused with normal Tyrode solution. Fluorescence measurements were performed on the stage of an Olympus IX 71 inverted fluorescence microscope. The dye was excited at 480 nm and the emitted fluorescence was detected at 535 nm. Optical signals were sampled at 1 kHz and recorded by a photon counting photomultiplier module (Hamamatsu, model H7828). Amplitudes of the Ca2+ transients were calculated as differences between systolic and diastolic values. To measure Ca2+ changes the cells were damaged by a patch pipette at the end of the experiment to obtain maximal fluorescence (Fmax). Ca2+ was calibrated using the following formula: Kd(F−Fmin)/(Fmax−F). Kd of the Fluo-4 AM was 335 nM.
Action Potential Measurements With Standard Microelectrode Technique
We have chosen multicellular preparations for action potential measurements for three reasons: 1) all of the ion channels remained intact (current density, kinetics) because of the lack of enzymatic dissociation, thus providing more precise estimates of the ratio between the currents, 2) since the SAN cells are surrounded with atrial cells having a more negative resting membrane potential, the electrotonic coupling may intimately influence the SAN cells. It may have great importance since the If current markedly increases as the membrane potential drops to more negative values (Morad and Zhang, 2017), (3) the action potential frequency was very stable with a cycle-length variability lower than 5 ms.
SAN regions obtained from right atria were mounted in a tissue chamber superfused with oxygenated Locke’s solution at 37°C. A conventional microelectrode technique was performed as previously described (Kormos et al., 2014; Kohajda et al., 2016; Oravecz et al., 2017). In the case of SAN, the action potentials were empirically found in the right atrium. SAN action potentials were verified by the maximum upstroke speed, which had to be lower than 15 V/s, the resting membrane potential (>−60 mV), and a clear diastolic depolarization (DD). Efforts were made to maintain the same impalement throughout each experiment. If impalement became dislodged, however, electrode adjustment was attempted and, if the action potential characteristics of the re-established impalement deviated by less than 5% from those of the previous measurement, the experiment was continued. When this 5% limit was exceeded, the experiment was terminated and all data were excluded from the analyses.
Action potential durations were measured at 90, 50, and 25% of repolarization. The maximal diastolic potential was defined as the most negative potential reached during the repolarization. The take off potential is the most negative point of the AP upstroke. DD was defined as the interval between the maximal diastolic potential and take off potential. The DD slope was calculated as ΔVm/Δt between these points. The cycle length was calculated as the peak-to-peak interval between two consecutive APs. The phase 0 depolarization velocity was defined as the maximum of the first derivative of the AP during the upstroke.
Modeling
To complement the experiments, we performed mechanistic computational modeling using the Yaniv et al. model of rabbit SAN cells (Yaniv et al., 2013b). The differential equations of the model were solved using a forward Euler scheme. Simulation results were analyzed when the system had converged to a cyclic steady-state.
Statistical Analysis
All data are expressed as mean ± standard error (SEM). Statistical analysis was performed with Student’s t-test and ANOVA with Bonferroni post-hoc test. The results were considered statistically significant when p was < 0.05. In the case of action potential experiments, all recordings were obtained from different hearts.
Materials
With the exception of ORM-10962 (ORM) (from Orion Pharma, Espoo, Finland), and Fluo-4 AM (Thermo Fisher Scientific, Waltham, MA, USA), all chemicals were purchased from Sigma-Aldrich Fine Chemicals (St. Louis, MO, USA). ORM was dissolved in dimethyl-sulfoxide (DMSO) to obtain a 1 mM stock solution. This stock solution was diluted to reach the desired final concentration (DMSO concentration not exceeding 0.1%) in the bath.
Results
ORM-10962 Has No Effect on Funny Current
Figure 1 shows the measurement of If in isolated SAN cells by applying the whole cell configuration of the patch clamp method. The selectivity of ORM on Na+-, Ca2+-, and major K+ currents was tested in a previous study from our laboratory (Kohajda et al., 2016). However, its potential effect on the If current was not investigated in that previous study. As Figures 1A, C show a slowly developed current at negative hyperpolarizing membrane potential (from −30 to −120 mV), which was not altered by application of 1 µM ORM (Figure 1B). In contrast, it was markedly inhibited by 10 µM IVA (69.3 ± 3.4%), a well known inhibitor of If.
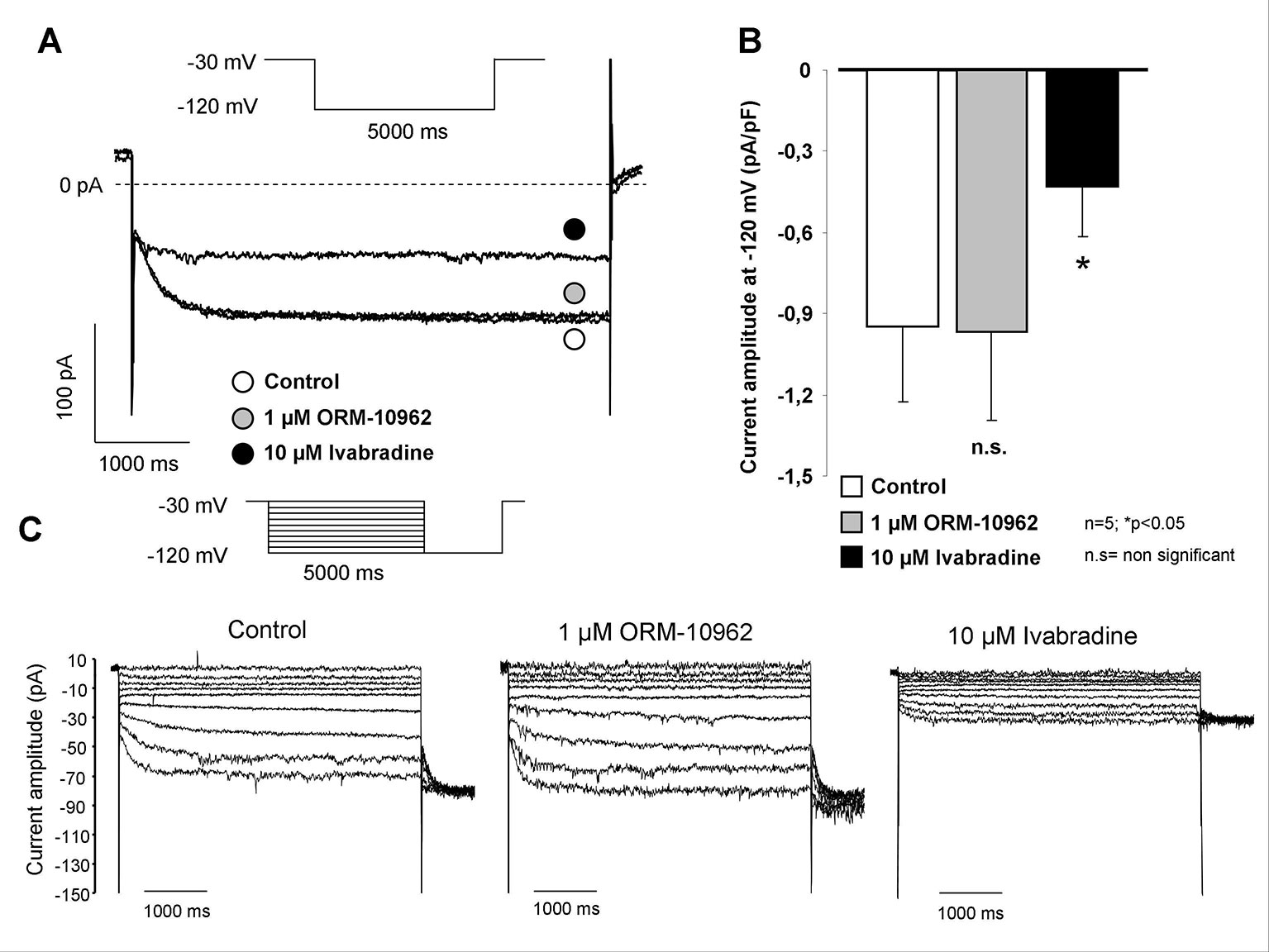
Figure 1 Investigation of the possible effect of ORM-10962 (ORM) on funny current (If) in isolated sinus node (SAN) cells. The hyperpolarization activated If was elicited by 5,000 ms long rectangle pulse potentials to −120 mV from a holding potential of −30 mV. As representative current traces indicate, the current amplitude after ORM application (gray circle) was identical with the control (open circle). The considerable effect of 10 µM ivabradine (IVA) verified that the elicited current was indeed If (A, B). Original traces in panel (C) represent the absence of ORM effects on current-voltage relationship of If by applying hyperpolarization pulses from −120 mV to −30 mV with 10 mV increments.
Na+/Ca2+ Exchanger Inhibition Exerted Moderate Bradycardic Effect on Sinus Node Tissue
Figure 2 summarizes the effect of selective NCX inhibition by ORM on the spontaneous automaticity in SAN. Following application of 1 µM ORM a moderate but significant lengthening effect on the CL was observed (455.6 ± 32 ms vs. 493.0 ± 38 ms; Δ = 8.1 ± 1.8% p < 0.05, n = 16/16 hearts; Figures 2A–C) without any influence on the action potential duration (APD) (94.3 ± 6.7 ms vs. 96.7 ± 5.9 ms; Figure 2D). The slope of the diastolic depolarization phase was significantly reduced after ORM application (15.7 ± 3.1 mV/s vs. 10.9 ± 2.8 mV/s; n = 14/14; p < 0.05 Figure 2E) while the CL variability remained unchanged (7.6 ± 1.2 ms vs. 8.1 ± 1.3 ms; Figure 2F). The slope of phase 0 AP depolarization was identical during control and ORM experiments (11.2 ± 2.7 V/s vs. 12.5 ± 2.3 V/s). The preparations maintained the stable frequency in the time control experiments when DMSO was applied (440 ± 36.1 ms vs. 445 ± 37.6; n = 4). In the computational SAN action potential model (Yaniv et al., 2013b), we identified the degree of NCX current suppression required to obtain a similar CL increase as was experimentally measured. Forty-one percent of NCX inhibition was required to obtain 8% CL increase which was equal with the CL change observed experimentally (Figure 2G).
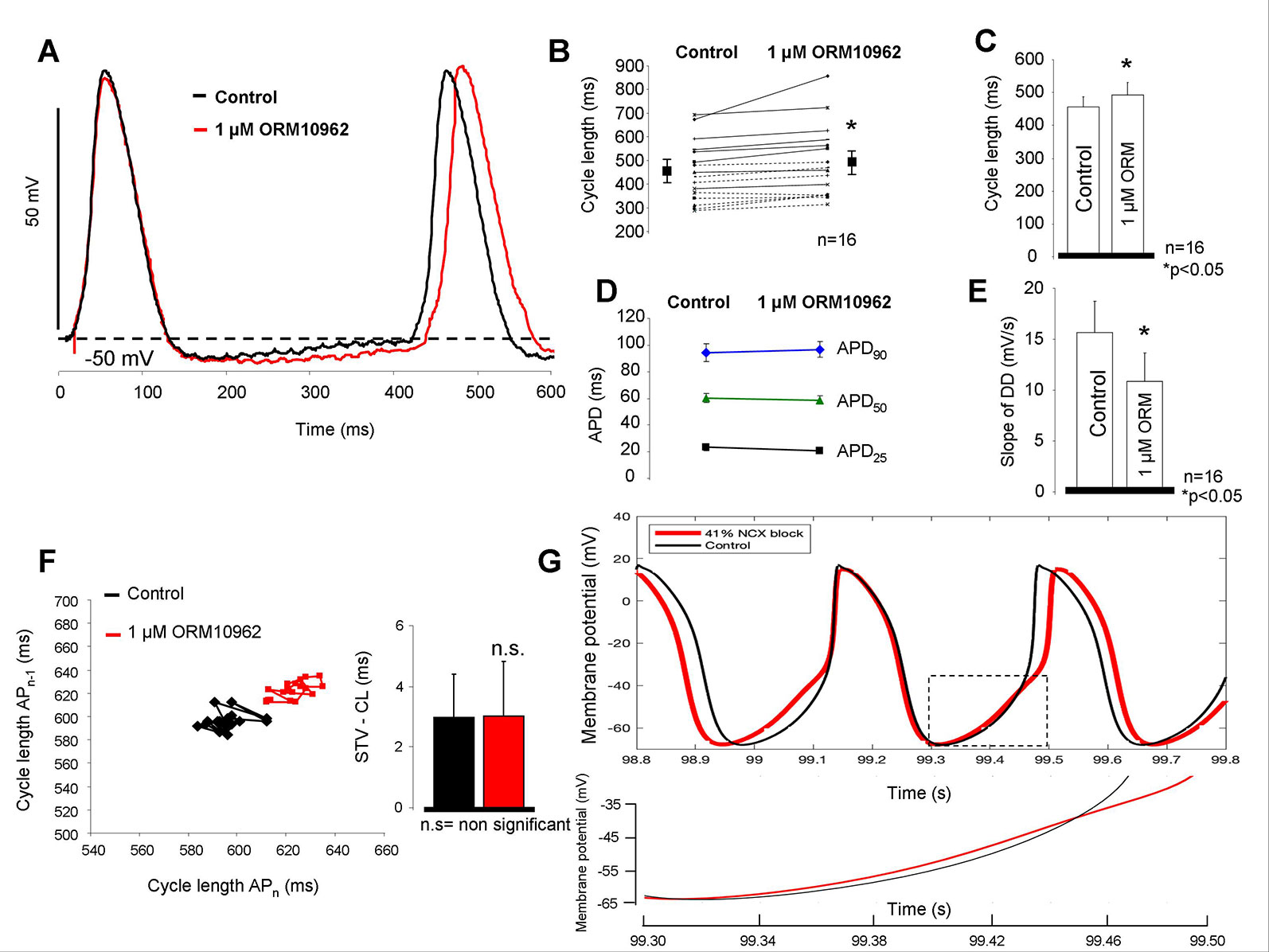
Figure 2 Estimation of the effect of selective Na+/Ca2+ exchanger (NCX) inhibition on sinus node (SAN) tissue. As representative action potential traces (A) as well as individual experiments (B) and bar graphs (C) indicate, application of 1 µM ORM-10962 (ORM) exerted a slight but statistically significant bradycardic effect on SAN tissue. The action potential duration (APD) did not change during the experiment (D), however the slope of the spontaneous depolarization was considerably decreased (E). 30 consecutive cycles were analyzed to estimate the pacing rate variability. Poincaré-plot and bar graphs depict that ORM did not alter the short-term cycle length (CL) variability (F). The Yaniv SAN cell model predicts 41% NCX inhibition to meet with the observed bradycardic effect of 1 µM ORM. The inset illustrates the reduced slope during late diastolic depolarization (DD) when 41% NCX inhibition was applied (red curve) (G).
Na+/Ca2+ Exchanger Inhibition Slightly Increased the Diastolic Ca2+ Level in Isolated Sinus Node Cells
The diastolic Ca2+ level increased in isolated SAN cells after ORM treatment (70 ± 11 nM vs. 130 ± 24 nM; p < 0.05, n = 10; Figures 3A, B), the effect was similar than was predicted by the Yaniv et al. SAN model (Figure 3D). In contrast to the model prediction, we found considerable increase in the transient amplitude (312 ± 37 nM vs. 568 ± 85 nM; p < 0.05, n = 10 Figure 3C), which was nearly doubled (82.1 ± 22%) in response to ORM application compared to the control value.
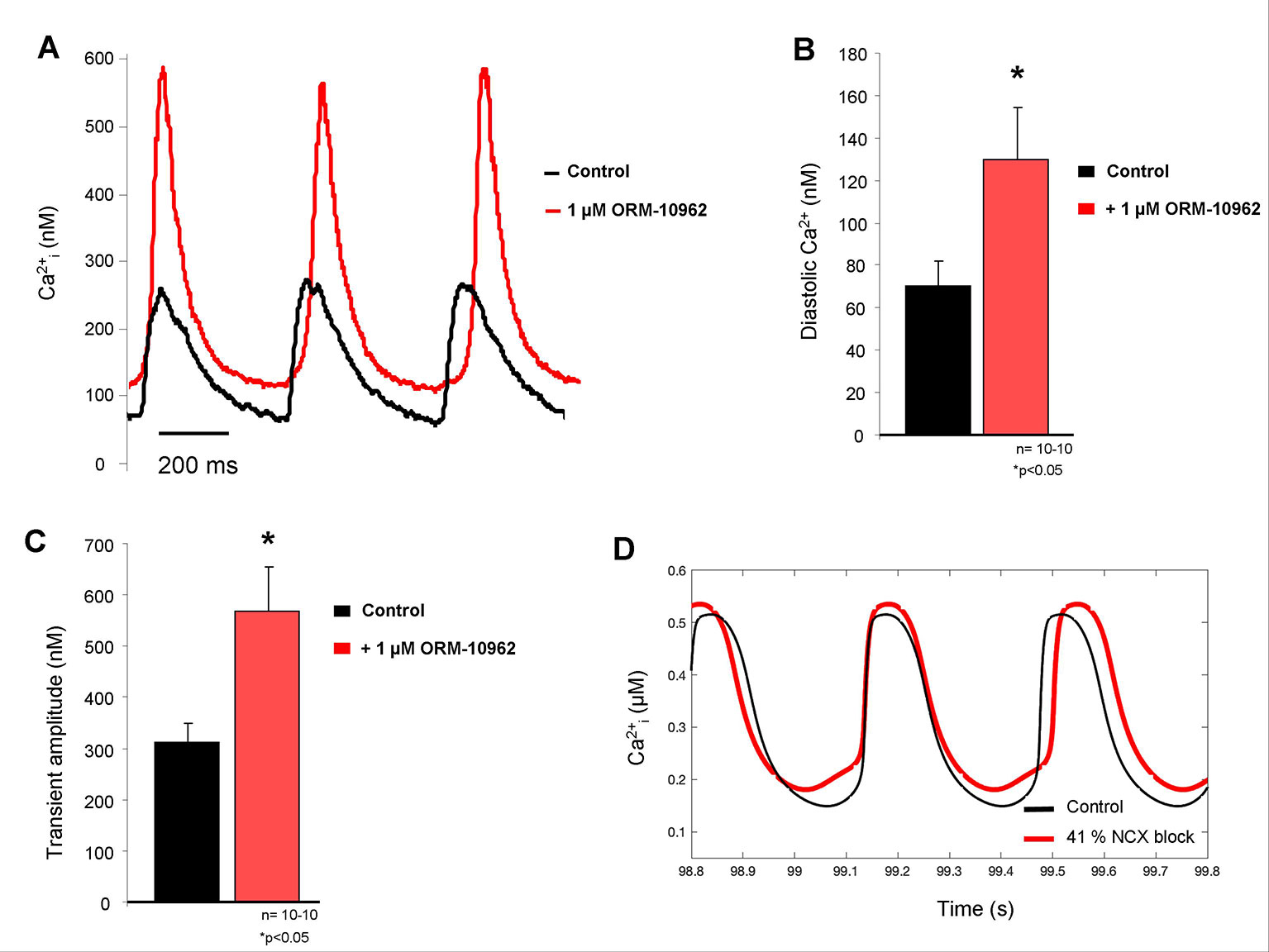
Figure 3 (A) Representative Ca2+ transient traces from spontaneously contracting isolated sinus node (SAN) cells under control conditions (black trace) and after 1 μM ORM-10962 (ORM) application (red trace). As original fluorescent data as well as bar graphs indicate, a slight but significant increase of the diastolic Ca2+ level (B) with increased transient amplitude (C) was observed. In line with experimental data, the Yaniv SAN cell model predicted similar diastolic Ca2+ gain after 41% Na+/Ca2+ exchanger (NCX) inhibition (D), however the increase of transient amplitude is much more pronounced during the experimental results.
The Concomitant Application of Ivabradine and ORM-10962 Revealed Coupled Frequency Control Between Funny Current and Na+/Ca2+ Exchanger Measured in Sinus Node Tissue
In the next set of experiments, IVA and ORM were subsequently applied to study a possible coupling between If and NCX. The effect of 1 µM ORM was substantially larger when If was previously inhibited (Figures 4A, B). Ca2+ transient measurements from spontaneously contracting SAN cells showed identical amplitudes (327 ± 23 nM vs. 337 ± 42 nM; n = 12) as well as diastolic Ca2+ levels (89 ± 22 nM vs. 85 ± 13 nM; n = 12) between control and 3 µM IVA (Figure 4C). A clear, gradual increase of ORM effect on the CL was observed with combined increasing concentration of IVA (1 µM ORM effect in the presence of 0 µM IVA: 8.1 ± 1.88%; in the presence of 0.5 µM IVA: 9.6 ± 2.3%; in the presence of 3 µM IVA: 17.1 ± 2.5%; Figure 4D). The ORM effect in the presence of 0.5 µM IVA did not differ significantly from the control, where 0 µM IVA was applied (8.1 ± 1.88% versus 9.6 ± 2.3%). In contrast, ORM effect was significantly larger on the CL in the presence of 3 µM IVA, compared with the control where IVA was not applied (8.1 ± 1.88% versus 17.1 ± 2.5%; p < 0.05, ANOVA, Bonferroni post hoc test). IVA significantly increased the CL both in 0.5 and in 3 µM concentrations (p < 0.05, ANOVA, Bonferroni post hoc test). In Figure 4E, we compare modeling and experimental results. In the Yaniv et al. model, based on a previous study (Bois et al., 1996), If inhibition was varied between 0%/20%/60% block (corresponding to 0, 0.5, and 3 µM IVA). Larger, 85% inhibition was only set in the model, since experimental application of 10 µM IVA is not feasible because of the marked IKr inhibition which can also reduce automaticity. The NCX inhibition was 41% in all cases. As Figure 4E shows, the modeling results do not match the experiments quantitatively, however they show a similar tendency (when If block increases, the same NCX inhibition causes larger CL prolongation) with markedly steeper correlation.
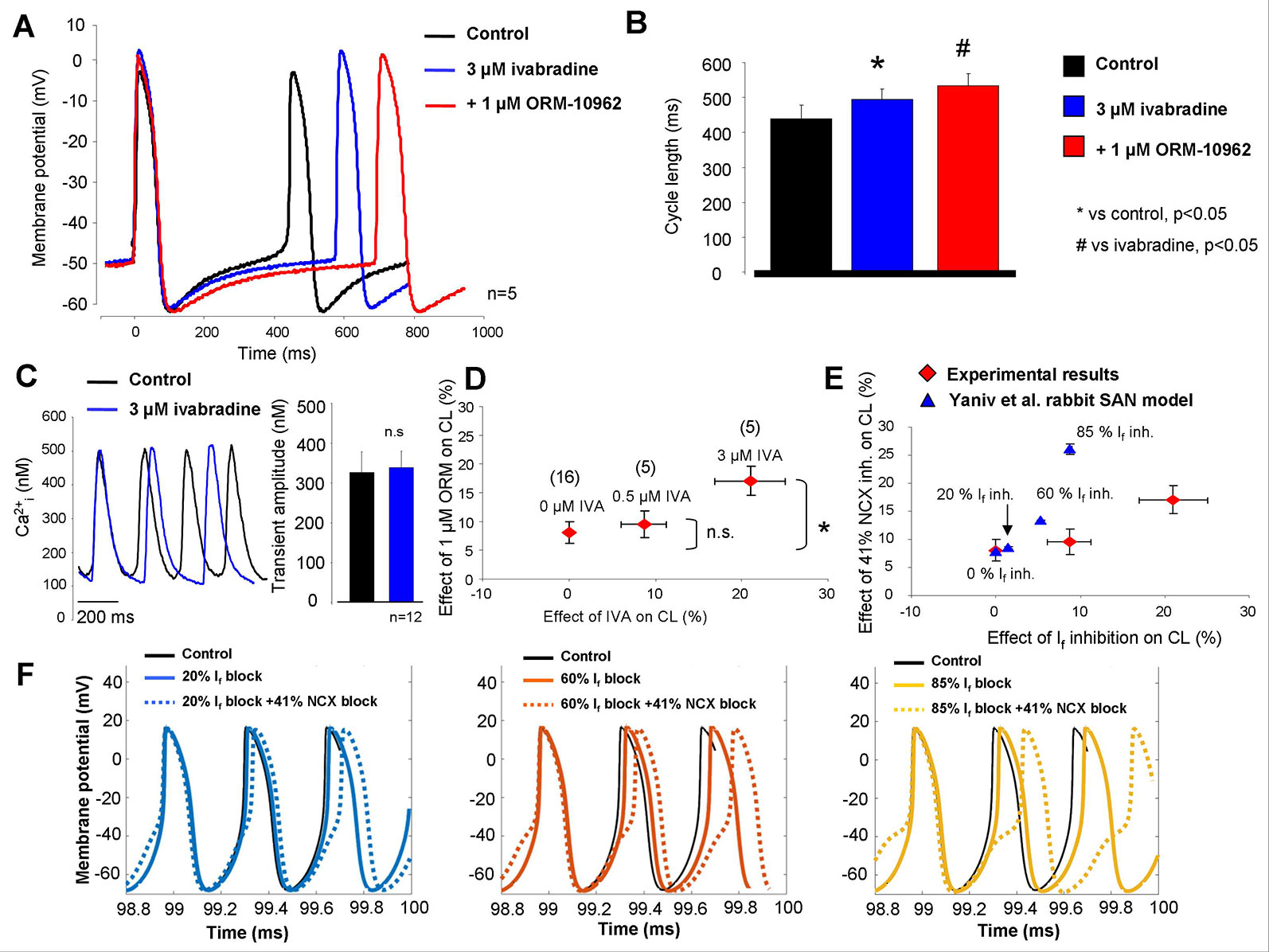
Figure 4 Combined inhibition of Na+/Ca2+ exchanger (NCX) and If in sinus node (SAN). As original SAN action potentials and bar graphs report (A, B), 1 μM ORM-10962 (ORM) (red trace) exerted an increased effect after 0.3 μM ivabradine (IVA) pretreatment (blue trace). Panel (C) represent Ca2+ transients measured from isolated SAN cells under control condition (black trace) and in the presence of 3 μM IVA (blue trace). We found identical Ca2+ levels as a result of IVA treatment. In panel (D), the dose dependent effect of IVA (abscissa) on SAN cycle length (CL) was plotted against the effect of consecutive application of 1 μM ORM on CL (ordinate). As was previously described in Figures 2A–C, 1 μM ORM has ≈8% effect without IVA. In the presence of 0.5 and 3 μM IVA, the ORM-induced reduction of pacing rate was gradually increased. The numbers in parentheses indicate the corresponding n. The experimental results (red) are compared with the Yaniv SAN cell model (blue) in panel (E). Based on a previous study, 0.5 and 3 µM IVA were represented by 20 and 60% funny current (If) inhibition in the presence of constant 41% NCX inhibition. Panel (F) represents the modeling results of combined If-NCX block. In the three panels, If was inhibited by varying degrees (straight lines) and combined with 41% NCX inhibition (dotted lines) yielding an increasing NCX inhibition effect on CL as If inhibition increases.
Figure 4F shows original modeling traces in the presence of 20% (left panel), 60% (middle panel), and 85% (right panel) If inhibition when NCX inhibition was 41% in all cases. The action potential modeling demonstrates an increased CL prolongation effect of NCX inhibition as If suppression becomes stronger, however, in contrast to the model prediction the steepness of NCX inhibition-induced CL increase was considerable flatter during experiments.
IKr Inhibition-Induced Bradycardia Did Not Facilitate the Effect of Selective Na+/Ca2+ Exchanger Inhibition on Cycle Length in Sinus Node Tissue
We investigated how bradycardia induced by a mechanism which does not directly involve the inward depolarizing currents (If and NCX) would influence the effect of NCX inhibition. Full IKr block induced by 100 nM dofetilide (DOF) markedly increased the CL of SAN AP (control: 489.3 ± 31 ms → 100 nM dofetilide: 649.1 ± 40.2 ms). This degree of increase of CL was due to the lengthening of APD without changing the DI. The subsequent application of 1 µM ORM exerted a similar effect (1 µM ORM-10962: 679.6 ± 52.6 ms; n = 7/7 hearts; Figures 5A, B), compared with results obtained after individual administration presented in Figure 2 (7.2 ± 1.8% vs. 8.1 ± 1.8%, Figure 5F). It is important that the effect of DOF on CL was nearly similar to 3 µM IVA (32.9 ± 6.7% vs. 20.9 ± 4.1%). However, the major difference was that the DOF-mediated increase in CL was practically entirely an APD increase-induced effect (APD90: 94.4 ± 3 ms vs. 187 ± 7.1 ms; p < 0.05, n = 7; diastolic interval (DI): 338.3 ± 39 ms vs. 352.7 ± 44.6 ms, n = 7) while the IVA influenced only the DI without affecting the APD90 (Figures 5C–E). In contrast, both NCX inhibition by ORM and If inhibition by IVA increased the CL due to lengthening of the time of the DI by decreasing its slope. When ORM was applied in combination with DOF the increase of the CL was not additive.
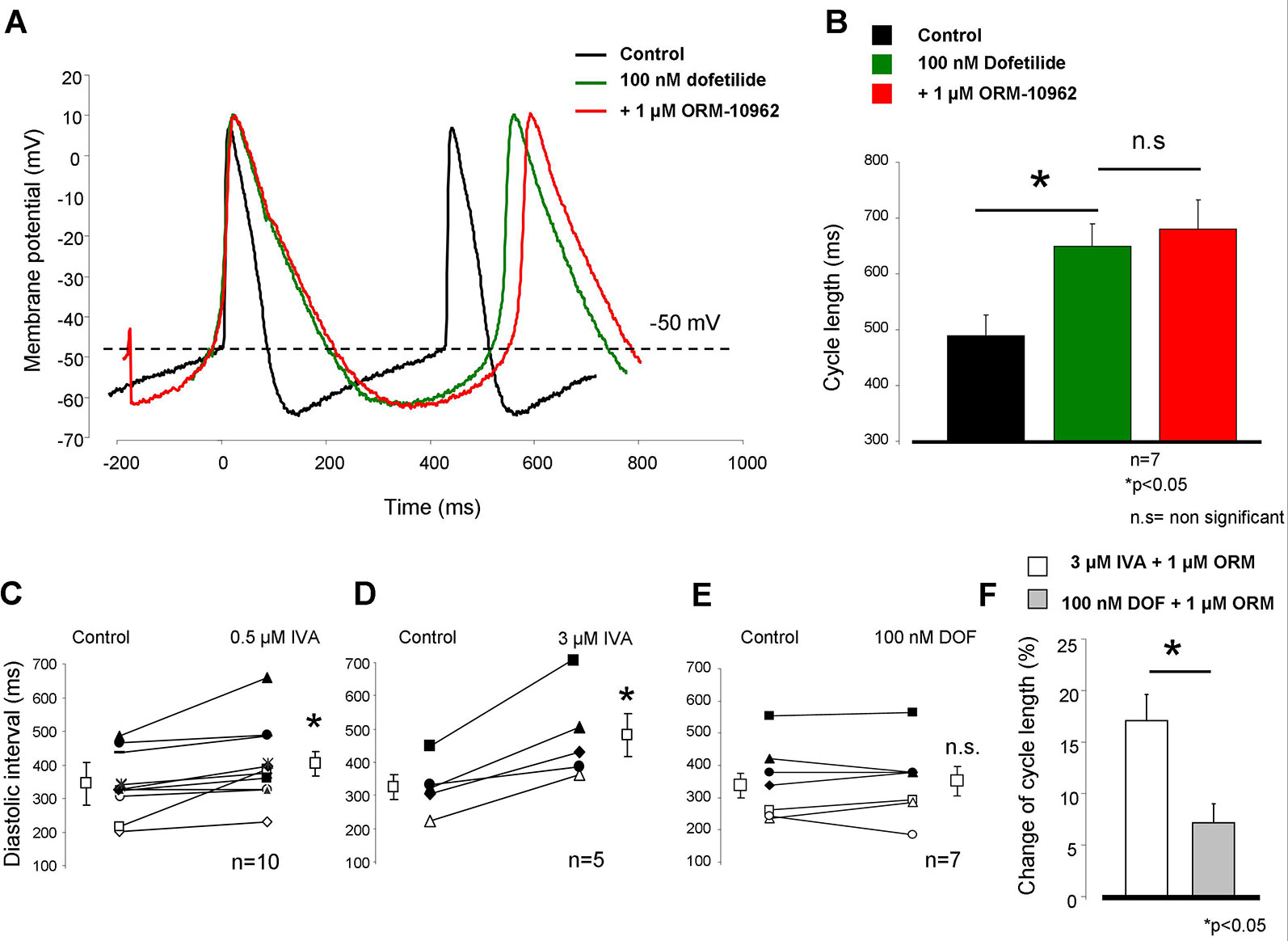
Figure 5 Sinus node (SAN) frequency was decreased by complete IKr inhibition by 100 nM dofetilide administration. The control cycle length (CL) (black trace) largely increased after dofetilide (green trace). However, the effect of 1 µM ORM-10962 (ORM) did not change compared with the individual effect (red trace, panels A, B, F). The main difference between ivabradine (IVA) and dofetilide (DOF)-induced bradycardia is that 0.5 and 3 µM IVA markedly increased the diastolic interval (C, D), which remained unchanged in the case of IKr inhibition by DOF (E).
Suppression of Ca2+ Increases the Effect of If Inhibition on Cycle Length in Sinus Node Tissue
In the next set of experiments, we investigated the potential effect of suppression of SR Ca2+ release on the effect of IVA (Figure 6A). The aim was to minimize the depolarizing activity of the Ca2+ release-induced augmentation of the forward NCX by application of 5 µM ryanodine (RYA) after the control recording. This caused a significant CL prolongation (437.8 ± 20.3 ms vs. 499.8 ± 10.4 ms; p < 0.05, n = 6/6). The subsequently applied 1 µM ORM-10962 marginally but statistically significantly increased the CL (499.8 ± 10.4 ms vs. 520.8 ± 29.9 ms; p < 0.05; n = 6/6). However, further 3 µM IVA markedly and significantly augmented the CL of the SAN preparations (520.8 ± 29.9 ms vs. 726.6 ± 39.8 ms; p < 0.05, n = 6/6; Figure 6B). In the Figure 6C we compared the IVA effects under normal condition (i.e., in the absence of any other inhibitors—20.9 ± 4.1%) and in the presence of RYA+ORM. As bar graphs in Figure 6C show, the IVA exerted markedly larger CL prolongation in the presence of RYA+ORM (42.4 ± 5.7%, p < 0.05, Student’s T-test).
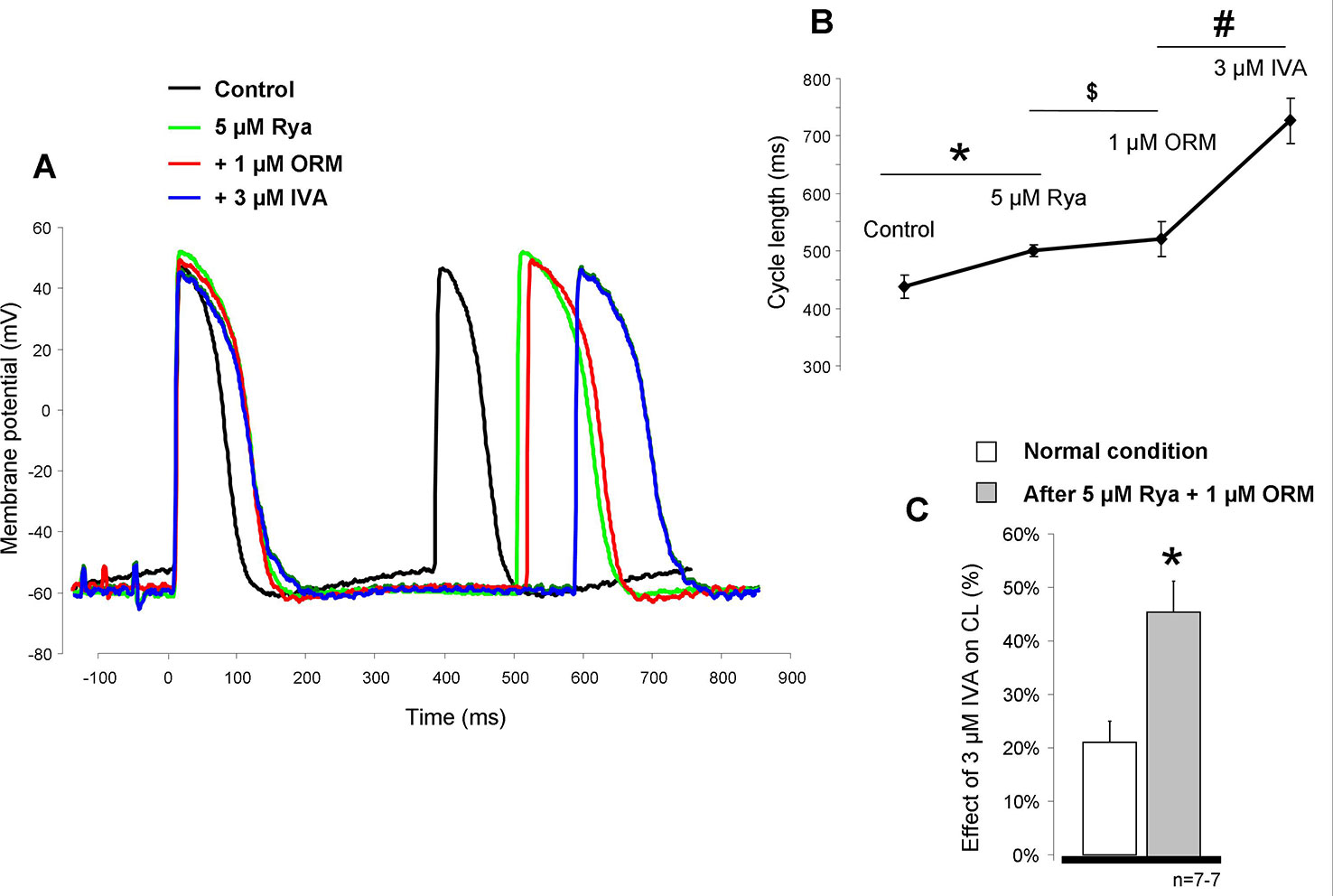
Figure 6 The potential influence of Ca2+ handling suppression by 5 µM ryanodine (RYA) (green line) and 1 µM ORM-10962 (red line) on the effect of funny current (If) reduction was investigated. As representative curves panel (A) and diagram panel (B) report, both drugs increased the cycle length (CL) of the action potential. Statistical analysis was achieved by ANOVA. * means RYA compared to control, $ means ORM-10962 (ORM) compared to RYA, and # means ivabradine (IVA) compared to ORM. Comparison of the effect of 3 µM IVA on the CL under normal condition (i.e., without any other inhibitors) and after 5 µM RYA + 1µM ORM shows that the same dose of IVA has markedly increased effect when the contribution of Na+/Ca2+ exchanger (NCX) is reduced by concomitant application of RYA and ORM panel (C).
Decrease of [Ca2+]O Increases the Effect of Funny Current Inhibition on Cycle Length in Sinus Node Tissue
We further tested the coupling between Ca2+ handling and If on CL control. Reduced [Ca2+]o (0.9 mM) external solution was selected to achieve this goal since in this concentration, the CL was only slightly reduced (10.3 ± 3.7%). We found that the reduced extracellular Ca2+ slightly increased the CL which was further increased after application of 3 µM IVA (control: 469 ± 39.5 ms → 0.9 mM [Ca2+]o: 515.8 ± 40.8 ms → 3 µM IVA: 777 ± 58.7 ms; p < 0.05, n = 6/6 hearts, Figures 7A, B). We compared again the effects of IVA on the CL under normal condition (i.e., 1.8 [Ca2+]o) and in the presence of low external Ca2+ (0.9 mM [Ca2+]o). As bar graphs in Figure 7C demonstrates the IVA has markedly improved effect when extracellular Ca2+ is low compared with normal Ca2+ settings (51.1 ± 5.1% versus 20.99 ± 4.1%, p < 0.05; Student’s t-test). Figure 7D represents Ca2+ transient measurements from spontaneously contracting isolated cells. We can observe that the application of 0.9 mM [Ca2+]o significantly decreased the transient amplitude (295 ± 52 nM vs. 185 ± 32 nM; p < 0.05, n = 8) which may reflect decreased Ca2+ influx, SR Ca2+ release which may decrease the NCX current and thus attenuate the compensating capacity of NCX. The diastolic Ca2+ also significantly decreased (127 ± 33 vs. 64 ± 10 nM; p < 0.05, n = 8). We addressed this question by using mechanistic modeling (Yaniv et al., 2013b). Left column of Figure 7E represents action potentials (upper traces), NCX and If current kinetics (middle traces), and global Ca2+ transients (lower traces) under normal condition. Upon application of 0.9 mM [Ca2+]o (right column) the CL slightly reduced, the integral of NCX current under the late phase of DD decreased while the magnitude of If current did not changed. The amplitude of the global transient decreased in similar extent as was obtained from SAN cell experiments.
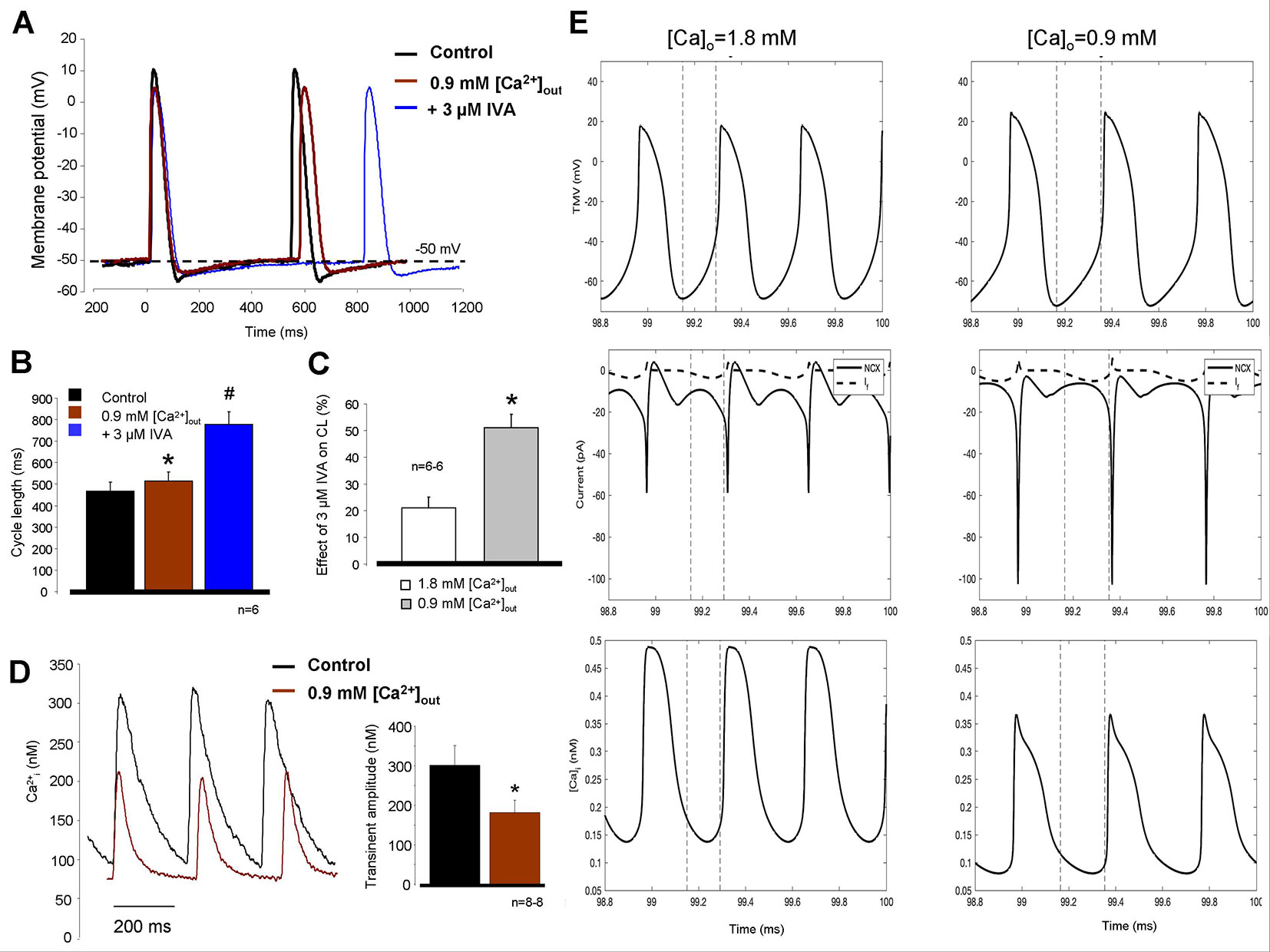
Figure 7 Decreased extracellular Ca2+ solution (0.9 mM) was used to suppress the intracellular Ca2+ cycling and therefore Na+/Ca2+ exchanger (NCX). The effect of hypocalcemic solution on the cycle length (CL) was marginal (A, B, brown trace) however the subsequently applied 3 µM ivabradine (IVA) (blue trace) caused considerable prolongation in the CL. Comparison of the IVA effect in the presence of normal (1.8 mM—white column) versus low (0.9 mM—gray column) CaCl2 on panel (C) demonstrates nearly doubled effect of IVA on the CL in response of Ca2+ reduction (C). * means 0.9 mM [Ca2+]o compared to control, # means IVA versus 0.9 mM [Ca2+]o. Original traces measured from isolated sinus node (SAN) cells in panel (D) demonstrate that 0.9 mM [Ca2+]o (brown trace) significantly decreased the transient amplitude without significant action on diastolic Ca2+ levels. (E) Modeling simulation of action potentials (upper traces), NCX currents (middle traces, solid lines), If currents (middle traces, dashed lines), and global Ca2+ transients (lower traces) in the present of normal (1.8 mM), external Ca2+ (left column), and 0.9 mM [Ca2+]o (right column). The results indicate decreased transient amplitude coupled with smaller NCX current in the late diastolic depolarization (DD) with maintained If current magnitude in the presence of 0.9 mM [Ca2+]o.
Concomitant Inhibition of Na+/Ca2+ Exchanger and Funny Current Increases the Cycle Length Variability in Sinus Node Tissue
The short term CL variability (CLV) was calculated by the analysis of CLs of N = 30 consecutive action potentials by applying the following formula:
One micrometer of ORM-10962 and 3 µM IVA individually prolong the CL without considerable influence on the CL variability (see the area covered in Figures 8A, B). The subsequent application of 5 µM RYA (Figure 8C, green line) and 5 µM RYA + 1 µM ORM-10962 (Figure 8C, red line) showed a tendency to increase the CL variability, however it proved not to be statistically significant. In contrast, additionally adding 3 µM IVA markedly and statistically significantly enhanced the variability parallel with the CL increase, when the Ca2+ release and NCX activity were suppressed (Figure 8C, blue line). As Figures 8D, E show, the CL variability exerts similar results as the CL measurements: individual inhibition of NCX (2.53 ± 0.8 ms vs. 2.71 ± 0.9 ms; n = 16/16; red line) and If (3.6 ± 0.9 ms vs. 5.19 ± 0.7 ms; n = 5/5; blue line) or Ca2+ handling suppression (3.03 ± 0.87 ms vs. 7.0 ± 2.73 ms, n = 7/7; green line) do not alter significantly the CLV while the variability was largely increased if IVA was administrated in the presence of reduced Ca2+ cycling activity (7.0 ± 2.73 ms vs. 15.29 ± 5.6 ms; orange line).
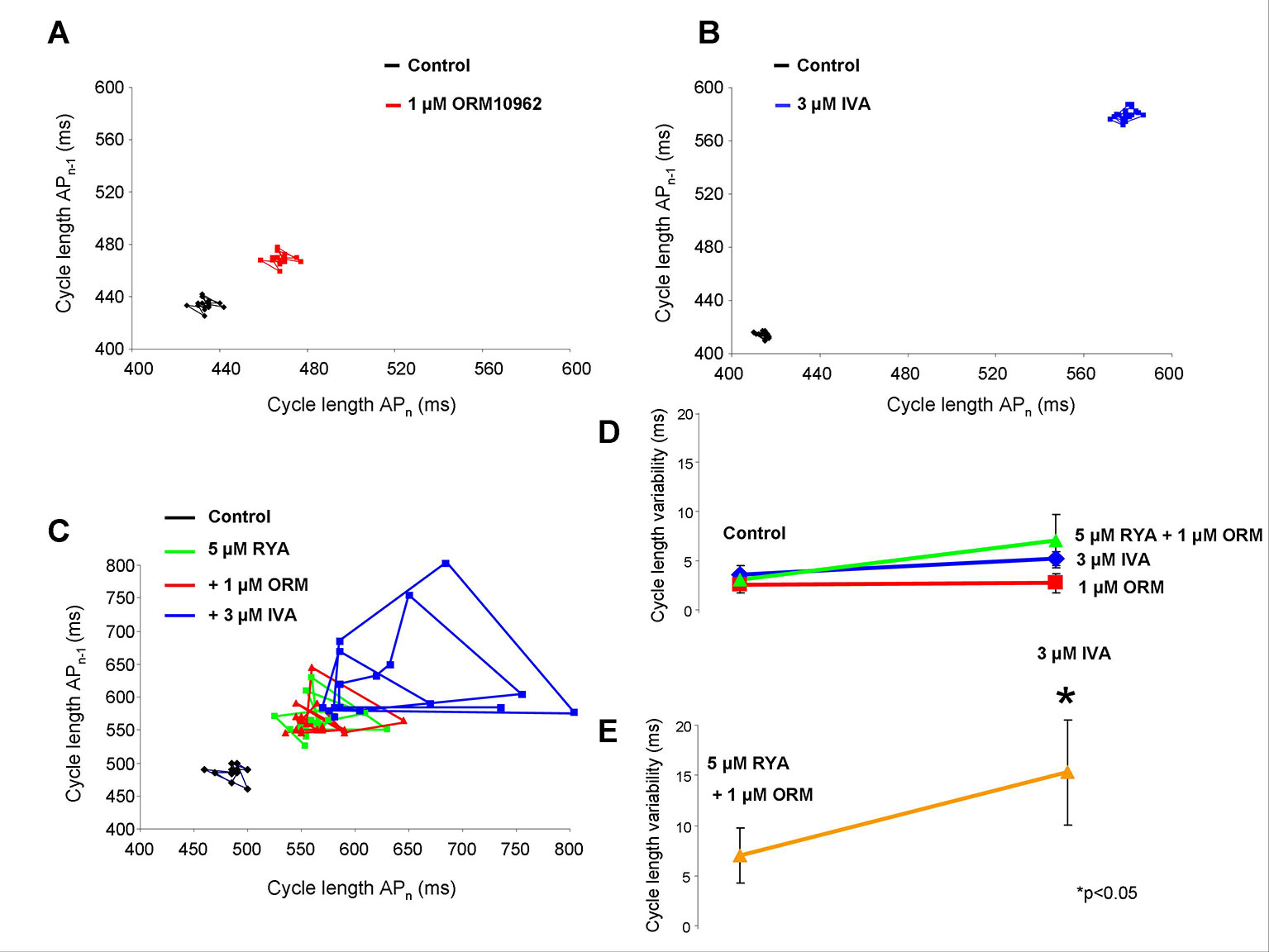
Figure 8 The effect of suppression of diastolic depolarization (DD) currents [Na+/Ca2+ exchanger (NCX) and If] on short time cycle length (CL) variability. When 1 µM ORM-10962 panel (A) or 3 µM ivabradine (IVA) panel (B) were applied, the variability did not change significantly. Instead, the CL was prolonged. Comparison of the effect of 1 µM ORM-10962 (ORM) (red line), 5 µM ryanodine (RYA) (blue line), and 5 µM RYA + 1 µM ORM (green line) on the CL variability shows that these compound do not change the variability significantly panel (D). In contrast IVA administration after the application of RYA+ORM caused considerably increased variability panel (E) as the Poincaré-plot (panel C, blue line) shows.
Discussion
The aim of this study was to verify and estimate the possible contribution of NCX function in SAN automaticity by direct selective pharmacological inhibition. Furthermore, we evaluated the functional consequences of the previously mentioned (Yaniv et al., 2015) If-NCX coupling in multicellular tissue level. In this study, we provided evidence for the first time regarding the essential role of NCX in spontaneous automaticity of the SAN by selective pharmacological inhibition. In addition, we described its functional interaction with If. This interaction between the DD currents is based on the following experimental results: i) 3 µM IVA has moderate effects on CL (~21%) and CLV (Δ ~ 2 ms), ii) 1 µM ORM has marginal effects on CL (~8%) and no change on CLV, iii) Ca2+ cycling suppression by 1 µM ORM + 5 µM RYA has moderate effects on CL (~19%) and CLV (Δ ~ 4 ms), iv) increasing If inhibition augments the effect of a fixed ORM dose (1 µM) on CL (~ 8 to 17%), v) the effect of 3 uM IVA is enhanced when Ca2+ cycling was previously suppressed (from ~ 20 to 42%).
ORM-10962 Does Not Inhibit the Funny Current
The effectiveness and selectivity of ORM-10962, a novel, potent NCX inhibitor was investigated in detail in our previous studies (Kohajda et al., 2016; Oravecz et al., 2017). In these studies, it was shown that ORM inhibited both forward and reverse mode NCX with an IC50 values of 55 and 67 nM without changing the ICa, INa, IK1, IKr, IKs, Ito, and INa/K pump currents even at high (1 μM) concentrations. However, the If current was not investigated. The present study demonstrates that 1 µM ORM did not influence If (Figure 1) in the presence of high Ca2+ buffering, which means that ORM is a suitable tool for the evaluation of NCX in SAN automaticity, however the indirect effects related with ORM-induced Ca2+ elevation (without Ca2+ buffering) may influence the If indirectly (Mattick et al., 2007).
Na+/Ca2+ Exchanger Inhibition Slightly Decreases Sinus Node Firing Rate
We found slight, but statistically significant reduction in the spontaneous firing rate in SAN tissue which is the consequence of the reduced rate of the DD (Figure 2). This result is a direct evidence and verification for the contribution of the inward NCX in rhythm generation. Previous studies (Yaniv et al., 2013a; Yaniv et al., 2015) reported that not only the increase of CL, but the parallel increase of pacing variability reports the uncoupling of the If-NCX and the destabilization of the DD. Since in our experiments the CL slightly increased while the pacing rate variability did not change, we conclude that the individual NCX inhibition did not cause considerable uncoupling of If-NCX.
The rate of forward NCX inhibition by using 1 µM ORM was estimated to ≈90% by applying conventional ramp protocol in the presence of ≈160 nM [Ca2+]i in canine ventricular myocytes in our previous study (Kohajda et al., 2016). The actual ratio of inhibited NCX which corresponds with the observed CL changes was calculated to 41% by using the Yaniv SAN cell model (Yaniv et al., 2013b). It is important to note, that in our previous study (Oravecz et al., 2017) we have demonstrated that the extent of NCX inhibition (via ORM-10962) is decreased when the intracellular Ca2+ is intact (i.e., in the presence of Ca2+ transient). The underlying mechanism could be asymmetrical block between two modes, autoregulation of the Ca2+i or by preserved inducibility of forward NCX by elevated Ca2+i.
Na+/Ca2+ Exchanger Inhibition Markedly Increases the Ca2+I Level
The selective NCX inhibition caused similar diastolic Ca2+ changes compared to the Yaniv model predicted, however, in contrast with modeling, we found markedly increased Ca2+ transient amplitude which is generally expected after decreased rate of Ca2+ extrusion (Figure 3). The observed quantitative discrepancy between experiments and modeling may indicate that the extent of NCX inhibition in the experiments could be larger than 41%.
We can speculate that the increasing intracellular Ca2+ is known to facilitate the inactivation of the L-type Ca2+ current as a part of the autoregulation (Eisner et al., 1998; Eisner et al., 2000). The gain of the [Ca2+]i may indirectly shortens the CL which means two parallel, counteracting effect of selective NCX inhibition: the inhibition of the inward NCX current may reduce the actual frequency by suppressing its contribution in the DD, however it is partially compensated for the CL abbreviating effect of increased [Ca2+]i. Furthermore, the If may also contribute in the limitation of the ORM effect: i) a theoretical possibility exists that ORM-induced Ca2+ elevation may increase the If, however this was ruled out by a previous work (Zaza et al., 1991). ii) It was reported that SAN myocytes express Ca2+-activated adenylate cyclase isoform, which might raise cAMP (and If) in response to NCX blockade (Mattick et al., 2007).
The Moderate Bradycardic Effect of Na+/Ca2+ Exchanger Inhibition May Be Explained by Funny Current-Na+/Ca2+ Exchanger Coupling
However, one may speculate after considering the crucial role of NCX in the coupled-clock theory, why the NCX inhibition-induced “bradycardia” exerted a relatively low influence. Using genetic mouse models Gao et al. claimed that partial ablation of NCX (≈70–80%) using an αMHC-inducible “Cre” transgenic line, has also a slight effect on the baseline spontaneous AP firing frequency (Gao et al., 2013). In line with this, it was found that even a small NCX fraction is able to generate sufficient inward current to provide appropriate depolarization current which is able to maintain normal SAN cell activity (Groenke et al., 2013). Our and these previous results highlight the possibility that a functional coupling between If and NCX represents a potency to compensate for the NCX inhibition-induced reduction of the pacing frequency.
In accordance with this theory our and previous results indicate a relatively moderate effect of IVA on the CL when it is administrated at 3 µM (Figure 4A) or at 10 µM (Yaniv et al., 2012). At the same time, caesium was unable to stop SAN beating even though it has a large effect on If (Noma et al., 1983). These inconsistent results can be explained by the voltage-dependent block of IVA or caesium (DiFrancesco, 1995), or by proposing an insulator function of the If to protect the SAN cells from the strong negative electrical sink of the connected atrial tissue (Morad and Zhang, 2017). However, a functional coupling between If and NCX (Bois et al., 1996; Lakatta et al., 2010; Yaniv et al., 2013a) providing redundant pacemaking systems could also explain—or at least contribute to—the observed results. This phenomenon, which could be very similar to the repolarization reserve (Biliczki et al., 2002; Herrmann et al., 2007; Lengyel et al., 2007; Nagy et al., 2009), may be also able to reduce the effects of the individual inhibition of NCX or If explaining the relatively small extent of IVA or NCX effects.
Indeed, we found that the effect of 1 µM ORM gradually increased as the rate of If block was enhanced (Figure 4). In line with this, the Yaniv model provided similar but steeper tendency, when we represented the 0.5, 1, and 3 µM IVA doses by 20, 60, and 80% If block based on previous results (Bois et al., 1996). While 10 µM IVA was not used experimentally due to selectivity problems, 85% If inhibition could be computed in the Yaniv model. The detailed modeling results are depicted in Figures 4E, F. Consistent with experimental results, the effect of 41% NCX inhibition on CL is increased as If inhibition becomes stronger. However, the modeling predicts a much steeper increase in the CL in the presence of enhancing If block. The underlying mechanism of this discrepancy could be the markedly higher Ca2+ increase measured during experiments which could limit the bradycardic effect of NCX inhibition. A previous study reported a decreased SR Ca2+ content after If inhibition by IVA (Yaniv et al., 2013a) demonstrating an indirect suppression of NCX during If inhibition. Our Ca2+ measurements (Figure 4C) indicate unchanged Ca2+ release after application of IVA, which may indicate that the underlying mechanism of increased ORM effect may be rather related with the increased sensitivity of DD when it is already inhibited by the If block.
IKr Inhibition Mediated Bradycardia Does Not Alter the Effect of Na+/Ca2+ Exchanger Inhibition
It is possible to decelerate spontaneous frequency without major direct influence on If or NCX. The SAN rate was reduced by 100% IKr block (Figure 5) in which the developed decrease in the firing rate was mainly achieved by APD prolongation without or minimal change in diastolic interval—instead of If block, which markedly increases the diastolic interval without effect on APD. This also means that despite the bradycardia, If is intact during these experiments. In line with this, NCX inhibition provided a similar effect to the one which was experienced when NCX was inhibited individually in Figure 2. This observation could be explained by an If dependent compensation of NCX reduction. At the same time it also means that the mechanism of the bradycardia is important regarding the effect of NCX inhibition. It seems possible that If mediated bradycardia and concomitant increase in diastolic interval may be important in the If-NCX interaction.
Suppression of Ca2+i Augments the Effect of Funny Current Inhibition
Assuming that a mutual interaction between If and NCX exists, this crosstalk should work in the opposite direction as well, i.e., a disturbance in the Ca2+ cycling should affect If. The suppression of the Ca2+ handling by the subsequent application of ryanodine and ORM together caused ≈20% increase in the CL in line with previous results (Bucchi et al., 2003). Under this condition, the effect of 3 µM IVA was considerably larger compared with normal settings (≈45% vs. 20%, see Figure 6C). In line with this, we found similar augmentation of IVA effect (21% vs. 51%) when Ca2+ handling was suppressed by low extracellular Ca2+ (Figure 7). Experimental as well as modeling simulations suppose that the Ca2+ handling and thus the NCX current suppression decreases the flux of the depolarizing NCX current, increases the length of DD, thus, the suppressed net current underlying the DD provides improved effect for If inhibition.
Funny Current-Na+/Ca2+ Exchanger Coupling Controls Cycle Length Variability
Previous studies (Yaniv et al., 2013a; Yaniv et al., 2015) reported that the If-NCX coupling not only controls the current CL but it may have a crucial role in maintaining the normal rhythm of the SAN. Therefore, the increase of the CL variability could be a further indicator of the integrity of If-NCX axis appearing after a considerable CL increase reporting significant If-NCX uncoupling. Our results support this assumption indicating that after individual inhibition of If or NCX, not only the excessive CL increase is restricted but the SAN rhythm is also maintained. However, when both of If and NCX are suppressed, besides the marked CL increase, a perturbation in the rhythm also appeared indicating the exhausted capacity of the If-NCX to depolarize the membrane during the DD (Figure 8). Since we could not reach complete inhibition of If and NCX in our experiments, we cannot estimate precisely the relative importance of these currents in the normal SAN rhythm. However, it seems possible that these currents contribute in the “depolarization reserve” (Herrmann et al., 2007) not only to the control of the current CL but also to the maintenance of the normal pacing rhythm as a consequence of the strong depolarizing of the If-NCX crosstalk.
Proposed Mechanism
We suggest that the observed NCX-If interplay is the consequence of the increased susceptibility of DD to any intervention when the DD was previously inhibited by another compound (Rocchetti et al., 2000; Zaza and Lombardi, 2001; Monfredi et al., 2014). This means that the bradycardic effect of NCX inhibition is larger when If was previously inhibited (independently from the Ca2+ handling). Vice versa, when the NCX was previously suppressed (as a consequence of reduced Ca2+ release) the decreased DD current density is more sensitive to changes, which increases the bradycardic effect of IVA.
Conclusion
In the present study, we provide direct pharmacological evidence regarding the role of NCX in pacemaker mechanism by its selective inhibition with the novel, highly selective compound ORM-10962. We found that individual inhibitions of NCX or If cause only moderate bradycardia and rhythm disturbance. However, combined suppression of these currents acted synergistically and supports the hypothesis of mutual crosstalk between NCX and If in SAN even in multicellular tissue having important functional consequences. This means that individual inhibition of DD currents may have moderated effect on CL and variability under normal conditions because the underlying currents may be able to compensate each other. This important crosstalk may provide a considerable safety margin for SAN pacemaking.
Study Limitations
Our study has three important limitations. 1) The action potentials measured in our experiments do not represent the characteristics of the core SAN cells. These cells are much more “follower” cells, having AP waveforms largely influenced by the cell-to-cell coupling. 2) The applied inhibitors (IVA, ORM, RYA) are not able to cause complete block of ion channels in the applied concentrations. Therefore, the described phenomena indicate only partial effects and not able to estimate the absolute contribution of NCX during the DD. 3) Since our aim was to explore ion current cooperation, our results represent ion channel function independent from the autonomic nervous system. The activation of the sympathetic or parasympathetic nervous system—or modulation of the β1/M2 receptors—intimately changes the cAMP, PKA, CaMKII levels which have effects on the DD currents, therefore the discussed If-NCX coupling cannot be directly extrapolated to in vivo systems. The ion current crosstalk characterization during intact autonomic control requires further experiments.
Data Availability Statement
All datasets generated for this study are included in the article/supplementary material.
Ethics Statement
The animal study was reviewed and approved by Munkahelyi Állatkísérleti Bizottság (MÁB).
Author Contributions
ZK performed ion current measurements and data analysis. NT performed fluorescent optical measurements, action potential measurements and data analysis. JS performed ion current measurements and data analysis. AL performed the computational modeling and data analysis, contributed to conception of the study as well as manuscript preparation and funding for the computational study. PG performed ion current measurements, GB and JP performed action potential measurements. NJ organized the database and ensured the financial support of the study. JL and PP contributed to the development of ORM-10962. JGYP contributed to manuscript preparation, AV and NN ensured the financial support of the study, contributed conception and design of the study, data analysis and visualization, and manuscript preparation. All authors contributed to manuscript revision, read and approved the submitted version.
Funding
This work was supported by grants from the National Research Development and Innovation Office (NKFIH PD-125402 (for NN), FK-129117 (for NN), GINOP-2.3.2-15-2016-00006, the LIVE LONGER EFOP-3.6.2-16-2017-00006 project, the János Bolyai Research Scholarship of the Hungarian Academy of Sciences (for NN), the UNKP-18-4-SZTE-76 New National Excellence Program of the Ministry for Innovation and Technology (for NN), the EFOP 3.6.3 VEKOP-16-2017-00009 (for NT), the Hungarian Academy of Sciences and by the Orion Pharma (ORM-10962).
Conflict of Interest
PP and JL are employed by Orion Pharma, which has been involved in the development of ORM-10962.
The remaining authors declare that the research was conducted in the absence of any commercial or financial relationships that could be construed as a potential conflict of interest.
Acknowledgments
We are grateful to Prof. Dr. David Eisner (University of Manchester, UK) for his help and suggestions for the manuscript. We gratefully acknowledge financial support by the Deutsche Forschungsgemeinschaft (DFG, German Research Foundation) – Project-ID 258734477 – SFB 1173 (to AL). The publication of this study was supported by the University of Szeged Open Access Fund (4309).
Abbreviations
AP, action potential; APD, action potential duration; CL, cycle length; CLV, cycle length variability; DD, diastolic depolarization; DI, diastolic interval; DOF, dofetilide; NCX, sodium-calcium exchanger; If, funny-current; IVA, ivabradine; ORM, ORM-10962; RYA, ryanodine; SAN, sinoatrial-node.
References
Biliczki, P., Virag, L., Iost, N., Papp, J. G., Varro, A. (2002). Interaction of different potassium channels in cardiac repolarization in dog ventricular preparations: role of repolarization reserve. Br. J. Pharmacol. 137 (3), 361–368. doi: 10.1038/sj.bjp.0704881
Bogdanov, K. Y., Vinogradova, T. M., Lakatta, E. G. (2001). Sinoatrial nodal cell ryanodine receptor and Na(+)-Ca(2+) exchanger: molecular partners in pacemaker regulation. Circ. Res. 88 (12), 1254–1258. doi: 10.1161/hh1201.092095
Bois, P., Bescond, J., Renaudon, B., Lenfant, J. (1996). Mode of action of bradycardic agent, S 16257, on ionic currents of rabbit sinoatrial node cells. Br. J. Pharmacol. 118 (4), 1051–1057. doi: 10.1111/j.1476-5381.1996.tb15505.x
Bucchi, A., Baruscotti, M., Robinson, R. B., DiFrancesco, D. (2003). I(f)-dependent modulation of pacemaker rate mediated by cAMP in the presence of ryanodine in rabbit sino-atrial node cells. J. Mol. Cell Cardiol. 35 (8), 905–913. doi: 10.1016/s0022-2828(03)00150-0
DiFrancesco, D., Noble, D. (2012). The funny current has a major pacemaking role in the sinus node. Heart Rhythm. 9 (2), 299–301. doi: 10.1016/j.hrthm.2011.09.021
DiFrancesco, D. (1981). A new interpretation of the pace-maker current in calf Purkinje fibres. J. Physiol. 314, 359–376. doi: 10.1113/JPHYSIOL.1981.SP013713
DiFrancesco, D. (1995). Cesium and the pacemaker current. J. Cardiovasc. Electrophysiol. 6 (12), 1152–1155.
Eisner, D. A., Trafford, A. W., Diaz, M. E., Overend, C. L., O’Neill, S. C. (1998). The control of Ca release from the cardiac sarcoplasmic reticulum: regulation versus autoregulation. Cardiovasc. Res. 38 (3), 589–604. doi: 10.1016/s6363(98)00062-5
Eisner, D. A., Choi, H. S., Diaz, M. E., O’Neill, S. C., Trafford, A. W. (2000). Integrative analysis of calcium cycling in cardiac muscle. Circ. Res. 87 (12), 1087–1094. doi: 10.1161/01.res.87.12.1087
Gao, Z., Rasmussen, T. P., Li, Y., Kutschke, W., Koval, O. M., Wu, Y., et al. (2013). Genetic inhibition of Na+-Ca2+ exchanger current disables fight or flight sinoatrial node activity without affecting resting heart rate. Circ. Res. 112 (2), 309–317. doi: 10.1161/CIRCRESAHA.111.300193
Groenke, S., Larson, E. D., Alber, S., Zhang, R., Lamp, S. T., Ren, X., et al. (2013). Complete atrial-specific knockout of sodium-calcium exchange eliminates sinoatrial node pacemaker activity. PloS One 8 (11), e81633. doi: 10.1371/journal.pone.0081633
Herrmann, S., Stieber, J., Stockl, G., Hofmann, F., Ludwig, A. (2007). HCN4 provides a ‘depolarization reserve’ and is not required for heart rate acceleration in mice. EMBO J. 26 (21), 4423–4432. doi: 10.1038/sj.emboj.7601868
Herrmann, S., Lipp, P., Wiesen, K., Stieber, J., Nguyen, H., Kaiser, E., et al. (2013). The cardiac sodium-calcium exchanger NCX1 is a key player in the initiation and maintenance of a stable heart rhythm. Cardiovasc. Res. 99 (4), 780–788. doi: 10.1093/cvr/cvt154
Himeno, Y., Toyoda, F., Satoh, H., Amano, A., Cha, C. Y., Matsuura, H., et al. (2011). Minor contribution of cytosolic Ca2+ transients to the pacemaker rhythm in guinea pig sinoatrial node cells. Am. J. Physiol. Heart Circ. Physiol. 300 (1), H251–H261. doi: 10.1152/ajpheart.00764.2010
Jost, N., Nagy, N., Corici, C., Kohajda, Z., Horvath, A., Acsai, K., et al. (2013). ORM-10103, a novel specific inhibitor of the Na+/Ca2+ exchanger, decreases early and delayed afterdepolarizations in the canine heart. Br. J. Pharmacol. 170 (4), 768–778. doi: 10.1111/bph.12228
Kohajda, Z., Farkas-Morvay, N., Jost, N., Nagy, N., Geramipour, A., Horvath, A., et al. (2016). The effect of a novel highly selective inhibitor of the Sodium/Calcium exchanger (NCX) on cardiac arrhythmias in In Vitro and In Vivo experiments. PloS One 11 (11), e0166041. doi: 10.1371/journal.pone.0166041
Kormos, A., Nagy, N., Acsai, K., Vaczi, K., Agoston, S., Pollesello, P., et al. (2014). Efficacy of selective NCX inhibition by ORM-10103 during simulated ischemia/reperfusion. Eur. J. Pharmacol. 740, 539–551. doi: 10.1016/j.ejphar.2014.06.033
Lakatta, E. G., DiFrancesco, D. (2009). What keeps us ticking: a funny current, a calcium clock, or both? J. Mol. Cell Cardiol. 47 (2), 157–170. doi: 10.1016/j.yjmcc.2009.03.022
Lakatta, E. G., Maltsev, V. A., Vinogradova, T. M. (2010). A coupled system of intracellular Ca2+ clocks and surface membrane voltage clocks controls the timekeeping mechanism of the heart’s pacemaker. Circ. Res. 106 (4), 659–673. doi: 10.1161/CIRCRESAHA.109.206078
Lengyel, C., Varro, A., Tabori, K., Papp, J. G., Baczko, I. (2007). Combined pharmacological block of I(Kr) and I(Ks) increases short-term QT interval variability and provokes torsades de pointes. Br. J. Pharmacol. 151 (7), 941–951. doi: 10.1038/sj.bjp.0707297
Mattick, P., Parrington, J., Odia, E., Simpson, A., Collins, T., Terrar, D. (2007). Ca2+-stimulated adenylyl cyclase isoform AC1 is preferentially expressed in guinea-pig sino-atrial node cells and modulates the I(f) pacemaker current. J.7 Physiol. 582 (Pt 3), 1195–1203. doi: 10.1113/jphysiol.2007.133439
Monfredi, O., Lyashkov, A. E., Johnsen, A. B., Inada, S., Schneider, H., Wang, R., et al. (2014). Biophysical characterization of the underappreciated and important relationship between heart rate variability and heart rate. Hypertension 64 (6), 1334–1343. doi: 10.1161/HYPERTENSIONAHA.114.03782
Morad, M., Zhang, X. H. (2017). Mechanisms of spontaneous pacing: sinoatrial nodal cells, neonatal cardiomyocytes, and human stem cell derived cardiomyocytes. Can. J. Physiol. Pharmacol. 95 (10), 1100–1107. doi: 10.1139/cjpp-2016-0743
Nagy, N., Szuts, V., Horvath, Z., Seprenyi, G., Farkas, A. S., Acsai, K., et al. (2009). Does small-conductance calcium-activated potassium channel contribute to cardiac repolarization? J. Mol. Cell Cardiol. 47 (5), 656–663. doi: 10.1016/j.yjmcc.2009.07.019
Noble, D., Noble, P. J., Fink, M. (2010). Competing oscillators in cardiac pacemaking: historical background. Circ. Res. 106 (12), 1791–1797. doi: 10.1161/CIRCRESAHA.110.218875
Noma, A., Morad, M., Irisawa, H. (1983). Does the “pacemaker current” generate the diastolic depolarization in the rabbit SA node cells? Pflugers Arch. 397 (3), 190–194. doi: 10.1007/bf00584356
Oravecz, K., Kormos, A., Gruber, A., Marton, Z., Kohajda, Z., Mirzaei, L., et al. (2017). Inotropic effect of NCX inhibition depends on the relative activity of the reverse NCX assessed by a novel inhibitor ORM-10962 on canine ventricular myocytes. Eur. J. Pharmacol. 818, 278–286. doi: 10.1016/j.ejphar.2017.10.039
Rocchetti, M., Malfatto, G., Lombardi, F., Zaza, A. (2000). Role of the input/output relation of sinoatrial myocytes in cholinergic modulation of heart rate variability. J. Cardiovasc. Electrophysiol. 11 (5), 522–530. doi: 10.1111/j.1540-8167.2000.tb00005.x
Sanders, L., Rakovic, S., Lowe, M., Mattick, P. A., Terrar, D. A. (2006). Fundamental importance of Na+-Ca2+ exchange for the pacemaking mechanism in guinea-pig sino-atrial node. J. Physiol. 571 (Pt 3), 639–649. doi: 10.1113/jphysiol.2005.100305
Sirenko, S. G., Maltsev, V. A., Yaniv, Y., Bychkov, R., Yaeger, D., Vinogradova, T., et al. (2016). Electrochemical Na+ and Ca2+ gradients drive coupled-clock regulation of automaticity of isolated rabbit sinoatrial nodal pacemaker cells. Am. J. Physiol. Heart Circ. Physiol. 311 (1), H251–H267. doi: 10.1152/ajpheart.00667.2015
Verkerk, A. O., den Ruijter, H. M., Bourier, J., Boukens, B. J., Brouwer, I. A., Wilders, R., et al. (2009). Dietary fish oil reduces pacemaker current and heart rate in rabbit. Heart Rhythm. 6 (10), 1485–1492. doi: 10.1016/j.hrthm.2009.07.024
Yaniv, Y., Maltsev, V. A., Ziman, B. D., Spurgeon, H. A., Lakatta, E. G. (2012). The “funny” current (I(f)) inhibition by ivabradine at membrane potentials encompassing spontaneous depolarization in pacemaker cells. Molecules 17 (7), 8241–8254.
Yaniv, Y., Sirenko, S., Ziman, B. D., Spurgeon, H. A., Maltsev, V. A., Lakatta, E. G. (2013a). New evidence for coupled clock regulation of the normal automaticity of sinoatrial nodal pacemaker cells: bradycardic effects of ivabradine are linked to suppression of intracellular Ca(2)(+) cycling. J. Mol. Cell Cardiol. 62, 80–89. doi: 10.1016/j.yjmcc.2013.04.026
Yaniv, Y., Stern, M. D., Lakatta, E. G., Maltsev, V. A. (2013b). Mechanisms of beat-to-beat regulation of cardiac pacemaker cell function by Ca(2)(+) cycling dynamics. Biophys. J. 105 (7), 1551–1561. doi: 10.1016/j.bpj.2013.08.024
Yaniv, Y., Lakatta, E. G., Maltsev, V. A. (2015). From two competing oscillators to one coupled-clock pacemaker cell system. Front. Physiol. 6, 28. doi: 10.3389/fphys.2015.00028
Zaza, A., Lombardi, F. (2001). Autonomic indexes based on the analysis of heart rate variability: a view from the sinus node. Cardiovasc. Res. 50 (3), 434–442. doi: 10.1016/s0008-6363(01)00240-1
Keywords: Na+/Ca2+ exchanger, funny-current, ORM-10962, pacemaking, sinus-node
Citation: Kohajda Z, Tóth N, Szlovák J, Loewe A, Bitay G, Gazdag P, Prorok J, Jost N, Levijoki J, Pollesello P, Papp JG, Varró A and Nagy N (2020) Novel Na+/Ca2+ Exchanger Inhibitor ORM-10962 Supports Coupled Function of Funny-Current and Na+/Ca2+ Exchanger in Pacemaking of Rabbit Sinus Node Tissue. Front. Pharmacol. 10:1632. doi: 10.3389/fphar.2019.01632
Received: 03 July 2019; Accepted: 13 December 2019;
Published: 29 January 2020.
Edited by:
Esther Pueyo, University of Zaragoza, SpainReviewed by:
Yael Yaniv, Technion Israel Institute of Technology, IsraelOliver Monfredi, University of Virginia, United States
Thomas Hund, The Ohio State University, United States
Copyright © 2020 Kohajda, Tóth, Szlovák, Loewe, Bitay, Gazdag, Prorok, Jost, Levijoki, Pollesello, Papp, Varró and Nagy. This is an open-access article distributed under the terms of the Creative Commons Attribution License (CC BY). The use, distribution or reproduction in other forums is permitted, provided the original author(s) and the copyright owner(s) are credited and that the original publication in this journal is cited, in accordance with accepted academic practice. No use, distribution or reproduction is permitted which does not comply with these terms.
*Correspondence: András Varró, varro.andras@med.u-szeged.hu
†These authors have contributed equally to this work