- 1Chemical Biology Research Center, School of Pharmaceutical Sciences, Wenzhou Medical University, Wenzhou, China
- 2Engineering Laboratory of Zhejiang Province for Pharmaceutical Development of Growth Factors, Biomedical Collaborative Innovation Center of Wenzhou, Wenzhou, China
- 3Department of Pharmacy, Sanmen People’s Hospital of Zhejiang, Sanmen, China
- 4Key Laboratory of Imaging Diagnosis and Minimally Invasive Intervention, The Fifth Affiliated Hospital of Wenzhou Medical University, Lishui, China
In the past decades, epidermal growth factor receptor-tyrosine kinase inhibitors (EGFR-TKIs) had been proved as an effective treatment strategy for the patients with EGFR-mutated non-small-cell lung cancer (NSCLC). However, the tolerance for the EGFR-TKI always occurred after continuous administration for a period of time and limiting the application of these drugs. Activation of FGFR1 signaling pathway was one of the important escape mechanisms for EGFR-TKI resistant in NSCLC. Here, a novel dual inhibitor of EGFRL858R/T790M and FGFR1, compound15c, was found and can efficiently overcame the EGFR-TKI resistance via its simultaneous inhibition of their kinase activities. Comparison with EGFRL858R/T790M and FGFR1 inhibitor treatment alone or combined revealed that the inhibition of EGFRL858R/T790M and FGFR1 activity by 15c was responsible for surmounting the intrinsic EGFR-TKI resistance in EGFRL858R/T790M-mutated H1975 cells and the acquired resistance in Afatinib-tolerant PC9 cells (AFA-PC9). Flow Cytometry and Caspase3 activity analysis assay showed that 15c induced significant the early apoptosis of H1975 cells. Xenograft tumor formation in BALB/c mice induced by a H1975 cells was suppressed by 15c treatment, with no changes in animal body weight. Generally, 15c may act as a new-generation EGFR-TKI for the therapy of NSCLC patients suffering a resistance to current TKI.
Introduction
In the past few decades, to improve therapeutic activity and selectivity while developing antitumor drugs is always a great challenge. By targeting the genetic differences of cancer cells, several molecule-targeting drugs, such as Afatinib and Gefitinib, had been approved by U.S. FDA and shown their promising therapeutic activity against various cancers (Cataldo et al., 2011; Hirsch and Bunn, 2012). However, the therapeutic strategies of “one drug-hits-one target-treats-one disease” still face significant challenges as the drug resistance (To et al., 2019; Wojtaszek et al., 2019). Recent studies suggested that targeting the multiple targets might be a practicable approach to ameliorate the therapeutic activity and selectivity, meanwhile preventing the drug resistance (To et al., 2019).
Among cancer-related deaths, lung cancer is the leading cause that contributed to 27% of cancer-related mortality (Quintanal-Villalonga et al., 2019) and almost 85% of lung cancers were identified as non-small cell lung cancer (NSCLC) (Chang, 2011). The high dynamic of epidermal growth factor receptor (EGFR), one of the classic receptor tyrosine kinase (RTK), in NSCLC (Hirsch et al., 2003) recommended it as an attractive target for the development of small-molecule tyrosine kinase inhibitors (TKIs) to treat with NSCLC. At the beginning, several first-generation EGFR-targeted TKIs, such as Erlotinib and Gefitinib, had been approved by U.S. FDA for the treatment of NSCLC patients that harbor activating mutations in the EGFR (L858R or delE746-A750) (Cohen et al., 2003; Cohen et al., 2005; Cataldo et al., 2011). However, resistance to those inhibitors can be acquired due to secondary mutations (Bean et al., 2007; Kuang et al., 2009). The most common secondary mutations in the EGFR is T790M at exon 20, which is named as “gatekeeper” mutation (Zhou et al., 2009). Subsequently, a series of second-generation and third-generation EGFR-TKIs had being developed to overcome the resistance, especially T790M-associated resistance (Riely, 2008; Niederst et al., 2015; Chen et al., 2017b). Unfortunately, tolerance still occurred as a second mechanism that activation of alternative pathways, such as cMet, IGF-1R, and FGFR (Kono et al., 2009). Unlike the widely reported cMet and IGF-1R, the FGFR-dependent signaling act as an escape mechanism for EGFR-TKI resistance still have long way to go (Kono et al., 2009).
Fibroblast growth factor receptors (FGFRs) are a family of RTKs with four different members (FGFR1–4), and each of the FGFR contain an extracellular immunoglobulin domains, a single transmembrane domain and an intercellular kinase domain (Li, 2019). Moreover, genetic abnormalities or aberrant activation of the FGFR signal transduction have been implicated with the pathogenesis of the various disease and disorders including cancer, Alzheimer’s disease, diabetes and its complications (Ferrer and Marti, 1998; Gozgit et al., 2012; Harrison, 2012; Gallagher and LeRoith, 2013; Touat et al., 2015; Li, 2019). Among these receptors, FGFR1 amplification is identified in about 20% of NSCLC. In addition, Azuma et al., reported that the enhanced expression of FGFR1 conducted as an escape mechanism for cell survival of Afatinib-resistant cancer cells and may compensate the loss of EGFR-driven signaling pathway (Azuma et al., 2014). Further, Quintanal-Villalonga and his colleagues found that highly-expressed FGFR1 may result in a higher resistance to EGFR-TKI in the patients with EGFR-mutated lung cancer and those patients may benefit from combined EGFR/FGFR inhibition (Quintanal-Villalonga et al., 2019). Raoof and her colleagues performed whole-genome CRISPR screening and identified FGFR3 as the top target facilitating the survival of mesenchymal EGFR mutant cancers (Raoof et al., 2019). Therefore, dual EGFR-FGFR1 blockade may be a promising clinical strategy to overcome EGFR-mutated NSCLC.
Nowadays, drugs with polypharmacological activities are shown to be advantageous over combination therapy as their lower incidences of side effects and more resilient therapies (Anighoro et al., 2014). Thus, in the current study, we find that compound 15c, an EGFRL858R/T790M selective inhibitor in our previous study (Chen et al., 2017a), exhibited a dual inhibitory activity against EGFRL858R/T790M and FGFR1 and efficiently overcame the EGFR-TKI tolerance in EGFR-mutated NSCLC cells via concurrently inhibiting these two kinases activity. We suggest that 15c may act as a new-generation EGFR-TKI for the therapy of NSCLC patients suffering a resistance to current TKI.
Materials and Methods
Cell Culture and Reagents
WZ4002 (#S1173) and AZD4547 (#S2801) were purchased from Selleck Chemicals. Human bronchial epithelial cell line BEAS-2B, human lung squamous cancer cell line H520, and human NSCLC cell lines H1975 and PC9 were procured from the Institute of Biochemistry and Cell Biology, Chinese Academy of Sciences and tested for mycoplasma contamination by DAPI staining before experiment. All the cells were maintained in RPMI-1640 medium (#C11875500BT, Gibco) with 10% FBS (#10270-106, Gibco), 100 μg/ml streptomycin, and 100 U/ml penicillin (#15140122, Gibco) and placed in a humidified cell incubator (5% CO2, 37°C). Antibodies including anti-p-EGFR (#3777S), anti-p-FGFR1 (#2544S), anti-EGFR (#2646S), anti-FGFR (#9740S), anti-GAPDH (#5174S), and HRP-linked anti-rabbit IgG (#7074S) were purchased from Cell Signaling Technology (Danvers, MA, USA).
Kinase Inhibition Assay
The kinase inhibitory activities of candidate and positive inhibitors were tested via a Caliper Mobility Shift Assay. The difference between substrate and its phosphorylated product was detected to characterize the activity. Shortly, the reaction solution containing compounds, substrates, ATP, and enzymes was mixed well and transferred to a 384-well plate for the experiment. EDTA was introduced to terminate the process after incubate for 1h at room temperature. The data was collected on an EZ Reader II (Caliper Life Sciences, MA). The inhibitory rates of tested compounds were calculated depending on the negative control wells (without ATP) and positive control wells (without compounds). The recombinant kinases, including EGFRWT (#08-115), EGFRL858R/T790M (#08-510), and FGFR1WT (#08-133) were acquired from Carna Biosciences (Kobe, Japan). All the independent experiments were executed in duplicate and three times at six concentrations (0.001, 0.01, 0.1, 1, 10, and 100 μM) and IC50 value was calculated.
Anti-Proliferation Assay (MTS Assay)
All kinds of cells (4×103) were planted in 96-well plate and cultured overnight before examination. The protocol was formulated according to CellTiter 96® AQueous One Solution Cell Proliferation Assay (MTS) Technical Bulletin. Briefly, after treated the cells with different compounds for 72 h, 20 μl CellTiter 96® AQueous One Solution Reagent (MTS, #G3580, Promega, San Luis Obispo, CA) was added and the system was incubated for another 4 h at 37°C. The absorbance at 490 nm was recorded by using a microplate reader (SpectraMax M2, Molecular Devices, Sunnyvale, CA). The results of three independent assays were exhibited as IC50 value (mean ± SEM).
Western Blot Analysis
After treated with compounds, cells or tumor tissues were harvested and lysed in protein lysate buffer followed by centrifugation (12,000 rpm, 10 min, 4°C), supernatants were collected. The protein concentrations were measured using the Quick Start™ Bradford Protein Assay Kit (#5000201, Bio-Rad, Hercules, CA). Equivalent amount of protein samples were separated by 12% SDS-polyacrylamide gel (SDS-PAGE) and then transferred to PVDF membrane. The blotting was blocked with 5% nonfat milk at room temperature for 2 h and then incubated with primary antibody at 4°C for overnight. At last, anti-rabbit HRP-conjugated secondary antibody was added and incubated with membrane for 1 h. Between every two steps, the membrane will be washed with TBST for three times. The immune-reactive bands were detected via Clarity Max Western ECL Substrate Kit (#1705062, Bio-Rad, Hercules, CA). The density of the immune-reactive bands was analyzed by Image J (National Institute of Health, MD).
Cell Apoptosis Analysis
H1975 cells were seeded on 60-mm dishes for 12 h, and then treated with DMSO (vehicle), 15c (0.5, 2.5, 5, or 10 μM), WZ4002 (10μM), AZD4547 (10μM), or WZ4002 (10 μM) combined with AZD4547 (10 μM) for 24 h. After harvested and washed with PBS, cells were double stained with FITC Annexin V Apoptosis Detection Kit I (#556547, BD Biosciences, CA). The apoptosis were evaluated by using a FACSCalibur flow cytometer (BD Biosciences, CA).
Analysis of Caspase-3 Activity
The activity of Caspase-3 in H1975 cell lysates was detected by using a Caspase-3 Activity Kit (C1115, Beyotime Institute of Biotechnology, Nantong, China). The caspase-3 activity was normalized and calculated as percentage of control group.
Establishment of Afatinib-Resistant PC9 Cells
PC9 cells cultured in RPMI 1640 with 10% FBS were continuously exposed to Afatinib at the stepwise increased concentrations up to 1 µM over the following 3 months. After that, cells remained were cultured in Afatinib-free growth medium until the stable growth was restored. The isolated Afatinib-resistant cell line was named as AFA-PC9.
In Vivo Antitumor Study
Protocols for animal studies were approved by the Wenzhou Medical University Animal Policy and Welfare Committee. Male BALB/c nu/nu mice (6 weeks old, 18–22 g) were acquired from Vital River Laboratory Animal Technology Co., Ltd. (Beijing, China). Animals were housed at a constant room temperature with a 12 h: 12 h light/dark cycle and fed with a standard rodent diet and water. H1975 cells were injected subcutaneously into the right flank (1×107 cells in 200 μl of PBS). 40 mice were divided into five groups (n = 8/group): control (vehicle), 15c (10 mg/kg/day), WZ4002 (10 mg/kg/day), AZD4547 (10 mg/kg/day), and WZ4002 (10 mg/kg/day) combined with AZD4547 (10 mg/kg/day). The compounds were given by intraperitoneal (i.p.) injection. The tumor volumes were determined at the indicated time points (V = 0.5 × L × W2; L: length, W: width, V: volume). After sacrificed, the tumors were weighed and collected for studies on the protein expression.
Statistical Analysis
At least three independent experiments were performed for each assay (n≥3). Data were shown as mean ± SEM. GraphPad Prism 5.0 (GraphPad, San Diego, CA) was used for the statistical analyses. Student’s t-test and two-way ANOVA were employed to analyze the differences between different sets of data. P < 0.05 was considered statistically significant and exhibited by * or # characters.
Results
Compound 15c Acted as an EGFRL858R/T790M/FGFR1 Dual Inhibitor and Selectively Kills Human Lung Cancer Cells
In our previous work (Chen et al., 2017a), we designed and optimized a series analogue of WZ4002 and evaluated their inhibition activity against EGFRL858R/T790M, and found that several derivatives exhibited a high potency and selectivity between EGFRL858R/T790M and EGFRWT. In order to explore whether those compounds can inhibit the FGFR1 kinase activity, all the compounds were measured the kinase inhibitory effect against EGFRWT, EGFRL858R/T790M, and FGFR1WT in a cell-free system called Caliper Mobility Shift Assay. As shown in Figures 1A, B, 15c exhibited the high inhibition towards EGFRL858R/T790M and FGFR1WT, and relatively lower inhibition against EGFRWT. Thus, 15c can act as an EGFRL858R/T790M and FGFR1WT dual inhibitor.
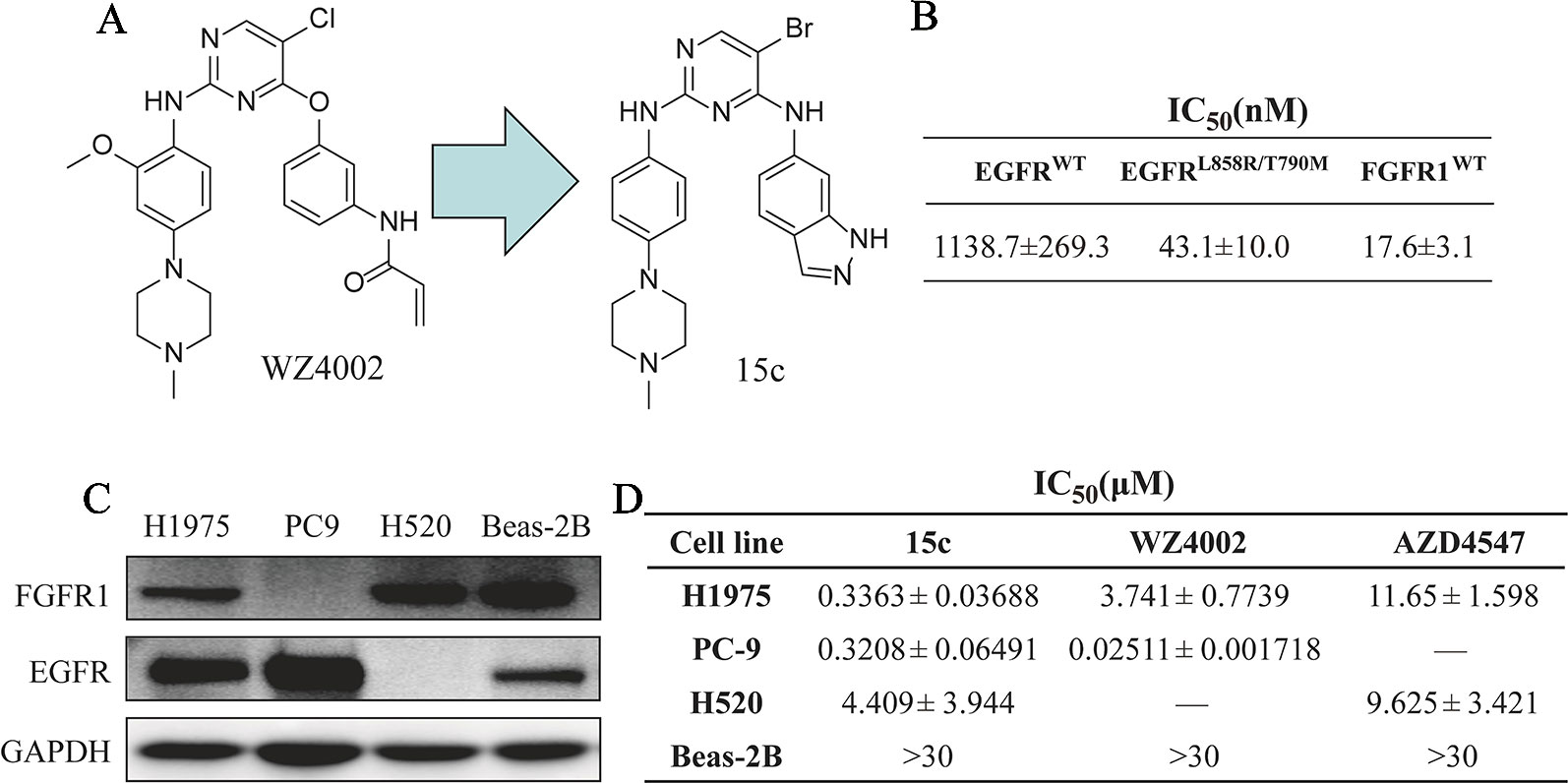
Figure 1 Compound 15c acted as an EGFRL858R/T790M/FGFR1 dual inhibitor and selectively kills human lung cancer cells. (A) The chemical structure of WZ4002 and 15c. (B) The kinase inhibitory activity against EGFRWT, EGFRL858R/T790M, and FGFR1WT of 15c. (C) Immunoblots of the expression levels of EGFR and FGFR1 protein in H1975, PC9, H520, and Beas-2B cell lines. (D) Anti-proliferation effect of 15c against H1975, PC9, H520, and Beas-2B cell lines. The IC50 values were reported as μM values (n = 3 independent experiments).
Kinases such as EGFR and FGFR play a critical role in the proliferation of cancer cells. Thus, we next detected the anti-proliferation effects of 15c on cultured human lung cancer cells and normal cells. Four cell lines, lung cancer PC9 cells (EGFRL858R+ and FGFR1−), lung cancer H520 cells (EGFR− and FGFR1+), lung cancer H1975 cells (EGFRL858R/T790M+ and FGFR1+), and normal lung epithelial cells Beas-2B cells (EGFR+ and FGFR1+), were chosen for the cytotoxic assay (Figure 1C). 15c exhibited high anti-proliferation activity against lung cancer cells rather than normal Beas-2B cells (highest concentration at 30 μM) (Figure 1D). Those findings indicated that 15c acted as an EGFRL858R/T790M/FGFR1 dual inhibitor in cell-free system and selectively inhibited the proliferation of human lung cancer cells.
Compound 15c Inhibited the Phosphorylation of EGFR and FGFR1 in the Cell System
In order to further confirm whether compound 15c can impede the phosphorylation of EGFR and FGFR1 in cells, the kinase inhibitory activity of 15c was detected in various ligand stimulated cancer cells. As mentioned before, PC9 is a human lung adenocarcinoma cell line with EGFRL858R mutation without FGFR1 expression, while H520 is a human lung squamous cell carcinoma cell line with the high expression of FGFR1 but without EGFR expression. Thus, the p-EGFR levels in EGF-induced PC9 cells and the p-FGFR1 levels in FGF-stimulated H520 cells were determined, respectively. The third-generation EGFR-TKI WZ4002 and the FGFR1-TKI AZD4547 were selected for comparison. The results were summarized in Figures 2A, B. Compound 15c, similar to the positive control, can inhibit the phosphorylation of FGFR1 and EGFR in a dose-dependent manner in different cell lines.
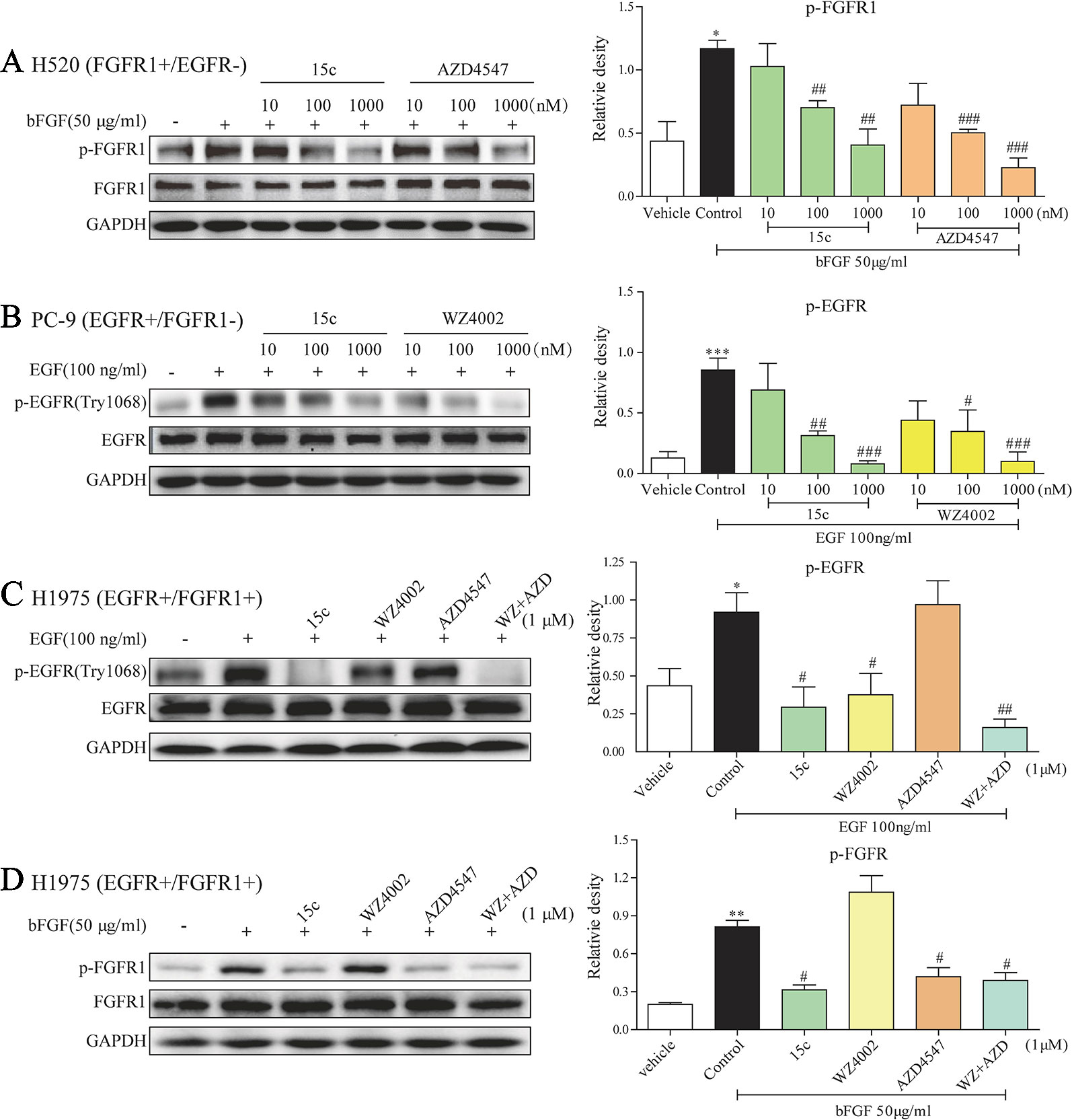
Figure 2 Compound 15c inhibited the phosphorylation of EGFR and FGFR1 in the cell system. (A–D) Representative immunoblots of phosphorylated FGFR1/EGFR, total FGFR1/EGFR, and GAPDH (sample loading controls) in three different cell lines that treated with inhibitors (n = 3 independent experiments for each panel). (A) Analysis of the p-FGFR1 level in bFGF-stimulated H520 cells. (B) Analysis of the p-EGFR level in EGF-stimulated PC9 cells. (C) Analysis of the p-EGFR level in EGF-stimulated H1975 cells. (D) Analysis of the p-FGFR1 level in bFGF-stimulated H1975 cells. The statistical results were shown as means ± SD (n = 3) on the right of each panels. Significant difference was indicated as * or # character (*p < 0.05, **p < 0.01, and ***p < 0.001 versus vehicle group, #p < 0.05, ##p < 0.01, and ###p < 0.001 versus control group).
In addition, H1975 is a human adenocarcinoma cell line with EGFRL858R/T790M mutation and FGFR1 expression, which can be used as an EGFR-TKI acquired resistant cell line. In this part, we treated the H1975 cells with 1 μM 15c, WZ4002, AZD4547, or WZ4002 and AZD4547 combination followed by bFGF or EGF stimulated. The p-FGFR1 and p-EGFR levels were determined by Western blot. In the EGF-stimulated H1975 cells (Figure 2C), 15c and WZ4002 exhibited the similar inhibitory activity against the phosphorylation of EGFR and AZD4547, a FGFR1 inhibitor, showed no effect on the phosphorylation of EGFR. The inhibitory effect of WZ4002 and AZD4547 combination was slightly better than 15c or WZ4002 alone, but no significant difference was noted. Similarly, in the bFGF-induced H1975 cells (Figure 2D), 15c and AZD4547 exhibited the comparable inhibitory activity against the phosphorylation of FGFR1 and WZ4002 showed no effect. The inhibitory impact of WZ4002 and AZD4547 combination was almost the same to 15c or WZ4002 alone. Those results revealed that 15c was a potential EGFRL858R/T790M/FGFR1 dual inhibitor in the cell system.
Compound 15c Induced Caspase-Dependent Apoptosis in H1975 Cells
To explore whether 15c can accelerate H1975 cells apoptosis, a series apoptosis related experiments were introduced. H1975 cells that starved for 12 h were treated with variable level of test compounds, positive inhibitors, or vehicle for 24 h. After harvested and washed with PBS, cells were double stained with FITC Annexin V Apoptosis Detection Kit I. The apoptotic cells were detected by flow cytometry analysis and the results were also illustrated in the column chart (Figures 3A, B). Significant apoptosis was observed in 15c treated H1975 cells in a dose-dependent manner compared with the vehicle group. Moreover, the apoptosis rates of 15c at 5 μM and 10 μM were much higher than that WZ4002 or AZD4547 treated alone or combined at 10 μM. In addition, caspase 3 activity assay were further applied to verify the effect of 15c on inducing H1975 apoptosis (Figure 3C). The results suggested that 15c could induced the apoptosis of H1975 cells via activating caspase-dependent apoptosis.
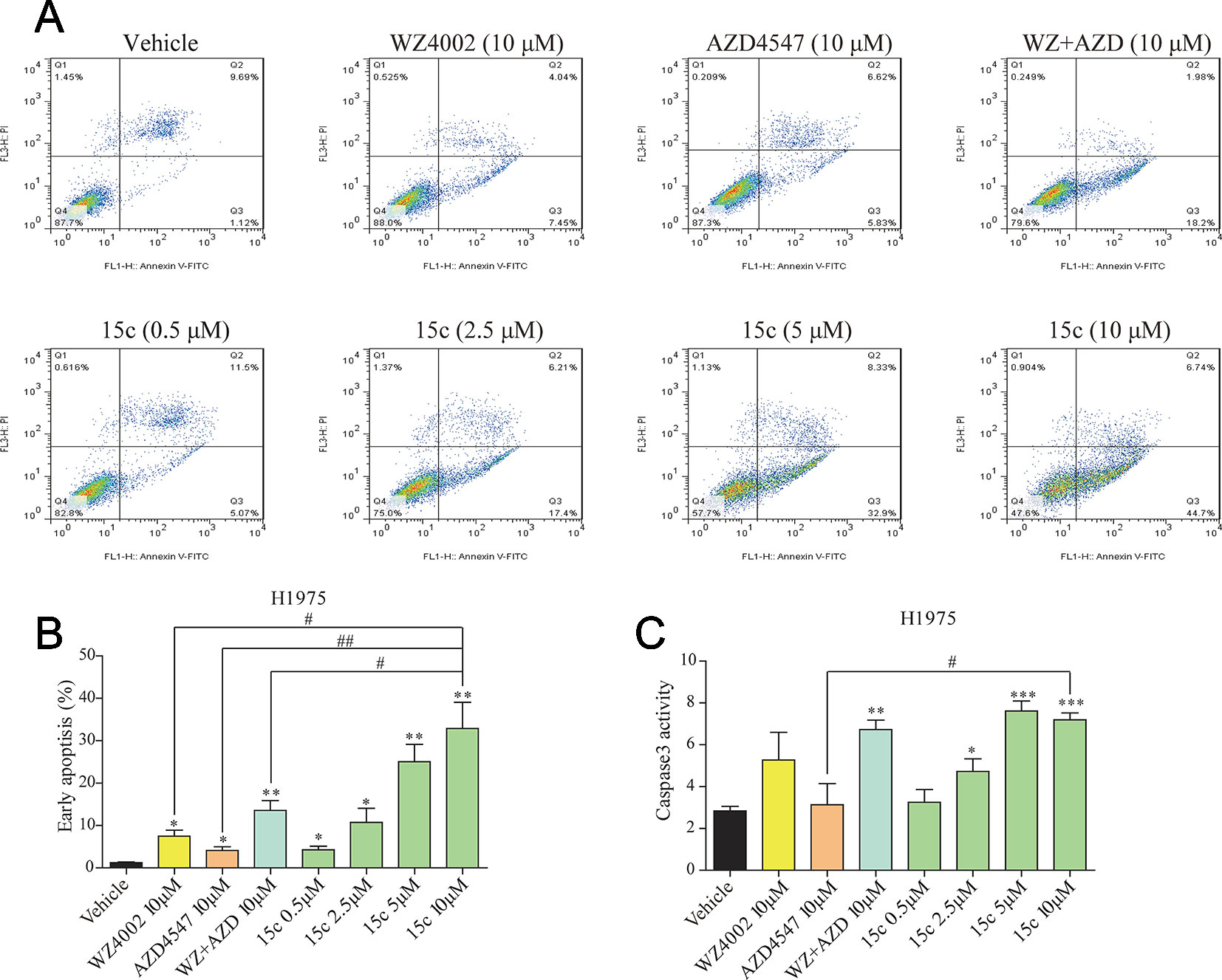
Figure 3 Compound 15c induced caspase-dependent apoptosis in H1975 cells. (A) Apoptosis induced by inhibitors treatment in H1975 cells. Cells were incubated with the indicated doses of 15c, WZ4002, AZD4547, and WZ4002 + AZD4547 for 24 h, and then harvested and analyzed by flow cytometry. Representative data from one experiment are shown. (B) The percentage of apoptotic cells in the treatment groups was calculated. (C) Caspase-3 activity of H1975 cells treated with 15c (0.5, 2.5, 5, 10 μM), AZD4547 (10 μM), WZ4002 (10 μM), or AZD4547 (10 μM) + WZ4002 (10 μM) for 24 h. All the statistical results are shown as means ± SD (n = 3). *p < 0.05, **p < 0.01, and ***p < 0.001 versus vehicle group, #p < 0.05 and ##p < 0.01 versus 10 μM 15c group.
Compound 15c Overcame Afatinib-Tolerant in Established Acquired Resistant PC9 Cell Line
The effect of 15c on reversing drug resistant in lung cancer cells was further studied by domesticated a cell line resistant to Afatinib. The PC9 cells were continuously exposed to Afatinib at the stepwise increased concentrations from 0.025 to 1 µM over the following 3 months to construct the Afatinib-resistant cell lines AFA-PC9. Western blot analyses of PC9 and AFA-PC9 were shown in Figure 4A, and the FGFR1 expression was significantly amplified in AFA-PC9 and the EGFR expression was not affected. The morphology change of the AFA-PC9 was demonstrated in Figure 4B. Compared to PC9, AFA-PC9 cells started to grow in clusters, which was completely consistent with the conclusions in previous studies. MTS analysis was also carried out on the drug-resistant AFA-PC9 (Figure 4C). Compared with the PC9 cells, the sensitivity of AFA-PC9 cells to Afatinib was reduced by more than 1000 times. Then, we compared the anti-proliferative effects of compound 15c and WZ4002 on AFA-PC9 and PC9 cells. It turned out that the sensitivity of AFA-PC9 to WZ4002 decreased more than 100 times (IC50 was changed from 0.025 to 3.2 μM) while only decreased two times against 15c (IC50 was changed from 0.32 to 0.66 μM), indicating that 15c can overcame the acquired resistant to Afatinib in PC9 cell line.
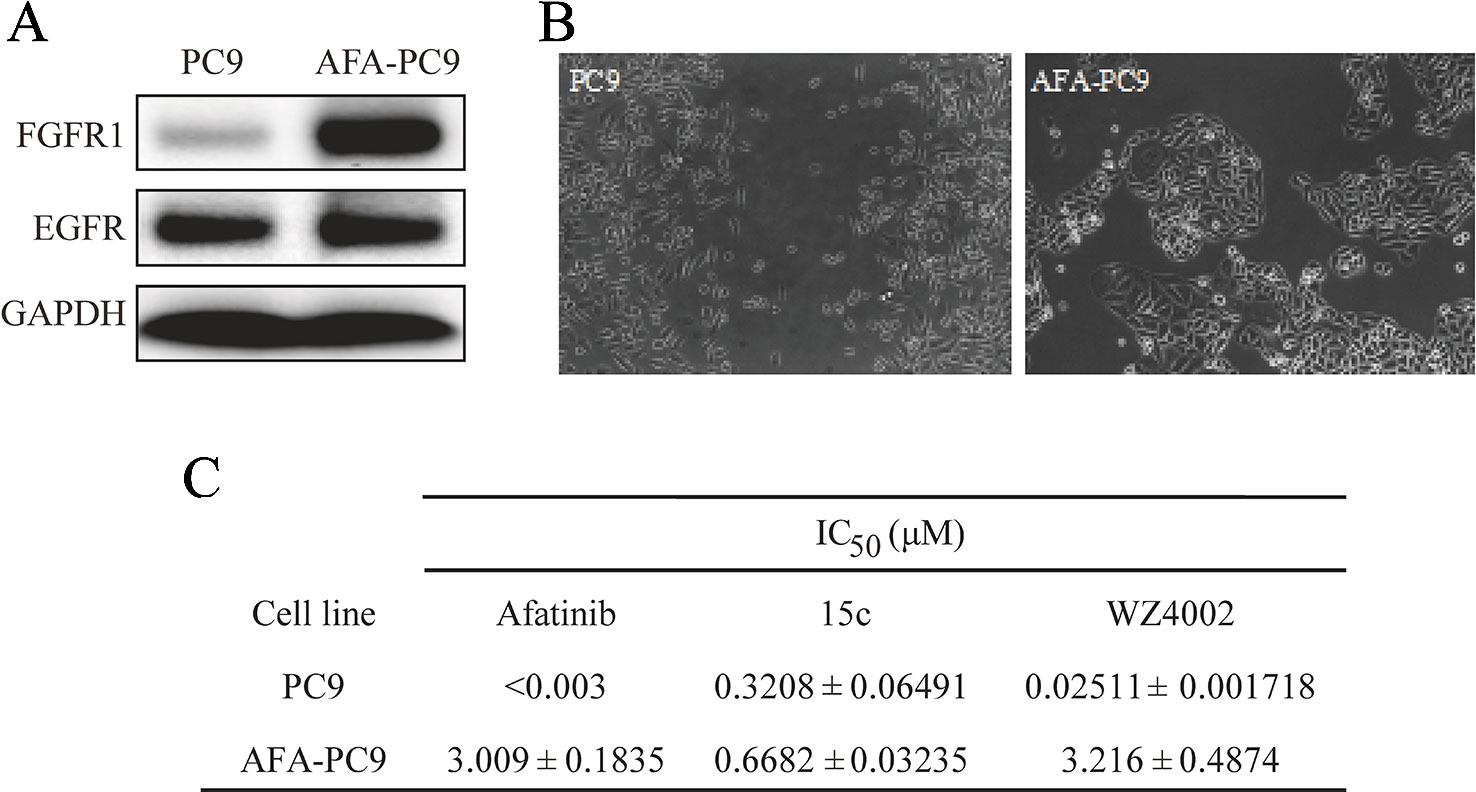
Figure 4 Compound 15c overcame Afatinib-tolerant in established acquired resistant PC9 cell (AFA-PC9). Cancer cell line PC9 was continuously exposed to Afatinib at stepwise increased concentrations up to 1 µM over the following 3 months to establish an Afatinib-resistant PC9 cell line named ADA-PC9. (A) Western Blot analysis of the EGFR and FGFR1 expression in PC9 cells and AFA-PC9 cells. (B) Morphological changes of AFA-PC9 cells compared to PC9 cells; (C) Anti-proliferation effect of 15c, WZ4002, and Afatinib against PC9 and AFA-PC9 cell lines. The IC50 values were reported as μM values.
Compound 15c Attenuated H1975 Xenograft Tumor Growth In Vivo by Suppressing p-EGFR and p-FGFR1 Levels
Subcutaneous xenograft model of H1975 cells in immunodeficient mice was applied to evaluate the in vivo anti-tumor activity of 15c. Male BALB/c nu/nu mice (6 weeks old, 18–22 g) were subcutaneously injected with H1975 cells (1×107 cells in 200 μl of PBS). When the tumor volume was around 80–100 mm3, the mice were randomly divided into five groups (eight in each) including negative control group (Vehicle group), 15c group (10 mg/kg/day, ip), WZ4002 group (10 mg/kg/day, ip), AZD4547 group (10 mg/kg/day, ip), and WZ4002 combined with AZD4547 group (10 mg/kg/day, ip). After intraperitoneal administration of compound for 20 days, the tumor volume and weight of each group were measured and shown in Figures 5A–C. 15c significantly reduced H1975 tumor volume and weight versus vehicle control. Even compared with the positive inhibitor alone, 15c still exhibited a better tumor-inhibition effect. Only WZ4002 and AZD4547 combination administration can restrain the H1975 tumor to the same size of the tumor treated by 15c. Importantly, these is no significant weight loss of mice even treated 15c for 20 days (Figure 5D). Mechanistically, western blot analysis of tumor tissues revealed that 15c attenuated H1975 xenograft tumor growth in vivo by suppressing the phosphorylation of FGFR1 and EGFR (Figures 5E, F).
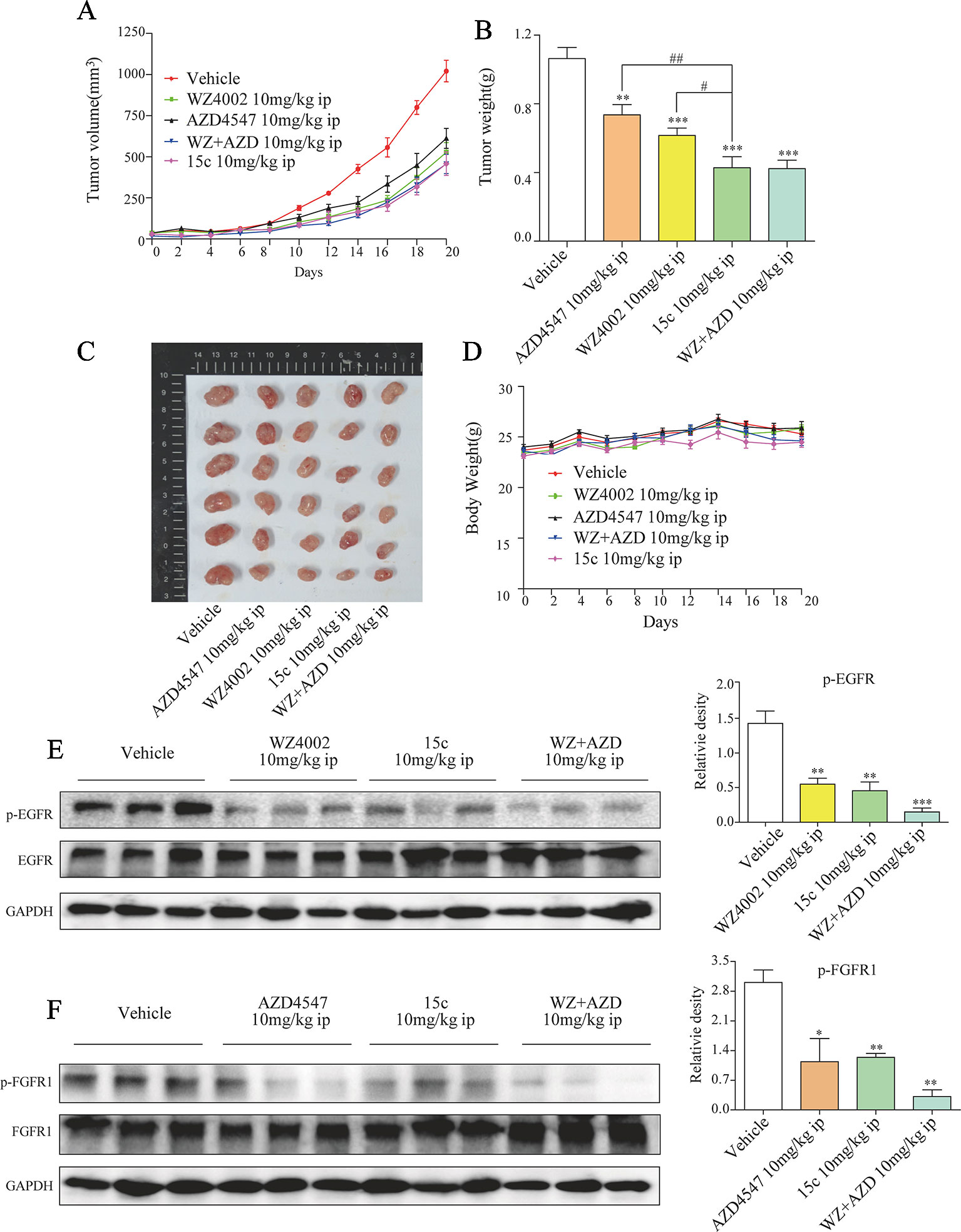
Figure 5 Compound 15c inhibited H1975 xenograft tumor growth in vivo, accompanied with decreased p-EGFR and p-FGFR1 levels. Tumor-bearing mice were intraperitoneally injected with 15c (10 mg/kg), WZ4002 (10 mg/kg), AZD4547 (10 mg/kg), or WZ4002 (10 mg/kg) + AZD4547 (10 mg/kg). (A–C) Tumor volume and tumor weight of H1975 human lung cancer xenografts in nude mice. (D) Body weight was measured every 2 days. Points, means of seven mice; bars, SEM. (E, F) Analysis of pFGFR1 and p-EGFR levels in tumor tissues. *p < 0.05, **p < 0.01, and ***p < 0.001 versus vehicle group, #p < 0.05 and ##p < 0.01 versus 10 mg/kg 15c group. ip, intraperitoneally injected.
Discussion
Lung cancer is the leading cause of cancer-related death worldwide with NSCLC accounting for 85% of cases. Therefore, it is of great theoretical and practical significance to study the pathogenesis of lung cancer and develop the corresponding therapeutic drugs. In the past few decades, it has been determined that the activation of EGFR is closely related to the genesis and progress of lung cancer (Lynch et al., 2004; Paez et al., 2004; Pao et al., 2004; Sharma et al., 2007). Thus, EGFR has attracted great attentions as it is an ideal target for the cancer therapy. There are a series of small molecular inhibitors that can inhibit the kinase activity of EGFR had been found and named as EGFR-TKIs (Sequist et al., 2007; Pao and Chmielecki, 2010). Gefitinib and Erotinib are the first-generation EGFR-TKIs that have been studied clinically with a great advance. However, patients generally develop secondary mutations, such as T790M gatekeeper, and lead to drug resistance after EGFR-TKIs treated for 12 months (Chen et al., 2017b). As a result, second and third-generation EGFR-TKIs, e.g. Afatinib, WZ4002, and Osimertinib, are developed to overcome the T790M mutation-associated resistance (Zhou et al., 2009; Mok et al., 2017). The poor therapeutic window of Afatinib limited its clinical application (Chen et al., 2017b). Osimertinib exhibits an excellent treatment effect for NSCLC patients with EGFR T790M-mutated, but acquired resistance is still inevitable (Oxnard et al., 2018). Thus, there is still an urgent demand to establish an effective antitumor strategy for those NSCLC patients with acquired resistance to second or third-generation EGFR-TKIs.
Müller-Tidow and his colleagues identified the expression of 56 RTKs in primary NSCLC tumors and found that 33 RTKs are expressed at the mRNA level in 25% samples (Müller-Tidow et al., 2005), indicating that those RTKs may function as an alternatives to EGFR signal pathway in NSCLC, such as cMet, Axl, IGF-1R, and PDGFR. The amplification of cMet is a major factor of acquired resistance in lung cancer and the combination of Gefitinib and cMet antibody can significantly enhance the growth inhibition in cMet over-expressing cell lines (Bean et al., 2007; Zucali et al., 2008). Similarly, the IGF-1R survival pathway contribute to Gefitinib resistance and synergistically inhibition of IGF-1R could strengthen the anti-tumor effect of Gefinitib (Desbois-Mouthon et al., 2006). In addition, Axl lead to EGFR-TKIs resistant by increasing expression and forming a hetero-dimerization with EGFR and inhibition of Axl could prevent or overcome acquired resistance to EGFR-TKIs in EGFR-mutated lung cancer (Zhang et al., 2012; Vouri et al., 2016). Recently, several researches mentioned that the activation of the autocrine ring of FGF/FGFR, one of the typical RTKs, could act as a compensatory mechanism to promote the survival and growth of EGFR-TKIs resistant lung cancer cells. These findings declared that simultaneous inhibition of EGFR and FGFR1 activity may be a novel and effective strategy to overcome FGFR1-related EGFR-TKIs resistant in NSCLC.
Nowadays, combination therapy of various drugs with different targets has become an important strategy for cancer treatment (Bell, 2013). Combined drugs can improve the curative effect as the synergism of different activity. However, increased off-target effect and metabolic pressure restricted the application of combined administration. Moreover, drugs with polypharmacological activities are shown to be advantageous over combination therapy as their lower incidences of side effects and more resilient therapies. Thus, developing dual-inhibitors that target FGFR1 and EGFR will be an efficient way to reverse FGFR1-related EGFR-TKIs resistant in NSCLC.
Here, we presented an EGFRL858R/T790M/FGFR1 dual-inhibitor 15c, which can simultaneous inhibited the kinase activity of EGFRL858R/T790M and FGFR1 both in kinase assay and cell study, for the treatment of FGFR1-amplified EGFR-TKIs resistant lung cancers. The anti-proliferation effects of 15c on NSCLC cell lines and normal BEAS-2B cell line showed that 15c could effectively hold back the proliferation of NSCLC cells rather than normal cells. Particularly, 15c exhibited a better inhibitory effect to H1975 cells and AFA-PC9 cells, which contained EGFR mutation and FGFR1 overexpression, than WZ4002 and AZD4547. Further flow cytometry and Caspase 3 activity analysis demonstrated that 15c could induce the apoptosis in a dose-dependent manner and the effect was better than WZ4002 combined AZD4547 treatment. Finally, the in vivo study as well revealed that the anti-tumor ability of 15c was comparable to the combination administration of WZ4002 and AZD4547 but better than the separate administration.
In summary, acquired resistant is the major reason for EGFR-TKI tolerant NSCLCs and FGFR1 amplification has been proved that contribute to the EGFR-TKI resistant. Thus, EGFRL858R/T790M/FGFR1 dual inhibitor 15c can be developed as an ideal candidate drug for FGFR1-amplified EGFR-TKIs resistant lung cancers.
Data Availability Statement
The raw data supporting the conclusions of this article will be made available by the authors, without undue reservation, to any qualified researcher.
Ethics Statement
The animal study was reviewed and approved by the Wenzhou Medical University Animal Policy and Welfare Committee.
Author Contributions
Participated in research design: GC, QW, GL. Cell and animal study: GC, YB, QW, YZ, and XL. Kinase study: LF. Performed chemical synthesis of 15c: LC and ZL. Contributed to the writing of the manuscript: GC, XZ, GL.
Funding
This work was supported by the National Key R&D Program of China (2017YFA0506000), the National Natural Science Foundation of China (Grants No. 81703352 and 81773579), the Zhejiang Province Natural Science Foundation (LY17B020008), the Zhejiang Medical Health Technology Platform Project (2018KY183) and the Zhejiang Natural Science Foundation Pharmaceutical Society Joint Fund Project (LYY19H310004).
Conflict of Interest
The authors declare that the research was conducted in the absence of any commercial or financial relationships that could be construed as a potential conflict of interest.
References
Anighoro, A., Bajorath, J., Rastelli, G. (2014). Polypharmacology: challenges and opportunities in drug discovery: miniperspective. J. Med. Chem. 57 (19), 7874–7887. doi: 10.1021/jm5006463
Azuma, K., Kawahara, A., Sonoda, K., Nakashima, K., Tashiro, K., Watari, K., et al. (2014). FGFR1 activation is an escape mechanism in human lung cancer cells resistant to afatinib, a pan-EGFR family kinase inhibitor. Oncotarget 5 (15), 5908. doi: 10.18632/oncotarget.1866
Bean, J., Brennan, C., Shih, J.-Y., Riely, G., Viale, A., Wang, L., et al. (2007). MET amplification occurs with or without T790M mutations in EGFR mutant lung tumors with acquired resistance to gefitinib or erlotinib. Proc. Nat. Acad. Sci. 104 (52), 20932–20937. doi: 10.1073/pnas.0710370104
Bell, D. (2013). Combine and conquer: advantages and disadvantages of fixed-dose combination therapy. Diabetes Obesity Metab. 15 (4), 291–300. doi: 10.1111/dom.12015
Cataldo, V. D., Gibbons, D. L., Pérez-Soler, R., Quintás-Cardama, A. (2011). Treatment of non–small-cell lung cancer with erlotinib or gefitinib. N. Engl. J. Med. 364 (10), 947–955. doi: 10.1056/NEJMct0807960
Chang, A. (2011). Chemotherapy, chemoresistance and the changing treatment landscape for NSCLC. Lung Cancer 71 (1), 3–10. doi: 10.1016/j.lungcan.2010.08.022
Chen, L., Fu, W., Feng, C., Qu, R., Tong, L., Zheng, L., et al. (2017a). Structure-based design and synthesis of 2, 4-diaminopyrimidines as EGFR L858R/T790M selective inhibitors for NSCLC. Eur. J. Med. Chem. 140, 510–527. doi: 10.1016/j.ejmech.2017.08.061
Chen, L., Fu, W., Zheng, L., Liu, Z., Liang, G. (2017b). Recent progress of small-molecule epidermal growth factor receptor (EGFR) inhibitors against C797S resistance in non-small-cell lung cancer: miniperspective. J. Med. Chem. 61 (10), 4290–4300. doi: 10.1021/acs.jmedchem.7b01310
Cohen, M. H., Williams, G. A., Sridhara, R., Chen, G., Pazdur, R. (2003). FDA drug approval summary: gefitinib (ZD1839) (Iressa®) tablets. Oncologist 8 (4), 303–306. doi: 10.1634/theoncologist.8-4-303
Cohen, M. H., Johnson, J. R., Chen, Y.-F., Sridhara, R., Pazdur, R. (2005). FDA drug approval summary: erlotinib (Tarceva®) tablets. Oncologist 10 (7), 461–466. doi: 10.1634/theoncologist.10-7-461
Desbois-Mouthon, C., Cacheux, W., Blivet-Van Eggelpoël, M. J., Barbu, V., Fartoux, L., Poupon, R., et al. (2006). Impact of IGF-1R/EGFR cross-talks on hepatoma cell sensitivity to gefitinib. Int. J. Cancer 119 (11), 2557–2566. doi: 10.1002/ijc.22221
Ferrer, I., Marti, E. (1998). Distribution of fibroblast growth factor receptor-1 (FGFR-1) and FGFR-3 in the hippocampus of patients with Alzheimer’s disease. Neurosci. Lett. 240 (3), 139–142. doi: 10.1016/s0304-3940(97)00948-8
Gallagher, E. J., LeRoith, D. (2013). Does a single nucleotide polymorphism in the FGFR explain the connection between diabetes and cancer? Cell Metab. 17 (6), 808–809. doi: 10.1016/j.cmet.2013.05.006
Gozgit, J. M., Wong, M. J., Moran, L., Wardwell, S., Mohemmad, Q. K., Narasimhan, N. I., et al. (2012). Ponatinib (AP24534), a multitargeted pan-FGFR inhibitor with activity in multiple FGFR-amplified or mutated cancer models. Mol. Cancer Ther. 11 (3), 690–699. doi: 10.1158/1535-7163.MCT-11-0450
Harrison, C. (2012). Obesity and diabetes: an FGFR antibody with long-lasting effects. Nat. Rev. Drug Discovery 11 (2), 106. doi: 10.1038/nrd3660
Hirsch, F. R., Bunn, P. A. (2012). A new generation of EGFR tyrosine-kinase inhibitors in NSCLC. Lancet Oncol. 13 (5), 442–443. doi: 10.1016/S1470-2045(12)70124-9
Hirsch, F. R., Varella-Garcia, M., Bunn, P. A., Jr., Di Maria, M. V., Veve, R., Bremnes, R. M., et al. (2003). Epidermal growth factor receptor in non–small-cell lung carcinomas: correlation between gene copy number and protein expression and impact on prognosis. J. Clin. Oncol. 21 (20), 3798–3807. doi: 10.1200/JCO.2003.11.069
Kono, S. A., Marshall, M. E., Ware, K. E., Heasley, L. E. (2009). The fibroblast growth factor receptor signaling pathway as a mediator of intrinsic resistance to EGFR-specific tyrosine kinase inhibitors in non-small cell lung cancer. Drug Resist. Updates 12 (4–5), 95–102. doi: 10.1016/j.drup.2009.05.001
Kuang, Y., Rogers, A., Yeap, B. Y., Wang, L., Makrigiorgos, M., Vetrand, K., et al. (2009). Noninvasive detection of EGFR T790M in gefitinib or erlotinib resistant non–small cell lung cancer. Clin. Cancer Res. 15 (8), 2630–2636. doi: 10.1158/1078-0432.CCR-08-2592
Lynch, T. J., Bell, D. W., Sordella, R., Gurubhagavatula, S., Okimoto, R. A., Brannigan, B. W., et al. (2004). Activating mutations in the epidermal growth factor receptor underlying responsiveness of non–small-cell lung cancer to gefitinib. N. Engl. J. Med. 350 (21), 2129–2139. doi: 10.1056/NEJMoa040938
Müller-Tidow, C., Diederichs, S., Bulk, E., Pohle, T., Steffen, B., Schwäble, J., et al. (2005). Identification of metastasis-associated receptor tyrosine kinases in non–small cell lung cancer. Cancer Res. 65 (5), 1778–1782. doi: 10.1158/0008-5472.CAN-04-3388
Mok, T. S., Wu, Y.-L., Ahn, M.-J., Garassino, M. C., Kim, H. R., Ramalingam, S. S., et al. (2017). Osimertinib or platinum–pemetrexed in EGFR T790M-positive lung cancer. N. Engl. J. Med. 376 (7), 629–640. doi: 10.1056/NEJMoa1612674
Niederst, M. J., Hu, H., Mulvey, H. E., Lockerman, E. L., Garcia, A. R., Piotrowska, Z., et al. (2015). The allelic context of the C797S mutation acquired upon treatment with third-generation EGFR inhibitors impacts sensitivity to subsequent treatment strategies. Clin. Cancer Res. 21 (17), 3924–3933. doi: 10.1158/1078-0432.CCR-15-0560
Oxnard, G. R., Hu, Y., Mileham, K. F., Husain, H., Costa, D. B., Tracy, P., et al. (2018). Assessment of resistance mechanisms and clinical implications in patients with EGFR T790M-positive lung cancer and acquired resistance to osimertinib. JAMA Oncol. 4 (11), 1527–1534. doi: 10.1001/jamaoncol.2018.2969
Paez, J. G., Jänne, P. A., Lee, J. C., Tracy, S., Greulich, H., Gabriel, S., et al. (2004). EGFR mutations in lung cancer: correlation with clinical response to gefitinib therapy. Science 304 (5676), 1497–1500. doi: 10.1126/science.1099314
Pao, W., Chmielecki, J. (2010). Rational, biologically based treatment of EGFR-mutant non-small-cell lung cancer. Nat. Rev. Cancer 10 (11), 760. doi: 10.1038/nrc2947
Pao, W., Miller, V., Zakowski, M., Doherty, J., Politi, K., Sarkaria, I., et al. (2004). EGF receptor gene mutations are common in lung cancers from “never smokers” and are associated with sensitivity of tumors to gefitinib and erlotinib. Proc. Nat. Acad. Sci. 101 (36), 13306–13311. doi: 10.1073/pnas.0405220101
Quintanal-Villalonga, A., Molina-Pinelo, S., Cirauqui, C., Ojeda-Márquez, L., Marrugal, Á., Suarez, R., et al. (2019). FGFR1 cooperates with EGFR in lung cancer oncogenesis, and their combined inhibition shows improved efficacy. J. Thorac. Oncol. 14 (4), 641–655. doi: 10.1016/j.jtho.2018.12.021
Raoof, S., Mulford, I. J., Frisco-Cabanos, H., Nangia, V., Timonina, D., Labrot, E., et al. (2019). Targeting FGFR overcomes EMT-mediated resistance in EGFR mutant non-small cell lung cancer. Oncogene 38 (37), 6399–6413. doi: 10.1038/s41388-019-0887-2
Riely, G. J. (2008). Second-generation epidermal growth factor receptor tyrosine kinase inhibitors in non-small cell lung cancer. J. Thorac. Oncol. 3 (6), S146–S149. doi: 10.1097/JTO.0b013e318174e96e
Sequist, L. V., Joshi, V. A., Jänne, P. A., Muzikansky, A., Fidias, P., Meyerson, M., et al. (2007). Response to treatment and survival of patients with non-small cell lung cancer undergoing somatic EGFR mutation testing. Oncologist 12 (1), 90–98. doi: 10.1634/theoncologist.12-1-90
Sharma, S. V., Bell, D. W., Settleman, J., Haber, D. A. (2007). Epidermal growth factor receptor mutations in lung cancer. Nat. Rev. Cancer 7 (3), 169. doi: 10.1038/nrc2088
To, C., Jang, J., Chen, T., Park, E., Mushajiang, M., De Clercq, D. J., et al. (2019). Single and dual targeting of mutant EGFR with an allosteric inhibitor. Cancer Discov. 9 (7), 926–943. doi: 10.1158/2159-8290.CD-18-0903.
Touat, M., Ileana, E., Postel-Vinay, S., André, F., Soria, J.-C. (2015). Targeting FGFR signaling in cancer. Clin. Cancer Res. 21 (12), 2684–2694. doi: 10.1158/1078-0432.CCR-14-2329
Vouri, M., Croucher, D., Kennedy, S., An, Q., Pilkington, G., Hafizi, S. (2016). Axl-EGFR receptor tyrosine kinase hetero-interaction provides EGFR with access to pro-invasive signalling in cancer cells. Oncogenesis 5 (10), e266. doi: 10.1038/oncsis.2016.66
Wojtaszek, J. L., Chatterjee, N., Najeeb, J., Ramos, A., Lee, M., Bian, K., et al. (2019). A small molecule targeting mutagenic translesion synthesis improves chemotherapy. Cell 178 (1), 152–159.e11. doi: 10.1016/j.cell.2019.05.028
Zhang, Z., Lee, J. C., Lin, L., Olivas, V., Au, V., LaFramboise, T., et al. (2012). Activation of the AXL kinase causes resistance to EGFR-targeted therapy in lung cancer. Nat. Genet. 44 (8), 852. doi: 10.1038/ng.2330
Zhou, W., Ercan, D., Chen, L., Yun, C.-H., Li, D., Capelletti, M., et al. (2009). Novel mutant-selective EGFR kinase inhibitors against EGFR T790M. Nature 462 (7276), 1070. doi: 10.1038/nature08622
Keywords: NSCLC, EGFR, FGFR1, drug resistance, kinase inhibitor
Citation: Chen G, Bao Y, Weng Q, Zhao Y, Lu X, Fu L, Chen L, Liu Z, Zhang X and Liang G (2020) Compound 15c, a Novel Dual Inhibitor of EGFRL858R/T790M and FGFR1, Efficiently Overcomes Epidermal Growth Factor Receptor-Tyrosine Kinase Inhibitor Resistance of Non-Small-Cell Lung Cancers. Front. Pharmacol. 10:1533. doi: 10.3389/fphar.2019.01533
Received: 22 October 2019; Accepted: 27 November 2019;
Published: 10 January 2020.
Edited by:
Zhouguang Wang, Albert Einstein College of Medicine, United StatesReviewed by:
Aiping Jiang, Harvard University, United StatesShuai Wu, Wistar Institute, United States
Copyright © 2020 Chen, Bao, Weng, Zhao, Lu, Fu, Chen, Liu, Zhang and Liang. This is an open-access article distributed under the terms of the Creative Commons Attribution License (CC BY). The use, distribution or reproduction in other forums is permitted, provided the original author(s) and the copyright owner(s) are credited and that the original publication in this journal is cited, in accordance with accepted academic practice. No use, distribution or reproduction is permitted which does not comply with these terms.
*Correspondence: Guang Liang, wzmcliangguang@163.com
†These authors have contributed equally to this work