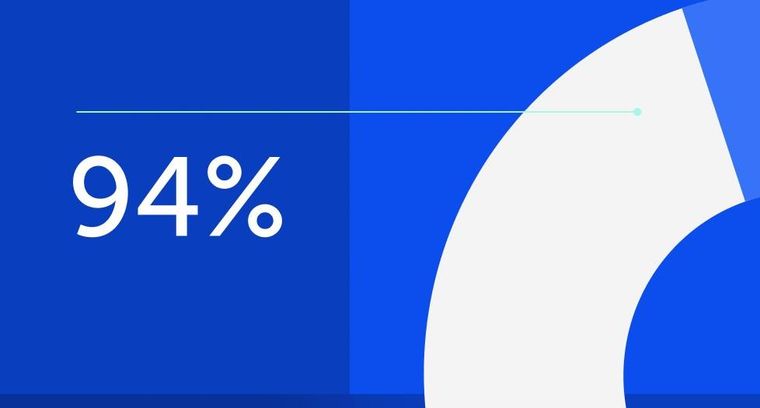
94% of researchers rate our articles as excellent or good
Learn more about the work of our research integrity team to safeguard the quality of each article we publish.
Find out more
ORIGINAL RESEARCH article
Front. Pharmacol., 24 January 2020
Sec. Ethnopharmacology
Volume 10 - 2019 | https://doi.org/10.3389/fphar.2019.01596
This article is part of the Research TopicApoptosis Induction/Suppression: A Feasible Approach for Natural Products to Treatment of DiseasesView all 29 articles
A retraction of this article was approved in:
Retraction: ErHuang formula improves renal fibrosis in diabetic nephropathy rats by inhibiting CXCL6/JAK/STAT3 signaling pathway
RETRACTED: Corrigendum: ErHuang Formula Improves Renal Fibrosis in Diabetic Nephropathy Rats by Inhibiting CXCL6/JAK/STAT3 Signaling Pathway
Citation: Shen Y-L, Jiang Y-p, Li X-Q, Wang S-J, Ma M-H, Zhang C-Y, Zhu J-Y, Rahman K, Zhang L-J, Luan X and Zhang H (2020) ErHuang Formula Improves Renal Fibrosis in Diabetic Nephropathy Rats by Inhibiting CXCL6/JAK/STAT3 Signaling Pathway. Front. Pharmacol. 10:1596. doi: 10.3389/fphar.2019.01596
Received: 07 August 2019; Accepted: 09 December 2019;
Published: 24 January 2020; Retracted: 02 December 2024.
Edited by:
Adolfo Andrade-Cetto, National Autonomous University of Mexico, MexicoReviewed by:
Yuntao Dai, Wangjing Hospital of China Academy of Chinese Medical Sciences, ChinaDisclaimer: All claims expressed in this article are solely those of the authors and do not necessarily represent those of their affiliated organizations, or those of the publisher, the editors and the reviewers. Any product that may be evaluated in this article or claim that may be made by its manufacturer is not guaranteed or endorsed by the publisher.
Research integrity at Frontiers
Learn more about the work of our research integrity team to safeguard the quality of each article we publish.