- 1Stem Cell Therapy Unit, “Jesús Usón” Minimally Invasive Surgery Centre, Cáceres, Spain
- 2CIBER de Enfermedades Cardiovasculares (CIBER-CV), Madrid, Spain
- 3Research and Development Department, ORTHOGEN AG, Düsseldorf, Germany
Osteoarthritis is one of the most common chronic health conditions associated with pain and disability. Advanced therapies based on mesenchymal stem cells have become valuable options for the treatment of these pathologies. Conditioned serum (CS, “Orthokine”) has been used intra-articularly for osteoarthritic patients. In this work, we hypothesized that the rich content on anti-inflammatory proteins and growth factors of CS may exert a beneficial effect on the biological activity of human adipose-derived mesenchymal stem cells (hAdMSCs). In vitro studies were designed using hAdMSCs cocultured with CS at different concentrations (2.5, 5, and 10%). Chondrogenic differentiation assays and immunomodulatory experiments using in vitro-stimulated lymphocytes were performed. Our results demonstrated that CS significantly enhanced the differentiation of hAdMSCs toward chondrocytes. Moreover, hAdMSCs pre-sensitized with CS reduced the lymphocyte proliferation as well as their differentiation toward activated lymphocytes. These results suggest that in vivo coadministration of CS and hAdMSCs may have a beneficial effect on the therapeutic potential of hAdMSCs. Moreover, these results indicate that intra-articular administration of CS might influence the biological behavior of resident stem cells increasing their chondrogenic differentiation and inherent immunomodulatory activity. To our knowledge, this is the first in vitro study reporting this combination.
Introduction
Osteoarthritis is one of the most common chronic health conditions associated with pain and disability (Allen and Golightly, 2015), being the most common form of arthritis followed by rheumatoid arthritis, gout, lupus, fibromyalgia, and others (Barbour et al., 2017). Osteoarthritis is a highly prevalent joint disorder worldwide affecting approximately 15% of the population (Johnson and Hunter, 2014), which is mainly characterized by cartilage destruction (Manek and Lane, 2000), affecting to the entire joint structure (Pelletier et al., 2001).
The traditional treatments of osteoarthritis are mainly based on a combination of pharmacological and non-pharmacological options aiming to reduce pain and gain quality of life in the patient (Hunter, 2011). These treatments do not address the cause of the disease being frequently associated to renal, cardiovascular, and gastrointestinal secondary effects mainly due to the use of nonsteroidal anti-inflammatory drugs. In the most severe cases, surgical joint prosthesis implantation is performed, also with serious side effects (Tonge et al., 2014).
At the present, great efforts are being made to develop new therapeutic tools for arthritic diseases. In this sense, platelet-rich plasma (PRP) has emerged in the last years as a promising therapeutic option for cartilage degeneration, demonstrating its chondroprotective effect (Andia and Maffulli, 2013; Moussa et al., 2017) and a temporal pain relief together with a functional improvement of the involved joint (Laver et al., 2017). However, the variation between different preparations makes difficult the establishment of its therapeutic potential (Richards et al., 2016), and postinjection pain seems to be more frequent after PRP intra-articular administration than with other treatments using the same route (Ornetti et al., 2016).
At the end of the 1990s, a method based on the exposure of blood leukocyte to pyrogen-free surfaces (medical-grade glass beads) has become a valuable option for the treatment of these pathologies. This autologous conditioned serum (ACS), firstly described by Meijer et al. in 2003, has shown a high concentration on interleukin 1 receptor antagonist (IL-1Ra) and other anti-inflammatory cytokines and growth factors such as interleukins 4 (IL-4), 6 (IL-6), and 10 (IL-10) epidermal growth factor (EGF), vascular endothelial growth factor (VEGF), hepatocyte growth factor (HGF), insulin-like growth factor 1 (IGF1), platelet-derived growth factor (PDGF), and transforming growth factor beta 1 (TGFβ1) (Meijer et al., 2003; Wehling et al., 2007). As IL-1 is a major contributor to osteoarthritis pathogenesis, stimulating chondrocytes and synovial fibroblasts to upregulate matrix metalloproteases that damage the cartilage, the blockade of this cytokine offers important benefits (Richards et al., 2016). This biological treatment has been assayed in veterinary medicine for the treatment of osteoarthritis in horses (Frisbie et al., 2007). Clinical trials have demonstrated its beneficial effect in knee osteoarthritis (Baltzer et al., 2009; Baselga García-Escudero and Miguel Hernández Trillos, 2015), lumbar radicular compression (Becker et al., 2007; Godek, 2016), supraspinatus pathology (von Wehren et al., 2016), and after anterior cruciate ligament surgery (Darabos et al., 2011) being superior to traditional treatments such as hyaluronan (for osteoarthritis) and triamcinolone (for lumbar radicular compression).
On the other hand, the administration of mesenchymal stem cell (MSC)-based therapies, either locally or systemically, has emerged in the last years as an encouraging therapeutic tool for the treatment of inflammatory-based diseases (de Witte et al., 2015). It has been demonstrated that they can also decrease the adverse effect of IL-1β in osteoarthritis (Jin et al., 2017). In veterinary medicine, intra-articular injections of MSCs have been administered in horses using xenogeneic, allogeneic, and autologous approaches (Pigott et al., 2013). These cells have demonstrated to exert an immunomodulatory effect on cells from the innate and adaptive immune system. More recently, they have shown very promising results in clinical trials addressing the treatment of osteoarticular diseases (Jorgensen and Noël, 2011; Pers and Jorgensen, 2016).
Putting together these new therapies, a review comparing bone marrow aspirates, PRP, and ACS as alternative treatment options for musculoskeletal diseases concluded that while bone marrow aspirates and PRP are successful in treating early arthritis, ACS is a better option for chronic joint arthritis (Patel and Strauss, 2015). In a recent review from Fotouhi et al., different treatments for knee osteoarthritis were discussed from a historical perspective. The authors suggest that PRP, stromal vascular fraction, and ACS seem to have favorable and promising results (Fotouhi et al., 2018).
In this work, we hypothesized that the rich content on anti-inflammatory proteins and growth factors of pooled ACS (CS) may exert a beneficial effect on the biological activity of human adipose-derived MSCs (hAdMSCs). Our results indicate that CS is an appropriate vehicle for MSCs administration, as their viability and proliferative behavior are comparable with standard culture conditions. Moreover, the in vitro experiments showed that CS induces the chondrogenic differentiation of hAdMSCs and enhances their immunomodulatory potential. This study suggests that CS sensitization may exert a beneficial effect on the therapeutic potential of hAdMSCs that needs to be further addressed in an appropriate in vivo model to confirm these results. To our knowledge, this is the first in vitro study reporting this combination.
Finally, it is important to note that our in vitro observations could be translated to the clinical situation when ACS is intra-articularly administered. In this sense, the induction of chondrogenic differentiation and the enhancement of the immunomodulatory potential of articular resident stem cells could be triggered by the administration of ACS.
Material and Methods
Collection of Human Conditioned Serum
Human CS was obtained from three volunteers as previously described (Wehling et al., 2007) by incubating 10 ml of venous blood in the presence of medical-grade glass beads (Orthogen, Düsseldorf, Germany) for 6 h at 37°C to ensure physiological conditions. These glass beads induce the production of anti-inflammatory cytokines (IL-1Ra, IL-10, and IL-6) and growth factors (EGF, PDGF, TGF-β1, VEGF, HGF, and IGF-1) by white blood cells. After incubation, the blood-filled syringes were centrifuged at room temperature in a universal compact centrifuge (Hermle Z 200 A) in a fixed angle rotor at 2,100 g for 10 min. The serum supernatant was filtered by Millex GP 0.22 µm syringe tip filter, pooled, filtered again, aliquoted, and stored at −20°C until use. The biological samples were obtained after written informed consent under the auspices of the appropriate research and ethics committees and in accordance with the Declaration of Helsinki. This study was approved by Minimally Invasive Surgery Centre Research Ethics Committee (approval number: SITC215).
Isolation and Expansion of Human Adipose Mesenchymal Stem Cells
The human adipose-derived mesenchymal stem cells (hAdMSCs) were obtained from lipoaspirated human adipose tissue from healthy adult donors as previously described (DelaRosa et al., 2012). Briefly, tissue was washed with phosphate-buffered saline (PBS) solution and digested at 37°C for 30 min with 0.075% collagenase type I (Invitrogen, Carlsbad, CA, USA) in PBS. Samples were then washed with 10% fetal bovine serum (FBS), incubated with ammonium chloride 160 mM at room temperature for 10 min to lyse red blood cells, suspended in Dulbecco’s modified Eagle’s medium (DMEM) containing 10% FBS, and filtered by a 40 μm nylon mesh. After cell seeding, hAdMSCs were expanded at 37°C and 5% CO2, with medium replacement every 7 days. When 90% of confluence was reached, cells were detached with a 0.25% trypsin solution and seeded again at a density of 5,000 cells/cm2 into new culture flasks to continue cell expansion. Three cell lines from different healthy donors were used for subsequent experiments. The biological samples were obtained after written informed consent under the auspices of the appropriate Research and Ethics Committees and in accordance with the Declaration of Helsinki. This study was approved by Minimally Invasive Surgery Centre Research Ethics Committee (approval number: SITC215).
Phenotypic Analysis
For flow cytometric analysis by fluorescence-activated cell sorting (FACS), the hAdMSCs were cultured in the presence of CS or FBS (GE Healthcare Hyclone, GE Healthcare, Chicago, IL, USA) at different concentrations (2.5, 5, and 10%) in the culture medium (DMEM supplemented with 1% penicillin/streptomycin and 1% glutamine). After 6 days, cells were trypsinized and labeled with fluorescein isothiocyanate (FITC)-conjugated human monoclonal antibodies (mAbs) against CD44, CD45, CD90, and human leucocyte antigen DR (HLA-DR), and phycoerythrin (PE)-conjugated human mAb against CD73 (BD Biosciences, San Jose, CA, USA). After incubating 200,000 cells with manufacturer’s suggested concentration of mAbs in PBS with 2% FBS at 4°C for 30 min, cells were washed with PBS and resuspended again. A FACScalibur cytometer (BD Biosciences, San Jose, CA, USA) was used for the cytometric analysis. A total of 100,000 events were acquired, and a selection based on forward and side scatter properties was performed before fluorescence analysis with CellQuest software (BD Biosciences, San Jose, CA, USA). Mean relative fluorescence intensity was obtained by dividing the mean fluorescent intensity by the mean fluorescent intensity of its negative control (isotype-matched antibodies).
Cell Proliferation Assays
Cell proliferation was determined using Cell Counting Kit 8 (CCK-8), which measures the activity of living cells by assessing their mitochondrial activity. The reaction product is directly proportional to the number of living cells and can be spectrophotometrically quantified. To perform this determination, cells were seeded at a density of 5,000 cells/well in 96-well plates in DMEM medium with different concentrations (2.5, 5, and 10%) of CS or FBS. The proliferative activity was measured at 0 and 6 days. For that, the culture medium was replaced by 200 μl of phenol-red free DMEM, and 20 μl of CCK-8 (WVR, Radnor, PA, USA) were added. The absorbance of the supernatants was read 2 h later at 450 nm.
Viability Determination
The viability of the hAdMSCs cultured for 6 days in standard culture conditions (10% FBS) and with different concentrations (2.5, 5, and 10%) of CS was calculated by a trypan blue dye-exclusion assay using the Countess® Automated Cell Counter (Thermo Fisher Scientific Inc., Waltham, MA, USA).
Chondrogenic Differentiation Assay
For the chondrogenic differentiation assay, hAdMSCs at 80% of confluence with CS or FBS at different concentrations (2.5, 5, and 10%) were cultured for 15 days in standard culture medium (DMEM supplemented with 1% penicillin/streptomycin and 1% glutamine), with or without StemPro Chondrogenesis Supplement (Thermo Fisher Scientific, Gibco™) and replacing the medium every 3 days. To evidence the chondrogenic differentiation, cultured cells were fixed, and an Alcian Blue 8GX staining was performed, as recommended by the International Society of Cellular Therapy (Dominici et al., 2006). To quantify the differentiation degree, cells were lysed with 6 M guanidine–HCl to extract the colorant, and the absorbance of extracts was read at 600 nm.
Active and Latent TGF-β Determination on Conditioned Serum
The quantification of active and latent TGF-β1 in CS was performed by emzyme-linked immunosorbent assay (ELISA) using the LEGEND MAX™ Free active TGF-β1 and LEGEND MAX™ Latent TGF-β1 ELISA kits (Biologend, San Diego, CA, USA) according to manufacturer’s instructions. Active and latent TGF-β1 were directly measured in CS and in a 1:10 dilution (CS in DMEM).
Lymphocytes Isolation and Preservation
Peripheral blood lymphocytes (PBLs) were isolated from peripheral blood samples of three healthy donors obtained after written informed consent under the auspices of the appropriate research and ethics committees and in accordance with the Declaration of Helsinki. Peripheral blood was diluted in PBS and centrifuged over Histopaque-1077 (Sigma, St. Louis, MO, USA) at 400 g for 20 min with brakes off. PBL-containing fraction was recovered, washed twice with PBS, and resuspended in FBS with 10% dimethyl sulfoxide (DMSO) for liquid nitrogen preservation. For thawing, aliquots were submerged in 37°C water and diluted in 10 ml Roswell Park Memorial Institute (RPMI) medium 1640. Subsequent centrifugation at 1,500 rpm for 5 min was performed to eliminate DMSO remnants. Cell pellet was resuspended in DMEM and used for experiments.
In Vitro Stimulation of T Cells and Coculture With Conditioned Serum-Sensitized Human Adipose-Derived Mesenchymal Stem Cells
To determine the immunomodulatory effect of CS-sensitized hAdMSCs on in vitro-stimulated PBLs, a previously described protocol was used (Blazquez et al., 2014). Briefly, 200 μl of a 1 × 106 PBLs/ml suspension was seeded per well in 96-well plates. A T cell activation/expansion kit (Miltenyi Biotec Inc, San Diego, CA, USA) was used to stimulate PBLs, following the manufacturer’s recommendations. Stimulated PBLs were cocultured for 6 days in contact with previously sensitized hAdMSCs. This sensitization was performed after seeding 5,000 hAdMSCs/well in a 96-well plate, by culturing them in the presence of different concentration (2.5, 5, and 10%) of CS or FBS in the culture medium (DMEM supplemented with 1% penicillin/streptomycin and 1% glutamine) for 6 days before the coculture with PBLs in the same culture medium. Negative controls (non-stimulated PBLs) and positive controls (stimulated PBLs without hAdMSCs) were used in all the experiments.
Carboxyfluorescein Succinimidyl Ester Proliferation Assay
To determine the proliferative behavior of T lymphocytes, these cells were stained with carboxyfluorescein succinimidyl ester (CFSE) using the CellTrace™ CFSE Cell Proliferation Kit (Invitrogen, Eugene, OR, USA) according to manufacturer’s instructions. Briefly, PBLs were incubated for 10 min at 37°C with the reagent at a final concentration of 10 μM. Culture medium (DMEM supplemented with 1% penicillin/streptomycin and 1% glutamine) was then added in order to remove dye remnants. Stained PBLs were cultured in the presence of CS-sensitized hAdMSCs for 6 days as described in “In Vitro Stimulation of T Cells and Co-culture With CS-Sensitized hAdMSCs”. These PBLs in suspension were collected from wells and stained with fluorescence-labeled human mAbs against CD4 and CD8 (BD Biosciences, San Jose, CA, USA).
For the fluorescence analysis, 200,000 cells were incubated with manufacturer’s suggested concentration of mAbs in PBS with 2% FBS at 4°C for 30 min, washed with PBS, and resuspended again. The FACScalibur cytometer (BD Biosciences, San Jose, CA, USA) was used for the cytometric analysis. A total of 100,000 events were acquired, and a selection based on forward and side scatter properties was performed before fluorescence analysis with CellQuest software (BD Biosciences, San Jose, CA, USA). Isotype-matched negative control antibodies were used in all the experiments. The percentage of proliferative cells was calculated as the percentage of CFSE-low fluorescence intensity cells on gated CD4+ and CD8+ T cells.
Differentiation/Activation Markers Expression Analysis on In Vitro-Stimulated PBLs
For flow cytometric analysis of in vitro-stimulated PBLs, these cells were collected from wells by recovering the supernatant after 6 days of culture in the presence of CS-sensitized hAdMSCs. The cells were stained with fluorescence-labeled human mAbs against CD62L and CD45RA (BD Biosciences, San Jose, CA, USA).
For the fluorescence analysis, 200,000 cells were incubated with manufacturer’s suggested concentration of mAbs in PBS with 2% FBS at 4°C for 30 min, washed with PBS, and resuspended again. The FACScalibur cytometer (BD Biosciences, San Jose, CA, USA) was used for the cytometric analysis. A total of 100,000 events were acquired, and a selection based on forward and side scatter properties was performed before fluorescence analysis with CellQuest software (BD Biosciences, San Jose, CA, USA). Isotype-matched negative control antibodies were used in all the experiments. The percentage of naïve cells was determined as the percentage of CD45RA+/CD62L+ cells on forward scatter (FSC)/side scatter (SSC)-gated cells.
Statistical Analysis
Data were statistically analyzed using a one-way ANOVA test. When statistically significant differences were found, Fisher’s least significant difference test was performed comparing equivalent concentrations of CS and FBS, C− and C+, and C+ with each treatment group. The p-values ≤0.05 were considered statistically significant. All the statistical determinations were made using SPSS-21 software (SPSS Chicago, IL, USA).
Results
Phenotypic Profile of Surface Markers on Human Adipose-Derived Mesenchymal Stem Cells Cultured in the Presence of Conditioned Serum
In order to compare the phenotype of hAdMSCs cultured in the presence of FBS with those cultured in the presence of CS, the expression of stem cell markers was analyzed by flow cytometry. The stem cell phenotype of hAdMSCs cultured with 2.5, 5, and 10% of FBS or CS was determined after 6 days by flow cytometry using commercially available mAbs against CD44, CD45, CD73, CD90, and HLA-DR. Our results showed that the phenotype profiles of hAdMSCs under different conditions were similar. As shown in Figure 1, the surface marker expression was CD44+/CD45-/CD73+/CD90+/HLA-DR- for both culture conditions. However, CD90 expression was more pronounced on hAdMSCs cultured with CS.
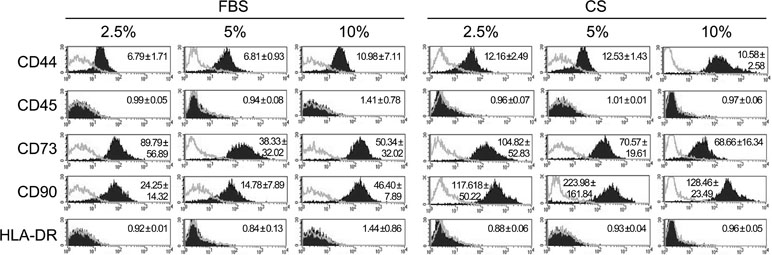
Figure 1 Phenotypic analysis of human adipose-derived mesenchymal stem cells (hAdMSCs) cultured in the presence of fetal bovine serum (FBS) or conditioned serum (CS). Different hAdMSCs cell lines (n = 3) were cultured in Dulbecco’s modified Eagle’s medium (DMEM) supplemented with 1% penicillin/streptomycin and 1% glutamine and in the presence of FBS or CS at different concentrations (2.5, 5, and 10%). After 6 days, the presence/absence of different surface markers was analyzed by flow cytometry. Representative histograms together with the relative expression of cell surface markers (mean ± SD) are shown. The relative expression is represented as mean relative fluorescence intensity, which is calculated by dividing the mean fluorescent intensity (filled histogram) by its negative control (gray-lined histogram).
Proliferative Ability of Human Adipose-Derived Mesenchymal Stem Cells Cultured in the Presence of Conditioned Serum
To determine whether CS has an effect on hAdMSCs’ proliferative behavior, the proliferation rate of hAdMSCs cultured in the presence of FBS or CS (2.5, 5, and 10%) was compared by a CCK-8 assay. The proliferation rate was quantified at day 0 (negative control) and at day 6. Our results showed no significant differences when comparing the proliferative behavior of cells cultured in the presence of different concentrations of FBS or CS. These results demonstrate that the proliferation rate of hAdMSCs was unaffected by the presence of CS. Interestingly, as shown in Figure 2, there was an increase, although it does not reach statistical significance (0.05 ≤ p ≤ 0.1), in the proliferative rate of hAdMSCs cultured in the presence of 2.5 and 10% CS (when compared with the same concentrations of FBS). Additionally, viability of hAdMSCs cultured in the different conditions was determined by a trypan blue dye-exclusion assay, without any significant difference (Supplementary Figure 1).
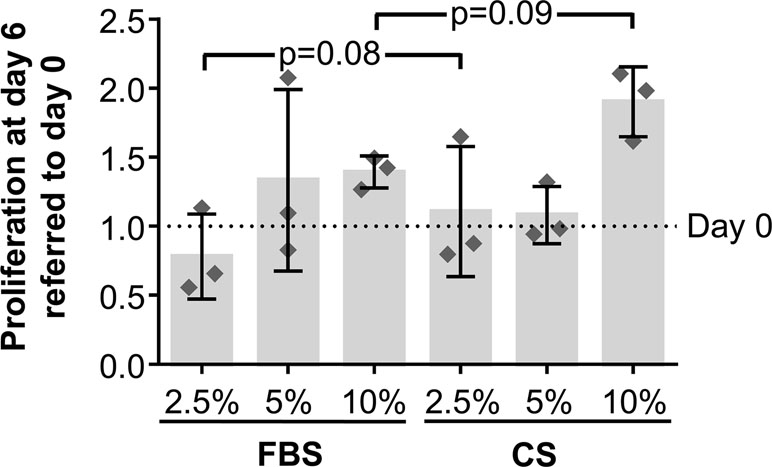
Figure 2 In vitro proliferation of hAdMSCs cultured in the presence of FBS or CS. The cells were cultured in DMEM supplemented with 1% penicillin/streptomycin and 1% glutamine and in the presence of FBS or CS at different concentrations (2.5, 5, and 10%). The proliferative behavior of cells was determined through a Cell Counting Kit 8 (CCK-8) assay, where the mitochondrial activity of living cells was spectrophotometrically quantified at 450 nm at days 0 and 6. The graph shows the increase or decrease of absorbance at day 6 normalized and expressed according to absorbance at day 0. Individual values (n = 3) as well as mean ± SD are shown. Data were statistically analyzed using a one-way ANOVA test followed by a Fisher’s least significant difference test to compare equivalent concentrations of CS and FBS. p-values <0.1 are shown.
Chondrogenic Differentiation of Human Adipose-Derived Mesenchymal Stem Cells Cultured in the Presence of Conditioned Serum
In order to assess the chondrogenic differentiation potential of hAdMSCs cultured in the presence of CS, cells were in vitro cultured for 15 days with standard culture medium or chondrogenic differentiation medium. These cells were also cultured in the presence of different concentration of FBS or CS (2.5, 5, and 10%). The chondrogenic differentiation was then quantified using Alcian Blue staining, which indicates glycosaminoglycan depositions in the hAdMSCs. Our results showed that, when cultured with standard culture medium (in the absence of chondrogenic differentiation medium), no significant differences could be observed in those cells cocultured in presence of growing concentrations of CS when compared with equivalent concentrations of FBS (negative control) (Figure 3A). However, when cultured with a chondrogenic differentiation-specific medium, the glycosaminoglycan depositions were significantly higher in hAdMSCs cultured with the different concentration of CS compared with those with equivalent concentrations of FBS (positive control) (Figure 3B). In summary, these results demonstrate that under pro-chondrogenic differentiation conditions, the chondrocyte differentiation of hAdMSCs was significantly enhanced by CS.
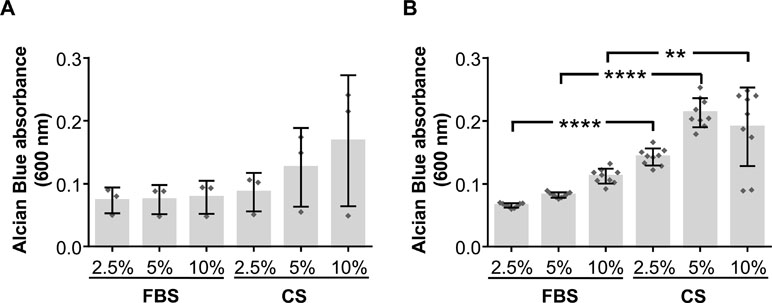
Figure 3 Chondrogenic differentiation potential of hAdMSCs cultured in the presence of FBS or CS. Cells were cultured in the presence of FBS or CS at different concentrations (2.5, 5, and 10%) for 15 days in standard medium (DMEM supplemented with 1% penicillin/streptomycin and 1% glutamine) as negative control (A) or in chondrogenic differentiation specific medium (B). Chondrogenic differentiation was confirmed by Alcian Blue 8GX staining that evidences the presence of accumulated glycosaminoglycans. For the quantification of the differentiation degree, cells were lysed to release the colorant, which was spectrophotometrically measured at 600 nm. Graphs represent individual values (A, n = 3; B, n = 9) as well as mean ± SD of three independently performed experiments. Data were statistically analyzed using a one-way ANOVA test followed by a Fisher’s least significant difference test to compare equivalent concentrations of CS and FBS. **p ≤ 0.01; ****p ≤ 0.0001.
Concentration of Active and Latent TGF-β1 in Conditioned Serum
The concentration of both active and latent TGF-β1 in CS was determined by ELISA tests. The results obtained for active TGF-β1 showed a concentration of 65.99 pg/ml in 100% CS and 0.71 pg/ml in 10% CS (1:10 dilution). For latent TGF-β1, the concentrations were 125.06 ng/ml in 100% CS and 26.24 ng/ml in 1:10 diluted CS.
Proliferative Behavior of In Vitro-Stimulated T Cells Cocultured in the Presence of Conditioned Serum-Sensitized Human Adipose-Derived Mesenchymal Stem Cells
In order to study the hypothetical effect of CS on the immunomodulatory activity of hAdMSCs, we firstly aimed to determine the proliferative ability of in vitro-stimulated T cells cultured in the presence of CS-sensitized hAdMSCs. For that, CFSE-labeled PBLs were in vitro stimulated and cocultured for 6 days with 2.5, 5, and 10% FBS-sensitized or CS-sensitized hAdMSCs. The percentage of proliferative cells was calculated as the percentage of CFSE-low fluorescence intensity cells on gated CD4+ and CD8+ T cells. As shown in Figure 4A, the proliferation of CD4+ T cells was significantly reduced when PBLs were cocultured with 10% CS-sensitized hAdMSCs, compared with positive control. Also, significant differences were found when equivalent concentrations of FBS and CS were compared, observing that PBLs cocultured with 5 and 10% CS-sensitized hAdMSCs showed a significantly lower proliferation than their FBS counterparts. Similarly, as shown in Figure 4B, the proliferation of CD8+ T cells was significantly decreased when PBLs were cocultured in the presence of 5 and 10% CS-sensitized hAdMSCs, compared with the positive control, and also when cultured with 10% CS-sensitized hAdMSCs compared with 10% FBS-sensitized hAdMSCs.
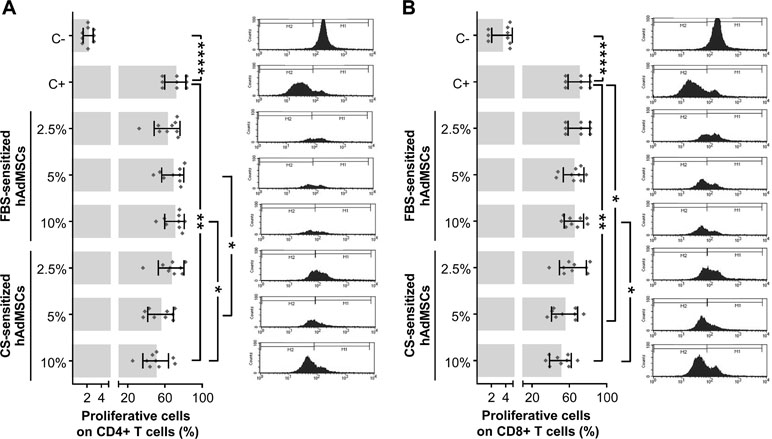
Figure 4 Proliferative ability of in vitro-stimulated T cells cocultured in the presence of FBS or CS-sensitized hAdMSCs. After 6 days of hAdMSCs sensitization with different concentrations (2.5, 5, and 10%) of FBS or CS in the culture medium (DMEM supplemented with 1% penicillin/streptomycin and 1% glutamine), these hAdMSCs (three different cell lines) were cocultured for 6 days with carboxyfluorescein succinimidyl ester (CFSE)-labeled peripheral blood lymphocytes (PBLs) (three different donors) during in vitro stimulation with T cell activation/expansion beads. As positive control (C+), stimulated PBLs without hAdMSCs were used, and as negative control (C−), non-stimulated PBLs were included. After coculture with hAdMSCs, PBLs were collected and stained with anti-CD4 and anti-CD8. The CFSE dilution assay allowed us to identify proliferative T cells (CFSE low) and non-proliferative T cells (CFSE high). Graphs representing individual values (n = 9), as well as mean ± SD of three independently performed experiments, together with representative histograms of each condition, are shown for CD4+ T cells (A) and CD8+ T cells (B). Data were statistically analyzed using a one-way ANOVA test followed by a Fisher’s least significant difference test to compare equivalent concentrations of CS and FBS, C− with C+, and C+ with each treatment group. *p ≤ 0.05; **p ≤ 0.01; ****p ≤ 0.0001.
Differentiation of In Vitro-Stimulated T Cells Cocultured in the Presence of Conditioned Serum-Sensitized Human Adipose-Derived Mesenchymal Stem Cells
To continue with the study of the immunomodulatory activity of CS-sensitized hAdMSCs, the percentage of naïve cells (CD45RA+/CD62L+) was determined by flow cytometry on in vitro-stimulated T cells cultured in the presence of CS-sensitized hAdMSCs. For that, CFSE-labeled PBLs were in vitro stimulated and cocultured with 2.5, 5, and 10% FBS- or CS-sensitized hAdMSCs. After 6 days, PBLs were stained with fluorescence-labeled human mAbs against CD62L and CD45RA and analyzed by flow cytometry. The percentage of naïve cells was determined as the percentage of CD45RA+/CD62L+ cells on FSC/SSC-gated cells.
As expected, positive control (stimulated PBLs without hAdMSCs) showed a significant decrease of naïve T cells when compared with non-stimulated PBLs (negative control). Significant differences were found when comparing in vitro-stimulated PBLs cocultured with FBS-sensitized hAdMSCs and cocultured with CS-sensitized hAdMSCs. As shown in Figure 5, when PBLs were cocultured with 2.5 and 5% FBS-sensitized hAdMSCs, the percentage of naïve cells significantly decreased in comparison with positive control. Additionally, no statistically significant differences were found when comparing the different treatment groups with the positive control. Interestingly, there were significant increases in the percentage of naïve cells in those PBLs cocultured with 5 and 10% CS-sensitized hAdMSCs when compared with their FBS counterparts. These results suggest that CS sensitization in hAdMSCs induced a higher immunomodulatory effect of these stem cells against T cell activation.
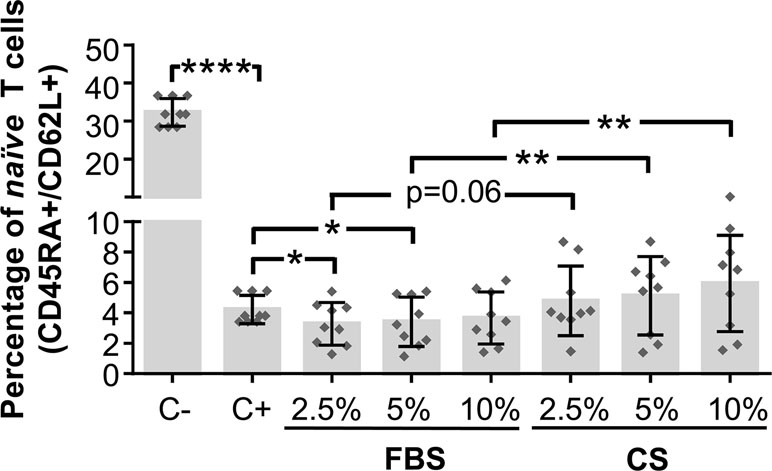
Figure 5 Activation and differentiation of in vitro-stimulated PBLs cultured in the presence of FBS or CS-sensitized hAdMSCs. After 6 days of hAdMSCs sensitization with different concentrations (2.5, 5, and 10%) of FBS or CS in the culture medium (DMEM supplemented with 1% penicillin/streptomycin and 1% glutamine), these hAdMSCs (three different cell lines) were cocultured for 6 days with PBLs (three different donors) during in vitro stimulation with T cell activation/expansion beads. As positive control (C+), stimulated PBLs without hAdMSCs were used, and as negative control (C−), non-stimulated PBLs were included. After coculture with hAdMSCs, CD45RA and CD62L co-expression was analyzed on PBLs by multicolor flow cytometry on FSC/SSC-gated cells. The graph shows individual values (n = 9) for the percentage of naïve T cells (CD45RA+/CD62L+), as well as mean ± SD of three independently performed experiments. Data were statistically analyzed using a one-way ANOVA test followed by a Fisher’s least significant difference test to compare equivalent concentrations of CS and FBS, C− with C+, and C+ with each treatment group. *p ≤ 0.05; **p ≤ 0.01; ****p ≤ 0.0001.
Discussion
Osteoarthritis is the most common form of arthritis, representing a major cause of disability, especially in elderly patients and causing an ever growing burden for health care systems (Mobasheri and Henrotin, 2015). Osteoarthritis is characterized by progressive cartilage degeneration, and emerging therapies are focused in the activation of cartilage regenerative potential to slow its degeneration and preserving joint function (Li et al., 2017). Osteoarthritis has been hypothesized to be a “chronic wound”-type disease (Scanzello et al., 2008; Wu and Henry, 2012) characterized among others by a chronically upregulated innate immune system. Interestingly, similar mechanisms are observed in chronic tendon injuries (Klatte-Schulz et al., 2018). These processes—far from perfectly understood—may have a major impact not only on osteoarthritis degenerative pathology but also on pain perception.
CS is a practical and relatively inexpensive method to generate IL-1Ra (Evans et al., 2016). There is a consensus in the literature that suggests that CS can reduce degenerative mechanisms and enhance tissue regeneration (Frizziero et al., 2013). Moreover, it is widely accepted that the local treatment is a safe and effective therapy (Fotouhi et al., 2018).
The aim of this work was to evaluate—under in vitro culture conditions—the effect of CS on the chondrogenic and immunomodulatory potential of hAdMSCs. Both CS (which contains anti-inflammatory cytokines and growth factors) and hAdMSCs (with a potent anti-inflammatory effect) are currently considered as some of the most promising emerging treatments for cartilage repair (de Girolamo et al., 2016). However, at present, there are no studies that explore the combination of these two therapies.
Our study was firstly conducted to evaluate the phenotype, viability, and proliferative behavior of hAdMSCs cultured in the presence of CS. Our results showed that the phenotypic profile and viability of hAdMSCs cocultured with CS were similar or equivalent to hAdMSCs cultured under standard culture conditions (supplemented with FBS). Uniquely, CD90 was increased in the CS groups, which could be directly related to the cell confluence (Dudakovic et al., 2014). These results suggest that, in future clinical trials aiming to combine CS and hAdMSCs, CS could be considered as an optimal delivery vehicle for stem cell administration.
Once demonstrated that CS does not exhibit any adverse effect on hAdMSCs, we aimed to determine the chondrogenic potential of hAdMSCs in the presence of CS. Interestingly, the chondrogenic differentiation of hAdMSCs was significantly enhanced by CS in the presence of a specific differentiation medium. These results may have a clinical relevance in stem cell-based therapies using CS as a vehicle and suggest that CS may improve the chondrogenic potential of implanted stem cells. Further studies should be conducted to determine if the therapeutic effect of CS (in terms of cartilage reparation) in osteoarthritis patients could be mediated, at least in part, by the chondrogenic activation of resident stem cells after intra-articular injections of CS. Interestingly, a muscle contusion mouse model showed increased numbers of satellite cells in the healing tissue after local CS injection vs saline, and clinically, this finding correlated to a significantly faster recovery of muscle injuries in athletes (Wright-Carpenter et al., 2004).
Although the identification of soluble factors involved in the chondrogenic activation of hAdMSCs is not the main issue of this paper, we hypothesize that the presence of TGF-β1 in CS could be a major mediator for the enhancement of chondrogenic differentiation. The role of TGF-β1 in the chondrogenic differentiation has been widely described in different cell lines such as MSCs (Cals et al., 2012), chondrocytes (Murphy et al., 2015), and human synovium-derived stem cells (Kim et al., 2014). Previous studies have also demonstrated that TGF-β1 is significantly increased in CS (Rutgers et al., 2010). Similarly to Rutgers et al., our quantitative analyses of TGF-β1 demonstrated that both active and latent TGF-β were abundant in CS (>50 pg/ml of active TGF-β1 and >100 ng/ml of latent TGF-β1). The hypothesis of TGF-β involvement in the chondrogenic activation of hAdMSCs could also be supported by previous studies that demonstrated that total TGF-β in CS from 80 patients was very high (97.93 ± 113.41 ng/ml) (Wehling et al., 2007). In contrast, although FBS contains total TGF-β (10–20 ng/ml), its concentration is about 10 times lower than in CS (Oida and Weiner, 2010).
Finally, it has been recently reported that IGF-1 (also present in CS) plays a role in the chondrogenic differentiation (Zhou et al., 2016), so we should not discard that the chondrogenic response of stem cells could also be mediated by IGF-1.
On the other hand, equivalent results on activated PRP have also been described. In a recent work published by Van Pham et al., the authors showed that activated PRP promoted the proliferation of hAdMSCs as well as their differentiation toward chondrocytes (Van Pham et al., 2013). Similarly, adipose- and bone marrow-derived stem cells seeded in PRP-derived scaffolds showed a higher proliferative rate and chondrocyte differentiation when compared with control cells (Xie et al., 2012). These reports suggest that both CS and PRP are providing a suitable environment for stem cell proliferation and differentiation toward chondrogenic lineage.
Regarding the differences between PRP and CS, in terms of soluble factors, the main difference has been previously described for IL-1Ra, which is high in CS but almost absent in PRP (Wehling et al., 2007; Mussano et al., 2016). This may explain the differences between CS and PRP in terms of duration of the achieved positive effects and the positive impact on the arthrologic status (Shirokova et al., 2017). Another issue with PRP upon intra-articular injection is the sudden presence of a high number of cells that originally do not belong to the joint. Both platelets and leukocytes may—in the presence of appropriate stimulus—act as amplifiers of innate immune reactions leading to transient intense pain in 1 out of 10 patients (Standford University Medical Center—Department of Radiology).
Considering that the therapeutic effect of adult stem cells has been attributed to their immunomodulatory effect in the inflammatory process, our experiments were conducted to evaluate the immunomodulatory capacity of hAdMSCs sensitized with CS. This immunomodulatory capacity was evaluated against in vitro-stimulated T cells using anti-CD2, anti-CD3, and anti-CD28 that partially mimic the stimulation by antigen-presenting cells (Trickett and Kwan, 2003). Based on previous methodologies from our group (Blazquez et al., 2014; Álvarez et al., 2018), the immunomodulation was assessed by measuring the proliferative behavior of T cells and the percentage of naïve T cells according to the co-expression of CD45RA and CD62L (Foster et al., 2004; Prabhu et al., 2016).
Our results showed that CS-sensitized hAdMSCs significantly decreased the proliferation and differentiation potential of in vitro-stimulated T cells. Although previous studies have reported that the immunomodulatory ability of MSCs can be triggered by different cytokines such as IFN-γ, TNF-α, IL-1β, or IL-17A (Prasanna et al., 2010; Gray et al., 2015; Mohammadpour et al., 2015; Sivanathan et al., 2015), here, we hypothesize that TGF-β1 may trigger this immunomodulatory effect on hAdMSCs. Supporting this hypothesis, de Witte et al. have previously shown on umbilical cord MSCs that TGF-β1-sensitized MSCs were able to significantly reduce T cell proliferation (de Witte et al., 2017). Finally, apart from TGF-β1, which has been also associated to MSCs migration and proliferation (Sun et al., 2013; Zhang et al., 2015; Tyurin-Kuzmin et al., 2016), the presence of different growth factors in CS such as VEGF or HGF has been demonstrated to be a key factor for the therapeutic effect of MSCs (Deuse et al., 2009; Tögel et al., 2009; Zisa et al., 2009).
To our knowledge, this is the first in vitro study that analyzes the combination of CS and hAdMSCs. Here, we demonstrate that CS enhances the chondrogenic potential and immunomodulatory activity of hAdMSCs, suggesting that CS pre-sensitization or in vivo coadministration may have a beneficial effect on the therapeutic potential of hAdMSCs. Moreover, these results suggest that intra-articular administration of CS might influence the biological behavior of resident stem cells, increasing their chondrogenic differentiation and immunomodulatory activity.
Author Contributions
RB, FS, and JC conceived and designed the experiments. VA, RB, and JC performed the experiments and analyzed the data. RB, FS, JR, and JC wrote the manuscript. EL and FM revised the statistical analysis, English grammar, manuscript discussion, and revision. All authors read and approved the final manuscript.
Funding
This work was supported in part by Zaraclinics S.L.; ISCIII grants (CP17/00021, MS17/00021, PI18/0911 to JGC), co-funded by ERDF/ESF, “Investing in your future”; one grant from Consejería de Economía e Infraestructuras, Junta de Extremadura co-funded by ERDF (IB16168 to JGC); one grant from GobEx (Ayuda a grupos catalogados de la Junta de Extremadura, GR18199) and by CIBERCV (CB16/11/00494 to FMSM). The funders had no role in study design, data collection, analysis and interpretation, and decision to publish or preparation of the manuscript.
Conflict of Interest Statement
The author JR is an employee of Orthogen AG.
The remaining authors declare that this research was conducted in the absence of any commercial or financial relationships that could be construed as a potential conflict of interest.
Acknowledgments
In vitro experiments have been performed by the ICTS “NANBIOSIS,” more specifically by the Unit 14—Cell Therapy of Jesus Uson Minimally Invasive Surgery Center (CCMIJU).
Supplementary Material
The Supplementary Material for this article can be found online at: https://www.frontiersin.org/articles/10.3389/fphar.2019.00699/full#supplementary-material
References
Allen, K. D., Golightly, Y. M. (2015). Epidemiology of osteoarthritis: state of the evidence. Curr. Opin. Rheumatol. 27, 276–283. doi: 10.1097/BOR.0000000000000161
Álvarez, V., Sánchez-Margallo, F. M., Macías-García, B., Gómez-Serrano, M., Jorge, I., Vázquez, J., et al. (2018). The immunomodulatory activity of extracellular vesicles derived from endometrial mesenchymal stem cells on CD4+ T cells is partially mediated by TGFbeta. J. Tissue Eng. Regen. Med. 12, 2088–2098. doi: 10.1002/term.2743
Andia, I., Maffulli, N. (2013). Platelet-rich plasma for managing pain and inflammation in osteoarthritis. Nat. Rev. Rheumatol. 9, 721–730. doi: 10.1038/nrrheum.2013.141
Baltzer, A. W. A., Moser, C., Jansen, S. A., Krauspe, R. (2009). Autologous conditioned serum (Orthokine) is an effective treatment for knee osteoarthritis. Osteoarthr. Cartil. 17, 152–160. doi: 10.1016/j.joca.2008.06.014
Barbour, K. E., Helmick, C. G., Boring, M., Brady, T. J. (2017). Vital signs: prevalence of doctor-diagnosed arthritis and arthritis-attributable activity limitation - united states, 2013-2015. MMWR Morb. Mortal. Wkly. Rep. 66, 246–253. doi: 10.15585/mmwr.mm6609e1
Baselga García-Escudero, J., and Miguel Hernández Trillos, P. (2015). Treatment of osteoarthritis of the knee with a combination of autologous conditioned serum and physiotherapy: a two-year observational study. PLoS One 10, e0145551. doi: 10.1371/journal.pone.0145551
Becker, C., Heidersdorf, S., Drewlo, S., de Rodriguez, S. Z., Krämer, J., Willburger, R. E. (2007). Efficacy of epidural perineural injections with autologous conditioned serum for lumbar radicular compression: an investigator-initiated, prospective, double-blind, reference-controlled study. Spine 32, 1803–1808. doi: 10.1097/BRS.0b013e3181076514
Blazquez, R., Sanchez-Margallo, F. M., de la Rosa, O., Dalemans, W., Alvarez, V., Tarazona, R., et al. (2014). Immunomodulatory potential of human adipose mesenchymal stem cells derived exosomes on in vitro stimulated t cells. Front. Immunol. 5, 556. doi: 10.3389/fimmu.2014.00556
Cals, F. L. J., Hellingman, C. A., Koevoet, W., Baatenburg de Jong, R. J., van Osch, G. J. V. M. (2012). Effects of transforming growth factor-β subtypes on in vitro cartilage production and mineralization of human bone marrow stromal-derived mesenchymal stem cells. J. Tissue Eng. Regen. Med. 6, 68–76. doi: 10.1002/term.399
Darabos, N., Haspl, M., Moser, C., Darabos, A., Bartolek, D., Groenemeyer, D. (2011). Intraarticular application of autologous conditioned serum (ACS) reduces bone tunnel widening after ACL reconstructive surgery in a randomized controlled trial. Knee Surg. Sports Traumatol. Arthrosc. Off. J. ESSKA 19 Suppl 1, S36–46. doi: 10.1007/s00167-011-1458-4
de Girolamo, L., Kon, E., Filardo, G., Marmotti, A. G., Soler, F., Peretti, G. M., et al. (2016). Regenerative approaches for the treatment of early OA. Knee Surg. Sports Traumatol. Arthrosc. 24, 1826–1835. doi: 10.1007/s00167-016-4125-y
de Witte, S. F. H., Franquesa, M., Baan, C. C., Hoogduijn, M. J. (2015). Toward development of imesenchymal stem cells for immunomodulatory therapy. Front. Immunol. 6, 648. doi: 10.3389/fimmu.2015.00648
de Witte, S. F. H., Merino, A. M., Franquesa, M., Strini, T., van Zoggel, J. A. A., Korevaar, S. S., et al. (2017). Cytokine treatment optimises the immunotherapeutic effects of umbilical cord-derived MSC for treatment of inflammatory liver disease. Stem Cell Res. Ther. 8, 140. doi: 10.1186/s13287-017-0590-6
DelaRosa, O., Sánchez-Correa, B., Morgado, S., Ramírez, C., del Río, B., Menta, R., et al. (2012). Human adipose-derived stem cells impair natural killer cell function and exhibit low susceptibility to natural killer-mediated lysis. Stem Cells Dev. 21, 1333–1343. doi: 10.1089/scd.2011.0139
Deuse, T., Peter, C., Fedak, P. W. M., Doyle, T., Reichenspurner, H., Zimmermann, W. H., et al. (2009). Hepatocyte growth factor or vascular endothelial growth factor gene transfer maximizes mesenchymal stem cell-based myocardial salvage after acute myocardial infarction. Circulation 120, S247–254. doi: 10.1161/CIRCULATIONAHA.108.843680
Dominici, M., Blanc, K. L., Mueller, I., Slaper-Cortenbach, I., Marini, F. C., Krause, D. S., et al. (2006). Minimal criteria for defining multipotent mesenchymal stromal cells. Cytotherapy 8, 315–317. doi: 10.1080/14653240600855905
Dudakovic, A. D., Camilleri, E., Riester, S. M., Lewallen, E. A., Kvasha, S., Chen, X., et al. (2014). High-resolution molecular validation of self-renewal and spontaneous differentiation in adipose-tissue derived human mesenchymal stem cells cultured in human platelet lysate. J. Cell. Biochem. 115, 1816–1828. doi: 10.1002/jcb.24852
Evans, C. H., Chevalier, X., Wehling, P. (2016). Autologous conditioned serum. Phys. Med. Rehabil. Clin. N. Am. 27, 893–908. doi: 10.1016/j.pmr.2016.06.003
Foster, A. E., Marangolo, M., Sartor, M. M., Alexander, S. I., Hu, M., Bradstock, K. F., et al. (2004). Human CD62L-memory T cells are less responsive to alloantigen stimulation than CD62L+ naive T cells: potential for adoptive immunotherapy and allodepletion. Blood 104, 2403–2409. doi: 10.1182/blood-2003-12-4431
Fotouhi, A., Maleki, A., Dolati, S., Aghebati-Maleki, A., Aghebati-Maleki, L. (2018). Platelet rich plasma, stromal vascular fraction and autologous conditioned serum in treatment of knee osteoarthritis. Biomed. Pharmacother. 104, 652–660. doi: 10.1016/j.biopha.2018.05.019
Frisbie, D. D., Kawcak, C. E., Werpy, N. M., Park, R. D., McIlwraith, C. W. (2007). Clinical, biochemical, and histologic effects of intra-articular administration of autologous conditioned serum in horses with experimentally induced osteoarthritis. Am. J. Vet. Res. 68, 290–296. doi: 10.2460/ajvr.68.3.290
Frizziero, A., Giannotti, E., Oliva, F., Masiero, S., Maffulli, N. (2013). Autologous conditioned serum for the treatment of osteoarthritis and other possible applications in musculoskeletal disorders. Br. Med. Bull. 105, 169–184. doi: 10.1093/bmb/lds016
Godek, P. (2016). Use of autologous serum in treatment of lumbar radiculopathy pain. Ortop. Traumatol. Rehabil. 18, 11–20. doi: 10.5604/15093492.1198829
Gray, A., Maguire, T., Schloss, R., Yarmush, M. L. (2015). Identification of IL-1β and LPS as optimal activators of monolayer and alginate-encapsulated mesenchymal stromal cell immunomodulation using design of experiments and statistical methods. Biotechnol. Prog. 31, 1058–1070. doi: 10.1002/btpr.2103
Hunter, D. J. (2011). Pharmacologic therapy for osteoarthritis–the era of disease modification. Nat. Rev. Rheumatol. 7, 13–22. doi: 10.1038/nrrheum.2010.178
Jin, R., Shen, M., Yu, L., Wang, X., Lin, X. (2017). Adipose-Derived stem cells suppress inflammation induced by IL-1β through down-regulation of P2X7R mediated by miR-373 in chondrocytes of osteoarthritis. Mol. Cells 40, 222–229. doi: 10.14348/molcells.2017.2314
Johnson, V. L., Hunter, D. J. (2014). The epidemiology of osteoarthritis. Best Pract. Res. Clin. Rheumatol. 28, 5–15. doi: 10.1016/j.berh.2014.01.004
Jorgensen, C., Noël, D. (2011). Mesenchymal stem cells in osteoarticular diseases. Regen. Med. 6, 44–51. doi: 10.2217/rme.11.80
Kim, Y. I., Ryu, J.-S., Yeo, J. E., Choi, Y. J., Kim, Y. S., Ko, K., et al. (2014). Overexpression of TGF-β1 enhances chondrogenic differentiation and proliferation of human synovium-derived stem cells. Biochem. Biophy.s Res. Commun. 450, 1593–1599. doi: 10.1016/j.bbrc.2014.07.045
Klatte-Schulz, F., Minkwitz, S., Schmock, A., Bormann, N., Kurtoglu, A., Tsitsilonis, S., et al. (2018). Different achilles tendon pathologies show distinct histological and molecular characteristics. Int. J. Mol. Sci. 19, E4040. doi: 10.3390/ijms19020404
Laver, L., Marom, N., Dnyanesh, L., Mei-Dan, O., Espregueira-Mendes, J., Gobbi, A. (2017). PRP for degenerative cartilage disease: a systematic review of clinical studies. Cartilage 8, 341–364. doi: 10.1177/1947603516670709
Li, M., Xiao, R., Li, J. B., Zhu, Q. (2017). Regenerative approaches for cartilage repair in the treatment of osteoarthritis. Osteoarthr. Cartil. 25, 1577–1587. doi: 10.1016/j.joca.2017.07.004
Manek, N. J., Lane, N. E. (2000). Osteoarthritis: current concepts in diagnosis and management. Am. Fam. Physician 61, 1795–1804.
Meijer, H., Reinecke, J., Becker, C., Tholen, G., Wehling, P. (2003). The production of anti-inflammatory cytokines in whole blood by physico-chemical induction. Inflamm. Res. 52, 404–407. doi: 10.1007/s00011-003-1197-1
Mobasheri, A., Henrotin, Y. (2015). Biomarkers of (osteo)arthritis. Biomarkers 20, 513–518. doi: 10.3109/1354750X.2016.1140930
Mohammadpour, H., Pourfathollah, A. A., Zarif, M. N., Tahoori, M. T. (2015). TNF-α modulates the immunosuppressive effects of MSCs on dendritic cells and T cells. Int. Immunopharmacol. 28, 1009–1017. doi: 10.1016/j.intimp.2015.07.045
Moussa, M., Lajeunesse, D., Hilal, G., El Atat, O., Haykal, G., Serhal, R., et al. (2017). Platelet rich plasma (PRP) induces chondroprotection via increasing autophagy, anti-inflammatory markers, and decreasing apoptosis in human osteoarthritic cartilage. Exp. Cell Res. 352, 146–156. doi: 10.1016/j.yexcr.2017.02.012
Murphy, M. K., Huey, D. J., Hu, J. C., Athanasiou, K. A. (2015). TGF-β1, GDF-5, and BMP-2 stimulation induces chondrogenesis in expanded human articular chondrocytes and marrow-derived stromal cells. Stem Cells 33, 762–773. doi: 10.1002/stem.1890
Mussano, F., Genova, T., Munaron, L., Petrillo, S., Erovigni, F., Carossa, S. (2016). Cytokine, chemokine, and growth factor profile of platelet-rich plasma. Platelets 27, 467–471. doi: 10.3109/09537104.2016.1143922
Oida, T., Weiner, H. L. (2010). Depletion of TGF-β from fetal bovine serum. J. Immunol. Methods 362, 195–198. doi: 10.1016/j.jim.2010.09.008
Ornetti, P., Nourissat, G., Berenbaum, F., Sellam, J., Richette, P., Chevalier, X., et al. (2016). Does platelet-rich plasma have a role in the treatment of osteoarthritis? Joint Bone Spine 83, 31–36. doi: 10.1016/j.jbspin.2015.05.002
Patel, D. N., Strauss, E. J. (2015). Alternative therapeutic modalities in sports medicine. Bull. Hosp. Jt. Dis. 2013 73, 122–127.
Pelletier, J. P., Martel-Pelletier, J., Abramson, S. B. (2001). Osteoarthritis, an inflammatory disease: potential implication for the selection of new therapeutic targets. Arthritis Rheum. 44, 1237–1247. doi: 10.1002/1529-0131(200106)44:6<1237::AID-ART214>3.0.CO;2-F
Pers, Y.-M., Jorgensen, C. (2016). Adipose derived stem cells for regenerative therapy in osteoarticular diseases. Horm. Mol. Biol. Clin. Investig. 28, 113–120. doi: 10.1515/hmbci-2016-0010
Pigott, J. H., Ishihara, A., Wellman, M. L., Russell, D. S., Bertone, A. L. (2013). Investigation of the immune response to autologous, allogeneic, and xenogeneic mesenchymal stem cells after intra-articular injection in horses. Vet. Immunol. Immunopathol. 156, 99–106. doi: 10.1016/j.vetimm.2013.09.003
Prabhu, S. B., Rathore, D. K., Nair, D., Chaudhary, A., Raza, S., Kanodia, P., et al. (2016). Comparison of human neonatal and adult blood leukocyte subset composition phenotypes. PLoS One 11, e0162242. doi: 10.1371/journal.pone.0162242
Prasanna, S. J., Gopalakrishnan, D., Shankar, S. R., Vasandan, A. B. (2010). Pro-Inflammatory cytokines, IFNγ and TNFα, influence immune properties of human bone marrow and wharton jelly mesenchymal stem cells differentially. PLoS ONE 5, e9016. doi: 10.1371/journal.pone.0009016
Richards, M. M., Maxwell, J. S., Weng, L., Angelos, M. G., Golzarian, J. (2016). Intra-articular treatment of knee osteoarthritis: from anti-inflammatories to products of regenerative medicine. Phys. Sportsmed. 44, 101–108. doi: 10.1080/00913847.2016.1168272
Rutgers, M., Saris, D. B., Dhert, W. J., Creemers, L. B. (2010). Cytokine profile of autologous conditioned serum for treatment of osteoarthritis, in vitro effects on cartilage metabolism and intra-articular levels after injection. Arthritis Res. Ther. 12, R114. doi: 10.1186/ar3050
Scanzello, C. R., Plaas, A., Crow, M. K. (2008). Innate immune system activation in osteoarthritis: is osteoarthritis a chronic wound? Curr. Opin. Rheumatol. 20, 565–572. doi: 10.1097/BOR.0b013e32830aba34
Shirokova, K., Noskov, S., Shirokova, L. (2017). Comparison of clinical efficacy of platelet-rich plasma and autologous conditioned serum treatment in patients with osteoarthritis of the knee. Osteoarthr. Cartil. 25, S438. doi: 10.1016/j.joca.2017.02.756
Sivanathan, K. N., Rojas-Canales, D. M., Hope, C. M., Krishnan, R., Carroll, R. P., Gronthos, S., et al. (2015). Interleukin-17A-induced human mesenchymal stem cells are superior modulators of immunological function. Stem Cells 33, 2850–2863. doi: 10.1002/stem.2075
Standford University Medical Center—Department of Radiology. Platelet Rich Plasma (PRP) Injection - Information and instructions for patients. Available at: https://stanfordhealthcare.org/content/dam/SHC/diagnosis/p/docs/petctscan-pdf-prpbeaulieuletter.pdf [Accessed July 24, 2018]
Sun, X., Gao, X., Zhou, L., Sun, L., Lu, C. (2013). PDGF-BB-induced MT1-MMP expression regulates proliferation and invasion of mesenchymal stem cells in 3-dimensional collagen via MEK/ERK1/2 and PI3K/AKT signaling. Cell. Signal. 25, 1279–1287. doi: 10.1016/j.cellsig.2013.01.029
Tögel, F., Zhang, P., Hu, Z., Westenfelder, C. (2009). VEGF is a mediator of the renoprotective effects of multipotent marrow stromal cells in acute kidney injury. J. Cell. Mol. Med. 13, 2109–2114. doi: 10.1111/j.1582-4934.2008.00641.x
Tonge, D. P., Pearson, M. J., Jones, S. W. (2014). The hallmarks of osteoarthritis and the potential to develop personalised disease-modifying pharmacological therapeutics. Osteoarthr. Cartil. 22, 609–621. doi: 10.1016/j.joca.2014.03.004
Trickett, A., Kwan, Y. L. (2003). T cell stimulation and expansion using anti-CD3/CD28 beads. J. Immunol. Methods 275, 251–255. doi: 10.1016/S0022-1759(03)00010-3
Tyurin-Kuzmin, P. A., Zhdanovskaya, N. D., Sukhova, A. A., Sagaradze, G. D., Albert, E. A., Ageeva, L. V., et al. (2016). Nox4 and Duox1/2 mediate redox activation of mesenchymal cell migration by PDGF. PLoS ONE 11, e0154157. doi: 10.1371/journal.pone.0154157
Van Pham, P., Bui, K. H.-T., Ngo, D. Q., Vu, N. B., Truong, N. H., Phan, N. L.-C., et al. (2013). Activated platelet-rich plasma improves adipose-derived stem cell transplantation efficiency in injured articular cartilage. Stem Cell Res. Ther. 4, 91. doi: 10.1186/scrt277
von Wehren, L., Blanke, F., Todorov, A., Heisterbach, P., Sailer, J., Majewski, M. (2016). The effect of subacromial injections of autologous conditioned plasma versus cortisone for the treatment of symptomatic partial rotator cuff tears. Knee Surg. Sports Traumatol. Arthrosc. Off. J. ESSKA 24, 3787–3792. doi: 10.1007/s00167-015-3651-3
Wehling, P., Moser, C., Frisbie, D., McIlwraith, C. W., Kawcak, C. E., Krauspe, R., et al. (2007). Autologous conditioned serum in the treatment of orthopedic diseases: the orthokine therapy. BioDrugs Clin. Immunother. Biopharm. Gene Ther. 21, 323–332. doi: 10.2165/00063030-200721050-00004
Wright-Carpenter, T., Opolon, P., Appell, H. J., Meijer, H., Wehling, P., Mir, L. M. (2004). Treatment of muscle injuries by local administration of autologous conditioned serum: animal experiments using a muscle contusion model. Int. J. Sports Med. 25, 582–587. doi: 10.1055/s-2004-821303
Wu, Q., Henry, J. L., (2012). “Toll-like Receptors: at the intersection of osteoarthritis pathology and pain,” in Principles of Osteoarthritis-Its Definition, Character, Derivation and Modality-Related Recognition. Ed. Rothschild, B. M. (London, UK: InTech), 429–444. Available at: http://www.intechopen.com/books/principles-of-osteoarthritis-its-definition-character-derivation-and-modalityrelated-recognition/toll-like-receptors-at-the-intersection-of-osteoarthritis-pathology-and-pain doi: 10.5772/27504
Xie, X., Wang, Y., Zhao, C., Guo, S., Liu, S., Jia, W., et al. (2012). Comparative evaluation of MSCs from bone marrow and adipose tissue seeded in PRP-derived scaffold for cartilage regeneration. Biomaterials 33, 7008–7018. doi: 10.1016/j.biomaterials.2012.06.058
Zhang, F., Ren, T., Wu, J., Niu, J. (2015). Small concentrations of TGF-β1 promote proliferation of bone marrow-derived mesenchymal stem cells via activation of Wnt/β-catenin pathway. Indian J. Exp. Biol. 53, 508–513.
Zhou, Q., Li, B., Zhao, J., Pan, W., Xu, J., Chen, S. (2016). IGF-I induces adipose derived mesenchymal cell chondrogenic differentiation in vitro and enhances chondrogenesis in vivo. In Vitro Cell. Dev. Biol. Anim. 52, 356–364. doi: 10.1007/s11626-015-9969-9
Keywords: autologous conditioned serum, mesenchymal stem cells, chondrogenic, immunomodulation, osteoarthritis, Orthokine
Citation: Blázquez R, Sánchez-Margallo FM, Reinecke J, Álvarez V, López E, Marinaro F and Casado JG (2019) Conditioned Serum Enhances the Chondrogenic and Immunomodulatory Behavior of Mesenchymal Stem Cells. Front. Pharmacol. 10:699. doi: 10.3389/fphar.2019.00699
Received: 27 November 2018; Accepted: 29 May 2019;
Published: 28 June 2019.
Edited by:
Mohamed M. Abdel-Daim, Suez Canal University, EgyptReviewed by:
Islam M. Saadeldin, King Saud University, Saudi ArabiaYasuhiko Tabata, Kyoto University, Japan
Ayman Samir Farid, Benha University, Egypt
Copyright © 2019 Blázquez, Sánchez-Margallo, Reinecke, Álvarez, López, Marinaro and Casado. This is an open-access article distributed under the terms of the Creative Commons Attribution License (CC BY). The use, distribution or reproduction in other forums is permitted, provided the original author(s) and the copyright owner(s) are credited and that the original publication in this journal is cited, in accordance with accepted academic practice. No use, distribution or reproduction is permitted which does not comply with these terms.
*Correspondence: Rebeca Blázquez, rblazquez@ccmijesususon.com
†These authors have contributed equally and share first authorship.