- 1Key Laboratory of Biorheological Science and Technology, Ministry of Education, College of Bioengineering, Chongqing University, Chongqing, China
- 2Department of Physiology, School of Basic Medical Sciences, Southwest Medical University, Luzhou, China
Salvianolic acids, the most effective and abundant compounds extracted from Salvia miltiorrhiza (Danshen), are well known for its good anti-oxidative activity. Danshen has been extensively used as a traditional medicine to treat cardiovascular-related diseases in China and other Asian countries for hundreds of years. Recently, more and more studies have demonstrated that salvianolic acids also have a good effect on the alleviation of fibrosis disease and the treatment of cancer. In vivo and in vitro experiments have demonstrated that salvianolic acids can modulate signal transduction within fibroblasts and cancer cells. It is discovered that the cancer treatment of salvianolic acids is not only because salvianolic acids promote the apoptosis of cancer cells, but also due to the inhibition of cancer-associated epithelial-mesenchymal transition processes. In this article, we review a variety of studies focusing on the comprehensive roles of salvianolic acids in the treatment of fibrosis disease and cancer. These perspectives on the therapeutic potential of salvianolic acids highlight the importance of these compounds, which could be the novel and attractive drugs for fibrosis disease and cancer.
Introduction
Salvia miltiorrhiza (Danshen) is one of the commonly used drugs in traditional Chinese medicine and has a long history of the clinical application. According to Chinese medicine records, S. miltiorrhiza can promote blood circulation and relieve congestion. Therefore, S. miltiorrhiza is widely used in patients suffered from cardiovascular diseases, hyperlipidemia, and acute ischemic stroke (Zhou et al., 2005; Wang et al., 2007). The composition of S. miltiorrhiza is complex and diverse. The active ingredients of S. miltiorrhiza are divided into water-soluble compounds and lipid-soluble compounds (Liu et al., 2007). Salvianolic acids are the most water-soluble compounds in S. miltiorrhiza. Among salvianolic acids, salvianolic acid A (Sal A) and salvianolic acid B (Sal B) are the most abundant components.
Fibrosis, a chronic stage of many diseases that affects millions of people of all racial and ages groups, is characterized by excessive deposition of extracellular matrix (ECM) and then leads to tissue structural damage and organ dysfunction (Zhang and Friedman, 2012; Krenkel and Tacke, 2017). Fibrosis is the end stage of chronic inflammatory responses caused by a variety of stimuli factors including chemical insults, autoimmune reactions, radiation, tissue injury, allergic responses, and persistent infections (Wynn, 2007). Fibrosis is the process that non-physiological scar formation which plays a crucial role in the destruction of the liver, kidney, heart, lung, and other parenchymal organs. More and more studies have shown that the development of cancer has an important relationship with fibrosis diseases. For instance, most lung cancer cases are found in the lung periphery and lower lobes, and changes in lung fibrosis also occur mainly in these areas (Nagai et al., 1992; Aubry et al., 2002). In addition, 80–90% of hepatocellular carcinomas (HCCs) develop toward cirrhosis or liver fibrosis (El-Serag, 2011). Although some great progress has been made to understanding the pathophysiological mechanism of fibrosis diseases, current therapeutic options are still limited to appear as effective anti-fibrosis agents. Currently, there are still no specific treatment drugs to prevent or reverse the fibrosis. Therefore, it is very urgent to find and develop anti-fibrosis drugs.
In the past few years, although the good therapeutic effect of salvianolic acids on cardiovascular protection and neural protection has been proved (Wang S. X. et al., 2010; Chen et al., 2011), the most important impacts of salvianolic acids are cancer treatment and alleviation of fibrosis diseases (Liu et al., 2010). The mechanism of how salvianolic acids regulate fibroblasts and cancer cells has been widely studied in recent years. In this article, we searched the references from relevant papers and PubMed databases. We showed an overview of the advances in illustrating the effects of salvianolic acids against fibrosis diseases and cancer. In addition, we classified the functional mechanisms and pharmacological activity of salvianolic acids in the treatment of fibrosis diseases and cancer. We also further summarized the therapeutic effects of salvianolic acids in animals (Table 1).
Salvianolic Acids
To date, there are more than 10 different salvianolic acids been identified and named: salvianolic acid A, B, C, D, E, F, G, etc. Sal A and Sal B are the most abundant compounds among salvianolic acids. Danshensu [(R)-3- (3, 4-Dihydroxyphenyl)-2-hydroxypropanoic acid] is the basic chemical structure of various salvianolic acids (Chen et al., 2014; Du et al., 2016). Salvianolic acid A is formed by a molecule Danshensu and two molecules caffeic acid (Xu et al., 2014). Salvianolic acid B is formed by three molecules Danshensu and a molecule caffeic acid. Salvianolic acid C is a combination of two molecules Danshensu (Tang et al., 2016). Salvianolic acid D is a dimer of caffeic acid. As is shown in Figure 1, the structure of these four salvianolic acids all contains a phenolic acid structure.
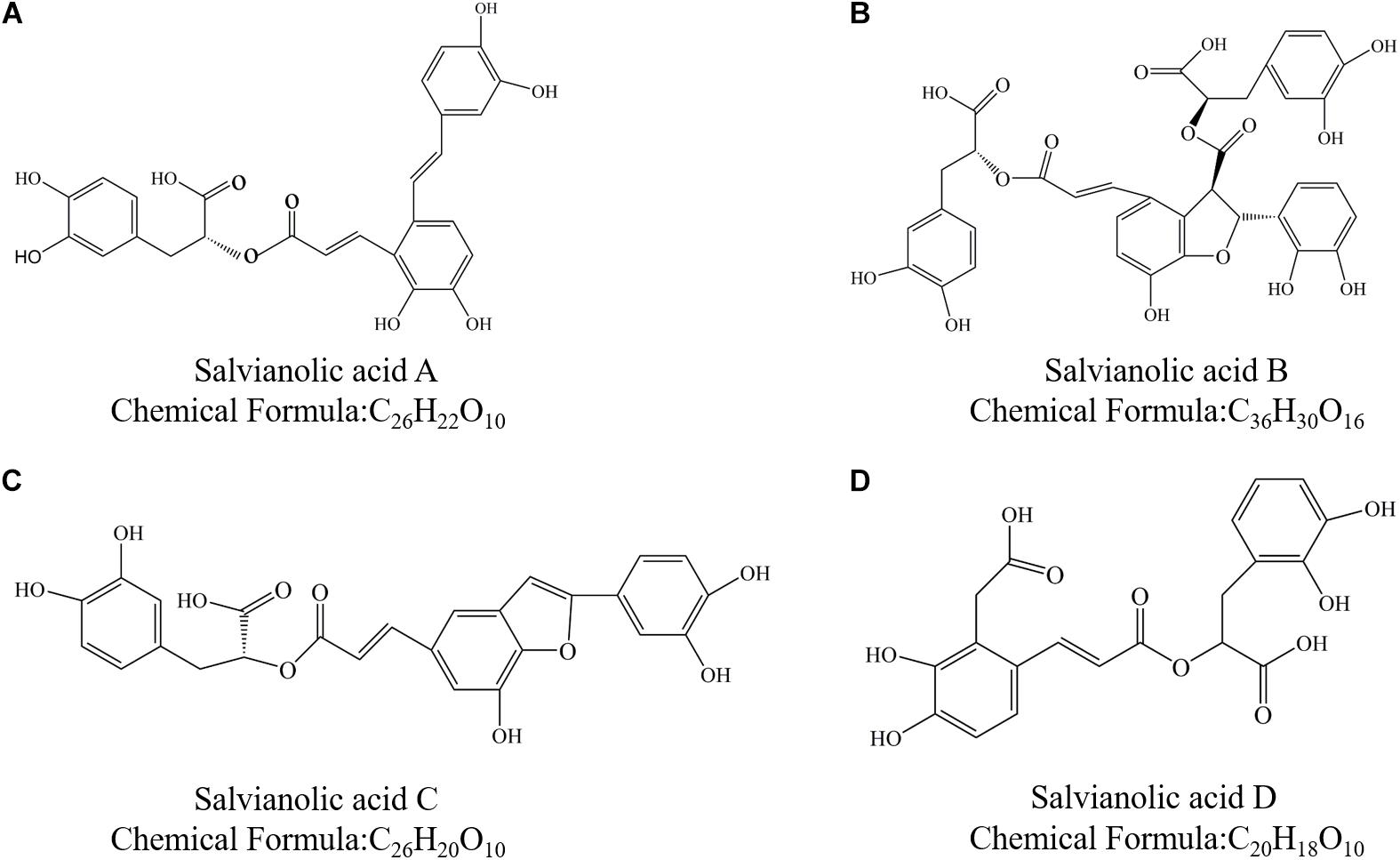
Figure 1. Chemical structure of Salvianolic acids. (A) Salvianolic acid A; (B) Salvianolic acid B; (C) Salvianolic acid C; and (D) Salvianolic acid D.
Salvianolic acids, the compounds found in danshen, have well-established bioactivities. Both in vitro and vivo, most of the salvianolic acids showed anti-inflammatory, antioxidative and the activities of free radical scavenging, and could protect cells from a variety of harmful factors (Zhao et al., 2008). Salvianolic acids have been used in traditional Chinese medicine for the treatment of cardiovascular diseases for more than a thousand years (Li et al., 2007). Compared with other phenolic compounds in danshen, salvianolic acids have more stronger antioxidant activity and other biological activities (Du et al., 2016). Although salvianolic acids could scavenge free radicals directly, they may not be existed in the body at the high concentrations. The antioxidant activity of salvianolic acids may lead to the increase in the expression of antioxidant enzymes and the decrease of the expression of pro-antioxidant enzymes, such as the activation of Nrf2/HO-1 signaling (Zhang et al., 2014).
Effects of Salvianolic Acids on Fibrosis Disease
Recent studies show that salvianolic acids have good effects on some chronic fibrosis disease, especially on liver fibrosis and pulmonary fibrosis. We summarized the functional role of salvianolic acids in the fibrosis of several organs and its potential as a novel therapeutic target (Table 2).
Salvianolic Acids and Liver Fibrosis
Liver fibrosis is a common final stage of several chronic liver diseases and is characterized by excessive deposition of ECM and collagen in response to liver injury. Many liver diseases, such as liver disease caused by drug damage, alcoholic liver disease, viral hepatitis, metabolic liver disease induced by excessive metal ionization, autoimmune liver disease and certain congenital diseases caused by liver damage, can be expected to result in liver fibrosis. Activation of hepatic stellate cells (HSCs) and their differentiation into myofibroblasts are deemed to be a critical step in the development of liver fibrosis (Friedman, 2003; Lotersztajn et al., 2005). Activation, chemotaxis and proliferation of HSCs, secretion of profibrotic cytokines and increased collagen synthesis can lead to imbalance of ECM accumulation and degradation, further causing abnormal deposition of liver fiber connective tissue, eventually leading to liver fibrosis, liver structural damage and abnormal liver function.
Numerous in vivo and in vitro experiments indicate the effectiveness of salvianolic acids for improving liver fibrosis (Figure 2). The inhibition of liver fibrosis by Sal A may relate to its anti-lipid peroxidation. As early as more than a decade ago, researchers have confirmed that Sal A inhibited the activities of aminotransferase (AlaAT) and aspartate aminotransferase (AspAT) in serum, decreased the content of hydroxyproline (Hyd) and malondialdehyde (MDA), alleviated live fibrosis, and reduced the deposition of type I and type III collagen in the liver matrix (Hu et al., 1997, 2000; Liu et al., 2000). In addition, Sal A also promoted the apoptosis of HSCs and inhibited the activation and proliferation of HSCs (Liu et al., 2000, Lin et al., 2006a). Sal A decreased the expression of Bcl-2 protein, Cyclin D1 protein and Cyclin E protein in HSCs cells, induced the expression of cyclic inhibitory proteins p21 and p27, inhibited the phosphorylation of AKT and PDGF, and enhanced the activity of caspase-3 (Lin et al., 2006a). Besides, the activity of alanine aminotransferase (ALT) in serum and the activity of MDA can be suppressed by Sal A (Hu et al., 2000; Liu et al., 2001). In general, patients with type 2 diabetes have an increased risk of developing liver fibrosis. Qiang et al. (2014) reported that Sal A prevented the pathological progression of liver fibrosis in streptozotocin (STZ)-induced diabetic rats. Sal A significantly reduced hepatocyte apoptosis and down-regulated the expression of α-smooth muscle actin (α-SMA) and transforming growth factor β1 (TGF-β1) in the liver (Qiang et al., 2014). The underlying mechanism may be linked to the reduction of oxidative stress and protection of mitochondria. In addition to Sal A, Sal B can also alleviate liver fibrosis (Li et al., 2012). Sal B inhibited the proliferation of LX-2 cells and down-regulated the expression of α-SMA protein (Hou et al., 2011). The study also found that Sal B protected the liver from damage by effectively blocking H2O2-induced mitochondrial deformation and dysfunction in the human liver cell line HL7702 (Liu et al., 2017).
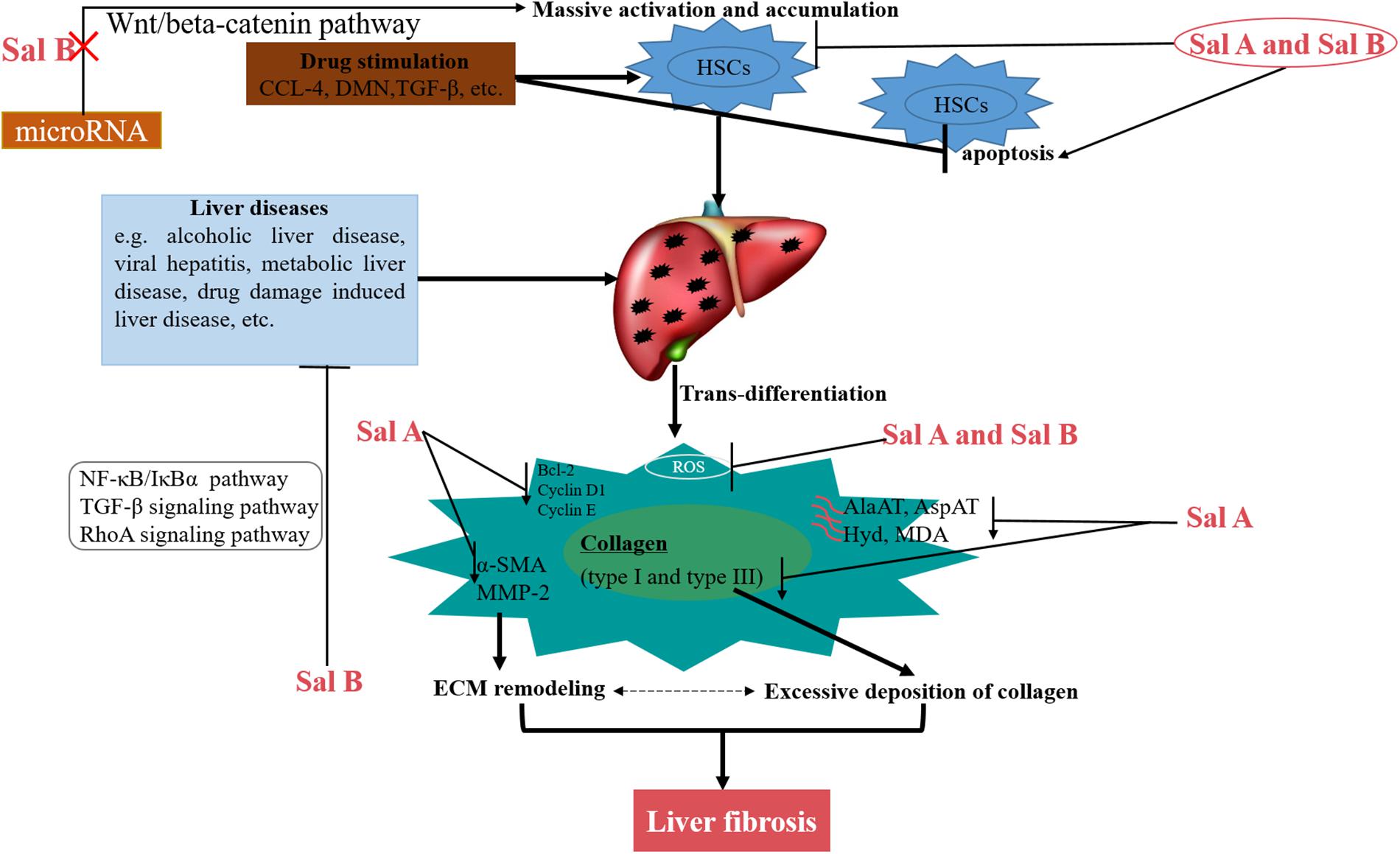
Figure 2. Schematic model of the multiple mechanisms of Salvianolic acids for liver fibrosis treatment.
The enhancement of oxidative stress is closely related to liver fibrosis (Sanchez-Valle et al., 2012; Domitrovic et al., 2013). Sal A and Sal B attenuated PDGF-induced ROS formation in HSCs through different signaling pathways (Tsai et al., 2011). The inhibition of oxidative stress by Sal A and Sal B may be through the inhibition of nicotinamide adenine dinucleotide phosphate (NADPH) oxidase (Tsai et al., 2010). Many other experiments have also demonstrated that Sal A and Sal B eliminated the accumulation of ROS, alleviated oxidative damage, and attenuated HSCs activation in hepatocytes (Lin et al., 2006b; He et al., 2010; Tsai et al., 2010). Zhu et al. (2018) demonstrated that Sal A treatment alleviated BDL- and PDGF-BB-induced liver injury and ER stress through SIRT1-mediated HSF1 deacetylation recently.
It is well known that aberrant activating of Wnt/beta-catenin pathway can accelerate liver fibrosis development (Yu et al., 2016a). It has been reported that microRNA (miRNA)-mediated Wnt/β-catenin is involved in the activation of HSCs during liver fibrosis (Li G. et al., 2016; Yu et al., 2016c). Sal B can alleviate liver fibrosis by inhibiting the activation of HSCs and decreasing the expression of type I collagen and α-SMA protein by the lincRNA-p21-mediated Wnt/β-catenin pathway (Yu et al., 2015, 2016b, 2017). Sal B also inhibited CCL4-induced liver fibrosis by the NF-Kb/IκBα signaling pathway (Wang et al., 2012). Besides, Sal B prevented the activation of HSCs by influencing the TGF-β signaling pathway in a rat model of liver fibrosis induced by intraperitoneal injection of dimethylnitrosamine (DMN) (Tao et al., 2013). Numerous in vivo and in vitro experiments have also demonstrated that Sal B attenuated liver fibrosis by affecting other signaling cascades. For example, Sal B attenuated ET-1-induced HSCs contraction by inhibiting the activation of Rho A and ROCK II and the downstream MYPT1 phosphorylation at Thr (696) (Xu et al., 2012), and this process is associated with inhibition of the Rho A signaling pathway. In LX-2 cells, Sal B can inhibit the expression of Col I independent of TGF-β1 stimulation, which is associated with direct inhibition of p38 signaling and inhibition of crosstalk between Smad and ERK signaling (Lv and Xu, 2012). When Sal B exerts its anti-liver fibrosis, it also down-regulated Ang II signaling during HSCs activation (Li et al., 2012).
Although a large number of experiments have proved that the salvianolic acids have a good treatment effect on liver fibrosis, there are still many proved shortcomings. For instance, experiment shows that Sal B may induce liver fibrosis in rats by down-regulating CD14 expression and blocking endotoxin signaling to antagonize CCL4 (Liu et al., 2011). Therefore, it is very necessary to verify the effect of salvianolic acids on liver fibrosis.
Salvianolic Acids and Pulmonary Fibrosis
Pulmonary fibrosis involves a series of heterogeneous lung diseases characterized by abnormally excessive accumulation of ECM leading to scarring and sclerosis of the lung tissue, loss of alveolar structure, ultimately leading to gas exchange and fatal respiratory failure (Strieter and Mehrad, 2009; Noble et al., 2012). Idiopathic pulmonary fibrosis (IPF) is the most common form of pulmonary fibrosis, which poses a serious threat to human health. Its pathogenesis is unknown, and its life expectancy after diagnosis is very short (Thannickal et al., 2014). To date, despite decades of extensive research on IPF, there is still a lack of effective treatment. Therefore, finding safe and effective drugs for the treatment of pulmonary fibrosis is essential (Thannickal, 2013; Wolters et al., 2014). As is shown in Figure 3, we summarized the multiple mechanisms of salvianolic acids for pulmonary fibrosis treatment.
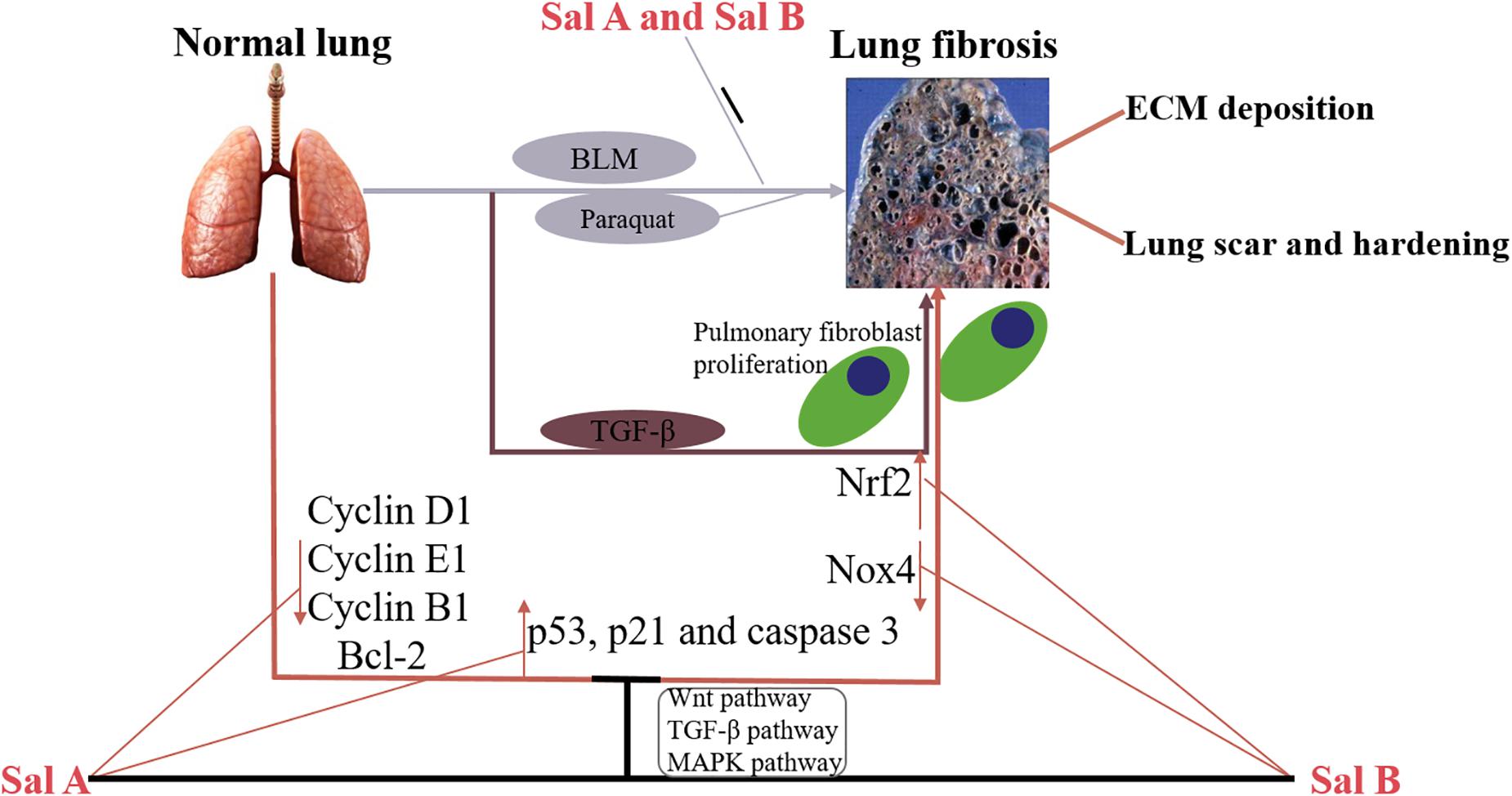
Figure 3. Schematic model of the multiple mechanisms of Salvianolic acids for pulmonary fibrosis treatment.
Pan et al. (2014) found in vivo that Sal A attenuated BLM-induced pulmonary fibrosis in rats, and reduced alveolar wall thickness and collagen deposition. Besides, in vitro experiments have demonstrated that Sal A significantly inhibited fibroblast proliferation, adhesion and migration by affecting the expression of cycle-associated proteins (cyclin D1, cyclin E1, and cyclin B1) and apoptosis-related proteins (Bcl-2 and caspase-3) (Pan et al., 2014; Chen Y. C. et al., 2016). Sal B also effectively inhibited BLM-induced pulmonary fibrosis in mice by reducing the mouse lung interstitial collagen fiber positive region model, reducing lung fibroblast number, and decreasing lung Hyd content and lung type I collagen expression (Liu et al., 2015).
Inhibition of pulmonary fibrosis by salvianolic acids may be associated with the Smad signaling pathway. For example, Sal A ameliorated pulmonary remodeling in monocrotaline (MCT)-induced pulmonary arterial hypertension (PAH) rats by activating the BMPRII-Smad pathway and inhibiting apoptosis (Chen Y. C. et al., 2016). Liu Q. et al. (2016) demonstrated that Sal B attenuated pulmonary fibrosis by inhibiting Smad-dependent signaling and the Smad-independent MAPK pathway. Sal B inhibited the expression of TGF-β1 and phosphorylation of Smad3 in a rat model of pulmonary fibrosis induced by paraquat. In addition, Sal B enhanced nuclear translocation and expression of nuclear factor erythrocyte 2-related factor 2 (Nrf2) and decreased the expression of ROS-producing enzyme Nox4 (Liu B. et al., 2016).
The Effects of Salvianolic Acids on Other Fibrosis Disease
Renal fibrosis is an inevitable process in the development of all chronic kidney diseases, eventually leading to renal failure. Renal fibrosis is characterized by accumulation of fibroblasts and excess matrix proteins (Eddy and Neilson, 2006). Numerous studies have demonstrated that renal fibrosis is mediated by a variety of mediators through a variety of mechanisms and pathways, including growth factors, cytokines, and metabolic toxin stress molecules. Among them, TGF-β1 has been taken into account as an important mediator in the pathogenesis of renal fibrosis (Wang et al., 2005; Bottinger, 2007). Studies have shown that both Sal A and Sal B can be involved in the regulation of TGF-β1-induced renal fibrosis. Ma et al. (2016) demonstrated that Sal A inhibited renal fibrosis by inhibiting the TGF-β1/Smad signaling pathway. Sal B can inhibit the fibrosis process of HK-2 cells by inducing the expression of miR-106b-25 (Tang et al., 2014). Similar studies have shown that Sal B does have a good relief effect on TGF-β1-induced renal fibrosis (Pan et al., 2011; Li et al., 2017). Studies also have shown that Sal A and Sal C inhibited renal fibrosis by attenuating the expression of human chemokine ligand 5 (CCL5) and chemokine ligand 10 (CXCL10) (Li et al., 2015). Besides, Sal B reduced inflammation and oxidative stress by modulating the PI3K/AKT signaling pathway (Ma Z. G. et al., 2017). When the experiment was supplemented with additional Sal B, the expression of PI3K protein and the ratio of p-AKT/AKT was significantly up-regulated. Sal B also has a beneficial effect on renal fibrosis by inhibiting the expression of α-SMA protein and maintaining epithelial phenotype (Zhou et al., 2009; Lu et al., 2010). Recent studies have also found that Sal A attenuated kidney damage in rats by inhibiting the activation of NF-κB and p38 MAPK signaling pathways (Zhang et al., 2018). Therefore, it is significant to further investigate the roles of salvianolic acids in renal fibrosis, which may have therapeutic potential for progressive renal fibrosis.
Cardiac fibrosis is a common pathological condition. Almost all major heart diseases, including hypertension, cardiomyopathy, and myocardial infarction, can lead to myocardial remodeling characterized by excessive deposition of ECM proteins secreted by myofibroblast, further leading to decreased myocardial compliance, cardiac dysfunction and even heart failure (Leask, 2015; Travers et al., 2016). Jiang et al. (2013) found Sal A inhibited fibroblast migration and the secretion of Cytokine such as intercellular adhesion molecule (ICAM), interleukin-6 (IL-6) and soluble vascular cell adhesion molecule-1 (sVCAM-1). These effects are achieved by competitively inhibiting the expression of matrix metalloproteinase-9 (MMP-9). Likewise, Sal B selectively inhibited MMP-9 activities to prevent fibrosis without affecting MMP-9 expression (Jiang et al., 2010). Recently, Wang et al. (2018) found that Sal B attenuated Ang II-induced myocardial fibrosis by inhibiting the NF-κB pathway. In this process, Sal B reduced the nuclear translocation of the NF-κB p65 subunit. It is highly desirable to further develop the potential clinical application of salvianolic acids to protect the heart.
Potential Applications of Salvianolic Acids for the Treatment of Cancer
Cancer is a serious threat to human health. It is extremely urgent to develop drugs that safely and effectively treat cancer and to find the corresponding targets for cancer treatment. It is worth noting that many traditional Chinese medicines have achieved good results in the treatment of cancer. Among them, a large number of studies have shown that salvianolic acids have a good effect in treating various types of cancer (Table 3).
Salvianolic Acids for the Treatment of Breast Cancer
Some studies have predicted that Sal A and Sal B have a good therapeutic effect on breast cancer. MCF-7/PTX cells have strong migration and invasion abilities and are highly resistant to the anticancer drug paclitaxel. Cai et al. (2014) and Zheng et al. (2015) found that Sal A reversed the resistance of ccc cells to paclitaxel. Transgelin 2 inhibited the apoptosis of MCF-7/PTX cells by activating phosphatidylinositol 3-kinase (PI3K)/Akt signaling pathway and mediating the drug resistance of paclitaxel in breast cancer patients. In this process, Sal A suppressed transgelin 2 expression by inhibiting PI3K/Akt pathway activation and adenosine triphosphate binding cassette (ABC) transporter (Cai et al., 2014). At the same time, the metastatic and invasive ability of MCF-7/PTX cells was also significantly inhibited by Sal A (Zheng et al., 2015). Sal A is considered a potential drug to against multidrug resistance (MDR), the main reason for incurable breast cancer. Wang et al. (2015) demonstrated that Sal A induced apoptosis in MCF-7 cells by increasing the activity of caspase-3 and Bax, disrupted the membrane potential of mitochondria, and down-regulated the expression of Bcl-2.
In addition, Sal B also inhibited the proliferation of breast cancer cells and promoted their apoptosis (Sha et al., 2009, 2011). Ding et al. (2016) demonstrated that the FA-PEG-TiO2 nanoparticles loaded with curcumin and Sal B has a good prospect for the treatment of breast cancer by optimizing the drug delivery system.
Salvianolic Acids for the Treatment of Squamous Cell Carcinoma
In recent years, studies have also focused on the effects of salvianolic acids on squamous cell carcinoma (SCC). Zhou et al. (2006) first discovered that Sal B reduced the incidence of SCC by inhibiting angiogenesis and decreasing the expression of hypoxia-inducible factor 1α (HIF-1α) and VEGF. In vivo and in vitro experiments further demonstrated that Sal B inhibited the growth of SCC of the head and neck through cyclooxygenase-2 and the apoptotic pathway (Hao et al., 2009; Zhao et al., 2009, 2010). Overexpression of cyclooxygenase-2 (COX-2) is associated with an increased risk of head and neck cancer. Studies have found that Sal B inhibited the growth of HNSCCs and reduced the formation of solid tumors by reducing the expression of COX-2 (Hao et al., 2009; Zhao et al., 2010). Li H. et al. (2016) found that nano-SalB arrested the cell cycle of head and neck squamous cell carcinoma cells (HNSCCs) and induced apoptosis. In addition, Sal B and low-dose celecoxib (a selective inhibitor of COX-2) work better for the treatment of head and neck SCC (Zhao et al., 2010). Sal B has a certain therapeutic effect on 7, 12-dimethylbenz[a]anthracene (DMBA)-induced oral cancer. Yang et al. (2011) also demonstrated that Sal B inhibited the growth and angiogenesis of oral cancer cells by down-regulating the expression of HIF-1α, TNFα and MMP9 genes and up-regulating the expression of THBS2. Wei et al. (2012, 2018) found that Sal B inhibited the glycolysis of oral SCC by targeting PI3K/AKT/HIF-1α signaling pathway and attenuated DMBA-induced metabolic disorders. Recently, Fang et al. (2018) found that Sal A inhibited the metastasis of oral SCC by inhibiting the c-Raf/MEK/ERK pathway controlling the expression of matrix metallopoteinase-2 (MMP-2).
Salvianolic Acids for the Treatment of Lung Cancer and Liver Cancer
Salvianolic acids also have a good effect in the treatment of lung cancer and liver cancer. Li et al. (2002) found that Sal A inhibited the growth of mouse lung cancer cells by inhibiting the expression of c-myc and P46 (JNK/SAPK). In vivo experiments have also demonstrated that Sal B has a certain inhibitory effect on lung cancer (Tao et al., 2014). Interaction of salvianolic acids with other compounds inhibited the migration and invasion of A549 cells, and inhibited the epithelial-mesenchymal transition (EMT) process of A549 cells through the PTEN/PI3K/AKT pathway (Yang et al., 2017). In addition, Chen F. Y. et al. (2016) also found that Sal A inhibited the expression of the MDR gene MDR1 in lung cancer through miRNA expression and regulation of target genes. Gong et al. (2016) found that Sal B induced apoptosis of human hepatocellular carcinoma cells (HCC) through the mitochondrial apoptosis pathway. Interestingly, Sal B induced autophagy in both hepatoma cells and colorectal cancer cell lines (Gong et al., 2016; Jing et al., 2016). Sal B-induced autophagy may play a pro-apoptotic role, and the AKT/mTOR signaling pathway may be involved in the Sal B induced autophagy process (Gong et al., 2016).
There are also studies on the effects of salvianolic acids on other cancers. For example, Liu (2012) found that Sal B inhibited the growth and promoted apoptosis of retinoblastoma HXO-RB44 cells by up-regulating Caspase-3 expression and inducing cell cycle arrest. Yan (2016) found that Sal B inhibited the growth of ovarian cancer cell line SKOV3 and promoted its apoptosis by up-regulating the expression of Caspase-3, down-regulating the expression of livin, and blocking the cell cycle. In addition, Danshensu, an active component of salvianolic acids, inhibited the development of melanoma by affecting tumor angiogenesis and tumor invasion (Zhang et al., 2010). Guo et al. (2018) demonstrated that Sal B reversed tumor MDR by down-regulating the expression of CD44, SOX2 and ABCG2 proteins in LoVo and HCT-116 colonic CSC xenografts. A recent study by Wu et al. (2018) indicated that S. miltiorrhiza extract inhibited hepatocarcinogenesis by modulating TGF-β/TβR and Imp7/8 protein expression, suggesting that S. miltiorrhiza has multiple targets in HCC treatment.
Inhibition of Cancer-Associated Epithelial-Mesenchymal Transition by Sal B
Epithelial-mesenchymal transition is an important cellular program involved in cancer development. It is characterized by the ability of epithelial cells to transform into mesenchymal cells and gain migration and invasion (Kalluri and Weinberg, 2009; Yilmaz and Christofori, 2009). When cells undergo an EMT process, the expression levels of proteins that are in contact with each other such as E-cadherin and γ-catenin is decreased, and the expression of mesenchymal markers such as vimentin, N-cadherin and fibronectin is increased. The main signaling pathway regulating EMT is TGF-β, Notch and Wnt signaling pathways (Nelson and Nusse, 2004; Katsuno et al., 2013). Studies over the past few years support a significant role of EMT in accelerating cancer metastasis, and new treatments targeting the residual EMT-driven cancer cells in combination with conventional treatments can decrease drug resistance and metastasis formation (Yeung and Yang, 2017). Thus, it can be seen that effective inhibition of EMT plays an important role in the treatment of cancer metastasis.
Recent studies have shown that Sal B inhibited the EMT process (Figure 4). Sal B can further inhibit the EMT process by regulating the levels of microRNAs. Yu et al. (2015) found that Sal B ameliorated liver fibrosis by inhibiting EMT and Hedgehog pathway, in which Sal B causes an increase in miR-152 and further upregulates the negative regulators of Hedgehog pathway (Patched1) and DNA Methyltransferase1 (DNMT1). In general, all three members of the miR-106b-25 cluster (miR-106b, miR-93, and miR-25) were significantly down-regulated during TGF-β1-induced EMT. However, Tang et al. (2014) found that Sal B treatment increased the miR-106b-25 cluster in a dose-dependent manner in HK-2 cells. MiR-106b attenuates EMT by reducing the expression of α-smooth muscle actin (α-SMA) and increasing the expression of E-cadherin. In addition, in vitro experiments also found that Sal B inhibited TGF-β1-induced EMT in HK-2 cells, a process involved in TGF-β/Smad signaling pathway (Yao et al., 2009; Wang Q. L. et al., 2010; Zhou et al., 2010). Another in vivo experiment by Wang Q. L. et al. (2010) demonstrated that Sal B inhibited HgCl2-induced kidney fibrosis in rats by decreasing the expression of α-SMA, TGF-β1, TbetaR-I, p-Smad2/3, and MMP-2 but increasing the expression of E-cadherin. Li et al. (2017) found that the nano-complex HCA-Chi-Ca-Sal B reversed TGF-β1-induced EMT in HK-2 cells. In addition, Yang et al. (2017) found that the complex formed by S. miltiorrhiza and other compounds inhibited EMT through the PTEN/PI3K/AKT signaling pathway.
These studies indicate that salvianolic acids have an inhibitory effect on EMT and can be used to treat cancer and fibrosis diseases. The reversal of EMT may have the consequence of promoting the regeneration of already disseminated cancer cells (Brabletz, 2012). Based on the understanding of cell plasticity, more in vitro and in vivo experiments should be conducted to study the combined effects of salvianolic acids for anti-EMT therapy.
Conclusion and Future Perspective
Naturally derived drugs are an important source of novel medicines. Salvianolic acids containing polyphenol structure is effective antioxidants. Salvianolic acids reduce intracellular and intravascular oxidative stress, which protect cells from peroxidation, and free radical damage. Numerous experimental data indicated that salvianolic acids slowed the progression of fibrosis diseases by reducing excessive deposition of ECM. At the same time, a number of studies have shown that salvianolic acids also have potential anticancer effects by inhibiting the EMT process and cancer-related signal transduction. However, some issues still need to be taken seriously. First, the safety of salvianolic acids on the human body should be evaluated more carefully. Although salvianolic acids are a natural compound extracted from S. miltiorrhiza, their potential toxic derivatives cannot be ignored. A lot of studies with cells and animal models must be established to study the anti-fibrotic and anti-cancer effects of salvianolic acids. Different animal models are needed to comprehensively study the most suitable concentrations and doses of salvianolic acids. Secondly, phenolic hydroxyl groups in salvianolic acids have antioxidant activity and are prone to oxidation, so more attention should be paid to the stability of these compounds. It is necessary to use different animal models to assess the biological activity of salvianolic acids in the body. Finally, although salvianolic acids are water-soluble compounds and have high water solubility, their bioavailability can be further improved. It requires more in vivo and in vitro experiments to increase the bioavailability of salvianolic acids.
Taken together, salvianolic acids are a valuable class of natural compounds with potential for the treatment of fibrosis diseases and cancer. The anti-fibrotic and anti-cancer effects of salvianolic acids are mediated through a variety of molecular mechanisms. Such particular property makes salvianolic acids exceptional choices for future anticancer and anti-fibrotic disease drugs development. It is worth mentioning that the Chinese Food and Drug Administration (SFDA) has approved salvianolic acids for the treatment of chronic angina. Currently, it is widely used in clinical practice due to its good efficacy and safety. Other potential roles and potential mechanisms of salvianolic acids are at present being studied in order to be better applied to the treatment of other diseases.
Author Contributions
All authors contributed for the preparation and read and approved the final manuscript. LM and LT were responsible for confirming the topic. LM were responsible for writing the first draft of this article. LT and QY contributed to furtherly editing and polishing the manuscript.
Funding
This work was funded by the Natural Science Foundation of China (No. 31670952), Graduate Research and Innovation Foundation of Chongqing, China (Grant No. CYB18029), the Fundamental Research Funds for the Central Universities (Nos. 106112017CDJXSYY0001 and 2018CQDYSG0021), Visiting Scholar Foundation of Key Laboratory of Biorheological Science and Technology (Chongqing University), and Ministry of Education (CQKLBST-2018-017).
Conflict of Interest Statement
The authors declare that the research was conducted in the absence of any commercial or financial relationships that could be construed as a potential conflict of interest.
References
Aubry, M. C., Myers, J. L., Douglas, W. W., Tazelaar, H. D., Stephens, T. L. W., Hartman, T. E., et al. (2002). Primary pulmonary carcinoma in patients with idiopathic pulmonary fibrosis. Mayo Clin. Proc. 77, 763–770. doi: 10.4065/77.8.763
Bottinger, E. P. (2007). TGF-beta in renal injury and disease. Semin. Nephrol. 27, 309–320. doi: 10.1016/j.semnephrol.2007.02.009
Brabletz, T. (2012). EMT and MET in metastasis: where are the cancer stem cells? Cancer Cell 22, 699–701. doi: 10.1016/j.ccr.2012.11.009
Cai, J., Chen, S., Zhang, W., Zheng, X., Hu, S., Pang, C., et al. (2014). Salvianolic acid A reverses paclitaxel resistance in human breast cancer MCF-7 cells via targeting the expression of transgelin 2 and attenuating PI3 K/Akt pathway. Phytomedicine 21, 1725–1732. doi: 10.1016/j.phymed.2014.08.007
Chen, F. Y., Bi, L., Qian, L., Gao, J., Jiang, Y. C., and Chen, W. P. (2016). Identification of multidrug resistance gene MDR1 associated microRNA of salvianolic acid A reversal in lung cancer. China J. Chinese Mater. Med. 41, 3279–3284. doi: 10.4268/cjcmm20161726
Chen, T., Liu, W., Chao, X., Zhang, L., Qu, Y., Huo, J., et al. (2011). Salvianolic acid B attenuates brain damage and inflammation after traumatic brain injury in mice. Brain Res. Bull. 84, 163–168. doi: 10.1016/j.brainresbull.2010.11.015
Chen, X., Guo, J., Bao, J., Lu, J., and Wang, Y. (2014). The anticancer properties of salvia miltiorrhiza bunge (danshen): a systematic review. Med. Res. Rev 34, 768–794. doi: 10.1002/med.21304
Chen, Y., Yuan, T., Zhang, H., Yan, Y., Wang, D., Fang, L., et al. (2017). Activation of Nrf2 attenuates pulmonary vascular remodeling via inhibiting endothelial-to-mesenchymal transition: an insight from a plant polyphenol. Int. J. Biol. Sci. 13, 1067–1081. doi: 10.7150/ijbs.20316
Chen, Y. C., Yuan, T. Y., Zhang, H. F., Wang, D. S., Yan, Y., Niu, Z. R., et al. (2016). Salvianolic acid A attenuates vascular remodeling in a pulmonary arterial hypertension rat model. Acta Pharmacol. Sin. 37, 772–782. doi: 10.1038/aps.2016.22
Ding, L., Li, J., Huang, R., Liu, Z., Li, C., Yao, S., et al. (2016). Salvianolic acid B protects against myocardial damage caused by nanocarrier TiO2; and synergistic anti-breast carcinoma effect with curcumin via codelivery system of folic acid-targeted and polyethylene glycol-modified TiO2 nanoparticles. Int. J. Nanomed. 11, 5709–5727. doi: 10.2147/IJN.S107767
Domitrovic, R., Jakovac, H., Marchesi, V. V., and Blazekovic, B. (2013). Resolution of liver fibrosis by isoquinoline alkaloid berberine in CCl(4)-intoxicated mice is mediated by suppression of oxidative stress and upregulation of MMP-2 expression. J. Med. food 16, 518–528. doi: 10.1089/jmf.2012.0175
Du, G., Sun, L., Zhao, R., Du, L., Song, J., Zhang, L., et al. (2016). Polyphenols: potential source of drugs for the treatment of ischaemic heart disease. Pharmacol. Ther. 162, 23–34. doi: 10.1016/j.pharmthera.2016.04.008
Eddy, A. A., and Neilson, E. G. (2006). Chronic kidney disease progression. J. Am. Soc. Nephrol. 17, 2964–2966. doi: 10.1681/ASN.2006070704
El-Serag, H. B. (2011). Hepatocellular carcinoma. N. Engl. J. Med. 365, 1118–1127. doi: 10.1056/NEJMra1001683
Fang, C. Y., Wu, C. Z., Chen, P. N., Chang, Y. C., Chuang, C. Y., Lai, C. T., et al. (2018). Antimetastatic potentials of salvianolic acid A on oral squamous cell carcinoma by targeting MMP-2 and the c-Raf/MEK/ERK pathway. Environ. Toxicol. 33, 545–554. doi: 10.1002/tox.22542
Friedman, S. L. (2003). Liver fibrosis – from bench to bedside. J. Hepatol. 38(Suppl. 1), S38–S53. doi: 10.1016/S0168-8278(02)00429-4
Gong, L., Di, C., Xia, X., Wang, J., Chen, G., Shi, J., et al. (2016). AKT/mTOR signaling pathway is involved in salvianolic acid B-induced autophagy and apoptosis in hepatocellular carcinoma cells. Int. J. Oncol. 49, 2538–2548. doi: 10.3892/ijo.2016.3748
Guo, P., Wang, J., Gao, W., Liu, X., Wu, S., Wan, B., et al. (2018). Salvianolic acid B reverses multidrug resistance in nude mice bearing human colon cancer stem cells. Mol. Med. Rep. 18, 1323–1334. doi: 10.3892/mmr.2018.9086
Hao, Y., Xie, T., Korotcov, A., Zhou, Y., Pang, X., Shan, L., et al. (2009). Salvianolic acid B inhibits growth of head and neck squamous cell carcinoma in vitro and in vivo via cyclooxygenase-2 and apoptotic pathways. Int. J. Cancer 124, 2200–2209. doi: 10.1002/ijc.24160
He, Q., Zhang, J., Chen, F., Guo, L., Zhu, Z., and Shi, J. (2010). An anti-ROS/hepatic fibrosis drug delivery system based on salvianolic acid B loaded mesoporous silica nanoparticles. Biomaterials 31, 7785–7796. doi: 10.1016/j.biomaterials.2010.07.008
Hou, J., Tian, J., Jiang, W., Gao, Y., and Fu, F. (2011). Therapeutic effects of SMND-309, a new metabolite of salvianolic acid B, on experimental liver fibrosis. Eur. J. Pharmacol. 650, 390–395. doi: 10.1016/j.ejphar.2010.10.019
Hu, Y., Wang, R., Zhang, X., Liu, C., Liu, C., Liu, P., et al. (2000). Effects of carbon tetrachloride-injured hepatocytes on hepatic stellate cell activation and salvianolic acid A preventive action in vitro. Chin. J. Hepatol. 8, 299–301.
Hu, Y. Y., Liu, P., Liu, C., Xu, L. M., Liu, C. H., Zhu, D. Y., et al. (1997). Actions of salvianolic acid A on CCl4-poisoned liver injury and fibrosis in rats. Acta Pharmacol. Sin. 18, 478–480.
Jiang, B., Chen, J., Xu, L., Gao, Z., Deng, Y., Wang, Y., et al. (2010). Salvianolic acid B functioned as a competitive inhibitor of matrix metalloproteinase-9 and efficiently prevented cardiac remodeling. BMC Pharmacol. 10:10. doi: 10.1186/1471-2210-10-10
Jiang, B., Li, D., Deng, Y., Teng, F., Chen, J., Xue, S., et al. (2013). Salvianolic acid A, a novel matrix metalloproteinase-9 inhibitor, prevents cardiac remodeling in spontaneously hypertensive rats. PLoS One 8:e59621. doi: 10.1371/journal.pone.0059621
Jing, Z., Fei, W., Zhou, J., Zhang, L., Chen, L., Zhang, X., et al. (2016). Salvianolic acid B, a novel autophagy inducer, exerts antitumor activity as a single agent in colorectal cancer cells. Oncotarget 7, 61509–61519. doi: 10.18632/oncotarget.11385
Kalluri, R., and Weinberg, R. A. (2009). The basics of epithelial-mesenchymal transition. J. Clin. Invest. 119, 1420–1428. doi: 10.1172/JCI39104
Katsuno, Y., Lamouille, S., and Derynck, R. (2013). TGF-beta signaling and epithelial-mesenchymal transition in cancer progression. Curr. Opin. Oncol. 25, 76–84. doi: 10.1097/CCO.0b013e32835b6371
Krenkel, O., and Tacke, F. (2017). Liver macrophages in tissue homeostasis and disease. Nat. Rev. Immunol. 17, 306–321. doi: 10.1038/nri.2017.11
Leask, A. (2015). Getting to the heart of the matter: new insights into cardiac fibrosis. Circ. Res. 116, 1269–1276. doi: 10.1161/CIRCRESAHA.116.305381
Li, G., Li, J., Li, C., Qi, H., Dong, P., Zheng, J., et al. (2016). MicroRNA-125a-5p contributes to hepatic stellate cell activation through targeting FIH1. Cell. Physiol. Biochem. 38, 1544–1552. doi: 10.1159/000443095
Li, H., Shi, L., Wei, J., Zhang, C., Zhou, Z., Wu, L., et al. (2016). Cellular uptake and anticancer activity of salvianolic acid B phospholipid complex loaded nanoparticles in head and neck cancer and precancer cells. Colloids Surf. B Biointerfaces 147, 65–72. doi: 10.1016/j.colsurfb.2016.07.053
Li, H. Y., Li, Y., Yan, C. H., Li, L. N., and Chen, X. G. (2002). Inhibition of tumor growth by S-3-1, a synthetic intermediate of salvianolic acid A. J. Asian Nat. Prod. Res. 4, 271–280. doi: 10.1080/1028602021000049069
Li, J., Gu, T., Fu, X., and Zhao, R. (2015). Effect of salvianolic acid A and C compatibility on inflammatory cytokines in rats with unilateral ureteral obstruction. J. Tradit. Chin. Med. 35, 564–570. doi: 10.1016/S0254-6272(15)30140-0
Li, J., Zhang, C., He, W., Qiao, H., Chen, J., Wang, K., et al. (2017). Coordination-driven assembly of catechol-modified chitosan for the kidney-specific delivery of salvianolic acid B to treat renal fibrosis. Biomaterials Sci. 6, 179–188. doi: 10.1039/c7bm00811b
Li, S., Wang, L., Yan, X., Wang, Q., Tao, Y., Li, J., et al. (2012). Salvianolic acid B Attenuates rat hepatic fibrosis via downregulating angiotensin II signaling, evidence-based complementary and alternative medicine. eCAM 2012:160726. doi: 10.1155/2012/160726
Li, X., Yu, C., Lu, Y., Gu, Y., Lu, J., Xu, W., et al. (2007). Pharmacokinetics, tissue distribution, metabolism, and excretion of depside salts from Salvia miltiorrhiza in rats. Drug Metab. Dispos. 35, 234–239. doi: 10.1124/dmd.106.013045
Li, Y.-J., Duan, C.-L., and Liu, J.-X. (2014). Salvianolic acid A promotes the acceleration of neovascularization in the ischemic rat myocardium and the functions of endothelial progenitor cells. J. Ethnopharmacol. 151, 218–227. doi: 10.1016/j.jep.2013.10.019
Lin, Y. L., Lee, T. F., Huang, Y. J., and Huang, Y. T. (2006a). Antiproliferative effect of salvianolic acid A on rat hepatic stellate cells. J. Pharm. Pharmacol. 58, 933–939.
Lin, Y. L., Wu, C. H., Luo, M. H., Huang, Y. J., Wang, C. N., Shiao, M. S., et al. (2006b). In vitro protective effects of salvianolic acid B on primary hepatocytes and hepatic stellate cells. J. Ethnopharmacol. 105, 215–222.
Liu, B., Cao, B., Zhang, D., Xiao, N., Chen, H., Li, G. Q., et al. (2016). Salvianolic acid B protects against paraquat-induced pulmonary injury by mediating Nrf2/Nox4 redox balance and TGF-beta1/Smad3 signaling. Toxicol. Appl. Pharmacol. 309, 111–120. doi: 10.1016/j.taap.2016.08.004
Liu, C. H., Liu, P., Hu, Y. Y., Xu, L. M., Tan, Y. Z., Wang, Z. N., et al. (2000). Effects of salvianolic acid-A on rat hepatic stellate cell proliferation and collagen production in culture. Acta Pharmacol. Sin. 21, 721–726.
Liu, J. G., Ding, Y. R., and Yang, S. L. (2011). Effect of salvianolic acid B on CD14 expression in rats with liver fibrosis. Zhongguo Zhong Xi Yi Jie He Za Zhi 31, 547–551.
Liu, M., Li, Y. G., Zhang, F., Yang, L., Chou, G. X., Wang, Z. T., et al. (2007). Chromatographic fingerprinting analysis of Danshen root (Salvia miltiorrhiza Radix et Rhizoma) and its preparations using high performance liquid chromatography with diode array detection and electrospray mass spectrometry (HPLC-DAD-ESI/MS). J. Sep. Sci. 30, 2256–2267. doi: 10.1002/jssc.200700149
Liu, M., Zheng, M., Xu, H., Liu, L., Li, Y., Xiao, W., et al. (2015). Anti-pulmonary fibrotic activity of salvianolic acid B was screened by a novel method based on the cyto-biophysical properties. Biochem. Biophys. Res. Commun. 468, 214–220. doi: 10.1016/j.bbrc.2015.10.127
Liu, P., Hu, Y., Liu, C., Liu, C., and Zhu, D. (2001). Effects of salviainolic acid A (SA-A) on liver injury: SA-A action on hepatic peroxidation. Liver 21, 384–390. doi: 10.1034/j.1600-0676.2001.210604.x
Liu, Q., Chu, H., Ma, Y., Wu, T., Qian, F., Ren, X., et al. (2016). Salvianolic acid b attenuates experimental pulmonary fibrosis through inhibition of the TGF-beta signaling pathway. Sci. Rep. 6:27610. doi: 10.1038/srep27610
Liu, X., Yang, Y., Zhang, X., Xu, S., He, S., Huang, W., et al. (2010). Compound astragalus and salvia miltiorrhiza extract inhibits cell invasion by modulating transforming growth factor-beta/Smad in HepG2 cell. J. Gastroenterol. Hepatol. 25, 420–426. doi: 10.1111/j.1440-1746.2009.05981.x
Liu, X. A. (2012). Effects of salvianolic acid B on in vitro growth inhibition and apoptosis induction of retinoblastoma cells. Int. J. Ophthalmol. 5, 272–276. doi: 10.3980/j.issn.2222-3959.2012.03.04
Liu, Y., Hu, Y., E, Q., Zuo, J., Yang, L., and Liu, W. (2017). Salvianolic acid B inhibits mitochondrial dysfunction by up-regulating mortalin. Sci. Rep. 7:43097. doi: 10.1038/srep43097
Lotersztajn, S., Julien, B., Teixeira-Clerc, F., Grenard, P., and Mallat, A. (2005). Hepatic fibrosis: molecular mechanisms and drug targets. Ann. Rev. Pharmacol. Toxicol. 45, 605–628. doi: 10.1146/annurev.pharmtox.45.120403.095906
Lu, H. Y., Zhou, J., Lu, M., Liu, Y. M., Wang, F., Lin, M., et al. (2010). Protection and mechanisms of salvianolic-acid B on experimental renal interstitial fibrosis in rats. J. Chin. Med. Mater. 33, 1755–1759.
Lv, Z., and Xu, L. (2012). Salvianolic acid B inhibits ERK and p38 MAPK signaling in TGF-beta1-stimulated human hepatic stellate cell line (LX-2) via distinct pathways, evidence-based complementary and alternative medicine. eCAM 2012:960128. doi: 10.1155/2012/960128
Ma, X., Xu, W., Zhang, Z., Liu, N., Yang, J., Wang, M., et al. (2017). Salvianolic acid b ameliorates cognitive deficits through IGF-1/Akt pathway in rats with vascular dementia. Cell. Physiol. Biochem. 43, 1381–1391. doi: 10.1159/000481849
Ma, Z., Tang, Y., Zhong, L., Yu, K., and He, L. (2016). Anti-fibrosis and relative mechanism of salvianolic acid A on rat model with renal fibrosis. Int. J. Clin. Exp. Med. 9, 12713–12720.
Ma, Z. G., Xia, H. Q., Cui, S. L., and Yu, J. (2017). Attenuation of renal ischemic reperfusion injury by salvianolic acid B via suppressing oxidative stress and inflammation through PI3K/Akt signaling pathway. Braz. J. Med. Biol. Res. 50:e5954. doi: 10.1590/1414-431X20175954
Mao, K., Shu, W., Qiu, Q., Gu, Q., and Wu, X. (2017). Salvianolic acid A protects retinal pigment epithelium from OX-LDL-induced inflammation in an age-related macular degeneration model. Discov. Med. 23, 129–148.
Nagai, A., Chiyotani, A., Nakadate, T., and Konno, K. (1992). Lung-cancer in patients with idiopathic pulmonary Fibrosis. Tohoku J. Exp. Med. 167, 231–237. doi: 10.1620/tjem.167.231
Nelson, W. J., and Nusse, R. (2004). Convergence of Wnt, beta-catenin, and cadherin pathways. Science 303, 1483–1487. doi: 10.1126/science.1094291
Noble, P. W., Barkauskas, C. E., and Jiang, D. (2012). Pulmonary fibrosis: patterns and perpetrators. J. Clin. Investig. 122, 2756–2762. doi: 10.1172/JCI60323
Pan, R.-H., Xie, F.-Y., Chen, H.-M., Xu, L.-Z., Wu, X.-C., Xu, L.-L., et al. (2011). Salvianolic Acid B reverses the epithelial-to-mesenchymal transition of HK-2 cells that is induced by transforming growth factor-beta. Arch. Pharm. Res. 34, 477–483. doi: 10.1007/s12272-011-0317-7
Pan, Y., Fu, H., Kong, Q., Xiao, Y., Shou, Q., Chen, H., et al. (2014). Prevention of pulmonary fibrosis with salvianolic acid a by inducing fibroblast cell cycle arrest and promoting apoptosis. J. Ethnopharmacol. 155, 1589–1596. doi: 10.1016/j.jep.2014.07.049
Pei, R., Si, T., Lu, Y., Zhou, J. X., and Jiang, L. (2018). Salvianolic acid A, a novel PI3K/Akt inhibitor, induces cell apoptosis and suppresses tumor growth in acute myeloid leukemia. Leuk. Lymphoma 59, 1959–1967. doi: 10.1080/10428194.2017.1399314
Qiang, G., Yang, X., Xuan, Q., Shi, L., Zhang, H., Chen, B., et al. (2014). Salvianolic Acid a prevents the pathological progression of hepatic fibrosis in high-fat diet-fed and streptozotocin-induced diabetic rats. Am. J. Chin. Med. 42, 1183–1198. doi: 10.1142/S0192415X14500748
Sanchez-Valle, V., Chavez-Tapia, N. C., Uribe, M., and Mendez-Sanchez, N. (2012). Role of oxidative stress and molecular changes in liver fibrosis: a review. Curr. Med. Chem. 19, 4850–4860. doi: 10.2174/092986712803341520
Sha, W., Gu, X., Guo, Y., Wang, P., Sukumar, S., and Zhou, Y. (2011). Salvianolic acid B inhibits both ER-alpha plus /- breast cancer cell growth in vivo and in vitro. Cancer Res. 71 (8 suppl.), 2128. doi: 10.1158/1538-7445.AM2011-2128
Sha, W., Zhou, Y., Ji, H., Shan, L., Pang, X., Califano, J., et al. (2009). Salvianolic acid B, a natural compound, showing anti-cancer activity by inhibiting breast cancer cell proliferation and inducing cell apoptosis. Cancer Res. 69.
Strieter, R. M., and Mehrad, B. (2009). New mechanisms of pulmonary fibrosis. Chest 136, 1364–1370. doi: 10.1378/chest.09-0510
Tang, H.-J., Zhang, X.-W., Yang, L., Li, W., Li, J.-H., Wang, J.-X., et al. (2016). Synthesis and evaluation of xanthine oxidase inhibitory and antioxidant activities of 2-arylbenzo b furan derivatives based on salvianolic acid C. Eur. J. Med. Chem. 124, 637–648. doi: 10.1016/j.ejmech.2016.08.019
Tang, Q., Zhong, H., Xie, F., Xie, J., Chen, H., and Yao, G. (2014). Expression of miR-106b-25 induced by salvianolic acid B inhibits epithelial-to-mesenchymal transition in HK-2 cells. Eur. J. Pharmacol. 741, 97–103. doi: 10.1016/j.ejphar.2014.07.051
Tao, L., Wang, S., Zhao, Y., Sheng, X., Wang, A., Zheng, S., et al. (2014). Phenolcarboxylic acids from medicinal herbs exert anticancer effects through disruption of COX-2 activity. Phytomedicine 21, 1473–1482. doi: 10.1016/j.phymed.2014.05.001
Tao, Y. Y., Wang, Q. L., Shen, L., Fu, W. W., and Liu, C. H. (2013). Salvianolic acid B inhibits hepatic stellate cell activation through transforming growth factor beta-1 signal transduction pathway in vivo and in vitro. Exp. Biol. Med. 238, 1284–1296. doi: 10.1177/1535370213498979
Thannickal, V. J. (2013). Mechanistic links between aging and lung fibrosis. Biogerontology 14, 609–615. doi: 10.1007/s10522-013-9451-6
Thannickal, V. J., Zhou, Y., Gaggar, A., and Duncan, S. R. (2014). Fibrosis: ultimate and proximate causes. J. Clin. Invest. 124, 4673–4677. doi: 10.1172/JCI74368
Travers, J. G., Kamal, F. A., Robbins, J., Yutzey, K. E., and Blaxall, B. C. (2016). Cardiac fibrosis: the fibroblast awakens. Circ. Res. 118, 1021–1040. doi: 10.1161/CIRCRESAHA.115.306565
Tsai, M. K., Lin, Y. L., and Huang, Y. T. (2010). Effects of salvianolic acids on oxidative stress and hepatic fibrosis in rats. Toxicol. Appl. Pharmacol. 242, 155–164. doi: 10.1016/j.taap.2009.10.002
Tsai, M. K., Lin, Y. L., and Huang, Y. T. (2011). Differential inhibitory effects of salvianolic acids on activation of rat hepatic stellate cells by platelet-derived growth factor. Planta Med. 77, 1495–1503. doi: 10.1055/s-0030-1270783
Wang, C., Luo, H., Xu, Y., Tao, L., Chang, C., and Shen, X. (2018). Salvianolic acid B-alleviated angiotensin II induces cardiac fibrosis by suppressing NF-kappa B pathway In Vitro. Med. Sci. Monitor. 24, 7654–7664. doi: 10.12659/MSM.908936
Wang, Q. L., Tao, Y. Y., Yuan, J. L., Shen, L., and Liu, C. H. (2010). Salvianolic acid B prevents epithelial-to-mesenchymal transition through the TGF-beta1 signal transduction pathway in vivo and in vitro. BMC Cell Biol. 11:31. doi: 10.1186/1471-2121-11-31
Wang, R., Yu, X. Y., Guo, Z. Y., Wang, Y. J., Wu, Y., and Yuan, Y. F. (2012). Inhibitory effects of salvianolic acid B on CCl(4)-induced hepatic fibrosis through regulating NF-kappaB/IkappaBalpha signaling. J. Ethnopharmacol. 144, 592–598. doi: 10.1016/j.jep.2012.09.048
Wang, S. X., Hu, L. M., Gao, X. M., Guo, H., and Fan, G. W. (2010). Anti-inflammatory activity of salvianolic acid B in microglia contributes to its neuroprotective effect. Neurochem. Res. 35, 1029–1037. doi: 10.1007/s11064-010-0151-1
Wang, W., Koka, V., and Lan, H. Y. (2005). Transforming growth factor-beta and smad signalling in kidney diseases. Nephrology 10, 48–56. doi: 10.1111/j.1440-1797.2005.00334.x
Wang, X., Morris-Natschke, S. L., and Lee, K. H. (2007). New developments in the chemistry and biology of the bioactive constituents of Tanshen. Med. Res. Rev. 27, 133–148. doi: 10.1002/med.20077
Wang, X., Wang, C., Zhang, L., Li, Y., Wang, S., Wang, J., et al. (2015). Salvianolic acid A shows selective cytotoxicity against multidrug-resistant MCF-7 breast cancer cells. Anticancer Drugs 26, 210–223. doi: 10.1097/CAD.0000000000000184
Wei, J., Wu, J., Xu, W., Nie, H., Zhou, R., Wang, R., et al. (2018). Salvanic acid B inhibits glycolysis in oral squamous cell carcinoma via targeting PI3K/AKT/HIF-1 alpha signaling pathway. Cell Death Dis. 9:599. doi: 10.1038/s41419-018-0623-9
Wei, J., Xie, G., Ge, S., Qiu, Y., Liu, W., Lu, A., et al. (2012). Metabolic Transformation of DMBA-induced carcinogenesis and inhibitory effect of salvianolic acid B and breviscapine treatment. J. Proteome Res. 11, 1302–1316. doi: 10.1021/pr2009725
Wolters, P. J., Collard, H. R., and Jones, K. D. (2014). Pathogenesis of idiopathic pulmonary fibrosis. Ann. Rev. Pathol. 9, 157–179. doi: 10.1146/annurev-pathol-012513-104706
Wu, C., Kan, H., Hu, M., Liu, X., Boye, A., Jiang, Y., et al. (2018). Compound astragalus and Salvia miltiorrhiza extract inhibits hepatocarcinogenesis via modulating TGF-beta/T beta R and Imp7/8. Exp. Ther. Med. 16, 1052–1060.
Wynn, T. A. (2007). Common and unique mechanisms regulate fibrosis in various fibroproliferative diseases. J. Clin. Investig. 117, 524–529. doi: 10.1172/JCI31487
Xu, H., Li, Y.-L., Tian, H.-C., and Zhai, W.-T. (2014). Preparation and chemical structure identification of methylated metabolites of salvianolic acid A. Chin. J. Anal. Chem. 42, 65–70.
Xu, H., Zhou, Y., Lu, C., Ping, J., and Xu, L. M. (2012). Salvianolic acid B lowers portal pressure in cirrhotic rats and attenuates contraction of rat hepatic stellate cells by inhibiting RhoA signaling pathway. Lab. Invest. 92, 1738–1748. doi: 10.1038/labinvest.2012.113
Yan, F. (2016). Effects of salvianolic acid B on growth inhibition and apoptosis induction of ovarian cancer SKOV3. Eur. J. Gynaecol. Oncol. 37, 653–656.
Yang, Y., Ge, P. J., Jiang, L., Li, F. L., and Zhu, Q. Y. (2011). Modulation of growth and angiogenic potential of oral squamous carcinoma cells in vitro using salvianolic acid B. BMC Complement. Altern. Med. 11:54. doi: 10.1186/1472-6882-11-54
Yang, Y., Qiu, S., Qian, L., Tian, Y., Chen, Y., Bi, L., et al. (2017). OCF can repress tumor metastasis by inhibiting epithelial-mesenchymal transition involved in PTEN/PI3K/AKT pathway in lung cancer cells. PLoS One 12:e0174021. doi: 10.1371/journal.pone.0174021
Yao, G., Xu, L., Wu, X., Xu, L., Yang, J., and Chen, H. (2009). Preventive effects of salvianolic acid B on transforming growth factor-beta1-induced epithelial-to-mesenchymal transition of human kidney cells. Biol. Pharm. Bull. 32, 882–886. doi: 10.1248/bpb.32.882
Yeung, K. T., and Yang, J. (2017). Epithelial-mesenchymal transition in tumor metastasis. Mol. Oncol. 11, 28–39. doi: 10.1002/1878-0261.12017
Yilmaz, M., and Christofori, G. (2009). EMT, the cytoskeleton, and cancer cell invasion. Cancer Metastasis Rev. 28, 15–33. doi: 10.1007/s10555-008-9169-0
Yu, F., Fan, X., Chen, B., Dong, P., and Zheng, J. (2016a). Activation of hepatic stellate cells is inhibited by microRNA-378a-3p via Wnt10a. Cell. Physiol. Biochem. 39, 2409–2420.
Yu, F., Lu, Z., Huang, K., Wang, X., Xu, Z., Chen, B., et al. (2016b). MicroRNA-17-5p-activated Wnt/beta-catenin pathway contributes to the progression of liver fibrosis. Oncotarget 7, 81–93. doi: 10.18632/oncotarget.6447
Yu, F., Yang, J., Huang, K., Pan, X., Chen, B., Dong, P., et al. (2016c). The epigenetically-regulated microRNA-378a Targets TGF-beta2 in TGF-beta1-treated hepatic stellate cells. Cell. Physiol. Biochem. 40, 183–194.
Yu, F., Guo, Y., Chen, B., Shi, L., Dong, P., Zhou, M., et al. (2017). LincRNA-p21 inhibits the Wnt/beta-catenin pathway in activated hepatic stellate cells via sponging microRNA-17-5p. Cell. Physiol. Biochem. 41, 1970–1980. doi: 10.1159/000472410
Yu, F., Lu, Z., Chen, B., Wu, X., Dong, P., and Zheng, J. (2015). Salvianolic acid B-induced microRNA-152 inhibits liver fibrosis by attenuating DNMT1-mediated Patched1 methylation. J. Cell. Mol. Med. 19, 2617–2632. doi: 10.1111/jcmm.12655
Zhang, D. Y., and Friedman, S. L. (2012). Fibrosis-dependent mechanisms of hepatocarcinogenesis. Hepatology 56, 769–775. doi: 10.1002/hep.25670
Zhang, H., Liu, Y.-Y., Jiang, Q., Li, K.-R., Zhao, Y.-X., Cao, C., et al. (2014). Salvianolic acid A protects RPE cells against oxidative stress through activation of Nrf2/HO-1 signaling. Free Radic. Biol. Med. 69, 219–228. doi: 10.1016/j.freeradbiomed.2014.01.025
Zhang, H.-F., Wang, Y.-L., Gao, C., Gu, Y.-T., Huang, J., Wang, J.-H., et al. (2018). Salvianolic acid A attenuates kidney injury and inflammation by inhibiting NF-kappaB and p38 MAPK signaling pathways in 5/6 nephrectomized rats. Acta Pharmacol. Sin. 39, 1855–1864. doi: 10.1038/s41401-018-0026-6
Zhang, L. J., Chen, L., Lu, Y., Wu, J. M., Xu, B., Sun, Z. G., et al. (2010). Danshensu has anti-tumor activity in B16F10 melanoma by inhibiting angiogenesis and tumor cell invasion. Eur. J. Pharmacol. 643, 195–201. doi: 10.1016/j.ejphar.2010.06.045
Zhao, G.-R., Zhang, H.-M., Ye, T.-X., Xiang, Z.-J., Yuan, Y.-J., Guo, Z.-X., et al. (2008). Characterization of the radical scavenging and antioxidant activities of danshensu and salvianolic acid B. Food Chem. Toxicol. 46, 73–81. doi: 10.1016/j.fct.2007.06.034
Zhao, Y., Gu, X., Hao, Y., and Guo, Y. (2009). Combinatorial effect of Salvianolic acid B and celecoxib on inhibition of head and neck squamous cell carcinoma. Cancer Res. 3, 787–796. doi: 10.1158/1940-6207.CAPR-09-0243
Zhao, Y., Hao, Y., Ji, H., Fang, Y., Guo, Y., Sha, W., et al. (2010). Combination effects of salvianolic acid B with low-dose celecoxib on inhibition of head and neck squamous cell carcinoma growth in vitro and in vivo. Cancer Prev. Res. 3, 787–796. doi: 10.1158/1940-6207.CAPR-09-0243
Zheng, X., Chen, S., Yang, Q., Cai, J., Zhang, W., You, H., et al. (2015). Salvianolic acid A reverses the paclitaxel resistance and inhibits the migration and invasion abilities of human breast cancer cells by inactivating transgelin 2. Cancer Biol. Ther. 16, 1407–1414. doi: 10.1080/15384047.2015.1070990
Zhou, J., Wang, F., Lu, H., and Zhang, Y. (2010). Impact of salvianolic acid-B on TGF-beta1-induced HK-2 epithelial-mesenchymal transition. China J. Chin. Mater. Med. 35, 89–93.
Zhou, J., Zhang, Y., Lu, H., Liu, Y., and Lin, M. (2009). Effect of salvianolic acid B on generation and activation of myofibroblast in rat with renal interstitial fibrosis. China J. Chin. Mater. Med. 34, 2790–2793.
Zhou, L., Zuo, Z., and Chow, M. S. (2005). Danshen: an overview of its chemistry, pharmacology, pharmacokinetics, and clinical use. J. Clin. Pharmacol. 45, 1345–1359. doi: 10.1177/0091270005282630
Zhou, Z. T., Yang, Y., and Ge, J. P. (2006). The preventive effect of salvianolic acid B on malignant transformation of DMBA-induced oral premalignant lesion in hamsters. Carcinogenesis 27, 826–832. doi: 10.1093/carcin/bgi271
Keywords: Salvia miltiorrhiza, compounds, fibroblasts, traditional medicine, epithelial-mesenchymal transition
Citation: Ma L, Tang L and Yi Q (2019) Salvianolic Acids: Potential Source of Natural Drugs for the Treatment of Fibrosis Disease and Cancer. Front. Pharmacol. 10:97. doi: 10.3389/fphar.2019.00097
Received: 08 October 2018; Accepted: 24 January 2019;
Published: 20 February 2019.
Edited by:
Hervé Emonard, Centre National de la Recherche Scientifique (CNRS), FranceReviewed by:
Mohamed M. Abdel-Daim, Suez Canal University, EgyptPaula Alexandra Oliveira, University of Trás-os-Montes and Alto Douro, Portugal
Copyright © 2019 Ma, Tang and Yi. This is an open-access article distributed under the terms of the Creative Commons Attribution License (CC BY). The use, distribution or reproduction in other forums is permitted, provided the original author(s) and the copyright owner(s) are credited and that the original publication in this journal is cited, in accordance with accepted academic practice. No use, distribution or reproduction is permitted which does not comply with these terms.
*Correspondence: Liling Tang, dGFuZ2xpbGluZ0BjcXUuZWR1LmNu Qian Yi, eWlxaWFuMjAxMEB5ZWFoLm5ldA==