- 1National “111” Center for Cellular Regulation and Molecular Pharmaceutics, Key Laboratory of Fermentation Engineering, Ministry of Education, Hubei University of Technology, Wuhan, China
- 2Stollery Children's Hospital, University Alberta Hospital, Edmonton, AB, Canada
- 3Department of Laboratory Medicine and Pathology, University of Alberta, Edmonton, AB, Canada
- 4Department of Orthopedics, Tianyou Hospital, Wuhan University of Science and Technology, Wuhan, China
The use of nutraceuticals is considerably increasing worldwide with a demand for organic and clean foods in the last two decades, which is probably incomparable with other periods of our civilization. The consistent application of nutraceuticals and so-called “superfood” may have remarkable effects on the prevention of several chronic diseases, including cancer. Moreover, the increased rate of overweight and obesity in Western countries does not spare childhood and youth, and the number of parents using natural remedies for preventing pediatric illness is vastly increasing worldwide. However, the overwhelming effects on diseases often overshadow the side effects of such nutrition, particularly in societies without millennial experience with botanicals and natural elements. Thus, the final result may be disastrous for some individuals. The liver is the most important and conspicuous target organ of numerous molecular compounds, and the cell damage is particularly striking on the infantile and pediatric liver due to the immaturity of the hepatocytes. Here, we target some generic data on fulminant hepatic failure, the benefits, and toxicity of epigallocatechin-3-gallate, which is one of the major components of green tea, and the histopathology of the “green-tea”-associated liver disease.
Introduction
Nutraceuticals are compounds collectively defined under a broad umbrella term. These compounds are mostly derived from food sources and exhibit some health benefits other than the essential nutritional value. The benefits are variable and address the promotion of a general better well-being. Nutraceuticals also spawn discussions and concepts how to control illness and prevent malignancy. Some of these benefits are scientifically substantiated, while some are probably non-scientifically proved and lack solid scientific knowledge (Ward et al., 2019). Data using magnesium sulfate, sulforaphane (broccoli sprouts), creatine, choline, melatonin, and resveratrol in animal models suggest that natural health products may provide safe, effective, accessible, and affordable prevention of fetal brain injury and resulting lifelong disabilities (Shaw and Yager, 2019). Currently, some nutraceutical claims have also a trend. In some societies, they are also becoming part of philosophy with intransigent views that may mirror the appearance of a cult. One note that is often missed in healthcare reports on social media is the lack of a balance with the presentation of side effects with the beneficial effects (Abdualmjid and Sergi, 2013). This aspect is crucial mainly in vulnerable populations, such as children and youth (Pignatelli and Basili, 2010; Cicero and Colletti, 2018). Some societies, particularly in the West that have not been exposed to such compounds for hundreds of years, may leave individuals to adopt diet supplements in a state that may be more harmful than beneficial at some point. In the last century, this phenomenon was poorly known. Currently, several deaths of children whose parents had refused conventional therapy have stricken our society and, to some degree, the relationship between pediatricians and families (Krautkramer, 2005; Committee On B., 2013). In this paper, we target the virus-triggered fulminant hepatic failure and the hepatotoxicity of green tea and/or green tea extracts (e.g., epigallocatechin-3-gallate, EGCG) showing some histopathology data of “green-tea”-associated liver disease. Although EGCG is the major component in green tea, EGCG is not equal to green tea. Green tea is safely used in oriental countries without concerns about hepatotoxicity. It is important to emphasize that the green tea extract sold in the United States and western countries is not usually the “real” extract from green tea. There is, often, an addition of EGCG into the extract of green tea. The EGCG contents in such green tea extracts are remarkably higher than the original extract of green tea.
Viruses-Trigged Fulminant Hepatic Failure
Fulminant hepatitis with acute failure of the liver or academically known as fulminant hepatic failure (FHF) is a rare event in childhood, although it is not minimal (Fargion et al., 1996a; Sergi et al., 1999). FHF is a clinical syndrome. It is initially defined by the occurrence of encephalopathy of hepatic origin (hepatic encephalopathy) in a patient without pre-existing liver disease within a specific timeframe, i.e., eight weeks after onset of the first clinical symptoms (Mendizabal and Silva, 2016; Rosenblatt and Brown, 2018). Although FHF definition has changed over the years, FHF may still be distinguished on clinical grounds. Epidemiological and statistical studies have revealed that there are still prognostic implications in subdividing FHF in hyperacute, acute, and subacute liver failure, depending on whether the mentioned above interval is 7 days, 28 days, or 12 weeks, respectively (O'Grady et al., 1993). The gross morphology and histopathology of the “failing” (terminal) liver are standard and not necessarily point to a specific etiology. In all zones of the hepatic lobulus there is massive necrosis of hepatocytes, although periportal sparing of hepatocytes may be typical. Reticulin collapse and biliary duct proliferation are standard and accompanied by minimal or mild inflammatory reaction. There are a plethora of etiologic factors that can be considered responsible for triggering FHF. On several occasions, viruses, drugs, toxins, and metabolic disorders play a significant role (Abdualmjid and Sergi, 2013; Kodali and McGuire, 2015; Mendizabal and Silva, 2016; Garoufalia et al., 2019). In youth and adulthood, particularly in Asian countries, where the hepatitis B virus (HBV) vaccination has not been universally implemented, and the hepatitis A virus (HAV) is not considered to be implemented in healthcare, viruses play almost certainly a significant role in FHF. Since the identification of hepatitis C virus (HCV) as the leading agent for chronic post-transfusional and sporadic Non-A, Non-B (NANB) liver disease, antagonistic data as to its role in FHF have been divulgated in the scientific literature with the highest incidences reported in Japan and Taiwan (Sergi et al., 1998). During the dramatic course of FHF, there are numerous tests and false-negative results in testing only a single serum sample or “false positive” results due to virus uptake with untested blood products have been suggested to clarify these discrepancies (Sergi et al., 1996; Sergi et al., 2002). Also, it has been suggested that the examination of hepatic tissue from the explanted organ of subjects with NANB FHF undergoing liver transplantation may overthrow this health issue. Another critical aspect to consider is the combination of viral infections with the augmented trigger effect on the liver, which is entirely or submassively necrotic in the event of liver failure with a fulminant course (Figure 1).
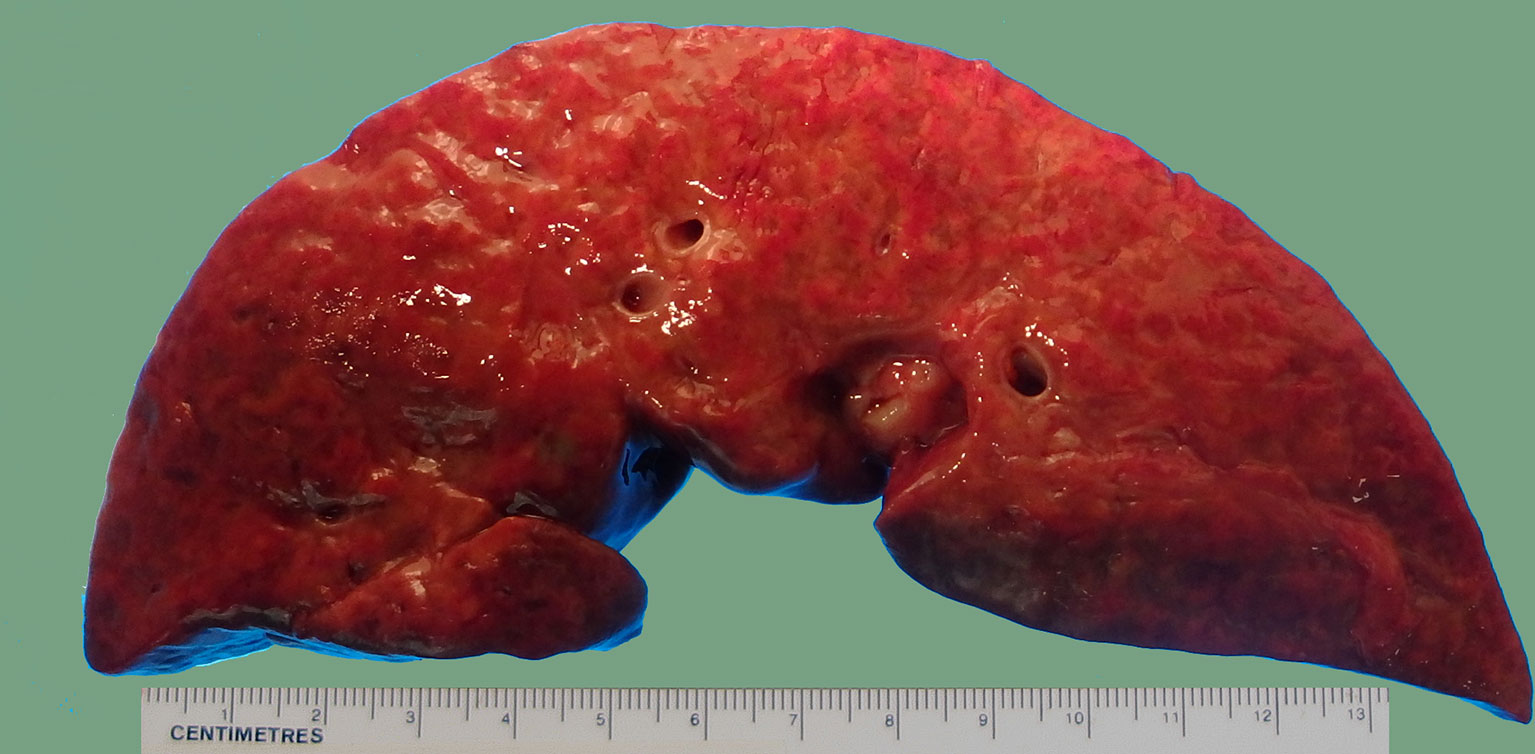
Figure 1 Gross photograph of a liver with fulminant hepatic failure. Note the bosselated appearance with wrinkling of the capsule and mottling cut surface due to intermingled red and yellow areas. No atresia of the extrahepatic biliary system was found by careful examination of the hilum.
Consequently, ruling out HBV induced FHF from investigations targeting the role of HCV in FHF may lead to miscalculating the role of this virus in FHF, but this aspect is also debated. Long-term prognosis and early post-liver transplantation may be jeopardized if an overlooking of a viral infection occurs. Recurrence of the original disease can be a relentless problem in the differential diagnostic procedures. As with HBV, the same setting may be valid for the hepatitis G virus (HGV). This virus has been found in chronic HCV infected patients (Sergi et al., 1998; Summerfield, 2000; Noor et al., 2018). Other uncommon viral etiologic factors that should be considered are cytomegalovirus (CMV) and parvovirus B19 (PVB19). In particular, CMV may both trigger an FHF and biliary atresia in infants (Spearman et al., 2005; Sergi, 2019). These etiologies may be obviously different according to the geographic area. Thus, FHF remains a challenging area in pediatric hepatology and is under investigation for formulating better guidelines worldwide.
Nutraceutical Use
Nutraceuticals can be a cause of FHF at any age (Karvellas et al., 2016; Cicero and Colletti, 2018; Stravitz and Lee, 2019). The exclusion of viral etiologic factors should suggest a toxic, autoimmune, or metabolic disorder as the culprit for FHF (Rosenblatt and Brown, 2018). A thorough clinical history among the patients and their parents is mandatory. The use of nutraceuticals is considerably increasing worldwide with demand in organic and clean foods, which is now seen in pediatrics and probably considered incomparable with other periods. The consistent application of nutraceuticals and so-called “superfood” has remarkable and tangible experiences in the prevention of numerous chronic non-oncological diseases and tumors. Also, the increased rate of obesity in Western countries does not spare childhood and youth, and the number of parents using nutraceuticals and natural remedies for pediatric obesity or illness is vastly increasing worldwide (Brunt, 2004; Hartman and Shamir, 2012). Sadly, the side effects of such nutrition are often overshadowed on both professional publications and social media. The resurgence of nutritional rickets in the 21st century may be associated, at least partially, to some vegan and vegetarian diet imposed on children by parents belonging to specific communities, no matter which faith they profess (Zhang et al., 2016; Antonucci et al., 2018; Yang et al., 2019). The histopathology of “green tea” or better green tea extracts-associated toxicity in children is poorly known. The potential dreadful event of FHF may need to be emphasized and extensively discussed in both professional and social media. Currently, pediatric obesity is a tragic evolution of the individual, and the use of social media and videogames have worsened the inactivity of youth worldwide. Obesity, which results from a positive energy balance, may represent an inexorable health problem, particularly in Western countries, but Asian countries, including China, have shown an increased rate in the last decade (Dong et al., 2019; Butler et al., 2019). Many children and youth wish to use one solution, and de-tox teas, as well as chemical compounds, are continually popping up in magazines and social media as well as in teenager events (Floyd, 2009; Cline, 2015). Obesity and wrong information on it, practically not curated by professional medical associations, is now found across well-developed and underdeveloped countries worldwide. Recently, the World Health Organization (WHO) appraised that more than 1.5 billion individuals with age more than 18 years worldwide are overweight. At least 500 million of which should be considered obese (Hartman and Shamir, 2012; West-Eberhard, 2019). The number of parents using nutraceuticals and natural remedies for pediatric illness and obesity is fast increasing, and some of these parents may also belong to the anti-vaxxer group, which is considered a new group of individuals against vaccination schedules (Signorelli, 2019). These groups may facilitate the recrudescence of exanthemata, which we considered almost disappeared (Leung et al., 2018; Leung et al., 2019). Although not comprehensive, we explored some studies that have been completed to characterize potential hazards following the consumption of green tea and/or green tea extracts. The liver is the target organ of this toxicity. The cell damage of EGCG, the major component of green tea, or similar components, to the infantile and pediatric liver may have fatal consequences.
Green Tea
In consideration of the increasing use of nutraceuticals in childhood and several youth pathologies, we performed a critical review of toxicology to portray potential hazards following the ingesting of green tea and its extracts. Hepatotoxicity is life-threatening and has been associated with certain dosing conditions. Tea (Camellia sinensis, Theaceae) is a favorite beverage and consumed by over two-thirds of the world population, particularly in China, India, Japan, and countries of the Commonwealth (Noor et al., 2018). The tea market is dominated by black tea, which is the kind of tea most largely traded worldwide (about 80%). The black tea is prevalent in North America and Europe, while the green variety is primarily consumed in mainland China, Taiwan, and Japan. The rarest tea used is white tea, which is also the least handled. The Oolong tea (semi-fermented) is a rare tea, which is in the middle between the fermented (black tea) and non-fermented tea (green tea). Three additional types of teas are also known. They include scented tea, compressed tea, and organic tea. In the scented tea, the additional flavoring is mixed to the non-/semi-/or fermented leaves as a final stage, while compressed tea is bricks of processed tea leaves that have been hydraulically compressed. In the production of organic tea, whose production started twelve to thirty years ago, the cultivation of tea follows strict rules which are controlled by organic agencies.
Green Tea Polyphenols (GTPs)
The major bioactive molecules of tea (30–42% of tea leaf dry weight) are the polyphenols in addition to methylxanthine alkaloids (caffeine, theophylline, and theobromine) (Balentine et al., 1997; Mazzanti et al., 2015). The group of the polyphenols contains the catechin, EGCG (Figure 2), along with epicatechin (EC), epigallocatechin (EGC), and epicatechin-3-gallate (ECG). EGCG is the most copious component reaching up to 80% of total content of polyphenols (Balentine et al., 1997; Ishii et al., 2011). Chemically, it is the ester of epigallocatechin and gallic acid. Polyphenols, which are also known as polyhydroxyphenols, are a structural class of mainly natural, but also synthetic or semisynthetic, organic molecules. Polyphenols contain large multiples of phenol structural units. EGCG is being used in many dietary supplements. According to the United States Department of Agriculture (USDA) Database including the Flavonoid Content of Selected Foods, EGCG is found in high content (7.4 g per 100 g) in the dried leaves of green tea, while almost half of this compound is present in white tea (4.2 g per 100 g). A decrease of 87.3% of this compound is found in black tea (0.9 g per 100 g) (Bhagwat et al., 2011). EGCG possesses the highest antioxidant potential and, to the best of our knowledge, is by far the most biologically active substance. The antioxidant properties with their protective roles against pathologies associated with a considerable amount of reactive oxygen species (ROS), such as cardiovascular diseases and cancer, are ascribed to the high catechin levels contained in the tea, particularly the green variety (Rietveld and Wiseman, 2003). The fermentation of the tea leaves induces the oxidization of catechins by polyphenol oxidase. The catechins are, then, changed into theaflavins and thearubigins. These chemical compounds are condensed polymeric molecules, which have been considered accountable for the typical organoleptic features of black tea. Non-fermented tea leaves only constitute the green tea, which is the primary source of catechins. EGCG has demonstrated poor oral absorption rates even considering a daily intake corresponding to 8–16 cups of green tea. Moreover, bioavailability investigations indicate that EGCG blood levels peak within 1.7 h after consumption (Lee et al., 2002; Chow et al., 2003). The EGCG has an absorbed plasma half-life of about 5 h, and most of the unaffected EGCG is excreted into the urine in a period of up to 8 h (Chow et al., 2003). Methylated metabolites seem to have demonstrated harboring longer half-lives than non-methylated compound. (Manach et al., 2005). In 2011, the European Food Safety Authority (EFSA) indicated that a cause-effect connection might not be demonstrated for a link between green tea catechins and the preservation of healthy blood low-density lipoprotein (LDL)-cholesterol rate (EFSA, 2011). Interestingly, five years later, Momose et al. unarguably identified that high diurnal EGCG doses ranging from 0.1 to 0.9 g/day administrated to individuals over 4 to 14 weeks promoted some decrease of LDL cholesterol (Momose et al., 2016). Undoubtedly, there are numerous benefits in using EGCG in the diet of a healthy individual and biochemically, and, specifically, cytologically effects have been studied in detail. Cytoprotective effects ascribed to this compound have been identified in the liver, kidney, heart, and lungs (Mezera et al., 2014; Kucera et al., 2015). Nuclear factor erythroid 2 -related factor 2 (NRF2) is a key regulator of the cellular antioxidant response. The induction of NRF2-dependent gene expression seems to be responsible for the protection exercised by this compound in addition to its direct antioxidant effect (Wang et al., 2015; Zhang et al., 2017). There are proofs of activation of glutathione (GSH) S-transferase other than superoxide dismutase and catalase (Augustyniak et al., 2005; Kim et al., 2015). EGCG is also responsible for inhibiting the expression of proinflammatory mediators such as cyclooxygenase 2 (COX-2), inducible nitric oxide synthase (iNOS), tumor necrosis factor α (TNFα) (Oz and Chen, 2008). It also acts in reducing the nuclear factor κB (NF κB) activity (Yang et al., 2001). Byun et al. found that some of the anti-inflammatory actions of EGCG can be attributed to the 67 kDa laminin receptor signaling (Hong Byun et al., 2010). In the setting of its anti-fibrotic effect, EGCG acts by inhibiting the expression of platelet-derived growth factor (PDGF) receptor-β, which is cell a surface tyrosine kinase receptor for members of the PDGF aiming to regulate cell proliferation, cellular differentiation, and cell growth (Zhen et al., 2007). The receptor for insulin-like growth factor 1 (IGF1R) is a transmembrane tyrosine kinase receptor that is activated by a hormone called IGF-1 and by a related hormone (IGF-2) (Sergi et al., 2019), as well as matrix metalloproteinase 2, which is involved in the breakdown of the extracellular matrix. These molecules are also regulated by EGCG in an inhibitory way (Zhen et al., 2007). In the liver, carbon tetrachloride (CCl4) can induce fibrosis and EGCG can prevent the development of hepatic fibrosis in rodents (both rats and mice) (Chen et al., 2004; Tipoe et al., 2010). The trigger of de novo synthesis of GSH in the hepatic stellate cells of rodent and the interruption of the transforming growth factor β (TGF β) signaling seem to be at the basis of its strong antifibrotic effect on the liver (Yumei et al., 2006). Moreover, EGCG ameliorates the liver tissue affected by non-alcoholic fat liver disease (NAFLD) and non-alcoholic steatohepatitis (NASH) using, for example, the ob/ob mice model, which is a mutant mouse that eats excessively due to mutations in the LEP (Leptin) gene (Fiorini et al., 2005; Chung et al., 2012). Even the autophagy, which is the regulated mechanism of the cell that removes dysfunctional or unnecessary components naturally (Chiu et al., 2017), is induced by EGCG (Zhou et al., 2014). Attenuation of ischemia/reperfusion injury was found in both healthy (Giakoustidis et al., 2010) and steatotic liver (Fiorini et al., 2005). Overall, the decrease of the risk of cardiovascular diseases seems to be due through its interference with PDGF signaling and, probably, through inhibition of vascular smooth muscle mitogenesis (Lee et al., 2013), by activation of endothelial nitric oxide synthase other than via a phosphatidylinositol 3-kinase/Akt-dependent pathway (Lorenz et al., 2004; Potenza et al., 2007), decreased hepatic VLDL secretion, and enhanced biliary secretion of cholesterol (Hirsova et al., 2013).
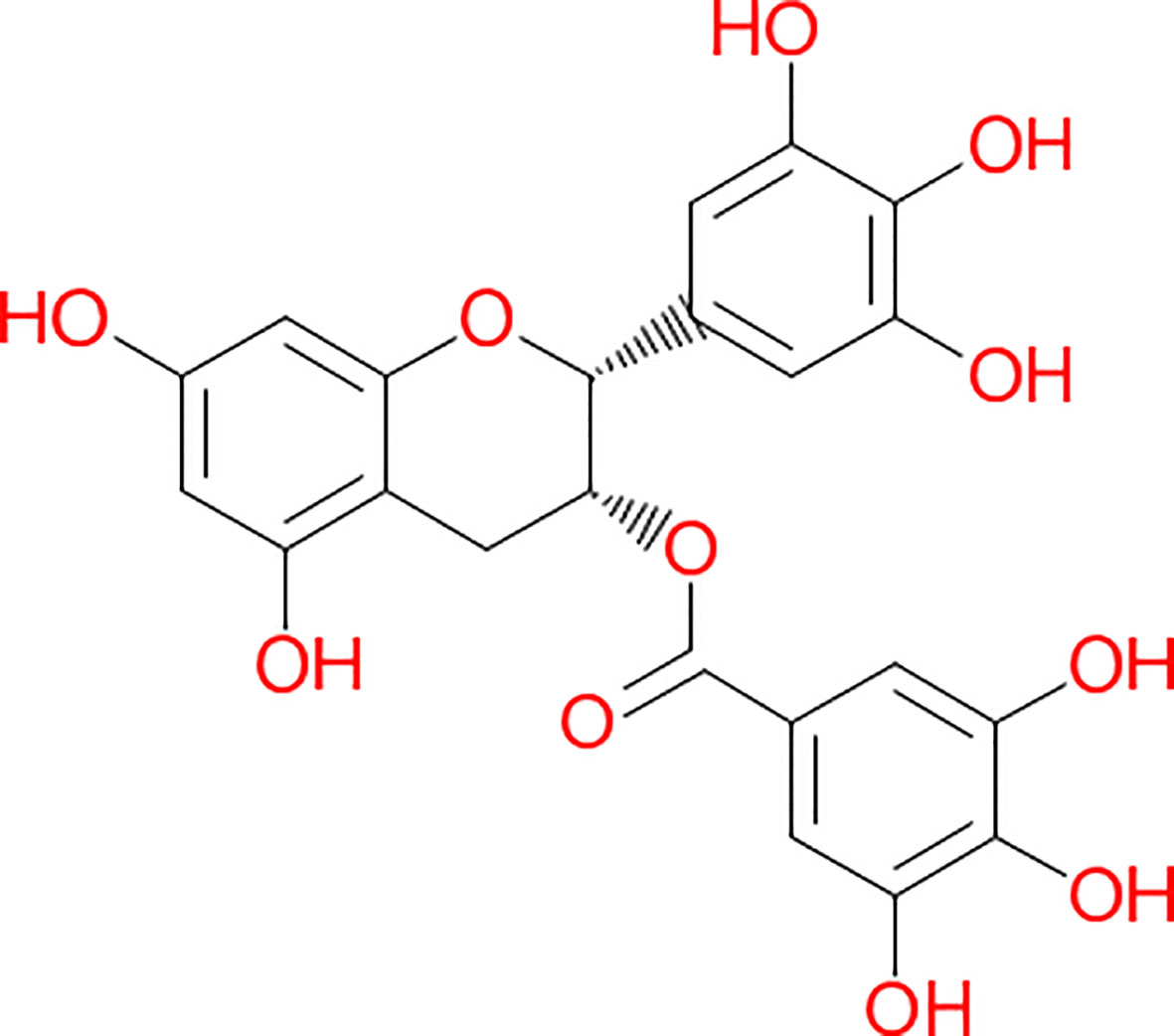
Figure 2 Chemical structure of (-)-Epigallocatechin-3-gallate. This structure was derived by the application of the software Chem4Word 3.0, United Kingdom.
Epigallocatechin Gallate Toxicity
In addition to the beneficial effects of GTPs, some studies have emphasized some harmful aspects (Murakami, 2014). In a 2018 review, it was suggested that excessive intake of EGCG might induce some hepatotoxicity (Hu et al., 2018). In the same year, the EFSA stated that a daily intake of 0.8 g might raise the risk of hepatocellular damage (EFSA, 2018). However, it seems that numerous individuals may have an idiosyncratic response to EGCG, and the degree of hepatotoxicity remains the most likely variable among individuals of the same age group. It has been suggested that hepatotoxicity is amplified by genetics, environmental factors, diet, as well as other factors, including age. The US Food and Drug Administration (USFDA) issued a series of reports between 2008 and 2017, targeting some administrative and quality assurance-associated violations by companies commercializing this compound (Wikipedia, 2019). An overdose with EGCG and/or green tea extract can lead to subcellular and cellular injuries (Schmidt et al., 2005; Galati et al., 2006). Pro-oxidative properties have been individuated when the compound is taken at high doses. This pro-oxidative induction is considered to be at the basis of the detrimental effects associated with EGCG. The pro-oxidative activities have been attributed to the catechol structures (ortho-diphenols). In pharmacology, an active moiety is the component of a molecule, which is considered to be responsible for either physiological or pharmacological action of a substance. The ortho-diphenol is, indeed, the functional moiety, which has the characteristic feature to prompt superoxide anion radical (O2-) from molecular oxygen (3O2) using an electrophilic o-quinone complement. Moreover, it has been found that an ester group in EGCG may be key in spawning biochemical interactions with the double lipid layer (Kajiya et al., 2001a; Kajiya et al., 2001b). These studies have evidenced a high affinity of this molecular compound for the cell membrane. GTPs covalently bind protein thiols. This covalent bond results from their conversion to an o-quinone counterpart. Elbling et al. showed that some cellular models may have EGCG-related pro-oxidative but not anti-oxidative properties raising crucial considerations on the diversity of cells, tissues, and organs (Elbling et al., 2005). Overall, the injury can be clarified by the prooxidant effect of high doses of EGCG (Nakagawa et al., 2004; Lambert et al., 2010) as well as by a decrease of both reduced GSH (Galati et al., 2006; Pohanka et al., 2011) and mitochondrial membrane potential (Galati et al., 2006). The single or combined effect induces hepatic necrosis accompanied by an infiltration of polymorphonucleate leukocytes (Goodin et al., 2006).
With regard to the mitochondria, these effects can be intensely argued in the literature. If some authors reported a protective effect of EGCG on mitochondria with a decrease of ROS formation (Jimenez-Lopez and Cederbaum, 2004; Valenti et al., 2013a), others found an inhibitory effect of EGCG on the activity of oxidative phosphorylation enzymes and respiration of mitochondria under ballooning state (Zheng and Ramirez, 2000; Weng et al., 2014). Recently, Kucera et al. (2015) tested the effect of EGCG on primary rat hepatocyte culture and liver mitochondria in permeabilized hepatocytes and found that EGCG promoted decreased ROS production on low doses, but increased ROS formation on high doses. The same authors also supported a decline in mitochondrial membrane potential in cells exposed to EGCG following a comparison with control cells. EGCG seems to determine the damage of the outer mitochondrial membrane and uncoupling of oxidative phosphorylation in permeabilized hepatocytes. EGCG in concentrations lower than 10 μmol/L has been, however, recognized as safe for hepatocytes in vitro.
Infantile and Pediatric Liver
Pre-school and school children may be exposed to numerous compounds that may have toxicity for the liver as the main organ. In consideration of the intricate cytoskeleton assembly and interaction with the extracellular environment the fetal liver development is crucial for a proper function of bile production and excretion and noxae to the developing liver may have dramatic consequences (Sergi et al., 2000a; Sergi et al., 2000b; Sergi et al., 2000c; Sergi et al., 2008). From birth through adolescence the developmental changes that occur in the liver's metabolic activity are remarkable and may contribute to the different sensitivity to toxins seen in infants, children, and adolescents (Pineiro-Carrero and Pineiro, 2004). The neonate has one fifth fewer hepatocytes than adult, and this amount is filled from birth through adult when the liver reaches its mature size (Karpen and Suchy, 2001; Wanless, 2002). In the neonate, the capacity to synthesize and excrete bile is immature. This situation gives the neonate a high susceptibility to significant cholestasis from toxic injury. Age-related sensitivity to molecular compounds or drugs is partly attributable to differences in metabolic activity between pediatric and adult liver. If toddlers and children are more resistant to acetaminophen hepatotoxicity when compared with adults, they are more susceptible to toxicity induced from valproic acid, a common anticonvulsant drug (Pineiro-Carrero and Pineiro, 2004). Acetaminophen toxicity is also different from children and adults. There are essential biochemical and subcellular differences between infants/children and adults. In the pediatric liver, sulfation predominates over glucuronidation, which leads to a decreased amount of toxic intermediates. Also, there is a higher capacity to synthesize GSH, thereby inactivating toxic metabolites of acetaminophen more effectively. The enzymatic efficiency is also different between pediatric and adult liver. The postnatal liver shows a decrease of thymidine kinase and ornithine decarboxylase, which is high during intrauterine life.
Conversely, other enzymes, such as fructose-1,6-diphosphatase and aspartate aminotransferase as well as uridine 5-diphosphate glucuronyl transferase, are mildly expressed in the fetus and increase postnatally only. The gray infant syndrome is characterized by the administration of chloramphenicol in an environment with decreased capacity of glucuronide conjugation as seen in the neonatal age (de Wildt et al., 1999). Numerous xenobiotics can be metabolized in the fetal liver, but the neonate exhibits a prolonged half-life for most drugs. It is the first year of life that shows significant and rapid maturation of the liver, and the most rapid elimination of drugs has been determined in school-age children and adolescents; thus, plasma clearance slows with age (Klinger, 2005). The cytochrome P450 system is a cluster of enzymes that control the rate of numerous molecular compounds. Most of these enzymes are located in the liver (hepatic microsomal enzymes) and gut. The main effect of the cytochrome P450 enzymes is to catalyze oxidation, which generally makes the substrate more hydrosoluble and able to be excreted by the kidneys. An alteration of the metabolism of drugs that are inactivated by the cytochrome P450 enzymes can be induced by either induction of these enzymes or the application of compounds that inhibit these enzymes with the final aim to increase the levels of the drug. The total cytochrome P450 (microsomal) content of the liver increases from 0.3 nmol/mg microsomal protein in the fetus/newborn to 0.5 in the full adult. Also, studies of transplacental transfer of aflatoxin B suggest that the developing human liver may be a very sensitive target for aflatoxin B damage (Hakkola et al., 1998; Hukkanen et al., 2002). Although very few data on the function of this enzyme with increasing age are available, it remains valid the concept that adult competence of the microsomal epoxide hydrolase activity is profoundly different between pediatric and adult liver, making infants and children without a doubt at increased risk of drug adverse outcome (Kearns, 1995; Leeder et al., 2010). Overall, it is hypothezable that EGCG may have a remarkable influence on the developing liver. The anti- and pro-oxidative properties of GTPs, as well as many other polyphenols, remain an intriguing field to investigate further. Substantially, both properties have been discovered to be linked to their daily doses, the variable co-presence of oxidants, the cued responsiveness of adaptation systems, and other factors, which are currently under intense investigation.
“Green Tea”-Associated Liver Disease (GTALD)
Overall, ingestion of high doses of GTPs and other related supplements has been associated with an increased risk of hepatocellular damage because of their pro-oxidative properties. The accurate pathways underlying this toxicity have not been fully elucidated. On the other side, it has been suggested that liver toxicity may exclusively occur with commercial supplements and not by oral consumption of green tea (Mazzanti et al., 2009; Mazzanti et al., 2015). As indicated above, the green tea extract sold in the United States and Western countries is often not the “real” extract from green tea. There is, indeed, an addition of EGCG into the extract of green tea. Thus, the EGCG contents in such GTE products are remarkably higher than the original extract of green tea, and a word of caution should be considered at this time. The impressive increase of traded nutraceuticals of the last two decades in Western countries may raise the awareness that some individuals may be particularly susceptible to EGCG and related compounds. Following the biochemical considerations detailed above, it seems that “green tea”-associated liver disease (GTALD) is mostly due to hepatocellular damage. In 15 out of the 19 cases reviewed by Mazzanti et al. (2015), a liver biopsy was performed. Liver histopathology showed mostly necrosis ranging from submassive, to massive or multifocal and accompanied by widespread inflammatory cellular infiltrates. The hepatocellular necrosis may evidence multilobular collapse of all three Rappaport hepatocytic zones (zone 1, periportal, zone 2 midlobular, and zone 3 pericentral). Livers with massive hepatic necrosis disclose a change in color and shape, exhibiting a shrinkage and wrinkling of the capsule. The cut surface of the liver is homogeneous with some mottling of the hepatic parenchyma. This aspect seems to be due to the intermingling of red (hyperemic) and yellow (steatotic) areas. In some organs, the necrotizing phenomenon may spare few regions of the liver parenchyma. This advance of disease may leave irregular islands of tissue or nodular clusters amongst collapsed parenchyma. Occasionally, cholestasis and mild fibrosis can be observed. Macroregenerative nodules need to be histologically investigated, although it does not seem that a hepatocellular carcinoma has been ever diagnosed in GTALD. According to Danan and Benichou (Danan and Benichou, 1993), the type of liver damage should be classified as hepatocellular in the majority of the cases (84.2%) and as cholestatic in two cases (10.5%). In Figures 3 and 4, is shown the liver histopathology with GTP-associated damage identified in a child whose nutrition was complemented with green tea extracts. It is important to reiterate at this time that pathology evidencing FHF is commonly non-specific and often points to viruses, autoimmunity, or congenital disorders of metabolism. A meticulous medical and drug history remains key representing an important task provided by pediatric gastroenterologists and hepatologists. The differential diagnosis between virus-triggered and toxic compounds-associated FHF relies on the first instance on a good medical history, indagating not only the pharmacological history but also the habit and the attitudes of the child or adolescent and both parents.
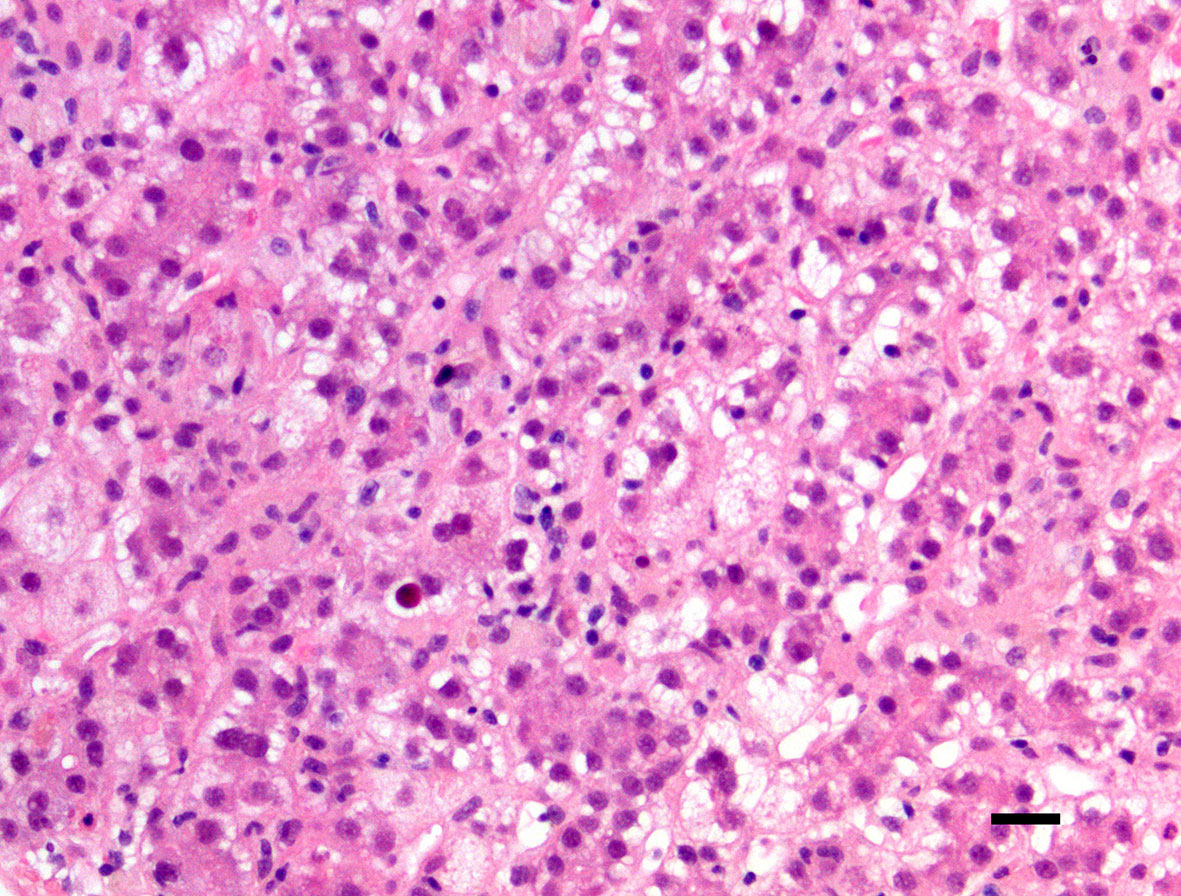
Figure 3 Central lobulus of the liver with hepatocyte ballooning, steatosis, and cell dropout (hematoxylin-eosin staining, ×200 original magnification, bar: 20 micrometers). The patient was a 15-year-old female child with hepatotoxicity due to administered green tea extracts.
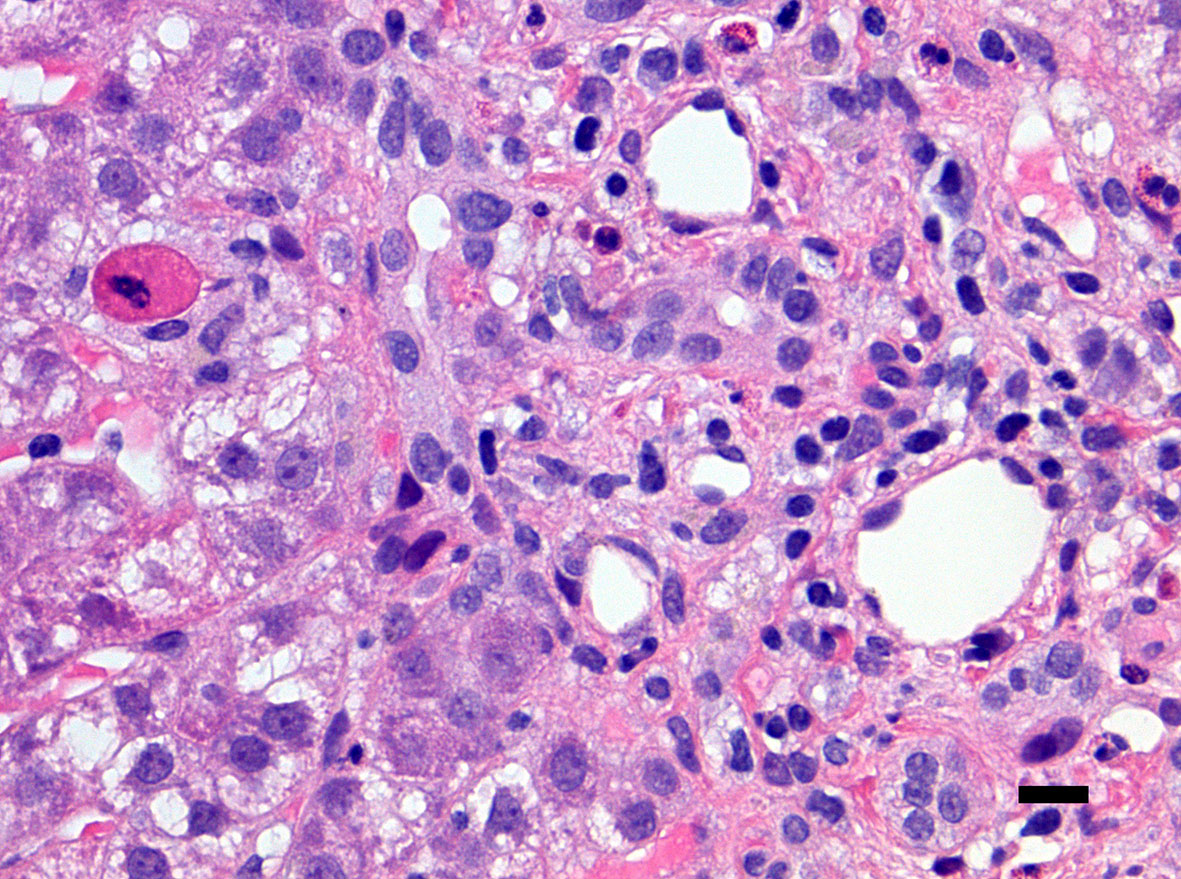
Figure 4 Close-up of a portal tract of the liver of the same patient presented in Figure 3 including neutrophils and eosinophils with an apoptotic hepatocyte close to the portal tract (left corner of the microphotograph). There is no ductular proliferation, some emperipolesis of inflammatory cells into the bile duct epithelium is noted (right side of the microphotograph) (hematoxylin-eosin staining, ×400 original magnification, bar: 25 micrometers).
Final Remarks and Conclusion
In internal medicine, cardiovascular disease, hypertension, hyperlipidemia, and diabetes mellitus are jointly known as “metabolic syndrome.” Also, there is evidence indicating that overweight and its related metabolic abnormalities are linked to an individual predisposition to develop some types of human epithelial malignancies, which score high in cancer statistics, including colorectal cancer and hepatocellular carcinoma (Shimizu et al., 2013; Shimizu et al., 2012). There is some pathophysiology linking overweight and tumorigenesis. It includes the emergence of insulin resistance, other than variations in the insulin-like growth factor-1 (IGF-1)/IGF-1 receptor (IGF-1R) axis, occurrence of adipocytokine imbalance, the state of chronic tissue inflammation, and the induction of cellular oxidative stress (Shimizu et al., 2012; Yang et al., 2018; Sergi et al., 2019). Green tea catechins have probably attracted considerable attention in the last two decades. The beneficial effects of improving metabolic abnormalities and preventing cancer growth are mostly based on tremendous interest worldwide. Although the use of nutraceuticals has been used in obese or overweighted adults, it should not be considered in childhood because of the potential adverse effect on immature or fast-developing liver cells. Hepatotoxicity and histologically confirmed hepatitis due to GTPs in children are probably very rare because of the occasional use, but these events may become more frequent in the future, because of the growing interest and/or change of the attitude toward botanicals. We must emphasize that liver failure remains a challenge for the pediatric gastroenterologist and nutritionist who need to stay knowledgeable in several fields of basic and clinical hepatology and hepatic pharmacology.
Author Contributions
CS conceptualized and designed the study, performed numerous clinical and surgical pathology procedures, collected data, carried out the histopathological analysis of innumerable liver samples with fulminant hepatic failure of various etiology, and revised the manuscript.
Funding
This research has been funded by the generosity of the Stollery Children’s Hospital Foundation and supporters of the Lois Hole Hospital for Women through the Women and Children’s Health Research Institute, Hubei Province Natural Science Funding for Hubei University of Technology (100-Talent Grant for Recruitment Program of Foreign Experts Total Funding: Digital PCR and NGS-based diagnosis for infection and oncology, 2017-2022), Österreichische Krebshilfe Tyrol (Krebsgesellschaft Tirol, Austrian Tyrolean Cancer Research Institute), Austrian Research Fund (Fonds zur Förderung der wissenschaftlichen Forschung, FWF), Canadian Foundation for Women’s Health, Cancer Research Society (von Willebrand factor gene expression in cancer cells), Canadian Institutes of Health Research (Omega-3 Fatty Acids for Treatment of Intestinal Failure Associated Liver Disease: A Translational Research Study), and the Saudi Cultural Bureau, Ottawa, Canada. The funders had no role in study design, data collection and analysis, decision to publish, or preparation of the manuscript.
Conflict of Interest
The author declares that the research was conducted in the absence of any commercial or financial relationships that could be construed as a potential conflict of interest.
References
Abdualmjid, R. J., Sergi, C. (2013). Hepatotoxic botanicals - an evidence-based systematic review. J. Pharm. Pharm. Sci. 16 (3), 376–404. doi: 10.18433/j36g6x
Anastassopoulou, C. G., Delladetsima, J. K., Anagnostopoulos, G., Katsoulidou, A., Papachristopoulos, A., Tassopoulos, N. C., et al. (2002). Fulminant hepatic failure in a pediatric patient with active GB virus C (GBV-C)/hepatitis G virus (HGV) infection. Hepatol. Res. 23 (2), 85–89. doi: 10.1016/s1386-6346(01)00166-8
Antonucci, R., Locci, C., Clemente, M. G., Chicconi, E., Antonucci, L. (2018). Vitamin D deficiency in childhood: old lessons and current challenges. J. Pediatr. Endocrinol. Metab. 31 (3), 247–260. doi: 10.1515/jpem-2017-0391
Augustyniak, A., Waszkiewicz, E., Skrzydlewska, E. (2005). Preventive action of green tea from changes in the liver antioxidant abilities of different aged rats intoxicated with ethanol. Nutrition 21 (9), 925–932. doi: 10.1016/j.nut.2005.01.006
Balentine, D. A., Wiseman, S. A., Bouwens, L. C. (1997). The chemistry of tea flavonoids. Crit. Rev. Food Sci. Nutr. 37 (8), 693–704. doi: 10.1080/10408399709527797
Bhagwat, S., Haytowitz, D. B., Holden, J. M. (2011). USDA database for the flavonoid content of selected foods, release 3. U.S. Department of Agriculture. September 2011. 156.
Brunt, E. M. (2004). Nonalcoholic steatohepatitis. Semin. Liver Dis. 24 (1), 3–20. doi: 10.1055/s-2004-823098
Bunchorntavakul, C., Reddy, K. R. (2013). Review article: herbal and dietary supplement hepatotoxicity. Aliment. Pharmacol. Ther. 37 (1), 3–17. doi: 10.1111/apt.12109
Butler, E. M., Suhag, A., Hong, Y., Liang, L., Gong, C., Xiong, F., et al. (2019). Parental perceptions of obesity in school children and subsequent action. Child Obes. 15 (7), 459–467. doi: 10.1089/chi.2018.0338
Chen, J. H., Tipoe, G. L., Liong, E. C., So, H. S., Leung, K. M., Tom, W. M., et al. (2004). Green tea polyphenols prevent toxin-induced hepatotoxicity in mice by down-regulating inducible nitric oxide-derived prooxidants. Am. J. Clin. Nutr. 80 (3), 742–751. doi: 10.1093/ajcn/80.3.742
Chiu, B., Jantuan, E., Shen, F., Chiu, B., Sergi, C. (2017). Autophagy-inflammasome interplay in heart failure: a systematic review on basics, pathways, and therapeutic perspectives. Ann. Clin. Lab. Sci. 47 (3), 243–252.
Chow, H. H., Cai, Y., Hakim, I. A., Crowell, J. A., Shahi, F., Brooks, C. A., et al. (2003). Pharmacokinetics and safety of green tea polyphenols after multiple-dose administration of epigallocatechin gallate and polyphenon E in healthy individuals. Clin. Cancer Res. 9 (9), 3312–3319.
Chung, M. Y., Park, H. J., Manautou, J. E., Koo, S. I., Bruno, R. S. (2012). Green tea extract protects against nonalcoholic steatohepatitis in ob/ob mice by decreasing oxidative and nitrative stress responses induced by proinflammatory enzymes. J. Nutr. Biochem. 23 (4), 361–367. doi: 10.1016/j.jnutbio.2011.01.001
Cicero, A. F. G., Colletti, A. (2018). An update on the safety of nutraceuticals and effects on lipid parameters. Expert Opin. Drug Saf. 17 (3), 303–313. doi: 10.1080/14740338.2018.1429404
Cicero, A. F., Ferroni, A., Ertek, S. (2012). Tolerability and safety of commonly used dietary supplements and nutraceuticals with lipid-lowering effects. Expert Opin. Drug Saf. 11 (5), 753–766. doi: 10.1517/14740338.2012.705827
Cline, J. C. (2015). Nutritional aspects of detoxification in clinical practice. Altern. Ther. Health Med. 21 (3), 54–62.
Committee On B. (2013). Conflicts between religious or spiritual beliefs and pediatric care: informed refusal, exemptions, and public funding. Pediatrics 132 (5), 962–965. doi: 10.1542/peds.2013-2716
Danan, G., Benichou, C. (1993). Causality assessment of adverse reactions to drugs–I. A novel method based on the conclusions of international consensus meetings: application to drug-induced liver injuries. J. Clin. Epidemiol. 46 (11), 1323–1330. doi: 10.1016/0895-4356(93)90101-6
de Boer, Y. S., Sherker, A. H. (2017). Herbal and dietary supplement-induced liver injury. Clin. Liver Dis. 21 (1), 135–149. doi: 10.1016/j.cld.2016.08.010
de Wildt, S. N., Kearns, G. L., Leeder, J. S., van den Anker, J. N. (1999). Glucuronidation in humans. Pharmacogenetic and developmental aspects. Clin. Pharmacokinet. 36 (6), 439–452. doi: 10.2165/00003088-199936060-00005
Dong, Y., Lau, P. W. C., Dong, B., Zou, Z., Yang, Y., Wen, B., et al. (2019). Trends in physical fitness, growth, and nutritional status of Chinese children and adolescents: a retrospective analysis of 1.5 million students from six successive national surveys between 1985 and 2014. Lancet Child Adolesc. Health. 3 (12), 871–880. doi: 10.1016/S2352-4642(19)30302-5.
EFSA. (2011). Scientific Opinion on the substantiation of health claims related to Camellia sinensis (L.) Kuntze (tea), including catechins in green tea, and improvement of endothelium-dependent vasodilation (ID 1106, 1310), maintenance of normal blood pressure. EFSA J. 9 (4), 2055. doi: 10.2903/j.efsa.2011.2055
EFSA. (2018). Scientific Opinion on the safety of green tea catechins. EFSA J. 16 (4), 4239. doi: 10.2903/j.efsa.2018.5239
El-Beshbishy, H. A. (2005). Hepatoprotective effect of green tea (Camellia sinensis) extract against tamoxifen-induced liver injury in rats. J. Biochem. Mol. Biol. 38 (5), 563–570. doi: 10.5483/bmbrep.2005.38.5.563
Elbling, L., Weiss, R. M., Teufelhofer, O., Uhl, M., Knasmueller, S., Schulte-Hermann, R., et al. (2005). Green tea extract and (-)-epigallocatechin-3-gallate, the major tea catechin, exert oxidant but lack antioxidant activities. FASEB J. 19 (7), 807–809. doi: 10.1096/fj.04-2915fje
Fargion, S., Bissoli, F., Fracanzani, A. L., Suigo, E., Sergi, C., Taioli, E., et al. (1996a). No association between genetic hemochromatosis and alpha1-antitrypsin deficiency. Hepatology 24 (5), 1161–1164. doi: 10.1002/hep.510240530
Fargion, S., Sergi, C., Bissoli, F., Fracanzani, A. L., Suigo, E., Carazzone, A., et al. (1996b). Lack of association between porphyria cutanea tarda and alpha 1-antitrypsin deficiency. Eur. J. Gastroenterol. Hepatol. 8 (4), 387–391. doi: 10.1097/00042737-199604000-00018
Fernandez, J., Navascues, C., Albines, G., Franco, L., Pipa, M., Rodriguez, M. (2014). Three cases of liver toxicity with a dietary supplement intended to stop hair loss. Rev. Esp. Enferm. Dig. 106 (8), 552–555.
Fiorini, R. N., Donovan, J. L., Rodwell, D., Evans, Z., Cheng, G., May, H. D., et al. (2005). Short-term administration of (-)-epigallocatechin gallate reduces hepatic steatosis and protects against warm hepatic ischemia/reperfusion injury in steatotic mice. Liver Transpl. 11 (3), 298–308. doi: 10.1002/lt.20348
Floyd, C. N. (2009). Hyperkalaemia. Time to flag up “detox” drinks? BMJ 339, b4824. doi: 10.1136/bmj.b4824
Galati, G., Lin, A., Sultan, A. M., O'Brien, P. J. (2006). Cellular and in vivo hepatotoxicity caused by green tea phenolic acids and catechins. Free Radic. Biol. Med. 40 (4), 570–580. doi: 10.1016/j.freeradbiomed.2005.09.014
Gao, Z., Pope, Z. C., Lee, J. E., Quan, M. (2019). Effects of active video games on children's psychosocial beliefs and school day energy expenditure. J. Clin. Med. 8 (9), 1268. doi: 10.3390/jcm8091268
Garoufalia, Z., Prodromidou, A., Machairas, N., Kostakis, I. D., Stamopoulos, P., Zavras, N., et al. (2019). Liver transplantation for Wilson's disease in non-adult patients: a systematic review. Transplant. Proc. 51 (2), 443–445. doi: 10.1016/j.transproceed.2019.01.017
Giakoustidis, D. E., Giakoustidis, A. E., Iliadis, S., Koliakou, K., Antoniadis, N., Kontos, N., et al. (2010). Attenuation of liver ischemia/reperfusion induced apoptosis by epigallocatechin-3-gallate via down-regulation of NF-kappaB and c-Jun expression. J. Surg. Res. 159 (2), 720–728. doi: 10.1016/j.jss.2008.08.038
Goodin, M. G., Bray, B. J., Rosengren, R. J. (2006). Sex- and strain-dependent effects of epigallocatechin gallate (EGCG) and epicatechin gallate (ECG) in the mouse. Food Chem. Toxicol. 44 (9), 1496–1504. doi: 10.1016/j.fct.2006.04.012
Grek, A., Arasi, L. (2016). Acute liver failure. AACN Adv. Crit. Care 27 (4), 420–429. doi: 10.4037/aacnacc2016324
Hakkola, J., Pelkonen, O., Pasanen, M., Raunio, H. (1998). Xenobiotic-metabolizing cytochrome P450 enzymes in the human feto-placental unit: role in intrauterine toxicity. Crit. Rev. Toxicol. 28 (1), 35–72. doi: 10.1080/10408449891344173
Hamden, K., Carreau, S., Marki, F. A., Masmoudi, H., El Feki, A. (2008). Positive effects of green tea on hepatic dysfunction, lipid peroxidation and antioxidant defence depletion induced by cadmium. Biol. Res. 41 (3), 331–339. doi: /S0716-97602008000300009
Han, X. D., Zhang, Y. Y., Wang, K. L., Huang, Y. P., Yang, Z. B., Liu, Z. (2017). The involvement of Nrf2 in the protective effects of (-)-Epigallocatechin-3-gallate (EGCG) on NaAsO2-induced hepatotoxicity. Oncotarget 8 (39), 65302–65312. doi: 10.18632/oncotarget.18582
Hartman, C., Shamir, R. (2012). Nutrition and growth: highlights from the first international meeting. Expert Rev. Endocrinol. Metab. 7 (4), 407–410. doi: 10.1586/eem.12.31
Hicks, J., Barrish, J., Zhu, S. H. (2001). Neonatal syncytial giant cell hepatitis with paramyxoviral-like inclusions. Ultrastruct. Pathol. 25 (1), 65–71. doi: 10.1080/019131201300004708
Hirsova, P., Karlasova, G., Dolezelova, E., Cermanova, J., Zagorova, M., Kadova, Z., et al. (2013). Cholestatic effect of epigallocatechin gallate in rats is mediated via decreased expression of Mrp2. Toxicology 303, 9–15. doi: 10.1016/j.tox.2012.10.018
Hong Byun, E., Fujimura, Y., Yamada, K., Tachibana, H. (2010). TLR4 signaling inhibitory pathway induced by green tea polyphenol epigallocatechin-3-gallate through 67-kDa laminin receptor. J. Immunol. 185 (1), 33–45. doi: 10.4049/jimmunol.0903742
Hu, J., Webster, D., Cao, J., Shao, A. (2018). The safety of green tea and green tea extract consumption in adults - Results of a systematic review. Regul. Toxicol. Pharmacol. 95, 412–433. doi: 10.1016/j.yrtph.2018.03.019
Hukkanen, J., Pelkonen, O., Hakkola, J., Raunio, H. (2002). Expression and regulation of xenobiotic-metabolizing cytochrome P450 (CYP) enzymes in human lung. Crit. Rev. Toxicol. 32 (5), 391–411. doi: 10.1080/20024091064273
Ishii, T., Ichikawa, T., Minoda, K., Kusaka, K., Ito, S., Suzuki, Y., et al. (2011). Human serum albumin as an antioxidant in the oxidation of (-)-epigallocatechin gallate: participation of reversible covalent binding for interaction and stabilization. Biosci. Biotechnol. Biochem. 75 (1), 100–106. doi: 10.1271/bbb.100600
James, J. A., Clark, C., Ward, P. S. (1985). Screening Rastafarian children for nutritional rickets. Br. Med. J. (Clin Res. Ed) 290 (6472), 899–900. doi: 10.1136/bmj.290.6472.899
Jia, P., Luo, M., Li, Y., Zheng, J. S., Xiao, Q., Luo, J. (2019). Fast-food restaurant, unhealthy eating, and childhood obesity: a systematic review and meta-analysis. Obes. Rev. doi: 10.1111/obr.12944
Jimenez-Lopez, J. M., Cederbaum, A. I. (2004). Green tea polyphenol epigallocatechin-3-gallate protects HepG2 cells against CYP2E1-dependent toxicity. Free Radic. Biol. Med. 36 (3), 359–370. doi: 10.1016/j.freeradbiomed.2003.11.016
Jimenez-Saenz, M., Martinez-Sanchez Mdel, C. (2006). Acute hepatitis associated with the use of green tea infusions. J. Hepatol. 44 (3), 616–617. doi: 10.1016/j.jhep.2005.11.041
Kajiya, K., Ichiba, M., Kuwabara, M., Kumazawa, S., Nakayama, T. (2001a). Role of lipophilicity and hydrogen peroxide formation in the cytotoxicity of flavonols. Biosci. Biotechnol. Biochem. 65 (5), 1227–1229. doi: 10.1271/bbb.65.1227
Kajiya, K., Kumazawa, S., Nakayama, T. (2001b). Steric effects on interaction of tea catechins with lipid bilayers. Biosci. Biotechnol. Biochem. 65 (12), 2638–2643. doi: 10.1271/bbb.65.2638
Karpen, S. J., Suchy, F. J. (2001). “Structural and functional development of the liver,” in Liver Disease in Children, 2nd ed. Eds. Suchy, F. J., Sokol, R. J., Balistreri, W. F.. (Philadelphia, PA: Lippincot Williams & Wilkins), 3–21 .
Karvellas, C. J., Tillman, H., Leung, A. A., Lee, W. M., Schilsky, M. L., Hameed, B., et al. (2016). Acute liver injury and acute liver failure from mushroom poisoning in North America. Liver Int. 36 (7), 1043–1050. doi: 10.1111/liv.13080
Kaviarasan, S., Ramamurthy, N., Gunasekaran, P., Varalakshmi, E., Anuradha, C. V. (2007). Epigallocatechin-3-gallate(-)protects Chang liver cells against ethanol-induced cytotoxicity and apoptosis. Basic Clin. Pharmacol. Toxicol. 100 (3), 151–156. doi: 10.1111/j.1742-7843.2006.00036.x
Kearns, G. L. (1995). Pharmacogenetics and development: are infants and children at increased risk for adverse outcomes? Curr. Opin. Pediatr. 7 (2), 220–233.
Kim, J. Y., Choi, J. Y., Lee, H. J., Byun, C. J., Park, J. H., Park, J. H., et al. (2015). The green tea component (-)-epigallocatechin-3-gallate sensitizes primary endothelial cells to arsenite-induced apoptosis by decreasing c-Jun N-terminal kinase-mediated catalase activity. PloS One 10 (9), e0138590. doi: 10.1371/journal.pone.0138590
Klinger, W. (2005). Developmental pharmacology and toxicology: biotransformation of drugs and other xenobiotics during postnatal development. Eur. J. Drug Metab. Pharmacokinet. 30 (1-2), 3–17. doi: 10.1007/BF03226403
Kodali, S., McGuire, B. M. (2015). Diagnosis and management of hepatic encephalopathy in fulminant hepatic failure. Clin. Liver Dis. 19 (3), 565–576. doi: 10.1016/j.cld.2015.04.006
Krautkramer, C. J. (2005). Pediatric resuscitation: questioning DNAR legitimacy and offering an alternative decision-making model. Am. J. Bioeth. 5 (1), 86–88. doi: 10.1080/152651690927917. author reply W19-21.
Kucera, O., Mezera, V., Moravcova, A., Endlicher, R., Lotkova, H., Drahota, Z., et al. (2015). In vitro toxicity of epigallocatechin gallate in rat liver mitochondria and hepatocytes. Oxid. Med. Cell Longev. 2015, 476180. doi: 10.1155/2015/476180
Kurien, B. T., Scofield, R. H. (2010). Time to flag up “detox” drinks? No need to flag up potassium. BMJ 340, c1180. doi: 10.1136/bmj.c1180
Lambert, J. D., Kennett, M. J., Sang, S., Reuhl, K. R., Ju, J., Yang, C. S. (2010). Hepatotoxicity of high oral dose (-)-epigallocatechin-3-gallate in mice. Food Chem. Toxicol. 48 (1), 409–416. doi: 10.1016/j.fct.2009.10.030
LeBlanc, A. G., Chaput, J. P. (2019). Urbanisation and fitness: worrying trends from China. Lancet Child Adolesc. Health 3 (12), 837–839. doi: 10.1016/S2352-4642(19)30303-7
Lee, M. J., Maliakal, P., Chen, L., Meng, X., Bondoc, F. Y., Prabhu, S., et al. (2002). Pharmacokinetics of tea catechins after ingestion of green tea and (-)-epigallocatechin-3-gallate by humans: formation of different metabolites and individual variability. Cancer Epidemiol. Biomarkers Prev. 11 (10 Pt 1), 1025–1032.
Lee, M. H., Kwon, B. J., Koo, M. A., You, K. E., Park, J. C. (2013). Mitogenesis of vascular smooth muscle cell stimulated by platelet-derived growth factor-bb is inhibited by blocking of intracellular signaling by epigallocatechin-3-O-gallate. Oxid. Med. Cell Longev. 2013, 827905. doi: 10.1155/2013/827905
Leeder, J. S., Kearns, G. L., Spielberg, S. P., van den Anker, J. (2010). Understanding the relative roles of pharmacogenetics and ontogeny in pediatric drug development and regulatory science. J. Clin. Pharmacol. 50 (12), 1377–1387. doi: 10.1177/0091270009360533
Leung, A. K., Hon, K. L., Leong, K. F., Sergi, C. M. (2018). Measles: a disease often forgotten but not gone. Hong Kong Med. J. 24 (5), 512–520. doi: 10.12809/hkmj187470
Leung, A. K. C., Sergi, C. M., Lam, J. M., Leong, K. F. (2019). Gianotti-Crosti syndrome (papular acrodermatitis of childhood) in the era of a viral recrudescence and vaccine opposition. World J. Pediatr. 15 (6), 521–527.doi: 10.1007/s12519-019-00269-9
Li, Y. M., Chan, H. Y., Huang, Y., Chen, Z. Y. (2007). Green tea catechins upregulate superoxide dismutase and catalase in fruit flies. Mol. Nutr. Food Res. 51 (5), 546–554. doi: 10.1002/mnfr.200600238
Licata, A. (2016). Adverse drug reactions and organ damage: the liver. Eur. J. Intern. Med. 28, 9–16. doi: 10.1016/j.ejim.2015.12.017
Lorenz, M., Wessler, S., Follmann, E., Michaelis, W., Dusterhoft, T., Baumann, G., et al. (2004). A constituent of green tea, epigallocatechin-3-gallate, activates endothelial nitric oxide synthase by a phosphatidylinositol-3-OH-kinase-, cAMP-dependent protein kinase-, and Akt-dependent pathway and leads to endothelial-dependent vasorelaxation. J. Biol. Chem. 279 (7), 6190–6195. doi: 10.1074/jbc.M309114200
Manach, C., Williamson, G., Morand, C., Scalbert, A., Remesy, C. (2005). Bioavailability and bioefficacy of polyphenols in humans. I. Review of 97 bioavailability studies. Am. J. Clin. Nutr. 81 (1 Suppl), 230S–242S. doi: 10.1093/ajcn/81.1.230S
Mazzanti, G., Menniti-Ippolito, F., Moro, P. A., Cassetti, F., Raschetti, R., Santuccio, C., et al. (2009). Hepatotoxicity from green tea: a review of the literature and two unpublished cases. Eur. J. Clin. Pharmacol. 65 (4), 331–341. doi: 10.1007/s00228-008-0610-7
Mazzanti, G., Di Sotto, A., Vitalone, A. (2015). Hepatotoxicity of green tea: an update. Arch. Toxicol. 89 (8), 1175–1191. doi: 10.1007/s00204-015-1521-x
Mendizabal, M., Silva, M. O. (2016). Liver transplantation in acute liver failure: A challenging scenario. World J. Gastroenterol. 22 (4), 1523–1531. doi: 10.3748/wjg.v22.i4.1523
Meng, Q., Velalar, C. N., Ruan, R. (2008). Regulating the age-related oxidative damage, mitochondrial integrity, and antioxidative enzyme activity in Fischer 344 rats by supplementation of the antioxidant epigallocatechin-3-gallate. Rejuvenation Res. 11 (3), 649–660. doi: 10.1089/rej.2007.0645
Mezera, V., Kucera, O., Moravcova, A., Peterova, E., Cervinkova, Z. (2014). The effect of epigallocatechin gallate on hepatocytes isolated from normal and partially hepatectomized rats. Can. J. Physiol. Pharmacol. 92 (6), 512–517. doi: 10.1139/cjpp-2014-0069
Momose, Y., Maeda-Yamamoto, M., Nabetani, H. (2016). Systematic review of green tea epigallocatechin gallate in reducing low-density lipoprotein cholesterol levels of humans. Int. J. Food Sci. Nutr. 67 (6), 606–613. doi: 10.1080/09637486.2016.1196655
Monobe, M., Ema, K., Tokuda, Y., Maeda-Yamamoto, M. (2014). Green tea catechin induced phagocytosis can be blocked by catalase and an inhibitor of transient receptor potential melastatin 2 (TRPM2). Cytotechnology 66 (4), 561–566. doi: 10.1007/s10616-013-9618-7
Murakami, A. (2014). Dose-dependent functionality and toxicity of green tea polyphenols in experimental rodents. Arch. Biochem. Biophys. 557, 3–10. doi: 10.1016/j.abb.2014.04.018
Nakagawa, H., Hasumi, K., Woo, J. T., Nagai, K., Wachi, M. (2004). Generation of hydrogen peroxide primarily contributes to the induction of Fe(II)-dependent apoptosis in Jurkat cells by (-)-epigallocatechin gallate. Carcinogenesis 25 (9), 1567–1574. doi: 10.1093/carcin/bgh168
Navarro, V. J., Khan, I., Bjornsson, E., Seeff, L. B., Serrano, J., Hoofnagle, J. H. (2017). Liver injury from herbal and dietary supplements. Hepatology 65 (1), 363–373. doi: 10.1002/hep.28813
Noor, A., Panwala, A., Forouhar, F., Wu, G. Y. (2018). Hepatitis caused by herpes viruses: a review. J. Dig. Dis. 19 (8), 446–455. doi: 10.1111/1751-2980.12640
O'Grady, J. G., Schalm, S. W., Williams, R. (1993). Acute liver failure: redefining the syndromes. Lancet 342 (8866), 273–275. doi: 10.1016/0140-6736(93)91818-7
O'Mathuna, D. P., Lang, K. (2008). Medicine vs. prayer: the case of Kara Neumann. Pediatr. Nurs. 34 (5), 413–416.
Oz, H. S., Chen, T. S. (2008). Green-tea polyphenols downregulate cyclooxygenase and Bcl-2 activity in acetaminophen-induced hepatotoxicity. Dig. Dis. Sci. 53 (11), 2980–2988. doi: 10.1007/s10620-008-0239-5
Pignatelli, P., Basili, S. (2010). Nutraceuticals in the early infancy. Cardiovasc. Ther. 28 (4), 236–245. doi: 10.1111/j.1755-5922.2010.00194.x
Pohanka, M., Sobotka, J., Stetina, R. (2011). Sulfur mustard induced oxidative stress and its alteration by epigallocatechin gallate. Toxicol. Lett. 201 (2), 105–109. doi: 10.1016/j.toxlet.2010.12.011
Potenza, M. A., Marasciulo, F. L., Tarquinio, M., Tiravanti, E., Colantuono, G., Federici, A., et al. (2007). EGCG, a green tea polyphenol, improves endothelial function and insulin sensitivity, reduces blood pressure, and protects against myocardial I/R injury in SHR. Am. J. Physiol. Endocrinol. Metab. 292 (5), E1378–E1387. doi: 10.1152/ajpendo.00698.2006
Rietveld, A., Wiseman, S. (2003). Antioxidant effects of tea: evidence from human clinical trials. J. Nutr. 133 (10), 3285S–3292S. doi: 10.1093/jn/133.10.3285S
Rosenblatt, R., Brown, R. S., Jr. (2018). Nonviral or drug-induced etiologies of acute liver failure. Clin. Liver Dis. 22 (2), 347–360. doi: 10.1016/j.cld.2018.01.008
Sanders, T. A., Reddy, S. (1994). Vegetarian diets and children. Am. J. Clin. Nutr. 59 (5 Suppl), 1176S–1181S. doi: 10.1093/ajcn/59.5.1176S
Sanders, T. A. (1995). Vegetarian diets and children. Pediatr. Clin. North Am. 42 (4), 955–965. doi: 10.1016/s0031-3955(16)40024-6
Schmidt, M., Schmitz, H. J., Baumgart, A., Guedon, D., Netsch, M. I., Kreuter, M. H., et al. (2005). Toxicity of green tea extracts and their constituents in rat hepatocytes in primary culture. Food Chem. Toxicol. 43 (2), 307–314. doi: 10.1016/j.fct.2004.11.001
Sergi, C., Linderkamp, O. (2001). Pathological case of the month: classic rickets in a setting of significant psychosocial deprivation. Arch. Pediatr. Adolesc. Med. 155 (8), 967–968. doi: 10.1001/archpedi.155.8.967
Sergi, C., Shen, F., Liu, S. M. (2019). Insulin/IGF-1R, SIRT1, and FOXOs pathways-an intriguing interaction platform for bone and osteosarcoma. Front. Endocrinol. (Lausanne) 10, 93. doi: 10.3389/fendo.2019.00093
Sergi, C., Goeser, T., Otto, G., Otto, H. F., Hofmann, W. J. (1996). A rapid and highly specific technique to detect hepatitis C RNA in frozen sections of liver. J. Clin. Pathol. 49 (5), 369–372. doi: 10.1136/jcp.49.5.369
Sergi, C., Jundt, K., Seipp, S., Goeser, T., Theilmann, L., Otto, G., et al. (1998). The distribution of HBV, HCV and HGV among livers with fulminant hepatic failure of different aetiology. J. Hepatol. 29 (6), 861–871. doi: S0168-8278(98)80112-8
Sergi, C., Beedgen, B., Linderkamp, O., Hofmann, W. J. (1999). Fatal course of veno-occlusive disease of the liver (endophlebitis hepatica obliterans) in a preterm infant. Pathol. Res. Pract. 195 (12), 847–851. doi: 10.1016/S0344-0338(99)80108-3
Sergi, C., Adam, S., Kahl, P., Otto, H. F. (2000a). Study of the malformation of ductal plate of the liver in Meckel syndrome and review of other syndromes presenting with this anomaly. Pediatr. Dev. Pathol. 3 (6), 568–583. doi: 10.1007/s100240010104
Sergi, C., Adam, S., Kahl, P., Otto, H. F. (2000b). The remodeling of the primitive human biliary system. Early Hum. Dev. 58 (3), 167–178. doi: 10.1016/s0378-3782(00)00065-7
Sergi, C., Kahl, P., Otto, H. F. (2000c). Contribution of apoptosis and apoptosis-related proteins to the malformation of the primitive intrahepatic biliary system in Meckel syndrome. Am. J. Pathol. 156 (5), 1589–1598. doi: 10.1016/S0002-9440(10)65031-6
Sergi, C., Arnold, J. C., Rau, W., Otto, H. F., Hofmann, W. J. (2002). Single nucleotide insertion in the 5'-untranslated region of hepatitis C virus with clearance of the viral RNA in a liver transplant recipient during acute hepatitis B virus superinfection. Liver 22 (1), 79–82. doi: 10.1046/j.0106-9543.2001.00001.x
Sergi, C., Benstz, J., Feist, D., Nutzenadel, W., Otto, H. F., Hofmann, W. J. (2008). Bile duct to portal space ratio and ductal plate remnants in liver disease of infants aged less than 1 year. Pathology 40 (3), 260–267. doi: 10.1080/00313020801911538
Sergi, C. M. (2019). “Genetics of biliary atresia: a work in progress for a disease with an unavoidable sequela into liver cirrhosis following failure of hepatic portoenterostomy,” in Liver Cirrhosis - Debates and Current Challenges. Ed. Tsoulfas, G.. (London, United Kingdom: IntechOpen), pp. 21–40.
Shaw, O. E. F., Yager, J. Y. (2019). Preventing childhood and lifelong disability: maternal dietary supplementation for perinatal brain injury. Pharmacol. Res. 139, 228–242. doi: 10.1016/j.phrs.2018.08.022
Shen, Z. Y., Wang, Z. F., Zhu, Z. J., Zang, Y. J., Zheng, H., Deng, Y. L., et al. (2008). Pediatric liver transplantation in 31 consecutive children. Chin. Med. J. (Engl) 121 (20), 2001–2003.
Shimizu, M., Kubota, M., Tanaka, T., Moriwaki, H. (2012). Nutraceutical approach for preventing obesity-related colorectal and liver carcinogenesis. Int. J. Mol. Sci. 13 (1), 579–595. doi: 10.3390/ijms13010579
Shimizu, M., Tanaka, T., Moriwaki, H. (2013). Obesity and hepatocellular carcinoma: targeting obesity-related inflammation for chemoprevention of liver carcinogenesis. Semin. Immunopathol. 35 (2), 191–202. doi: 10.1007/s00281-012-0336-6
Signorelli, C. (2019). Forty years (1978–2018) of vaccination policies in Italy. Acta BioMed. 90 (1), 127–133. doi: 10.23750/abm.v90i1.7900
Spearman, C. W., McCulloch, M., Millar, A. J., Burger, H., Numanoglu, A., Goddard, E., et al. (2005). Liver transplantation for children: red cross children's hospital experience. Transplant. Proc. 37 (2), 1134–1137. doi: 10.1016/j.transproceed.2004.12.285
Stravitz, R. T., Lee, W. M. (2019). Acute liver failure. Lancet 394 (10201), 869–881. doi: 10.1016/S0140-6736(19)31894-X
Tipoe, G. L., Leung, T. M., Liong, E. C., Lau, T. Y., Fung, M. L., Nanji, A. A. (2010). Epigallocatechin-3-gallate (EGCG) reduces liver inflammation, oxidative stress and fibrosis in carbon tetrachloride (CCl4)-induced liver injury in mice. Toxicology 273 (1-3), 45–52. doi: 10.1016/j.tox.2010.04.014
Valenti, D., De Rasmo, D., Signorile, A., Rossi, L., de Bari, L., Scala, I., et al. (2013a). Epigallocatechin-3-gallate prevents oxidative phosphorylation deficit and promotes mitochondrial biogenesis in human cells from subjects with Down's syndrome. Biochim. Biophys. Acta 1832 (4), 542–552. doi: 10.1016/j.bbadis.2012.12.011
Valenti, D., de Bari, L., Manente, G. A., Rossi, L., Mutti, L., Moro, L., et al. (2013b). Negative modulation of mitochondrial oxidative phosphorylation by epigallocatechin-3 gallate leads to growth arrest and apoptosis in human malignant pleural mesothelioma cells. Biochim. Biophys. Acta 1832 (12), 2085–2096. doi: 10.1016/j.bbadis.2013.07.014
Wang, D., Wang, Y., Wan, X., Yang, C. S., Zhang, J. (2015). Green tea polyphenol (-)-epigallocatechin-3-gallate triggered hepatotoxicity in mice: responses of major antioxidant enzymes and the Nrf2 rescue pathway. Toxicol. Appl. Pharmacol. 283 (1), 65–74. doi: 10.1016/j.taap.2014.12.018
Wanless, I. R. (2002). “Anatomy, histology, embryology, and developmental anomalies of the liver,” in Sleisenger & Fordtran"s Gastrointestinal and Liver Disease, 7th ed. Eds. Feldman, M., Friedman, L. S., Sleisenger., M. H. (Philadelphia, PA: WB Saunders), 1195–1201 .
Ward, W. E., Chilibeck, P. D., Comelli, E. M., Duncan, A. M., Phillips, S. M., Robinson, L. E., et al. (2019). Research in nutritional supplements and nutraceuticals for health, physical activity, and performance: moving forward (1). Appl. Physiol. Nutr. Metab. 44 (5), 455–460. doi: 10.1139/apnm-2018-0781
Weng, Z., Zhou, P., Salminen, W. F., Yang, X., Harrill, A. H., Cao, Z., et al. (2014). Green tea epigallocatechin gallate binds to and inhibits respiratory complexes in swelling but not normal rat hepatic mitochondria. Biochem. Biophys. Res. Commun. 443 (3), 1097–1104. doi: 10.1016/j.bbrc.2013.12.110
West-Eberhard, M. J. (2019). Nutrition, the visceral immune system, and the evolutionary origins of pathogenic obesity. Proc. Natl. Acad. Sci. U.S.A. 116 (3), 723–731. doi: 10.1073/pnas.1809046116
Wikipedia (2019). Epigallocatechin gallate.: Wikipedia, The Free Encyclopedia. [Accessed on June 30, 2019]. Available from: https://en.wikipedia.org/w/index.php?title=Epigallocatechin_gallate&oldid=903889712.
Wong, L. L., Lacar, L., Roytman, M., Orloff, S. L. (2017). Urgent liver transplantation for dietary supplements: an under-recognized problem. Transplant. Proc. 49 (2), 322–325. doi: 10.1016/j.transproceed.2016.11.041
Xin, S., Xiao, L. (2016). Clinical manifestations of Hepatitis E. Adv. Exp. Med. Biol. 948, 175–189. doi: 10.1007/978-94-024-0942-0_10
Yang, F., Oz, H. S., Barve, S., de Villiers, W. J., McClain, C. J., Varilek, G. W. (2001). The green tea polyphenol (-)-epigallocatechin-3-gallate blocks nuclear factor-kappa B activation by inhibiting I kappa B kinase activity in the intestinal epithelial cell line IEC-6. Mol. Pharmacol. 60 (3), 528–533.
Yang, Y., Shen, F., Huang, W., Qin, S., Huang, J. T., Sergi, C., et al. (2018). Glucose is involved in the dynamic regulation of m6A in patients with type 2 diabetes. J. Clin. Endocrinol. Metab. 104 (3), 665–673. doi: 10.1210/jc.2018-00619
Yang, M. Y., Huang, C. Y., Chiu, T. H. T., Chang, K. C., Lin, M. N., Chen, L. Y., et al. (2019). Using gas chromatography and mass spectrometry to determine 25-hydroxyvitamin D levels for clinical assessment of vitamin D deficiency. J. Food Drug Anal. 27 (2), 494–501. doi: 10.1016/j.jfda.2018.12.010
Yumei, F., Zhou, Y., Zheng, S., Chen, A. (2006). The antifibrogenic effect of (-)-epigallocatechin gallate results from the induction of de novo synthesis of glutathione in passaged rat hepatic stellate cells. Lab. Invest. 86 (7), 697–709. doi: 10.1038/labinvest.3700425
Zhang, M., Shen, F., Petryk, A., Tang, J., Chen, X., Sergi, C. (2016). “English Disease”: historical notes on rickets, the bone-lung link and child neglect issues. Nutrients 8 (11), E722. doi: 10.3390/nu8110722.
Zhang, K., Dong, R., Sun, K., Wang, X., Wang, J., Yang, C. S., et al. (2017). Synergistic toxicity of epigallocatechin-3-gallate and diethyldithiocarbamate, a lethal encounter involving redox-active copper. Free Radic. Biol. Med. 113, 143–156. doi: 10.1016/j.freeradbiomed.2017.09.027
Zhen, M. C., Wang, Q., Huang, X. H., Cao, L. Q., Chen, X. L., Sun, K., et al. (2007). Green tea polyphenol epigallocatechin-3-gallate inhibits oxidative damage and preventive effects on carbon tetrachloride-induced hepatic fibrosis. J. Nutr. Biochem. 18 (12), 795–805. doi: 10.1016/j.jnutbio.2006.12.016
Zheng, J., Ramirez, V. D. (2000). Inhibition of mitochondrial proton F0F1-ATPase/ATP synthase by polyphenolic phytochemicals. Br. J. Pharmacol. 130 (5), 1115–1123. doi: 10.1038/sj.bjp.0703397
Zhou, J., Farah, B. L., Sinha, R. A., Wu, Y., Singh, B. K., Bay, B. H., et al. (2014). Epigallocatechin-3-gallate (EGCG), a green tea polyphenol, stimulates hepatic autophagy and lipid clearance. PloS One 9 (1), e87161. doi: 10.1371/journal.pone.0087161
Zmora, E., Gorodischer, R., Bar-Ziv, J. (1979). Multiple nutritional deficiencies in infants from a strict vegetarian community. Am. J. Dis. Child 133 (2), 141–144. doi: 10.1001/archpedi.1979.02130020031005
Keywords: green tea, toxicity, hepatotoxicity, acute liver failure, pediatrics, metabolism, pharmacology, subcellular environment
Citation: Sergi CM (2020) Epigallocatechin-3-Gallate Toxicity in Children: A Potential and Current Toxicological Event in the Differential Diagnosis With Virus-Triggered Fulminant Hepatic Failure. Front. Pharmacol. 10:1563. doi: 10.3389/fphar.2019.01563
Received: 01 July 2019; Accepted: 03 December 2019;
Published: 29 January 2020.
Edited by:
Jia-bo Wang, Fifth Medical Center of the PLA General Hospital, ChinaReviewed by:
Xiumin Fu, Chinese Academy of Sciences, ChinaChung S. Yang, The State University of New Jersey, United States
Copyright © 2020 Sergi. This is an open-access article distributed under the terms of the Creative Commons Attribution License (CC BY). The use, distribution or reproduction in other forums is permitted, provided the original author(s) and the copyright owner(s) are credited and that the original publication in this journal is cited, in accordance with accepted academic practice. No use, distribution or reproduction is permitted which does not comply with these terms.
*Correspondence: Consolato M. Sergi, c2VyZ2lAdWFsYmVydGEuY2E=