- 1Department of Anatomy, College of Medicine and Health Sciences, United Arab Emirates University, Al Ain, United Arab Emirates
- 2Department of Pharmacology and Therapeutics, College of Medicine and Health Sciences, United Arab Emirates University, Al Ain, United Arab Emirates
α-Synuclein (α-syn) is a presynaptic protein that regulates the release of neurotransmitters from synaptic vesicles in the brain. α-Syn aggregates, including Lewy bodies, are features of both sporadic and familial forms of Parkinson's disease (PD). These aggregates undergo several key stages of fibrillation, oligomerization, and aggregation. Therapeutic benefits of drugs decline with disease progression and offer only symptomatic treatment. Novel therapeutic strategies are required which can either prevent or delay the progression of the disease. The link between α-syn and the etiopathogenesis and progression of PD are well-established in the literature. Studies indicate that α-syn is an important therapeutic target and inhibition of α-syn aggregation, oligomerization, and fibrillation are an important disease modification strategy. However, recent studies have shown that plant extracts and phytochemicals have neuroprotective effects on α-syn oligomerization and fibrillation by targeting different key stages of its formation. Although many reviews on the antioxidant-mediated, neuroprotective effect of plant extracts and phytochemicals on PD symptoms have been well-highlighted, the antioxidant mechanisms show limited success for translation to clinical studies. The identification of specific plant extracts and phytochemicals that target α-syn aggregation will provide selective molecules to develop new drugs for PD. The present review provides an overview of plant extracts and phytochemicals that target α-syn in PD and summarizes the observed effects and the underlying mechanisms. Furthermore, we provide a synopsis of current experimental models and techniques used to evaluate plant extracts and phytochemicals. Plant extracts and phytochemicals were found to inhibit the aggregation or fibril formation of oligomers. These also appear to direct α-syn oligomer formation into its unstructured form or promote non-toxic pathways and suggested to be valuable drug candidates for PD and related synucleinopathy. Current evidences from in vitro studies require confirmation in the in vivo studies. Further studies are needed to ascertain their potential effects and safety in preclinical studies for pharmaceutical/nutritional development of these phytochemicals or dietary inclusion of the plant extracts in PD treatment.
Introduction
Parkinson's disease (PD) is a progressive, debilitating neurodegenerative disease that often begins with the gradual loss of dopaminergic neurons in the substantia nigra pars compacta (SNc) (Herrera et al., 2017). It is a common age-related movement disorder that often appears sporadically (Collier et al., 2017). The pathogenesis of PD remains poorly understood, but emerging evidence implicates various genetic and environmental factors in the initiation and progression of PD (Cannon and Greenamyre, 2013). The multifactorial etiopathogensis of PD includes mitochondrial dysfunction, excitotoxicity, endoplasmic reticulum stress, oxidative/nitrosative stress, and inflammation, along with ubiquitin-proteasome system dysfunction (Moore et al., 2005; Lashuel et al., 2013; Ur Rasheed et al., 2016; Gelders et al., 2018). Altogether, these events lead to the accumulation of abnormal or misfolded α-synuclein (α-syn) protein (Moore et al., 2005; Lashuel et al., 2013; Ur Rasheed et al., 2016; Gelders et al., 2018). Numerous genetic, biochemical, cellular, pathological, and molecular studies indicate PD pathogenesis is associated with environments where α-syn is susceptible to polymerization, aggregation and fibril formation, and propagation (Moore et al., 2005; Hansen and Li, 2012; Lashuel et al., 2013; Gelders et al., 2018; Ghiglieri et al., 2018). The α-syn oligomers cause mitochondrial dysfunction and induce endoplasmic stress, oxidative stress, neuroinflammation, and inhibit proteasomal activity and autophagy (Ghiglieri et al., 2018).
Current PD treatment options, such as dopamine agonists, cholinesterase, and monoamine oxidase inhibitors provide only symptomatic relief (Ellis and Fell, 2017). Dopamine-based drugs have reduced effectiveness in relieving symptoms with disease progression (Ceravolo et al., 2016). The oligomerization and fibrillation of α-syn is linked with the onset and progression of PD (Hansen and Li, 2012), and is believed to be a unique and convincing disease-modification therapeutic strategy for PD, dementia with Lewy body (DLB), and related α-synucleinopathy (Kalia et al., 2015; Török et al., 2016; Brundin et al., 2017). Several molecules including antibodies (Bergström et al., 2016), polyamines (Büttner et al., 2014), heat shock proteins (Cox et al., 2016), chaperones (Friesen et al., 2017), and pharmaceuticals (Lauterbach et al., 2010) have been shown to affect different forms of α-syn (i.e., monomers, soluble oligomers, protofibrils, or fibrils) and oligomerization, fibrillation, and clearance. Therefore, targeting α-syn aggregation, oligomerization, fibrillation, and propagation to reduce α-syn toxicity emerged as an important therapeutic target for slowing or halting disease progression (Kalia et al., 2015; Török et al., 2016; Brundin et al., 2017).
Several recent reviews highlighted the neuroprotective potential of plant extracts and phytochemicals in PD through antioxidant and anti-inflammatory activities (Sarrafchi et al., 2016; da Costa et al., 2017; Mazo et al., 2017; Morgan and Grundmann, 2017; Wang et al., 2017; Zhang et al., 2017; Amro and Srijit, 2018). However, despite the enormous success of antioxidants (whether of synthetic or natural origin) in preclinical studies, coenzyme Q10 (Beal et al., 2014), creatine (Attia et al., 2017), and vitamin E (Ahlskog, 1994) either failed or showed marginal neuroprotection in patients. Recently, α-syn antibodies (PRX002) showed safety in phase 1 studies and were indicated for further phases of clinical studies (Schenk et al., 2017; Jankovic et al., 2018). Similarly, natural products (mainly plant extracts and phytochemicals) emerged to specifically target α-syn (Masuda et al., 2006; Meng et al., 2009, 2010; Caruana et al., 2011; Marchiani et al., 2013). Yet, no comprehensive review is available on these plant extracts and phytochemicals, or on how they target the different steps leading to α-syn oligomerization or fibrillation.
This review, therefore, focuses on the neuroprotective properties and mechanism of action of plant extracts, extract-based formulations, and plant-derived phytochemicals that target α-syn oligomerization, fibrillation, aggregation, and toxicity in various experimental PD models. Furthermore, we also elaborate on the suitability of biochemical, biophysical, and neurochemical techniques to evaluate plant extracts and phytochemicals that ameliorate α-syn neurotoxicity. The source of phytochemicals, the models used, and the effect/mechanisms observed are presented in Tables 1–7. The chemical structures of these phytochemicals are presented in Figure 1. A scheme on the action of the plant extracts and phytochemicals targeting α-syn is presented in Figure 2.
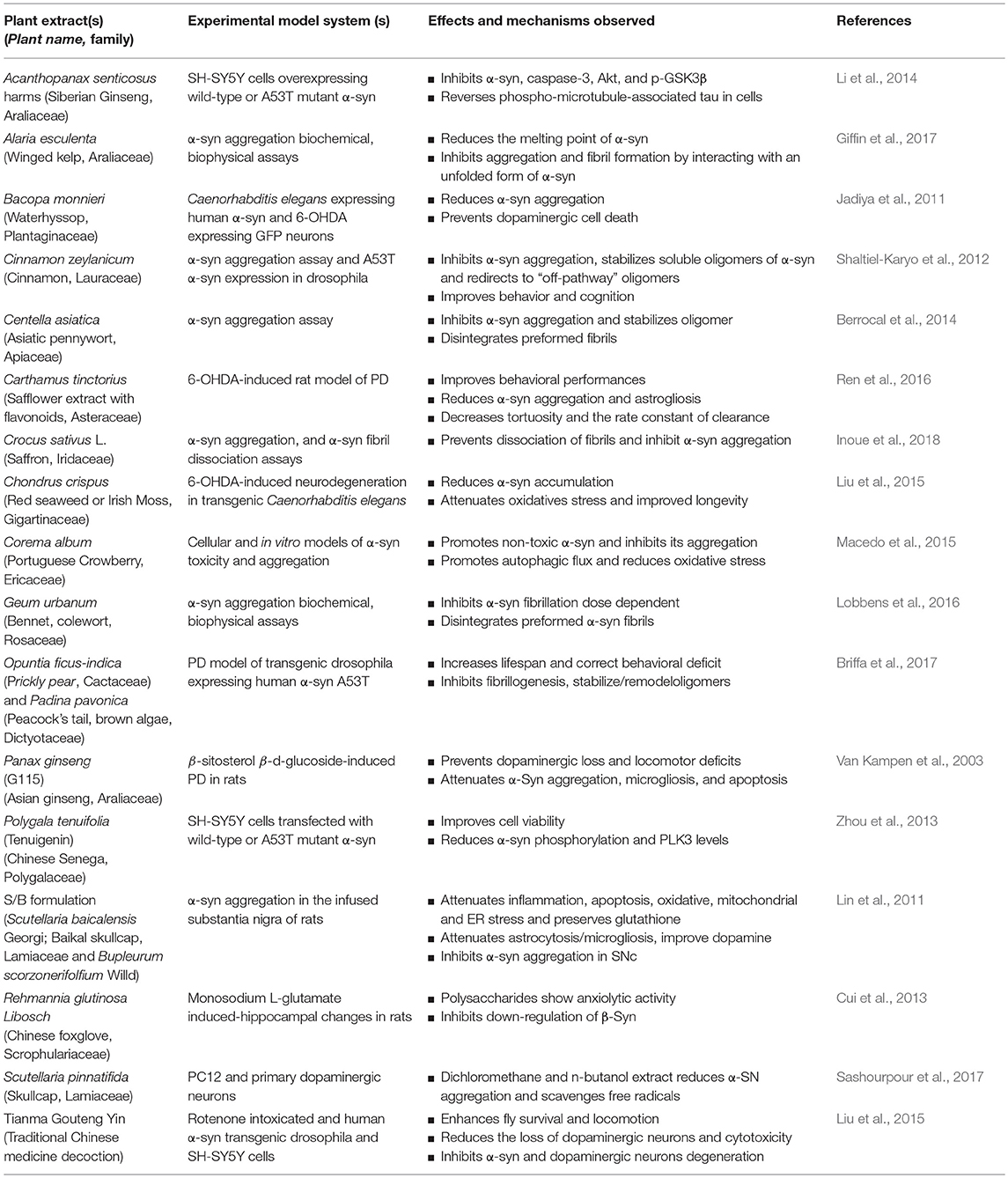
Table 1. The plant extracts and formulations providing neuroprotection in Parkinson's disease models by targeting α-synuclein.
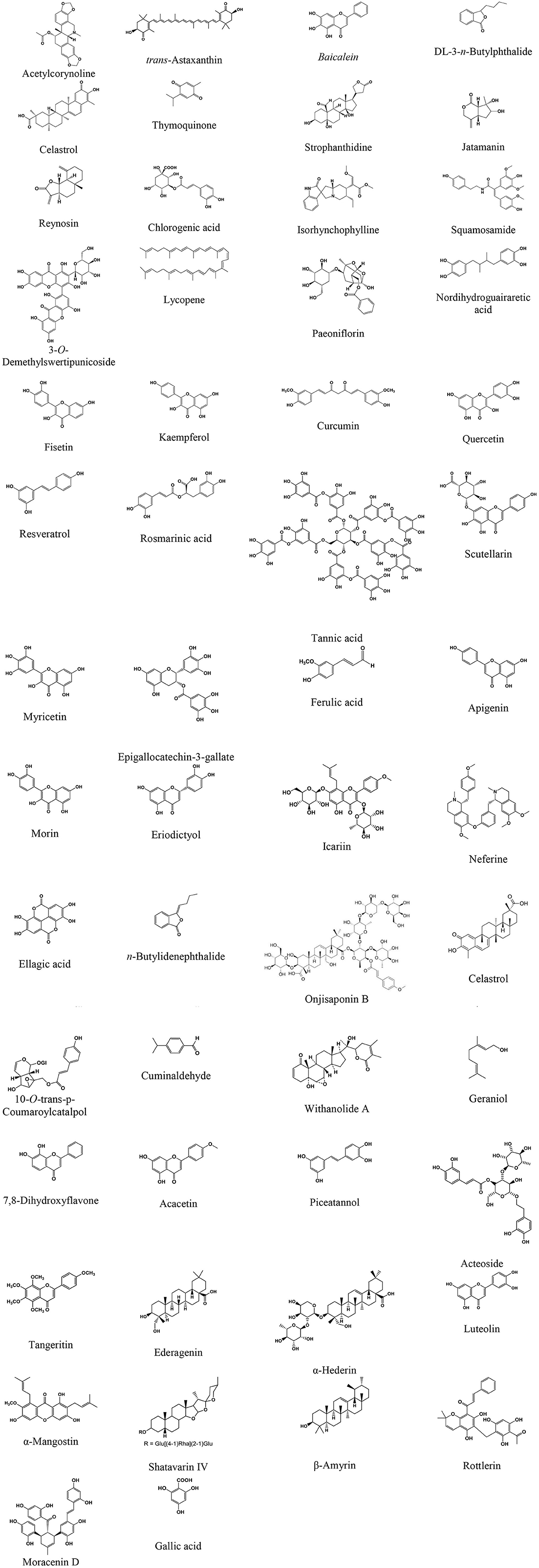
Figure 1. Chemical structure of the phytochemicals attenuating α-synuclein activity in animal models of Parkinson's disease.
α-synuclein as a Therapeutic Target for PD
α-syn, a 140-residue presynaptic protein in the brain, plays a key role in the trafficking and fusion of synaptic vesicles and it regulates dopamine release at presynaptic terminals (Burre et al., 2010; Bendor et al., 2013). The physiological concentration of α-syn is 1 μM in the normal human brain and 70 pM in cerebrospinal fluid (Borghi et al., 2000). It exists natively as an unfolded monomer and attains an α-helical secondary structure after binding lipid vesicles. Upon destabilization, this leads to the misfolding and aggregation of α-syn in neurons (Ruipérez et al., 2010; Bartels et al., 2011; Broersen et al., 2018). Monomeric α-syn is an intrinsically disordered protein found in different conformational states. It plays a significant role in many key biochemical processes (Tompa, 2005), as well as in a rising number of diseases involving misfolding, notably PD (Uversky and Dunker, 2010). In dopaminergic neurons, the intracytoplasmic inclusions of α-syn (Spillantini et al., 1998), synphilin-1 (Wakabayashi et al., 2000) and ubiquitin (Kuzuhara et al., 1988) form Lewy bodies, a pathological characteristic of PD. The cascade of α-syn aggregation begins with dimer formation, then tiny oligomers/protofibrils that lead to the development of β-sheet-rich α-syn fibrils. These eventually lead to end-stage fibrils and aggregated α-syn that are the major component of Lewy bodies (Ghiglieri et al., 2018). Thus, in the multistep process of α-syn-mediated neuronal toxicity, oligomerization of α-syn monomers is the primary phase that facilitates the development of intracytoplasmic inclusions and fibrils (Spillantini et al., 1997).
Numerous theories have been proposed on the role of α-syn in initiating dopaminergic neurodegeneration in PD (Herrera et al., 2017; Ghiglieri et al., 2018). These include the interaction of α-syn aggregates with biomolecules, impaired fusion, and trafficking of vesicles, excessive free radical generation, mitochondrial dysfunction, endoplasmic reticulum stress, and synaptic dysfunction (Herrera et al., 2017; Longhena et al., 2017; Ghiglieri et al., 2018). The α-syn protein consists of three distinct domains, where the central region is critical for α-syn fibril aggregation, a key component of Lewy bodies. α-syn can adopt a wide range of conformational structures ranging from compact to fully extended (Winner et al., 2011). The interactions between the N- and C-termini of α-syn play a role in its stabilization into a compact, monomeric conformation that is non-toxic (Bertoncini et al., 2005). The agents that bind to α-syn and form a loop structure between the N- and C-terminus are believed to confer neuroprotection. In contrast, the agents which induce more compressed structures are considered neurotoxic in nature (Karpinar et al., 2009; Lashuel et al., 2013). Mutations in α-syn can contribute to multiple forms of PD including genetic and rare forms of PD with early onset (Singleton et al., 2003; Simon-Sanchez et al., 2009). Monomeric α-syn is a potential therapeutic target as it is an upstream form of the protein during the aggregation process and the etiopathogenesis of PD (Winner et al., 2011; Lashuel et al., 2013; Brundin et al., 2017; Ghiglieri et al., 2018). The agents stabilizing, promoting clearance, degrading misfolded proteins, solubilizing oligomers, or inhibiting the propagation of α-syn aggregates are pharmacologically appropriate and a clinically relevant therapeutic strategy for PD.
Medicinal Plants Targeting α-synuclein Cascade and Toxicity
Recently, many plant extracts appear to inhibit oligomerization and fibrillization of α-syn, an emerging therapeutic target in PD (Lobbens et al., 2016; Ren et al., 2016; Briffa et al., 2017; Cheon et al., 2017). The plant extracts, which were shown to be neuroprotective in PD, target various pathogenic stages of α-syn conformations ranging from fibrillation to oligomerization in experimental models and are listed in Table 1. Plants, such as Acanthopanax senticosus [Eleutherococcus senticosus (Rupr. & Maxim.) Maxim.], Bacopa monnieri [Bacopa monnieri (L.) Wettst.], Cinnamon extract precipitate [Cinnamomum verum J. Presl], Centella asiatica [Centella asiatica (L.) Urb.], Panax ginseng [Panax ginseng C.A. Mey.], Polygala tenuifolia [Polygala tenuifolia Willd.], Rehmannia glutinosa [Rehmannia glutinosa (Gaertn.) DC.], Corema album [Trema micranthum (L.) Blume], Opuntia ficus-indica [Opuntia ficus-indica (L.) Mill.], Padina pavonica [Sagina japonica (Sw. ex Steud.) Ohwi], Carthamus tinctorius L., and Crocus sativus L. are neuroprotective in PD by targeting oligomerization, fibrillation, and disaggregation of preformed α-syn fibrils. A scheme is presented in Figure 2 to depict the potential mechanism of action of the plant extracts and phytochemicals on α-syn oligomerization, fibrillation, and aggregation.
Many plant extracts show (often in vitro) effects in experimental models of PD by targeting α-syn. However, the bioactive constituents attributing to this effect are not available. Bacopa monnieri prevents neurodegeneration in A53T α-syn-induced PD in Caenorhabditis elegans (Jadiya et al., 2011). However, the chemical constituents collectively known as bacosides have not been investigated in experimental PD models or their effect on α-syn. Centella asiatica (L.) Urb., known as Asiatic pennywort, reportedly prevents α-syn aggregation in vitro (Berrocal et al., 2014). Yet, the principal constituent asiatic acid failed to prevent α-syn aggregation. Meanwhile, asiaticoside and madecassic acid have not been investigated for their effects on α-syn. Cinnamon extract precipitate reportedly inhibits α-syn aggregation and stabilizes oligomers in vitro and in vivo in A53T α-syn-induced PD in flies (Shaltiel-Karyo et al., 2012). However, cinnamaldehyde, a major ingredient of cinnamon extract has not yet been investigated. Eucalyptus citriodora improves climbing ability and attenuates oxidative stress in transgenic drosophila expressing human α-syn (Siddique et al., 2013a). The effects of the bioactive contents citronellol, linalool, and isopulegol of Eucalyptus citriodora on α-syn are not known. Crocus sativus L., popularly known as saffron, is widely used for its color, flavor, and aroma in food and beverages. Saffron and its constituents, such as crocin-1, crocin-2, crocetin, safranal, and the crocetin structural analogs hexadecanedioic acid, norbixin, and trans-muconic acid, were found to affect α-syn fibrillation and aggregation (Inoue et al., 2018). However, some crocetin analogs failed to affect α-syn aggregation and dissociation. Sorbus alnifolia, also known as Korean mountain ash, improved viability of rat pheochromocytoma (PC12) cells while also improving the longevity, food sensing, and reducing dopaminergic neurodegeneration in Caenorhabditis elegans model of PD (Cheon et al., 2017). However, the extract failed to alter α-syn aggregation in the NL5901 strain (Cheon et al., 2017).
From the perspective of traditional medicine, targeting α-syn with plant extracts containing phytochemicals could be considered beneficial using dietary intervention. This could be due to the synergy in action and superior therapeutic effects, along with polypharmacological properties (Wagner and Ulrich-Merzenich, 2009; Wu et al., 2013). The fraction that termed active from Radix Polygalae was found more potent than the constituent, where onjisaponin B increased mutant huntingtin removal and reduced α-syn aggregation. This plant could be a good source of phytochemicals and a template for novel small molecule inhibitors of α-syn (Wu et al., 2013). Plant-based formulations, such as S/B which contain extracts of Scutellaria baicalensis Georgi and Bupleurum scorzonerifolfium and a traditional Chinese medicine decoction known as Tianma Gouteng Yin, were also found to diminish α-syn accumulation and aggregation in experimental PD models (Lin et al., 2011).
The majority of plant extracts used in traditional medicines are based on long-established knowledge of their health benefits, time tested safety due to ancient use, and potential therapeutic effects. However, some plants are not as beneficial as documented or are detrimental; the essential oil from Myrtus communis, which is popular in the Zoroastrian community for aroma (Morshedi and Nasouti, 2016), increases α-syn fibrillation and enhances α-syn toxicity in human neuroblastoma cells (Morshedi and Nasouti, 2016). This study suggests that essential oils used in aromatherapy should be investigated for their potential neurotoxicity or neurodegenerative ability.
The attenuation of α-syn toxicity by plant extracts validates traditional claims of medicinal plants. It may also provide the basis for dietary or nutritional inclusion of these plants in foods to achieve neuroprotective effects. This is not only based on antioxidant approaches but also inhibition of α-syn aggregation. However, in-depth studies are needed for a dietary or therapeutic recommendation on the use of plant extracts in humans.
Plant Extracts and Phytochemicals as Pharmacological Chaperones for PD
Pharmacological chaperoning is emerging as a potential therapeutic approach for the treatment of numerous diseases associated with single gene mutations (Srinivasan et al., 2014). These chaperones are small molecules that bind proteins and stabilize them against proteolytic degradation or protect them from thermal denaturation. Furthermore, they assist in or prevent certain protein-protein assemblies similar to the molecular chaperones (Ringe and Petsko, 2009). Chaperoning is beneficial in cystic fibrosis (Chanoux and Rubenstein, 2012), Gaucher's disease (Sawkar et al., 2002), nephrogenic diabetes insipidus (Tamarappoo and Verkman, 1998), and retinitis pigmentosa (Noorwez et al., 2003). Mechanistically, ligand-mediated chaperoning is believed to correct receptor mislocalization and inhibit mutant proteins from forming toxic intracellular aggregates (Loo and Clarke, 2007). This has been shown to be successful with the pharmacological chaperone, tafamadis, in a clinical trial for the treatment of transthyretin familial amyloid polyneuropathy (Coelho et al., 2013). Several of the molecular chaperones, such as Hsp70, Hsp40, and torsin A either prevent the misfolding of proteins or promote the degradation and elimination of misfolded proteins; they provide a novel therapeutic approach in PD (Dimant et al., 2012).
Although molecular chaperoning is therapeutically significant in α-syn-associated neurodegeneration, the structural heterogeneity and deficiency of persistent structural components for α-syn creates a major issue in the discovery, design, and development of small molecules targeting α-syn (Lester et al., 2009). Plant-derived phytochaperones are a good source of molecules that target protein misfolding in neurotherapeutics (Bernd, 2008). In a chaperone-based approach, Ginkgo biloba is being utilized to search for lead molecules in drug discovery and in the development of protein-misfolding diseases leading to neurodegeneration (Kastenholz and Garfin, 2009). Thus, plant extracts and phytochemicals are a novel source of pharmacological chaperones for a disease-modifying approach that could be promising against neurodegenerative diseases. Following the reductionist approach of drug discovery from plant extracts, it is also important to characterize the bioactive constituents contributing to these pharmacological effects.
Phytochemicals Targeting α-synuclein Assembly and Toxicity
The phytochemicals are non-nutritive secondary metabolites that are heavily utilized for drug discovery and development; they remain an important source of drugs (Beutler, 2009; Henrich and Beutler, 2013). The phytochemicals that target α-syn at different stages of pathogenicity are represented in Table 2 (in vitro studies), Table 3 (in vivo studies), and Table 4 (in vitro and in vivo, both studies), respectively. A benefit of the phytochemicals is their huge structural diversity that offers lead structures for drug discovery and development. They belong to many classes, such as alkaloids, saponins, carotenoids, lignans, glycosides, etc. Briefly, the alkaloids are a nitrogen-containing, structurally-diverse group of secondary metabolites that are protective against neurodegenerative diseases (Hussain et al., 2018). To name a few, galantamine is used in the pharmacotherapy of mild to moderate Alzheimer's disease. Many of the alkaloids, such as acetylcorynoline, 3α-acetoxyeudesma-1,4 (15),11 (13)-trien-12, 6α-olide, corynoxine B, dl-3-n-butylphthalide, isorhynchophylline, and squamosamide attenuate neurotoxicity in experimental models by directly inhibiting α-syn aggregation or fibril formation.
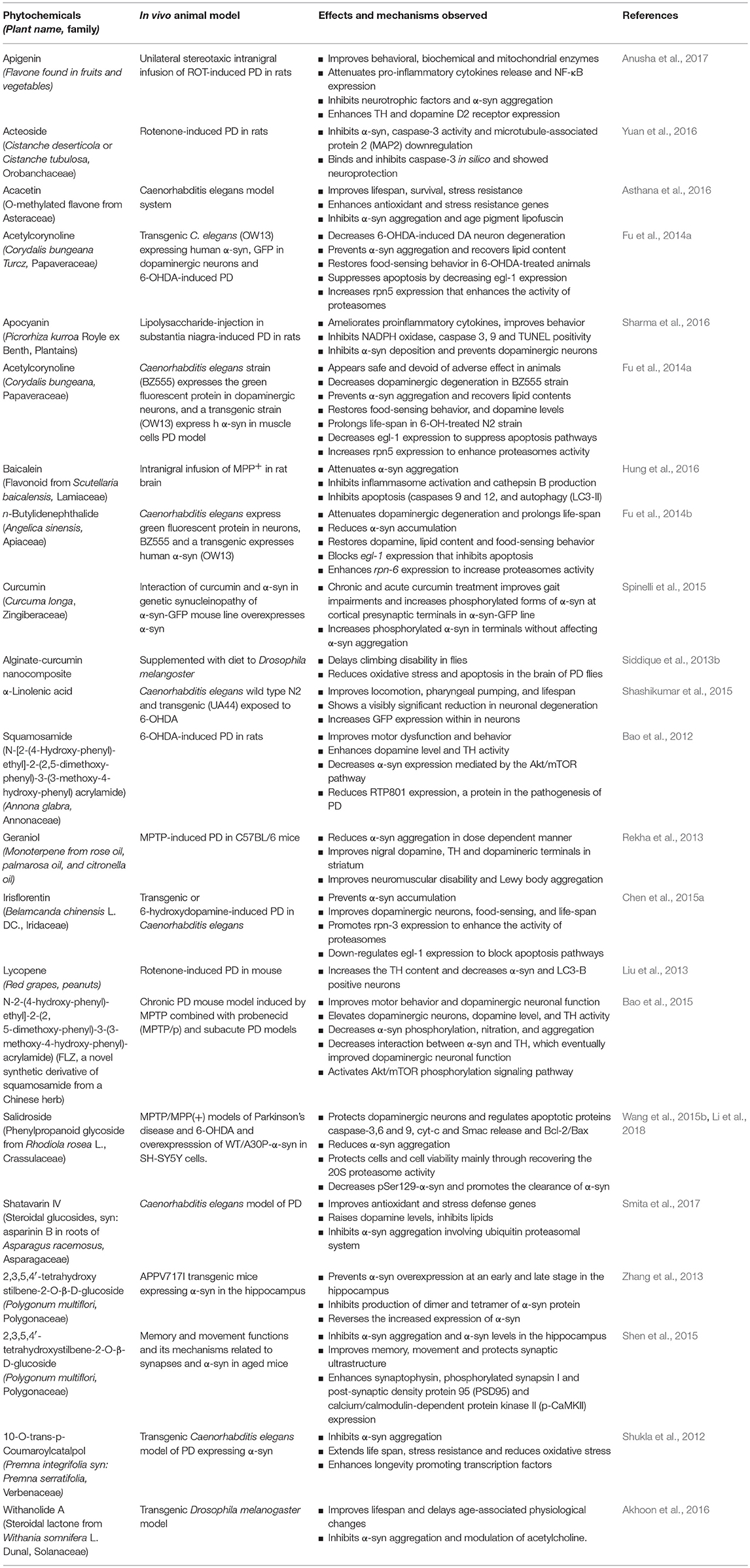
Table 3. The phytochemicals showed neuroprotective effects in the in vivo models of Parkinson's disease by targeting α-synuclein.
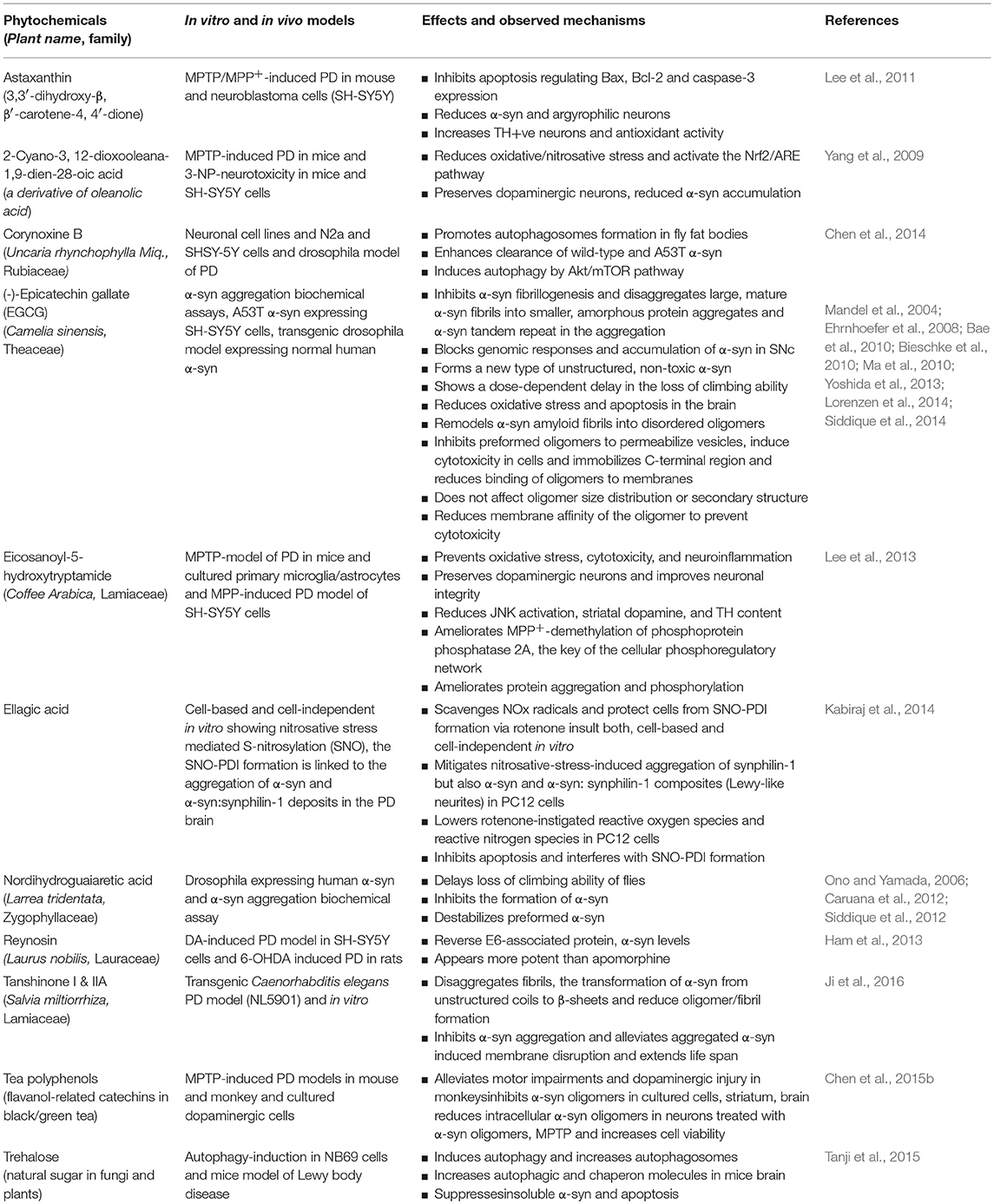
Table 4. The phytochemicals showed neuroprotective effects in both, the in vitro and in vivo models of Parkinson's disease by targeting α-synuclein.
Saponins are an abundant group of secondary metabolites that can be classified as triterpenoids, steroids, and glycosides (Dinda et al., 2010). Their effects in neurodegenerative, neuropsychiatric, and affective disorders were recently reviewed (Sun et al., 2015). Saponins possess surface-active and amphipathic properties (Lorenzen et al., 2014) that may contribute to their membrane-permeabilizing actions and surfactant-based disruption of α-syn fibril formation. Many of the glucosides, such as 3-O-demethylswertipunicoside, jatamanin 11, paeoniflorin, 2,3,5,4′-tetrahydroxy stilbene-2-O-β-D-glucoside, 10-O-trans-p-coumaroylcatalpol and strophanthidine attenuate neurotoxicity in experimental models by directly inhibiting α-syn aggregation or fibril formation. Similarly, many terpenoids, such as celastrol, 2-cyano-3, 12-dioxo-oleana-1,9-dien-28-oic acid, geraniol, reynosin, thymoquinone, and ginkgolide A, B, and C attenuate neurotoxicity in experimental models by directly inhibiting α-syn aggregation or fibril formation. However, asiatic acid failed to prevent α-syn aggregation and protofibril formation (Masuda et al., 2006).
Dietary intake of polyphenolic compounds is protective against neurodegeneration as evidenced from many epidemiologic and experimental studies (Ho and Pasinetti, 2010; Caruana et al., 2016; da Costa et al., 2017). Popular polyphenols in food are curcumin (present in turmeric), oleuropein (present in olive oil), resveratrol (present in grapes), catechins (present in black and green tea), astaxanthin (a carotenoid present in vegetables and fruits) and lycopene (present in tomato) (da Costa et al., 2017). Polyphenols inhibit α-syn aggregation and fibrillation (Masuda et al., 2006; Caruana et al., 2011, 2012; Sivanesam and Andersen, 2016) and formation of amyloid protofilaments and fibrils (Kumar et al., 2012; Velander et al., 2017) and confer protective effects in neurodegenerative diseases. Masuda et al. (2006) tested 79 compounds from different chemical classes of compounds including polyphenols, benzothiazoles, terpenoids, steroids, porphyrins, lignans, phenothiazines, polyene macrolides, and Congo red and its derivatives for their potential to inhibit α-syn assembly. Out of 39 polyphenolic compounds tested, 26 were found to inhibit α-syn assembly. These findings establish that polyphenols constitute a major class of compounds that can inhibit the assembly of α-syn. Several of them inhibited α-syn filament assembly with IC50 values in the low micromolar range. Caruana et al. (2011) investigated 14 polyphenolic compounds and black tea extract containing theaflavins and found that baicalein, scutellarein, myricetin, (-)-epigallocatechin-3-gallate (EGCG), nordihydroguaiaretic acid and black tea extract are the ideal candidates to investigate in experimental models for their direct effect on the inhibition of α-syn oligomer formation. The polyphenolic compounds are believed to interact with receptors or plasma membrane transporters and activate intracellular signaling pathways. Among several polyphenols, EGCG associates with the laminin receptor on vascular cells (Tachibana et al., 2004). Currently, numerous polyphenolic compounds have been studied for their effect on α-syn aggregation, fibrillation, elongation, nitration, and oligomerization using biophysical and biochemical techniques (Meng et al., 2010; Caruana et al., 2011, 2012; Takahashi et al., 2015). The list of these compounds is presented in Table 5. A scheme is presented in Figure 2 to depict the mechanism of action of the plant extracts and phytochemicals on α-syn oligomerization, fibrillation, and aggregation. An overview of some important phytochemicals which target α-syn aggregation and fibrillation and appear ideal candidates for further development is presented below.
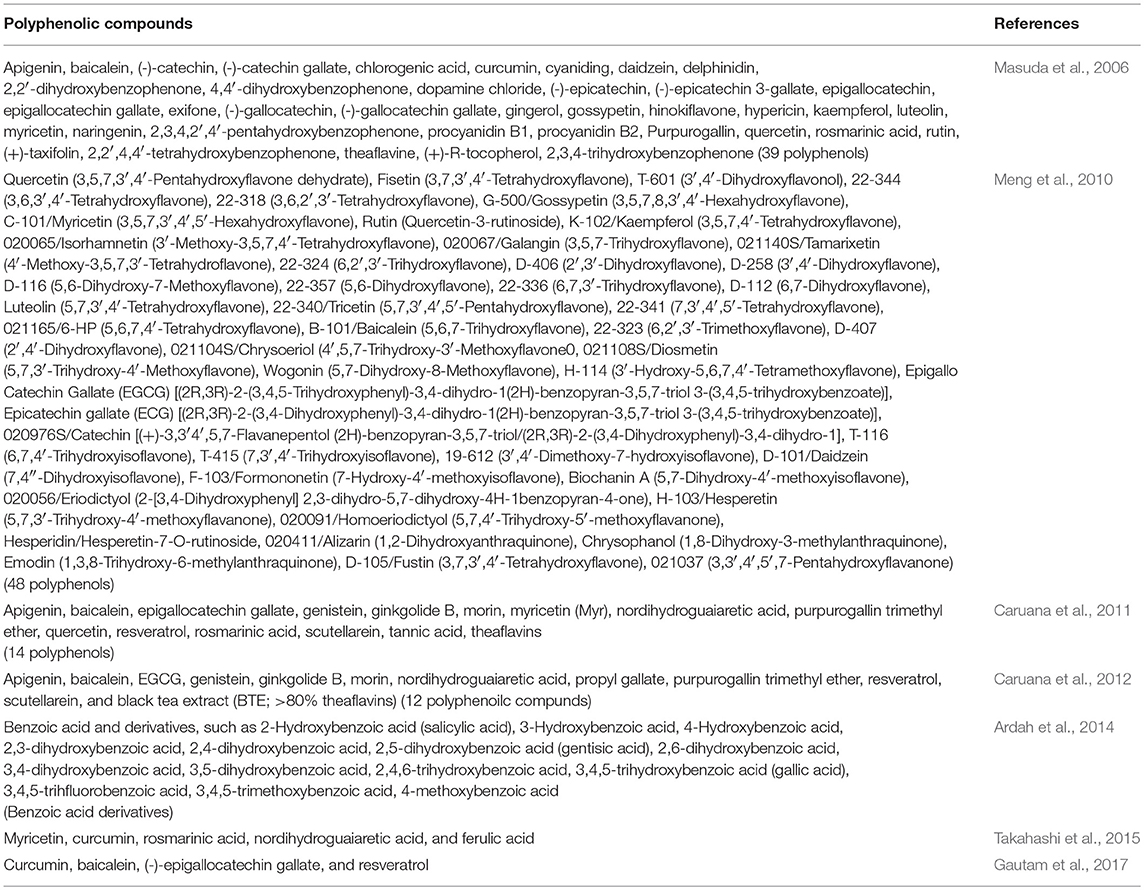
Table 5. The polyphenol compounds investigated for their action on α-synuclein fibrillation, aggregation, and cytotoxicity.
Baicalein
Baicalein is a flavone isolated from the roots of Scutellaria baicalensis Georgi (“Huang Qin” in Chinese), a reputed plant in traditional Chinese medicine (Gasiorowski et al., 2011) and Scutellaria pinnatifida grown in Iran (Sashourpour et al., 2017). In many studies, baicalein was shown to prevent α-syn oligomerization and fibrillation (Bomhoff et al., 2006; Meng et al., 2009; Caruana et al., 2011; Gasiorowski et al., 2011; Sashourpour et al., 2017). Baicalein interacts with α-syn through a tyrosine residue. Following oxidation, it generates quinone metabolites that bind covalently with a lysine side chain in α-syn. It prevents fibril formation and degrades preformed fibrils at low micromolar concentrations (Zhu et al., 2004). In another study, its non-covalent binding with α-syn and covalent modification by the oxidized form restricts the conformational changes in the unfolded protein that results in α-syn monomer and oligomer stabilization (Meng et al., 2009). The oligomers cause impairment of neuronal membrane integrity that results in disruption or permeabilization of the membrane, impairment of calcium homeostasis, and cell death (Caruana et al., 2012).
Baicalein prevents α-syn fibrillation and protects against neurotoxicity by preventing α-syn oligomer formation in SH-SY5Y and HeLa cells (Lu et al., 2011). It also stabilizes the oligomers, prevents further fibrillation (Hong et al., 2008) and tandem repeats of α-syn in the aggregation process (Bae et al., 2010). Further, it prevents the formation of annular protofibrils of α-syn induced by copper and reduces the β-sheet contents (Zhang et al., 2015). In another study, using JC-1, a probe that binds the α-syn C-terminal region, baicalein differentiated the α-syn fibrillation states (monomeric, oligomeric intermediate, and fibrillar forms) and reconfirmed the defibrillation action of baicalein on α-syn (Lee et al., 2009). In PC12 cells, it ameliorates cytotoxicity, mitochondrial depolarization, and inhibits proteasome inhibition induced by E46K, an α-syn point mutation that mimics familial PD (Jiang et al., 2010). In a recent study, baicalein induces autophagy, increases cell viability and reduces α-syn in the media of dopaminergic cell lines (SN4741) overexpressing A53T-syn (Li et al., 2017). Baicalein diminished the transmission of α-syn and prompted the polymerization of α-syn to a big complex rather than promoting clearance (Li et al., 2017). A recent study in rotenone-induced PD in rats showed reduced α-syn oligomer formation along with behavioral improvement and neurotransmitters in the striatum. However, it failed to reduce α-syn mRNA expression but prevented the transition from α-syn monomers to oligomers (Hu et al., 2016). Furthermore, baicalein attenuated α-syn aggregate formation, induced autophagy, inhibited apoptosis, reduced inflammation, and restored dopamine in PD induced by MPP+ infusion in the SNc of mice (Hung et al., 2016).
The baicalein derivative N′-benzylidene-benzohydrazide also attenuated oligomer formation (Kostka et al., 2008). Baicalein in combination with β-cyclodextrin (β-CD) synergistically inhibited α-syn aggregation and disaggregated preformed fibrils even at very low concentrations (Gautam et al., 2017). A combination of baicalein with specific proteolytic peptide sequences of α-syn was developed for targeted drug delivery and found to prevent α-syn fibrillation (Yoshida et al., 2013). Integrating evidence from in vitro and in vivo studies, baicalein appears to be a potential drug to inhibit α-syn aggregation, fibrillation, and propagation among the neurons.
Curcumin
Curcumin, chemically known as diferuloylmethane, is one of the most popular natural leads to drug discovery and development from turmeric (Molino et al., 2016). It is reputed for its dietary importance and health benefits and is the most studied phytochemical in experimental and clinical studies (Molino et al., 2016). It is a beneficial treatment in neurodegenerative diseases, including PD, and has antioxidant, anti-inflammatory, and antiapoptotic properties (Kim et al., 2012; Singh et al., 2013; Ji and Shen, 2014). Ono and Yamada (2006) found that curcumin possesses anti-fibrillogenic activity by inhibiting α-syn fibril formation and destabilizing preformed fibrils (Ono and Yamada, 2006). It was found to inhibit oligomerization of mutant α-syn into higher molecular weight aggregates (Pandey et al., 2008) and induce the dissociation of α-syn fibrils (Shoval et al., 2008). Curcumin treatment on mesencephalic cells did not affect α-syn fibril formation but enhanced LRRK2 mRNA and protein expression in rats (Ortiz-Ortiz et al., 2010). In neuroblastoma cells, curcumin attenuates cytotoxicity from aggregated α-syn, ROS generation, and diminished caspase-3 activation (Wang et al., 2010). In PC12 cells, curcumin ameliorates A53T mutant α-syn-induced PD (Liu et al., 2011). Further, curcumin reduces mutant α-syn accumulation by restoring macroautophagy, a process in the degradation pathway that clears proteins in cells by activating the mTOR/p70S6K signaling pathway (Jiang et al., 2013). Mechanistically, curcumin preferentially binds oligomeric intermediates rather than monomeric α-syn (Singh et al., 2013). Also, it binds strongly to the hydrophobic non-amyloid-β component of α-syn (Ahmad and Lapidus, 2012). The ordered structure is vital for effective binding and affects the extent of binding and potential in inhibiting oligomers or fibrils (Singh et al., 2013). The conformational and reconfiguration changes appear to govern the binding of curcumin to α-syn (Ahmad and Lapidus, 2012; Tavassoly et al., 2014). Curcumin, in combination with β-cyclodextrin, showed a synergistic inhibition of α-syn aggregation and degraded the preformed aggregates into monomers at very low concentrations (Gautam et al., 2014, 2017). Gautam et al. (2017) further demonstrated that a balanced arrangement of the phenolic groups, benzene rings, and flexibility attributes to the ability of curcumin. The phenolic groups enhance curcumin interactions with α-syn monomers as well as oligomers. In PC12 cells transfected with recombinant plasmids, α-syn-pEGFP-A53T downregulated α-syn expression or oligomer formation by regulating apoptosis-mediated mitochondrial membrane potential (Chen et al., 2015a). The effect of curcumin on α-syn observed in vitro was reconfirmed in vivo in genetic mouse models of synucleinopathy (Spinelli et al., 2015). Curcumin increased phosphorylated forms of α-syn at cortical presynaptic terminals but had no direct effect on α-syn aggregation. However, curcumin improved motor and behavioral performance (Spinelli et al., 2015).
Curcumin is less stable and soluble and has limited oral bioavailability. To improve its stability, solubility, and oral bioavailability, many nanoformulations or structural analogs have been developed (Gadad et al., 2012; Kundu et al., 2016; Taebnia et al., 2016). Curc-gluc, a modified curcumin preparation, inhibits α-syn oligomerization and fibrillation (Gadad et al., 2012). In another study, dehydrozingerone, zingerone; an O-methyl derivative of dehydrozingerone and their biphenyl analogs were investigated for their cytoprotective effects in PC12 cells challenged with H2O2, MPP+, and MnCl2 (Marchiani et al., 2013). The biphenyl analogs of dehydrozingerone and O-methyl-dehydrozingerone prevent α-syn aggregation; the biphenyl zingerone analog is the most potent inhibitor and has the most potent antioxidant activity. This activity was attributed to the hydroxylated biphenyl scaffold in the pharmacophore (Marchiani et al., 2013). In another study, stable curcumin analogs, such as curcumin pyrazole, curcumin isoxazole, and their derivatives, were evaluated against α-syn aggregation, fibrillation, and toxicity. Curcumin pyrazole and its derivative N-(3-Nitrophenyl pyrazole) curcumin reduces A53T-α-syn-induced neurotoxicity by preventing fibrillation and disrupting preformed fibrils (Ahsan et al., 2015). Taebnia et al. (2016) developed amine-functionalized mesoporous silica nanoparticles of curcumin to enhance its bioavailability and evaluated its effect against cytotoxicity and α-syn fibrillation (Taebnia et al., 2016). This nanoformulation showed interaction with α-syn species and prevented fibrillation with negligible effect on cytotoxicity (Taebnia et al., 2016). A nanoformulation containing curcumin and piperine with glyceryl monooleate nanoparticles coated with various surfactants was developed for targeted delivery to enhance its bioavailability in the brain (Kundu et al., 2016). The nanoformulation has been shown to attenuate oxidative stress, apoptosis, prevent α-syn oligomerization and fibrillation, and induce autophagy. Another nanoformulation prepared with lactoferrin by sol-oil chemistry ameliorates rotenone-induced neurotoxicity in dopaminergic SK-N-SH cells (Bollimpelli et al., 2016). This nanoformulation exhibited better availability, improved cell viability, attenuated oxidative stress, and reduced tyrosine hydroxylase and α-syn expression. Nine curcumin analogs were synthesized by substitution of groups on the aromatic ring which alters the hydrophobicity, promotes stability, and facilitates binding with the fibrils as well as oligomers (Jha et al., 2016). Some of the analogs showed improved stability and appeared to interact with oligomers and preformed fibrils. The analogs exhibited differential binding patterns and augmented α-syn aggregation, generating different kinds of amyloid fibrils. The liposomal nanohybrid of curcumin with polysorbate 80-modified cerasome was developed for targeted drug delivery in the striatum and showed better half-life and bioavailability (Zhang et al., 2018). This nanoformulation ameliorated motor deficits and improved dopamine and tyrosine hydroxylase expression by promoting α-syn clearance in a mouse model of MPTP-induced PD (Zhang et al., 2018). Curcumin inhibited α-syn aggregates in dopaminergic neurons and attenuated oxidative stress, inflammation, apoptosis, and motor deficits in a rat model of lipopolysaccharide-induced PD (Sharma and Nehru, 2018). These reports demonstrate the effect of curcumin on α-syn aggregation- and fibrillation-induced neurotoxicity but further studies are still needed to demonstrate therapeutic success.
Cuminaldehyde
Cuminaldehyde is isolated from many edible plants including Artemisia salsoloides, Aegle marmelos, and spices cumin (Cuminum cyminum L.) and is used as a food additive and flavoring agent in many cuisines in the Middle East, South Asia, and Mediterranean countries. Cuminaldehyde isolated from Iranian cumin showed to inhibit α-syn fibrillation (Morshedi et al., 2015). It prevented α-syn fibrillation even in the presence of seeds with negligible disaggregating effect on the preformed fibrils of α-syn. Interestingly, it was found to be superior to baicalein, a known inhibitor of α-syn fibrillation and blocked protein assembly into β-structural fibrils that were attributed to interaction with amine and aldehyde groups in the chemical structure (Morshedi and Nasouti, 2016).
Catechins, Theaflavins, and (-)-Epigallocatechin-3-Gallate (EGCG)
Catechins, the polyphenolic compounds present in black and green tea, are protective in neurodegenerative diseases (Caruana and Vassallo, 2015; Jha et al., 2017; Xu et al., 2017; Pervin et al., 2018). Theaflavins present in fermented black tea inhibits fibrillogenesis of α-syn and amyloid-β formation (Grelle et al., 2011). These compounds facilitate the assembly of amyloid-β and α-syn into non-toxic, spherical aggregates which are unable to undergo seeding to form amyloid plaques. They were also found to remodel the formed amyloid-β fibrils into non-toxic aggregates and these effects were comparable to EGCG. Theaflavins also appeared less vulnerable to oxidation in air and exhibited better activity in oxidizing environments in comparison with EGCG (Grelle et al., 2011).
One of the most popular catechins, (-)-Epigallocatechin 3-gallate (EGCG), is a flavonol compound predominantly present in green tea, a popular beverage across the world. EGCG inhibited α-syn aggregation and fibrillation in a concentration-dependent manner (Šneideris et al., 2015; Xu et al., 2017), and by disaggregating mature and large α-syn fibrils into smaller, non-toxic, amorphous aggregates (Ehrnhoefer et al., 2008). EGCG binds directly to the natively unfolded polypeptides and inhibits their conversion into toxic intermediates (Ehrnhoefer et al., 2008). It induces a conformational change without their disassembly into monomers or small diffusible oligomers (Bieschke et al., 2010). It appears to bind directly with β-sheet-rich aggregates and reduces its concentration required to induce conformational changes (Liu et al., 2018). Furthermore, it showed neuroprotection against free radicals and α-syn toxicity by chelating Fe (III) in PC12 cells transfected with α-syn and exposed to β-sheet-enriched α-syn fibrils (Zhao et al., 2017). EGCG appears to disaggregate α-syn fibrils by preventing the amyloid formation of α-syn tandem repeat and destabilizing α-syn fibrils into soluble amorphous aggregates (Bae et al., 2010). This study also revealed that the tandem repeat of α-syn may be used as a molecular model to study the mechanism of α-syn aggregation (Bae et al., 2010). Further, EGCG also prevents α-syn aggregation and accumulation by activating the hypoxia-inducible factor (HIF)-1 signaling mechanism that controls α-syn aggregation by regulating antioxidant and iron homeostasis (Weinreb et al., 2013).
Lorenzen et al. (2014) showed that EGCG has potential to prevent α-syn oligomer formation and attenuate the oligomer cytotoxicity by preventing vesicle permeabilization and blocking the membrane affinity of syn to bind and immobilize in the C-terminal region. Though, it failed to affect the oligomer size distribution or secondary structure. Recently, in primary cortical neuron cultures challenged with oxidative injury, quercetin, EGCG, and cyanidin-3-glucoside inhibited fibrillation of α-syn and apoptosis (Pogacnik et al., 2016). Further, it decreased amyloid fibril formation on the surface of liposomal membranes and generates compact oligomers following off-pathway, as well as facilitating the conversion of active oligomers into amyloid fibrils (Yang et al., 2017). A combination of EGCG with specific α-syn proteolytic peptide sequences was developed for targeted drug delivery and found to prevent the α-syn fibrillation (Yoshida et al., 2013). In combination, this evidence suggests EGCG could be a promising treatment in neurodegenerative diseases and a good candidate for pharmaceutical development and dietary inclusion.
Gallic Acid
Gallic acid, a type of phenolic acid chemically known as 3,4,5-trihydroxybenzoic acid, is found in free form or as part of the hydrolyzable tannins in many plants, such as gallnuts, sumac, witch hazel, tea leaves, and oak bark (Kosuru et al., 2018). Gallic acid and esters are well-known food additives, nutritional supplements, and a common reagent in the pharmaceutical analysis (Kosuru et al., 2018). Over the last few decades, many investigators showed the antioxidative, antiapoptotic, cardioprotective, neuroprotective and anticancer properties of gallic acid and gallates (Blainski et al., 2013; Choubey et al., 2018; Kosuru et al., 2018). It is used as a reference compound for the quantification of the phenolic contents in biochemical assays; Folin-Ciocalteau assay or Folin's phenol reagent or Folin-Denis reagent which determines the antioxidant power in gallic acid equivalents (Blainski et al., 2013). The polyphenolic compound gallic acid and its structurally similar benzoic acid derivatives elicit anti-aggregating effects (Ardah et al., 2014). Gallic acid impedes α-syn fibrillation and disaggregates the preformed fibrils of α-syn in a battery of biophysical, biochemical, and cell viability assays. In addition to inhibiting aggregation and disaggregation, it also binds to soluble and non-toxic oligomers devoid of β-sheet content and confers structural stability. Numerous benzoic acid derivatives have been developed using structure-activity relationship and all inhibit α-syn fibrillation (Ardah et al., 2014). The number of hydroxyl groups and their presence on the phenyl ring in these structural derivatives of gallic acid are believed to attribute to the potential mechanism in binding and inhibiting α-syn fibrillation. Furthermore, gallic acid prevents α-syn amyloid fibril formation, stabilizes the extended intrinsic structure of α-syn, and reacts rapidly in biochemical assays (Liu et al., 2014).
Ginsenosides
Ginseng, also known as red ginseng (Panax ginseng, Araliaceae), is a popular source of saponins and is reputed in the folk medicine of the Far East countries. It has shown neuroprotective effects in numerous neurodegenerative diseases including PD (Van Kampen et al., 2003; Chen et al., 2005; Luo et al., 2011). The ginseng extract, abbreviated as G115, confers neuroprotection against MPTP and its neurotoxic metabolite, MPP+ in murine models of PD (Van Kampen et al., 2003). Van Kampen et al. (2014) reported that G115 treatment reduces dopaminergic cell loss, microgliosis, the buildup of α-syn aggregates, and improves locomotor activity and coordination in rats chronically exposed to the dietary phytosterol glucoside, β-sitosterol β-d-glucoside, which recapitulates features of PD.
Several studies identified the active constituents of ginseng known as ginsenosides, a group of 60 compounds that possess a wide range of pharmacological and physiological actions (Mohanan et al., 2018; Zheng et al., 2018). Several ginsenosides, Rg1, Rg3, and Rb1, were investigated for their effect on α-syn aggregation using biophysical and biochemical techniques (Ardah et al., 2015; Heng et al., 2016). Upon oral treatment, Rg1 attenuated neurodegeneration in a mouse model of MPTP-induced PD by inhibiting pro-inflammatory cytokines; reducing mortality, behavioral defects, and dopamine neuron loss; and correcting ultrastructure changes in the SNc (Heng et al., 2016). Rg1 also reduced oligomeric, phosphorylated, and disease-related α-syn in the SNc. In contrast, a separate study identified only Rb1 as a strong inhibitor of α-syn fibrillation; it also disaggregated preformed fibrils and inhibited the seeded polymerization of α-syn (Ardah et al., 2015). Further, Rb1 binds to the oligomers and causes stabilization of soluble, non-toxic oligomers with negligible involvement of β-sheets that depicts a novel mechanism of action (Ardah et al., 2015). Although the authors did not find a significant effect of Rg1 and Rg3 on α-syn aggregation in cellular models (Ardah et al., 2015). In one of the study, Rg3, another ginsenoside present in Panax ginseng, reduced α-syn expression in stress models (Xu et al., 2013). The evidence of Rg3-mediated changes in α-syn in stress models needs to be investigated in PD (Xu et al., 2013). A detailed investigation is required to understand the observed differences in the in vitro and in vivo studies.
Resveratrol
Resveratrol, a natural phytoestrogen found in grapes and red wine, is reputed for its neuroprotective properties by attenuating oxidative stress, mitochondrial impairment, inducing apoptotic cell death and promoting autophagy (Caruana et al., 2016; Ur Rasheed et al., 2016). Wu et al. (2011) showed that resveratrol enhanced α-syn degradation in PC12 cells expressing α-syn by activating autophagy and mediating the induction of AMP-activated protein kinase (AMPK) mammalian silent information regulator 2 (SIRT1) signaling mechanism. This reduces protein levels of microtubule-associated protein 1 light chain 3 (LC3-II) and preserves neuronal cells. AMPK is a serine/threonine kinase which acts as a metabolic energy sensor to maintain energy balance; upon activation, it induces neuronal cell apoptosis and decreases SIRT1 leading to activation of the ubiquitin-proteasome pathway by enhancing ubiquitination and promoting SUMOylation that may be important in reducing the progression of neurodegeneration (Wu et al., 2011). The induction of autophagy and apoptotic pathways represents an important approach in the therapeutic targeting of α-syn (Ghavami et al., 2014). Further, in MPTP-induced PD in mice, resveratrol corrected the behavioral and motor deficits and attenuated neurodegeneration by inducing autophagy of α-syn via activation of SIRT1 and subsequent deacetylation of LC3 (Guo et al., 2016). Recently, in an effort to enhance the bioavailability to attain therapeutic benefits, resveratrol was prepared with β-CD; this combination was found synergistic in showing activity at very low concentrations to prevent α-syn aggregation as well as disaggregate preformed fibrils (Gautam et al., 2017). Resveratrol treatment reduced α-syn oligomers in S1/S2 transfected human H4 neuroglioma cells by activating peroxisome proliferator-activated receptor γ (PPARγ), which regulates energy metabolism and mitochondrial biogenesis, and plays a role in the pathogenesis of PD (Eschbach et al., 2015). At the molecular level, resveratrol downregulates α-syn expression mediating miR-214 in the MPTP-induced mouse model of PD and MPP+ induced neurotoxicity in neuroblastoma cells (Wang et al., 2015a).
The phytochemicals that inhibit fibrils and oligomer formation along with the ability to stabilize the α-syn oligomers or disaggregate α-syn oligomers can be potential compounds for pharmaceutical development. The in vitro data reveals success with many phytochemicals in ameliorating the fibrils and oligomer formation of α-syn as well as inducing degradation of α-syn and promoting autophagy. However, many polyphenolic compounds showed difficulty in crossing the blood brain barrier due to their non-lipophilic nature. Therefore, they may not attain the required concentration to exert effects in the brain (Pandareesh et al., 2015; Pogacnik et al., 2016). Several factors, such as stability, solubility in an acidic environment at gastric pH, absorption pattern, gut microflora, enterohepatic circulation, first pass metabolism, and metabolic pattern either phase I or phase II play a key role in achieving the ideal bioavailability of the phytochemicals in the brain (Scholz and Williamson, 2007). Additionally, the inconsistency between the in vitro concentration and in vivo dose in certain models encourages systematic pharmacokinetic evaluations to understand the variation between the in vitro and in vivo data.
Experimental Techniques to Assess the α-syn Inhibitory Activity of Phytochemicals
Several biophysical and biochemical techniques used to assess the ability of phytochemicals and plant extracts in preventing α-syn oligomerization and fibrillation are represented in Table 6. These experimental techniques include surface plasmon resonance imaging (SPRi), Thioflavin-T (ThT) fluorescence, transmission electron microscopy (TEM), small angle X-ray scattering (SAXS), circular dichroism (CD) spectroscopy, fourier transform infrared spectroscopy (FTIR), nuclear magnetic resonance (NMR), and absorption spectroscopy of Congo red (CR) binding assay (Luk et al., 2007; Kostka et al., 2008; Celej et al., 2009; Yamaguchi et al., 2010; Giehm et al., 2011; da Silva et al., 2013; Aelvoet et al., 2014; Coelho-Cerqueira et al., 2014; Cheng et al., 2015; Fazili and Naeem, 2015; Takahashi et al., 2015; Pujols et al., 2017; Das et al., 2018). The biochemical and biophysical assays employed to measure the α-syn aggregation are efficient in providing a high-resolution structure of α-syn oligomers, but not free from the restrictions and misconceptions on their efficacy (Coelho-Cerqueira et al., 2014). The polyphenolic nature of phytochemicals may cause some variations in interferences in spectrophotometric and fluorescent assays used to measure α-syn formation (Coelho-Cerqueira et al., 2014). Coelho-Cerqueira et al. (2014) show the drawbacks related to the application of ThT assays to examine α-syn fibrillation as ThT reacts with disordered α-syn monomer and augments protein fibrillation in vitro. As a result, phytochemicals may also bias the ThT assay and ambiguous results may be interpreted from the application of ThT based real-time assays in the screening of anti-fibrillogenic compounds. Therefore, a battery of techniques is recommended to support or confirm the anti-aggregatory and anti-fibrillogenic activity in side-stepping the possible artifacts associated with the measure of ThT fluorescence (Coelho-Cerqueira et al., 2014).
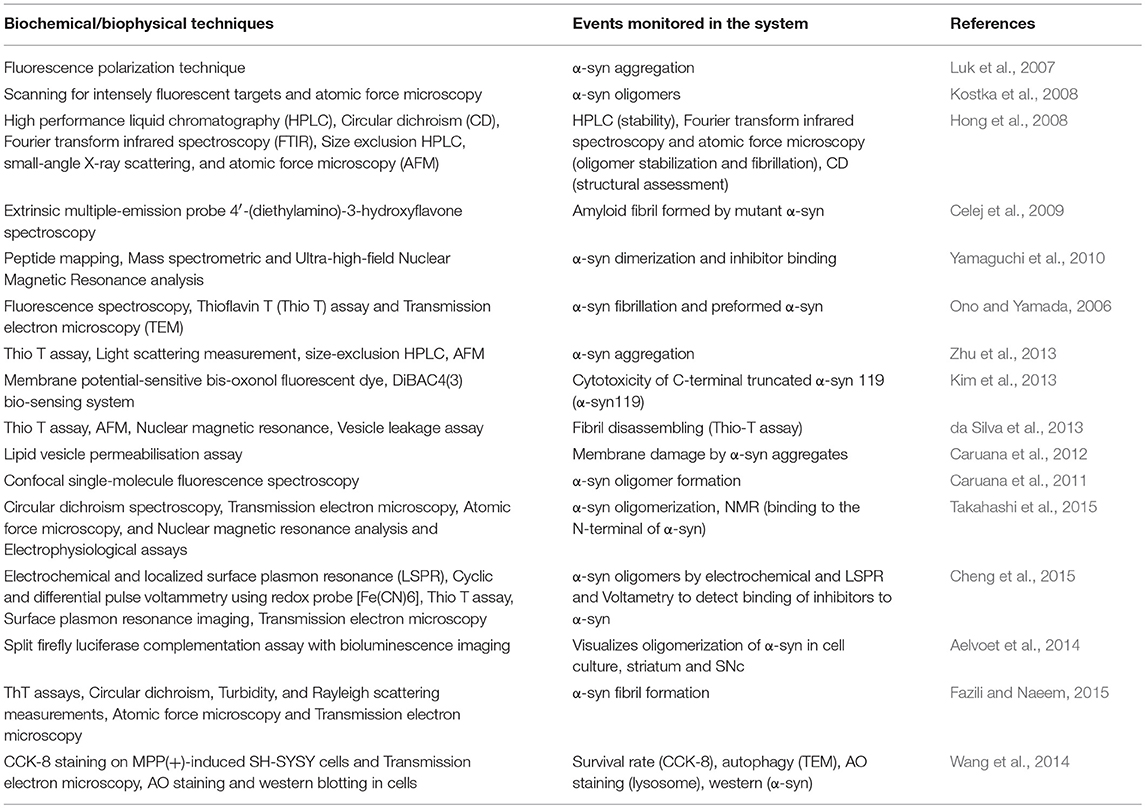
Table 6. The bioanalytical techniques employed to determine α-synuclein oligomerization, fibrillation, and cytotoxicity.
The knowledge of the stage, form, nature, and propagation of α-syn that play a key role in enhanced toxicity in PD highlights the multitude of techniques needed to gauge α-syn disposition in experimental situations (Giehm et al., 2011; Pujols et al., 2017). Recently, Moree et al. (2015) developed a novel assay to recognize compounds that control the conformation of monomeric α-syn in a direct manner to reduce the encounters associated with conventional small molecule screening of α-syn. This novel assay may aid in understanding the role of α-syn oligomers in PD and opens new avenues to evaluate α-syn-based potential neuroprotective agents.
Experimental Models for Screening Agents Targeting α-syn Toxicity
Apart from biophysical and biochemical assays, numerous models including cell lines (in vitro) and animal models (in vivo) have been developed to study the role of α-syn in the etiology and disease modification of PD and evaluate test compounds against α-syn (Skibinski and Finkbeiner, 2011; Javed et al., 2016; Visanji et al., 2016; Ko and Bezard, 2017; Lázaro et al., 2017). The experimental models showing α-syn fibrillation, oligomerization, and neurotoxicity are summarized in Table 7. The toxicant models and mutation-based in vivo models are popularly used to mimic sporadic and familial PD, respectively (Javed et al., 2016). The experimental models often present issues and challenges in separating the complexities of cellular and molecular mechanisms and are infeasible for high-throughput screening and other drug development stages, such as dose-response toxicology studies. Cell-based models including stem cells and primary neurons with features of dopaminergic neurons give important insights into the cellular mechanisms of PD for drug discovery (Lázaro et al., 2017). This enables the recognition of agents targeting α-syn and their molecular mechanisms. Some of the prominent human cell models for PD drug screening are SK-N-SH, SHSY5Y, and SK-N-MC (Skibinski and Finkbeiner, 2011; Lázaro et al., 2017). The development of α-syn-based experimental models of sporadic or familial PD that show progressive forms of the disease will elucidate the mechanisms of neurodegeneration and aid in the identification of phytochemicals modulating α-syn. The uptake of recombinant α-syn from the culture medium has been reported in many cellular models (Reyes et al., 2015). Recently, Reyes et al. (2015) established a culture system that is a physiologically appropriate assay for the characterization of genetic modifiers or small molecules which prevent cell-to-cell transfer or propagation of α-syn.
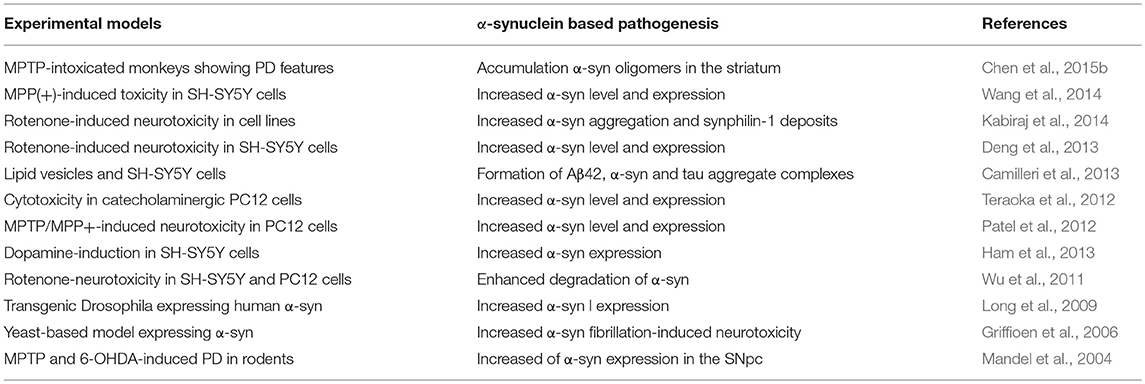
Table 7. The experimental models used to evaluate plant extracts and phytochemicals against neurotoxicity mediating α-synuclein oligomerization, and fibrillation.
Concluding Remarks and Future Prospects
This comprehensive review presents an overview of the plant extracts and phytochemicals specifically targeting α-syn oligomerization, fibrillation, and aggregation in different models of PD and their underlying mechanisms. It also discusses the experimental techniques and models used to evaluate the plant extracts and phytochemicals. The literature review suggests that many phytochemicals are promising in targeting α-syn in the in vitro studies; however, the actions observed in vitro need to be reconfirmed in vivo. Indeed, the screening of phytochemicals or plant extracts in cell lines often lacks clinical applicability due to physiological, biochemical, and pharmacological relevancy. The available literature from a convincing number of in vitro studies and few in vivo studies demonstrates that phytochemicals, such as baicalein, curcumin, resveratrol, and epigallocatechin gallate have promising therapeutic potential in inhibiting α-syn oligomerization, fibrillation, aggregation, and accumulation. All these promising compounds should be studied in the in vivo studies to proceed further for clinical studies and thereon.
The process of α-syn oligomerization and fibrillation were well-recognized but the triggers that induce α-syn aggregation are not yet well-established. Thus, for a fair translational disease modifying approach, evaluation of phytochemicals in animal models involving α-syn aggregation and mimicking the progressive nature of PD pathogenesis is desired as a proof of concept. Although the available preclinical studies are encouraging, they are markedly speculative for clinical success. The issues, such as bioavailability, stability, metabolism, as well as long-term safety and toxicity, should be resolved before pharmaceutical development and further testing in humans. Based on the available preclinical studies, it can be concluded that these phytochemicals could possibly be novel drug candidates for neurodegenerative diseases, such as PD.
Author Contributions
All the authors provided important intellectual content, reviewed the content and approved the final version of this manuscript. AA and SO conceptualized the idea for this review. MFNM, SA, HJ, and SO performed the literature search. HJ and SO wrote the first draft of the manuscript. SO and AA thoroughly revised and edited the manuscript. BS drew the chemical structures.
Conflict of Interest Statement
The authors declare that the research was conducted in the absence of any commercial or financial relationships that could be construed as a potential conflict of interest.
Acknowledgments
The authors sincerely acknowledge the research grant support from United Arab Emirates University awarded as University Program for Advanced Research and Center Based Interdisciplinary research grant #31R127.
References
Aelvoet, S. A., Ibrahimi, A., Macchi, F., Gijsbers, R., Van den Haute, C., Debyser, Z., et al. (2014). Noninvasive bioluminescence imaging of α-synuclein oligomerization in mouse brain using split firefly luciferase reporters. J. Neurosci. 34, 16518–16532. doi: 10.1523/JNEUROSCI.4933-13.2014
Ahlskog, J. E. (1994). Treatment of Parkinson's disease. From theory to practice. Postgrad. Med. 95, 52–54, 57–58. doi: 10.1080/00325481.1994.11945830
Ahmad, B., and Lapidus, L. J. (2012). Curcumin prevents aggregation in α-synuclein by increasing reconfiguration rate. J. Biol. Chem. 287, 9193–9199. doi: 10.1074/jbc.M111.325548
Ahsan, N., Mishra, S., Jain, M. K., Surolia, A., and Gupta, S (2015). Curcumin pyrazole and its derivative (N-(3-Nitrophenylpyrazole) Curcumin inhibit aggregation, disrupt fibrils and modulate toxicity of wild type and mutant α-synuclein. Sci. Rep. 5:9862. doi: 10.1038/srep09862
Akhoon, B. A., Pandey, S., Tiwari, S., and Pandey, R. (2016). Withanolide A offers neuroprotection, ameliorates stress resistance and prolongs the life expectancy of Caenorhabditis elegans. Exp. Gerontol. 78, 47–56. doi: 10.1016/j.exger.2016.03.004
Albani, D., Polito, L., Batelli, S., De Mauro, S., Fracasso, C., Martelli, G., et al. (2009). The SIRT1 activator resveratrol protects SK-N-BE cells from oxidative stress and against toxicity caused by alpha-synuclein or amyloid-beta (1-42) peptide. J. Neurochem. 110, 1445–1456. doi: 10.1111/j.1471-4159.2009.06228.x
Alhebshi, A.H., Odawara, A., Gotoh, M., and Suzuki, I. (2014). Thymoquinone protects cultured hippocampal and human induced pluripotent stem cells-derived neurons against α-synuclein-induced synapse damage. Neurosci. Lett. 570, 126–131. doi: 10.1016/j.neulet.2013.09.049
Amro, M. S, Teoh, S. L, Norzana, A. G., and Srijit, D. (2018). The potential role of herbal products in the treatment of Parkinson's disease. Clin. Ter. 169, e23–e33. doi: 10.7417/T.2018.2050
Andrich, K., and Bieschke, J. (2015). The Effect of (-)-Epigallo-catechin-(3)-gallate on amyloidogenic proteins suggests a common mechanism. Adv. Exp. Med. Biol. 863, 139–161. doi: 10.1007/978-3-319-18365-7_7
Anusha, C., Sumathi, T., and Joseph, L.D. (2017). Protective role of apigenin on rotenone induced rat model of Parkinson's disease: suppression of neuroinflammation and oxidative stress mediated apoptosis. Chem. Biol. Interact. 269, 67–79. doi: 10.1016/j.cbi.2017.03.016
Ardah, M. T., Paleologou, K. E., Lv, G., Abul Khair, S. B., Kazim, A. S., Minhas, S. T., et al. (2014). Structure activity relationship of phenolic acid inhibitors of α-synuclein fibril formation and toxicity. Front. Aging Neurosci. 6:197. doi: 10.3389/fnagi.2014.00197
Ardah, M. T., Paleologou, K. E., Lv, G., Menon, S. A., Abul Khair, S. B., Lu, J.H., et al. (2015). Ginsenoside Rb1 inhibits fibrillation and toxicity of alpha-synuclein and disaggregates preformed fibrils. Neurobiol. Dis. 74, 89–101. doi: 10.1016/j.nbd.2014.11.007
Asthana, J., Mishra, B. N., and Pandey, R. (2016). Acacetin promotes healthy aging by altering stress response in Caenorhabditis elegans. Free Radic. Res. 50, 861–874. doi: 10.1080/10715762.2016.1187268
Attia, A. H., Gadelkarim, M., Morsi, M., Awad, K., Elnenny, M., Ghanem, E., et al. (2017). Meta-analysis of creatine for neuroprotection against Parkinson's disease. CNS Neurol. Disord. Drug Targets 16, 169–175. doi: 10.2174/1871527315666161104161855
Bae, S. Y., Kim, S., Hwang, H., Kim, H. K., Yoon, H. C., Kim, J. H., et al. (2010). Amyloid formation and disaggregation of α-synuclein and its tandem repeat (α-TR). Biochem. Biophys. Res. Commun. 400, 531–536. doi: 10.1016/j.bbrc.2010.08.088
Bagchi, P., and Hopper, W. (2011). “Virtual screening of compounds from Valeriana Jatamansi with α-Synuclein,” in 2011 International Conference on Bioscience, Biochemistry, and Bioinformatics, IPCBEE, Vol. 5. © (2011) (Singapore: IACSIT Press).
Bao, X. Q., Kong, X. C., Qian, C., and Zhang, D. (2012). FLZ protects dopaminergic neuron through activating protein kinase B/mammalian target of rapamycin pathway and inhibiting RTP801 expression in Parkinson's disease models. Neuroscience 202, 396–404. doi: 10.1016/j.neuroscience.2011.11.036
Bao, X. Q., Wu, L. Y., Wang, X. L., Sun, H., and Zhang, D. (2015). Squamosamide derivative FLZ protected tyrosine hydroxylase function in a chronic MPTP/probenecid mouse model of Parkinson's disease. Naunyn Schmiedebergs. Arch. Pharmacol. 388, 549–556. doi: 10.1007/s00210-015-1094-5
Bartels, T., Choi, J. G., and Selkoe, D. J. (2011). α-synuclein occurs physiologically as a helically folded tetramer that resists aggregation. Nature 477, 107–110. doi: 10.1038/nature10324
Beal, M. F., Oakes, D., Shoulson, I., Henchcliffe, C., Galpern, W. R., Haas, R., et al. (2014). Parkinson Study Group QE3 Investigators, a randomized clinical trial of high-dosage coenzyme Q10 in early Parkinson disease: no evidence of benefit. JAMA Neurol. 71, 543–552. doi: 10.1001/jamaneurol.2014.131
Bendor, J. T., Logan, T. P., and Edwards, R. H. (2013). The function of -synuclein. Neuron 79, 1044–1066. doi: 10.1016/j.neuron.2013.09.004
Bergström, A. L., Kallunki, P., and Fog, K. (2016). Development of passive immunotherapies for synucleinopathies. Mov. Disord. 31, 203–213. doi: 10.1002/mds.26481
Bernd, K. (2008). Phytochemical approach and bioanalytical strategy to develop chaperone-based medications. Open Biochem. J. 2, 44–48. doi: 10.2174/1874091X00802010044
Berrocal, R., Vasudevaraju, P., Indi, S. S., Sambasiva Rao, K. R., and Rao, K. S. (2014). In vitro evidence that an aqueous extract of Centella asiatica modulates α-synuclein aggregation dynamics. J. Alzheimers Dis. 39, 457–465. doi: 10.3233/JAD-131187
Bertoncini, C. W., Jung, Y.-S., Fernandez, C. O., Hoyer, W., Griesinger, C., Jovin, T. M., et al. (2005). Release of long-range tertiary interactions potentiates aggregation of natively unstructured α-synuclein. Proc. Natl. Acad. Sci. U.S.A. 102, 1430–1435. doi: 10.1073/pnas.0407146102
Beutler, J. A. (2009). Natural products as a foundation for drug discovery. Curr. Protoc. Pharmacol. 9, 9–11. doi: 10.1002/0471141755.ph0911s46
Bieschke, J., Russ, J., Friedrich, R. P., Ehrnhoefer, D. E., Wobst, H., Neugebauer, K., et al. (2010). EGCG remodels mature alpha-synuclein and amyloid-beta fibrils and reduces cellular toxicity. Proc. Natl. Acad. Sci. USA. 107, 7710–7715. doi: 10.1073/pnas.0910723107
Blainski, A., Lopes, G. C., and de Mello, J. C. (2013). Application and analysis of the Folin Ciocalteu method for the determination of the total phenolic content from Limonium brasiliense L. Molecules 18, 6852–6865. doi: 10.3390/molecules18066852
Bollimpelli, V. S., Kumar, P., Kumari, S., and Kondapi, A. K. (2016). Neuroprotective effect of curcumin-loaded lactoferrin nano particles against rotenone induced neurotoxicity. Neurochem. Int. 95, 37–45. doi: 10.1016/j.neuint.2016.01.006
Bomhoff, G., Sloan, K., McLain, C., Gogol, E. P., and Fisher, M. T. (2006). The effects of the flavonoid baicalein and osmolytes on the Mg2+ accelerated aggregation/fibrillation of carboxymethylated bovine 1SS-alpha-lactalbumin. Arch. Biochem. Biophys. 453, 75–86. doi: 10.1016/j.abb.2006.02.001
Borghi, R., Marchese, R., Negro, A., Marinelli, L., Forloni, G., Zaccheo, D., et al. (2000). Full length alpha-synuclein is present in cerebrospinal fluid from Parkinson's disease and normal subjects. Neurosci. Lett. 287, 65–67. doi: 10.1016/S0304-3940(00)01153-8
Briffa, M., Ghio, S., Neuner, J., Gauci, A. J., Cacciottolo, R., Marchal, C., et al. (2017). Extracts from two ubiquitous Mediterranean plants ameliorate cellular and animal models of neurodegenerative proteinopathies. Neurosci. Lett. 638, 12–20. doi: 10.1016/j.neulet.2016.11.058
Broersen, K., Ruiperez, V., and Davletov, B. (2018). Structural and aggregation properties of alpha-synuclein linked to phospholipase A2 action. Protein Pept. Lett. 25, 368–378. doi: 10.2174/0929866525666180326120052
Brundin, P., Dave, K. D., and Kordower, J. H. (2017). Therapeutic approaches to target alpha-synuclein pathology. Exp. Neurol. 298, 225–235. doi: 10.1016/j.expneurol.2017.10.003
Burre, J., Sharma, M., Tsetsenis, T., Buchman, V., Etherton, M. R., and Sudhof, T. C. (2010). α-synuclein promotes SNARE-complex assembly in vivo and in vitro. Science 329, 1663–1667. doi: 10.1126/science.1195227
Büttner, S., Broeskamp, F., Sommer, C., Markaki, M., Habernig, L., Alavian-Ghavanini, A., et al. (2014). Spermidine protects against α-synuclein neurotoxicity. Cell Cycle 13, 3903–3908. doi: 10.4161/15384101.2014.973309
Camilleri, A., Zarb, C., Caruana, M., Ostermeier, U., Ghio, S., Högen, T., et al. (2013). Mitochondrial membrane permeabilisation by amyloid aggregates and protection by polyphenols. Biochim. Biophys. Acta 1828, 2532–2543. doi: 10.1016/j.bbamem.2013.06.026
Cannon, J. R., and Greenamyre, J. T. (2013). Gene-environment interactions in Parkinson's disease: specific evidence in humans and mammalian models. Neurobiol. Dis. 57, 38–46. doi: 10.1016/j.nbd.2012.06.025
Caruana, M., Cauchi, R., and Vassallo, N. (2016). Putative role of red wine polyphenols against brain pathology in Alzheimer's and Parkinson's disease. Front Nutr. 3:31. doi: 10.3389/fnut.2016.00031
Caruana, M., Högen, T., Levin, J., Hillmer, A., Giese, A., and Vassallo, N. (2011). Inhibition and disaggregation of α-synuclein oligomers by natural polyphenolic compounds. FEBS Lett. 585, 1113–1120. doi: 10.1016/j.febslet.2011.03.046
Caruana, M., Neuner, J., Högen, T., Schmidt, F., Kamp, F., Scerri, C., et al. (2012). Polyphenolic compounds are novel protective agents against lipid membrane damage by α-synuclein aggregates in vitro. Biochim. Biophys. Acta 1818, 2502–2510. doi: 10.1016/j.bbamem.2012.05.019
Caruana, M., and Vassallo, N. (2015). Tea polyphenols in Parkinson's disease. Adv. Exp. Med. Biol. 863, 117–137. doi: 10.1007/978-3-319-18365-7_6
Celej, M. S., Caarls, W., Demchenko, A. P., and Jovin, T. M. (2009). A triple-emission fluorescent probe reveals distinctive amyloid fibrillar polymorphism of wild-type alpha-synuclein and its familial Parkinson's disease mutants. Biochemistry 48, 7465–7472. doi: 10.1021/bi9003843
Ceravolo, R., Rossi, C., Del Prete, E., and Bonuccelli, U. (2016). A review of adverse events linked to dopamine agonists in the treatment of Parkinson's disease. Expert Opin. Drug Saf. 15, 181–198. doi: 10.1517/14740338.2016.1130128
Chanoux, R. A., and Rubenstein, R. C. (2012). Molecular chaperones as targets to circumvent the cftr defect in cystic fibrosis. Front. Pharmacol. 3:137. doi: 10.3389/fphar.2012.00137
Chen, L. L., Song, J. X., Lu, J. H., Yuan, Z. W., Liu, L. F., Durairajan, S. S., et al. (2014). Corynoxine, a natural autophagy enhancer, promotes the clearance of alpha-synuclein via Akt/mTOR pathway. J. Neuroimmune Pharmacol. 9, 380–387. doi: 10.1007/s11481-014-9528-2
Chen, M., Wang, T., Yue, F., Li, X., Wang, P., Li, Y., et al. (2015b). Tea polyphenols alleviate motor impairments, dopaminergic neuronal injury, and cerebral α-synuclein aggregation in MPTP-intoxicated parkinsonian monkeys. Neuroscience 286, 383–392. doi: 10.1016/j.neuroscience.2014.12.003
Chen, X. C., Zhou, Y. C., Chen, Y., Zhu, Y. G., Fang, F., and Chen, L. M. (2005). Ginsenosdie Rg1 reduces MPTP-induced substantia nigra neuron loss by suppressing oxidative stress. Yao Xue Xue Bao 26, 56–62. doi: 10.1111/j.1745-7254.2005.00019.x
Chen, Y. M., Liu, S. P., Lin, H. L., Chan, M. C., Chen, Y. C., Huang, Y. L., et al. (2015a). Irisflorentin improves α-synuclein accumulation and attenuates 6-OHDA-induced dopaminergic neuron degeneration, implication for Parkinson's disease therapy. Biomedicine 5:4. doi: 10.7603/s40681-015-0004-y
Cheng, X. R., Wallace, G. Q., Lagugné-Labarthet, F., and Kerman, K. (2015). Au nanostructured surfaces for electrochemical and localized surface plasmon resonance-based monitoring of α-synuclein-small molecule interactions. ACS Appl. Mater. Interfaces 7, 4081–4088. doi: 10.1021/am507972b
Cheon, S. M., Jang, I., Lee, M. H., Kim, D. K., Jeon, H., and Cha, D. S. (2017). Sorbus alnifolia protects dopaminergic neurodegeneration in Caenorhabditis elegans. Pharm. Biol. 55, 481–486. doi: 10.1080/13880209.2016.1251468
Choubey, S., Goyal, S., Varughese, L. R., Kumar, V., Sharma, A. K., and Beniwal, V (2018). Probing gallic acid for its broad spectrum applications. Mini Rev. Med. Chem. 18, 1283–1293. doi: 10.2174/1389557518666180330114010
Coelho, T., Maia, L. F., da Silva, A. M., Cruz, M. W., Plante-Bordeneuve, V., Suhr, O. B, et al. (2013). Long-term effects of tafamidis for the treatment of transthyretin familial amyloid polyneuropathy. J. Neurol. 260, 2802–2814. doi: 10.1007/s00415-013-7051-7
Coelho-Cerqueira, E., Pinheiro, A. S., and Follmer, C. (2014). Pitfalls associated with the use of Thioflavin-T to monitor anti-fibrillogenic activity. Bioorg. Med. Chem. Lett. 24, 3194–3198. doi: 10.1016/j.bmcl.2014.04.072
Collier, T. J., Kanaan, N. M., and Kordower, J. H. (2017). Aging and Parkinson's disease: different sides of the same coin? Mov. Disord. 32, 983–990. doi: 10.1002/mds.27037
Cox, D., Selig, E., Griffin, M. D., Carver, J. A., and Ecroyd, H. (2016). Small heat-shock proteins prevent α-synuclein aggregation via transient interactions and their efficacy is affected by the rate of aggregation. J. Biol. Chem. 291, 22618–22629. doi: 10.1074/jbc.M116.739250
Cui, Y., Rong, C., Wang, J., Cui, C., Wang, L., Feng, Z., et al. (2013). Mechanism-based anti-anxiety effects of polysaccharides extracted from shudihuang (radix rehmanniae preparata) by two-dimensional electrophoresis analysis in rat hippocampus proteins. J. Tradit. Chin. Med. 33, 524–530. doi: 10.1016/S0254-6272(13)60159-4
da Costa, I. M., Cavalcanti, J. R. L. P., de Queiroz, D. B., de Azevedo, E. P., do Rêgo, A. C. M., Araújo Filho, I., et al. (2017). Supplementation with herbal extracts to promote behavioral and neuroprotective effects in experimental models of Parkinson's disease: a systematic review. Phytother. Res. 31, 959–970. doi: 10.1002/ptr.5813
da Silva, F. L., Coelho Cerqueira, E., de Freitas, M. S., Gonçalves, D. L., Costa, L. T., and Follmer, C. (2013). Vitamins K interact with N-terminus, α-synuclein and modulate the protein fibrillization in vitro. Exploring the interaction between quinones, and α-synuclein. Neurochem. Int. 62, 103–112. doi: 10.1016/j.neuint.2012.10.001
Das, S., Pukala, T.L., and Smid, S.D. (2018). Exploring the structural diversity in inhibitors of α-synuclein amyloidogenic folding, aggregation, and neurotoxicity. Front. Chem. 6:181. doi: 10.3389/fchem.2018.00181
Deng, Y. N., Shi, J., Liu, J., and Qu, Q. M. (2013). Celastrol protects human neuroblastoma SH-SY5Y cells from rotenone-induced injury through induction of autophagy. Neurochem. Int. 63, 1–9. doi: 10.1016/j.neuint.2013.04.005
Dimant, H., Ebrahimi-Fakhari, D., and McLean, P. J. (2012). Molecular chaperones and co-chaperones in Parkinson disease. Neuroscientist 18, 589–601. doi: 10.1177/1073858412441372
Dinda, B., Debnath, S., Mohanta, B. C., and Harigaya, Y. (2010). Naturally occurring triterpenoid saponins. Chem. Biodivers. 7, 2327–2580. doi: 10.1002/cbdv.200800070
Ehrnhoefer, D. E., Bieschke, J., Boeddrich, A., Herbst, M., Masino, L., Lurz, R., et al. (2008). EGCG redirects amyloidogenic polypeptides into unstructured, off-pathway oligomers. Nat. Struct. Mol. Biol. 15, 558–566. doi: 10.1038/nsmb.1437
Ellis, J. M., and Fell, M. J. (2017). Current approaches to the treatment of Parkinson's disease. Bioorg. Med. Chem. Lett. 27, 4247–4255. doi: 10.1016/j.bmcl.2017.07.075
Eschbach, J., von Einem, B., Müller, K., Bayer, H., Scheffold, A., Morrison, B. E., et al. (2015). Mutual exacerbation of peroxisome proliferator-activated receptor γ coactivator 1α deregulation and α-synuclein oligomerization. Ann. Neurol. 77, 15–32. doi: 10.1002/ana.24294
Fazili, N. A., and Naeem, A. (2015). Anti-fibrillation potency of caffeic acid against an antidepressant induced fibrillogenesis of human α-synuclein: implications for Parkinson's disease. Biochimie 108, 178–185. doi: 10.1016/j.biochi.2014.11.011
Friesen, E. L., De Snoo, M. L., Rajendran, L., Kalia, L. V., and Kalia, S. K. (2017). Chaperone-based therapies for disease modification in Parkinson's disease. Parkinsons Dis. 2017:5015307. doi: 10.1155/2017/5015307
Fu, G., Zhang, W., Du, D., Ng, Y. P., Ip, F. C. F., Tong, R., et al. (2017). Diarylheptanoids from rhizomes of Alpinia officinarum inhibit aggregation of α-synuclein. J. Agric. Food Chem. 65, 6608–6614. doi: 10.1021/acs.jafc.7b02021
Fu, R. H., Harn, H. J, Liu, S. P., Chen, C. S., Chang, W. L., Chen, Y. M., et al. (2014b). n-butylidenephthalide protects against dopaminergic neuron degeneration and α-synuclein accumulation in Caenorhabditis elegans models of Parkinson's disease. PLoS ONE 9:e85305. doi: 10.1371/journal.pone.0085305
Fu, R. H., Wang, Y. C., Chen, C. S., Tsai, R. T., Liu, S. P., Chang, W. L., et al. (2014a). Acetylcorynoline attenuates dopaminergic neuron degeneration and α-synuclein aggregation in animal models of Parkinson's disease. Neuropharmacology 82, 108–120. doi: 10.1016/j.neuropharm.2013.08.007
Gadad, B. S., Subramanya, P. K., Pullabhatla, S., Shantharam, I. S., and Rao, K. S. (2012). Curcumin-glucoside, a novel synthetic derivative of curcumin, inhibits α-synuclein oligomer formation: relevance to Parkinson's disease. Curr. Pharm. Des. 18, 76–84. doi: 10.2174/138161212798919093
Gasiorowski, K., Lamer-Zarawska, E., Leszek, J., Parvathaneni, K., Yendluri, B. B., Błach-Olszewska, Z., et al. (2011). Flavones from root of Scutellaria baicalensis Georgi: drugs of the future in neurodegeneration? CNS Neurol. Disord. Drug Targets 10, 184–191. doi: 10.2174/187152711794480384
Gautam, S., Karmakar, S., Batra, R., Sharma, P., Pradhan, P., Singh, J., et al. (2017). Polyphenols in combination with β-cyclodextrin can inhibit and disaggregate α-synuclein amyloids under cell mimicking conditions: a promising therapeutic alternative. Biochim. Biophys. Acta 1865, 589–603. doi: 10.1016/j.bbapap.2017.02.014
Gautam, S., Karmakar, S., Bose, A., and Chowdhury, P. K. (2014). β-cyclodextrin and curcumin, a potent cocktail for disaggregating and/or inhibiting amyloids: a case study with α-synuclein. Biochemistry 53, 4081–4083. doi: 10.1021/bi500642f
Gelders, G., Baekelandt, V., and Van der Perren, A. (2018). Linking neuroinflammation and neurodegeneration in Parkinson's disease. J. Immunol. Res. 2018:4784268. doi: 10.1155/2018/4784268
Ghavami, S., Shojaei, S., Yeganeh, B., Ande, S. R., Jangamreddy, J. R., Mehrpour, M., et al. (2014). Autophagy and apoptosis dysfunction in neurodegenerative disorders. Prog. Neurobiol. 112, 24–49. doi: 10.1016/j.pneurobio.2013.10.004
Ghiglieri, V., Calabrese, V., and Calabresi, P. (2018). Alpha-synuclein: from early synaptic dysfunction to neurodegeneration. Front. Neurol. 9:295. doi: 10.3389/fneur.2018.00295
Giehm, L., Lorenzen, N., and Otzen, D. E. (2011). Assays for α-synuclein aggregation. Methods 53, 295–305. doi: 10.1016/j.ymeth.2010.12.008
Giffin, J. C., Richards, R. C., Craft, C., Jahan, N., Leggiadro, C., Chopin, T., et al. (2017). An extract of the marine alga Alaria esculenta modulates α-synuclein folding and amyloid formation. Neurosci. Lett. 644, 87–93. doi: 10.1016/j.neulet.2017.02.055
Grelle, G., Otto, A., Lorenz, M., Frank, R. F., Wanker, E. E., and Bieschke, J. (2011). Black tea theaflavins inhibit formation of toxic amyloid-β and α-synuclein fibrils. Biochemistry 50, 10624–10636. doi: 10.1021/bi2012383
Griffioen, G., Duhamel, H., Van Damme, N., Pellens, K., Zabrocki, P., Pannecouque, C., et al. (2006). A yeast-based model of alpha-synucleinopathy identifies compounds with therapeutic potential. Biochim. Biophys. Acta. 1762, 312–318. doi: 10.1016/j.bbadis.2005.11.009
Guo, Y. J., Dong, S. Y., Cui, X. X., Feng, Y., Liu, T., Yin, M., et al. (2016). Resveratrol alleviates MPTP-induced motor impairments and pathological changes by autophagic degradation of α-synuclein via SIRT1-deacetylated LC3. Mol. Nutr. Food Res. 60, 2161–2175. doi: 10.1002/mnfr.201600111
Ham, A., Kim, D. W., Kim, K. H., Lee, S. J., Oh, K. B., Shin, J., et al. (2013). Reynosin protects against neuronal toxicity in dopamine-induced SH-SY5Y cells and 6-hydroxydopamine-lesioned rats as models of Parkinson's disease: reciprocal up-regulation of E6-AP and down-regulation of α-synuclein. Brain Res. 1524, 54–61. doi: 10.1016/j.brainres.2013.05.036
Ham, A., Lee, H. J., Hong, S. S., Lee, D., and Mar, W. (2012b). Moracenin D from Mori Cortex radicis protects SH-SY5Y cells against dopamine-induced cell death by regulating nurr1 and α-synuclein expression. Phytother. Res. 26, 620–624. doi: 10.1002/ptr.3592
Ham, A., Lee, S. J., Shin, J., Kim, K. H., and Mar, W. (2012a). Regulatory effects of costunolide on dopamine metabolism-associated genes inhibit dopamine-induced apoptosis in human dopaminergic SH-SY5Y cells. Neurosci. Lett. 507, 101–105. doi: 10.1016/j.neulet.2011.10.037
Hansen, C., and Li, J. Y. (2012). Beyond α-synuclein transfer: pathology propagation in Parkinson's disease. Trends Mol. Med. 18, 248–255. doi: 10.1016/j.molmed.2012.03.002
Hao, X. M., Li, L. D., Duan, C. L., and Li, Y. J. (2017). Neuroprotective effect of α-mangostin on mitochondrial dysfunction and α-synuclein aggregation in rotenone-induced model of Parkinson's disease in differentiated SH-SY5Y cells. J. Asian Nat. Prod. Res. 8, 833–845. doi: 10.1080/10286020.2017.1339349
Heng, Y., Zhang, Q. S., Mu, Z., Hu, J. F., Yuan, Y. H., and Chen, N. H. (2016). Ginsenoside Rg1 attenuates motor impairment and neuroinflammation in the MPTP-probenecid-induced parkinsonism mouse model by targeting α-synuclein abnormalities in the substantia nigra. Toxicol. Lett. 243, 7–21. doi: 10.1016/j.toxlet.2015.12.005
Henrich, C. J., and Beutler, J. A. (2013). Matching the power of high throughput screening to the chemical diversity of natural products. Nat. Prod. Rep. 30, 1284–1298. doi: 10.1039/c3np70052f
Herrera, A., Muñoz, P., Steinbusch, H. W. M., and Segura-Aguilar, J. (2017). Are dopamine oxidation metabolites involved in the loss of dopaminergic neurons in the nigrostriatal system in Parkinson's disease? ACS Chem. Neurosci. 8, 702–711. doi: 10.1021/acschemneuro.7b00034
Ho, L., and Pasinetti, G. M. (2010). Polyphenolic compounds for treating neurodegenerative disorders involving protein misfolding. Expert Rev. Proteomics 7, 579–589. doi: 10.1586/epr.10.69
Hong, D. P., Fink, A. L., and Uversky, V. N. (2008). Structural characteristics of alpha-synuclein oligomers stabilized by the flavonoid baicalein. J. Mol. Biol. 383, 214–223. doi: 10.1016/j.jmb.2008.08.039
Hu, Q., Uversky, V. N., Huang, M., Kang, H., Xu, F., Liu, X., et al. (2016). Baicalein inhibits α-synuclein oligomer formation and prevents progression of α-synuclein accumulation in a rotenone mouse model of Parkinson's disease. Biochim. Biophys. Acta 1862, 1883–1890. doi: 10.1016/j.bbadis.2016.07.008
Huang, J. Z., Chen, Y. Z., Su, M., Zheng, H. F., Yang, Y. P., Chen, J., et al. (2010). dl-3-n-Butylphthalide prevents oxidative damage and reduces mitochondrial dysfunction in an MPP(+)-induced cellular model of Parkinson's disease. Neurosci. Lett. 475, 89–94. doi: 10.1016/j.neulet.2010.03.053
Hung, K. C., Huang, H. J., Wang, Y. T., and Lin, A. M. (2016). Baicalein attenuates α-synuclein aggregation, inflammasome activation and autophagy in the MPP(+)-treated nigrostriatal dopaminergic system in vivo. J. Ethnopharmacol. 194, 522–529. doi: 10.1016/j.jep.2016.10.040
Hussain, G., Rasul, A., Anwar, H., Aziz, N., Razzaq, A., Wei, W., et al. (2018). Role of plant derived alkaloids and their mechanism in neurodegenerative disorders. Int. J. Biol. Sci. 14, 341–357. doi: 10.7150/ijbs.23247
Inoue, E., Shimizu, Y., Masui, R., Hayakawa, T., Tsubonoya, T., Hori, S., et al. (2018). Effects of saffron and its constituents, crocin-1, crocin-2, and crocetin on α-synuclein fibrils. J. Nat. Med. 72, 274–279. doi: 10.1007/s11418-017-1150-1
Jadiya, P., Khan, A., Sammi, S. R., Kaur, S., Mir, S. S., Nazira, A., et al. (2011). Anti-Parkinsonian effects of Bacopa monnieri: insights from transgenic and pharmacological Caenorhabditis elegans models of Parkinson's disease. Biochem. Biophys. Res. Commun. 413, 605–610. doi: 10.1016/j.bbrc.2011.09.010
Jankovic, J., Goodman, I., Safirstein, B., Marmon, T. K., Schenk, D. B., Koller, M., et al. (2018). Safety and tolerability of multiple ascending doses of PRX002/RG7935, an anti-α-synuclein monoclonal antibody, in patients with parkinson disease: a randomized clinical trial. JAMA Neurol. 75, 1206–1214. doi: 10.1001/jamaneurol.2018.1487
Javed, H., Kamal, M. A., and Ojha, S. (2016). An overview on the role of α-synuclein in experimental models of Parkinson's disease from pathogenesis to therapeutics. CNS Neurol. Disord. Drug Targets 15, 1240–1252. doi: 10.2174/1871527315666160920160512
Jha, N. N., Ghosh, D., Das, S., Anoop, A., Jacob, R. S., Singh, P. K., et al. (2016). Effect of curcumin analogs on α-synuclein aggregation and cytotoxicity. Sci. Rep. 6:28511. doi: 10.1038/srep28511
Jha, N. N., Kumar, R., Panigrahi, R., Navalkar, A., Ghosh, D., Sahay, S., et al. (2017). Comparison of α-synuclein fibril inhibition by four different amyloid inhibitors. ACS Chem. Neurosci. 20, 2722–2733. doi: 10.1021/acschemneuro.7b00261
Ji, H. F., and Shen, L. (2014). The multiple pharmaceutical potential of curcumin in Parkinson's disease. CNS Neurol. Disord. Drug Targets 13, 369–373. doi: 10.2174/18715273113129990077
Ji, K., Zhao, Y., Yu, T., Wang, Z., Gong, H., Yang, X., et al. (2016). Inhibition effects of tanshinone on the aggregation of α-synuclein. Food Funct. 7, 409–416. doi: 10.1039/C5FO00664C
Jiang, M., Porat-Shliom, Y., Pei, Z., Cheng, Y., Xiang, L., and Sommers, K. (2010). Baicalein reduces E46K alpha-synuclein aggregation in vitro and protects cells against E46K alpha-synuclein toxicity in cell models of familiar Parkinsonism. J. Neurochem. 114, 419–429. doi: 10.1111/j.1471-4159.2010.06752.x
Jiang, T. F., Zhang, Y. J., Zhou, H. Y., Wang, H. M., Tian, L. P, and Liu, J. (2013). Curcumin ameliorates the neurodegenerative pathology in A53T α-synuclein cell model of Parkinson's disease through the downregulation of mTOR/p70S6K signaling and the recovery of macroautophagy. J. Neuroimmune Pharmacol. 8, 356–369. doi: 10.1007/s11481-012-9431-7
Kabiraj, P., Marin, J. E., Varela-Ramirez, A., Zubia, E., and Narayan, M. (2014). Ellagic acid mitigates SNO-PDI induced aggregation of Parkinsonian biomarkers. ACS Chem. Neurosci. 5, 1209–1220. doi: 10.1021/cn500214k
Kalia, L. V., Kalia, S. K., and Lang, A. E. (2015). Disease-modifying strategies for Parkinson's disease. Mov. Disord. 30, 1442–1450. doi: 10.1002/mds.26354
Karpinar, D. P., Balija, M. B., Kügler, S., Opazo, F., Rezaei-Ghaleh, N., Wender, N., et al. (2009). Pre-fibrillar α-synuclein variants with impaired β-structure increase neurotoxicity in Parkinson's disease models. EMBO J. 28, 3256–3268. doi: 10.1038/emboj.2009.257
Kastenholz, B., and Garfin, D. E. (2009). Medicinal plants: a natural chaperones source for treating neurological disorders. Protein Pept. Lett. 16, 116–120. doi: 10.2174/092986609787316234
Kim, D. S., Kim, J. Y., and Han, Y. (2012). Curcuminoids in neurodegenerative diseases. Recent Pat. CNS Drug Discov. 7, 184–204. doi: 10.2174/157488912803252032
Kim, J., Sasaki, Y., Yoshida, W., Kobayashi, N., Veloso, A. J., Kerman, K., et al. (2013). Rapid cytotoxicity screening platform for amyloid inhibitors using a membrane-potential sensitive fluorescent probe. Anal. Chem. 85, 185–192. doi: 10.1021/ac302442q
Ko, W. K. D., and Bezard, E. (2017). Experimental animal models of Parkinson's disease: a transition from assessing symptomatology to α-synuclein targeted disease modification. Exp. Neurol. 298, 172–179. doi: 10.1016/j.expneurol.2017.07.020
Koo, U., Nam, K. W., Ham, A., Lyu, D., Kim, B., Lee, S. J., et al. (2011). Neuroprotective effects of 3α-acetoxyeudesma-1,4(15),11(13)-trien-12,6α-olide against dopamine-induced apoptosis in the human neuroblastoma SH-SY5Y cell line. Neurochem. Res. 36, 1991–2001. doi: 10.1007/s11064-011-0523-1
Kostka, M., Högen, T., Danzer, K. M., Levin, J., Habeck, M., Wirth, A., et al. (2008). Single particle characterization of iron-induced pore-forming alpha-synuclein oligomers. J. Biol. Chem. 83, 10992–11003. doi: 10.1074/jbc.M709634200
Kosuru, R. Y., Roy, A., Das, S. K., and Bera, S. (2018). Gallic acid and gallates in human health and disease: do mitochondria hold the key to success? Mol. Nutr. Food Res. 62. doi: 10.1002/mnfr.201700699
Kumar, S., Okello, E. J., and Harris, J. R. (2012). Experimental inhibition of fibrillogenesis and neurotoxicity by amyloid-beta (Aβ) and other disease-related peptides/proteins by plant extracts and herbal compounds. Subcell. Biochem. 65, 295–326. doi: 10.1007/978-94-007-5416-4_13
Kundu, P., Das, M., Tripathy, K., and Sahoo, S. K. (2016). Delivery of dual drug loaded lipid based nanoparticles across the blood-brain barrier imparts enhanced neuroprotection in a rotenone induced mouse model of Parkinson's disease. ACS Chem. Neurosci. 7, 1658–1670. doi: 10.1021/acschemneuro.6b00207
Kuzuhara, S., Mori, H., Izumiyama, N., Yoshimura, M., and Ihara, Y. (1988). Lewy bodies are ubiquitinated. A light and electron microscopic immunocytochemical study. Acta Neuropathol. 75, 345–353. doi: 10.1007/BF00687787
Lashuel, H. A., Overk, C. R., Oueslati, A., and Masliah, E. (2013). The many faces of α-synuclein: from structure and toxicity to therapeutic target. Nat. Rev. Neurosci. 14, 38–48. doi: 10.1038/nrn3406
Lauterbach, E. C., Victoroff, J., Coburn, K. L., Shillcutt, S. D., Doonan, S. M., and Mendez, M. F. (2010). Psychopharmacological neuroprotection in neurodegenerative disease: assessing the preclinical data. J. Neuropsychiatry Clin. Neurosci. 22, 8–18. doi: 10.1176/jnp.2010.22.1.8
Lázaro, D. F., Pavlou, M. A. S., and Outeiro, T. F. (2017). Cellular models as tools for the study of the role of alpha-synuclein in Parkinson's disease. Exp. Neurol. 298, 162–171. doi: 10.1016/j.expneurol.2017.05.007
Lee, D. H., Kim, C. S., and Lee, Y. J. (2011). Astaxanthin protects against MPTP/MPP+-induced mitochondrial dysfunction and ROS production in vivo and in vitro. Food Chem. Toxicol. 49, 271–280. doi: 10.1016/j.fct.2010.10.029
Lee, J. H., Lee, I. H., Choe, Y. J., Kang, S., Kim, H. Y., Gai, W. P., et al. (2009). Real-time analysis of amyloid fibril formation of alpha-synuclein using a fibrillation-state-specific fluorescent probe of JC-1. Biochem. J. 418, 311–323. doi: 10.1042/BJ20081572
Lee, K. W., Im, J. Y., Woo, J. M., Grosso, H., Kim, Y. S., Cristovao, A. C., et al. (2013). Neuroprotective and anti-inflammatory properties of a coffee component in the MPTP model of Parkinson's disease. Neurotherapeutics 10, 143–153. doi: 10.1007/s13311-012-0165-2
Lester, H. A., Xiao, C., Srinivasan, R., Son, C. D., Miwa, J., Pantoja, R., et al. (2009). Nicotine is a selective pharmacological chaperone of acetylcholine receptor number and stoichiometry. Implications for drug discovery. AAPS J. 11, 167–177. doi: 10.1208/s12248-009-9090-7
Li, T., Feng, Y., Yang, R., Wu, L., Li, R., Huang, L., et al. (2018). Salidroside promotes the pathological α-synuclein clearance through ubiquitin-proteasome system in SH-SY5Y cells. Front. Pharmacol. 9:377. doi: 10.3389/fphar.2018.00377
Li, X., Zhang, G., Nie, Q., Wu, T., Jiao, L., Zheng, M., et al. (2017). Baicalein blocks α-synuclein secretion from SN4741 cells and facilitates α-synuclein polymerization to big complex. Neurosci. Lett. 655, 109–114. doi: 10.1016/j.neulet.2017.06.031
Li, X. Z., Zhang, S. N., Wang, K. X., Liu, H. Y., Yang, Z. M., Liu, S. M., et al. (2014). Neuroprotective effects of extract of Acanthopanax senticosus harms on SH-SY5Y cells overexpressing wild-type or A53T mutant α-synuclein. Phytomedicine 21, 704–711. doi: 10.1016/j.phymed.2013.10.012
Lin, A. M., Ping, Y. H., Chang, G. F., Wang, J. Y., Chiu, J. H., Kuo, C. D., et al. (2011). Neuroprotective effect of oral S/B remedy (Scutellaria baicalensis Georgi and Bupleurum scorzonerifolfium Willd) on iron-induced neurodegeneration in the nigrostriatal dopaminergic system of rat brain. J. Ethnopharmacol. 134, 884–891. doi: 10.1016/j.jep.2011.01.056
Liu, C. B., Wang, R., Pan, H. B., Ding, Q. F., and Lu, F. B. (2013). Effect of lycopene on oxidative stress and behavioral deficits in rotenone induced model of Parkinson's disease. Zhongguo Ying Yong Sheng Li Xue Za Zhi. 29, 380–384.
Liu, L. F., Song, J. X., Lu, J. H., Huang, Y. Y., Zeng, Y., Chen, L. L., et al. (2015). Tianma Gouteng Yin, a Traditional Chinese Medicine decoction, exerts neuroprotective effects in animal and cellular models of Parkinson's disease. Sci. Rep. 5:16862. doi: 10.1038/srep16862
Liu, X., Zhou, S., Shi, D., Bai, Q., Liu, H., and Yao, X. (2018). Influence of EGCG on α-synuclein (αS) aggregation and identification of their possible binding mode: a computational study using molecular dynamics simulation. Chem. Biol. Drug Des. 91, 162–171. doi: 10.1111/cbdd.13067
Liu, Y., Carver, J. A., Calabrese, A. N., and Pukala, T. L. (2014). Gallic acid interacts with α-synuclein to prevent the structural collapse necessary for its aggregation. Biochim. Biophys. Acta 1844, 1481–1485. doi: 10.1016/j.bbapap.2014.04.013
Liu, Z., Yu, Y., Li, X., Ross, C. A., and Smith, W. W. (2011). Curcumin protects against A53T alpha-synuclein-induced toxicity in a PC12 inducible cell model for Parkinsonism. Pharmacol. Res. 63, 439–444. doi: 10.1016/j.phrs.2011.01.004
Lobbens, E. S., Breydo, L., Skamris, T., Vestergaard, B., Jäger, A. K., Jorgensen, L., et al. (2016). Mechanistic study of the inhibitory activity of Geum urbanum extract against α-synuclein fibrillation. Biochim. Biophys. Acta 1864, 1160–1169. doi: 10.1016/j.bbapap.2016.06.009
Long, J., Gao, H., Sun, L., Liu, J., and Zhao-Wilson, X. (2009). Grape extract protects mitochondria from oxidative damage and improves locomotor dysfunction and extends lifespan in a Drosophila Parkinson's disease model. Rejuvenation Res. 12, 321–331. doi: 10.1089/rej.2009.0877
Longhena, F., Faustini, G., Missale, C., Pizzi, M., Spano, P., and Bellucci, A. (2017). The contribution of α-synuclein spreading to Parkinson's disease synaptopathy. Neural Plast. 2017:5012129. doi: 10.1155/2017/5012129
Loo, T. W., and Clarke, D. M. (2007). Chemical and pharmacological chaperones as new therapeutic agents. Expert Rev. Mol. Med. 9, 1–18. doi: 10.1017/S1462399407000361
Lorenzen, N., Nielsen, S. B., Yoshimura, Y., Vad, B. S., Andersen, C. B., Betzer, C., et al. (2014). How epigallocatechin gallate can inhibit α-synuclein oligomer toxicity in vitro. J. Biol. Chem. 289, 21299–212310. doi: 10.1074/jbc.M114.554667
Lu, J. H., Ardah, M. T., Durairajan, S. S., Liu, L. F., Xie, L. X., Fong, W. F., et al. (2011). Baicalein inhibits formation of α-synuclein oligomers within living cells and prevents Aβ peptide fibrillation and oligomerisation. Chembiochem 12, 615–624. doi: 10.1002/cbic.201000604
Lu, J. H., Tan, J. Q., Durairajan, S. S., Liu, L. F., Zhang, Z. H., Ma, L., et al. (2012). Isorhynchophylline, a natural alkaloid, promotes the degradation of alpha-synuclein in neuronal cells via inducing autophagy. Autophagy 8, 98–108. doi: 10.4161/auto.8.1.18313
Luk, K. C., Hyde, E. G., Trojanowski, J. Q., and Lee, V. M. (2007). Sensitive fluorescence polarization technique for rapid screening of alpha-synuclein oligomerization/fibrillization inhibitors. Biochemistry 46, 12522–12529. doi: 10.1021/bi701128c
Luo, F. C., Wang, S. D., Qi, L., Song, J. Y., Lv, T., and Bai, J. (2011). Protective effect of panaxatriol saponins extracted from Panax notoginseng against MPTP-induced neurotoxicity in vivo. J. Ethnopharmacol. 133, 448–453. doi: 10.1016/j.jep.2010.10.017
Ma, L., Cao, T. T., Kandpal, G., Warren, L., Fred Hess, J., Seabrook, G. R., et al. (2010). Genome-wide microarray analysis of the differential neuroprotective effects of antioxidants in neuroblastoma cells overexpressing the familial Parkinson's disease alpha-synuclein A53T mutation. Neurochem. Res. 35, 130–142. doi: 10.1007/s11064-009-0038-1
Macedo, D., Tavares, L., McDougall, G. J., Vicente Miranda, H., Stewart, D., Ferreira, R. B., et al. (2015). (Poly)phenols protect from α-synuclein toxicity by reducing oxidative stress and promoting autophagy. Hum. Mol. Genet. 24, 1717–1732. doi: 10.1093/hmg/ddu585
Maioli, E., Torricelli, C., and Valacchi, G. (2012). Rottlerin and curcumin: a comparative analysis. Ann. N. Y. Acad. Sci. 1259, 65–76. doi: 10.1111/j.1749-6632.2012.06514.x
Mandel, S., Weinreb, O., Amit, T., and Youdim, M. B. (2004). Cell signaling pathways in the neuroprotective actions of the green tea polyphenol (-)-epigallocatechin-3-gallate: implications for neurodegenerative diseases. J. Neurochem. 88, 1555–1569. doi: 10.1046/j.1471-4159.2003.02291.x
Marchiani, A., Mammi, S., Siligardi, G., Hussain, R., Tessari, I., Bubacco, L., et al. (2013). Small molecules interacting with α-synuclein: antiaggregating and cytoprotective properties. Amino Acids 45, 327–338. doi: 10.1007/s00726-013-1503-3
Masuda, M., Suzuki, N., Taniguchi, S., Oikawa, T., Nonaka, T., Iwatsubo, T., et al. (2006). Small molecule inhibitors of α-synuclein filament assembly. Biochemistry 45, 6085–6094. doi: 10.1021/bi0600749
Mazo, N. A., Echeverria, V., Cabezas, R., Avila-Rodriguez, M., Tarasov, V. V., Yarla, N. S., et al. (2017). Medicinal plants as protective strategies against Parkinson's disease. Curr. Pharm. Des. 23, 4180–4188. doi: 10.2174/1381612823666170316142803
Meng, F., Abedini, A., Plesner, A., Verchere, C. B., and Raleigh, D. P. (2010). The flavanol (-)-epigallocatechin 3-gallate inhibits amyloid formation by islet amyloid polypeptide, disaggregates amyloid fibrils, and protects cultured cells against IAPP-induced toxicity. Biochemistry 49, 8127–8133. doi: 10.1021/bi100939a
Meng, X., Munishkina, L. A., Fink, A. L., and Uversky, V. N. (2009). Molecular mechanisms underlying the flavonoid-induced inhibition of alpha-synuclein fibrillation. Biochemistry 48, 8206–8224. doi: 10.1021/bi900506b
Mohanan, P., Subramaniyam, S., Mathiyalagan, R., and Yang, D. C. (2018). Molecular signaling of ginsenosides Rb1, Rg1, and Rg3 and their mode of actions. J. Ginseng Res. 42, 123–132. doi: 10.1016/j.jgr.2017.01.008
Molino, S., Dossena, M., Buonocore, D., Ferrari, F., Venturini, L., Ricevuti, G., et al. (2016). Polyphenols in dementia: From molecular basis to clinical trials. Life Sci. 61, 69–77. doi: 10.1016/j.lfs.2016.07.021
Moore, D. J., West, A. B., Dawson, V. L., and Dawson, T. M. (2005). Molecular pathophysiology of Parkinson's disease. Annu. Rev. Neurosci. 28, 57–87. doi: 10.1146/annurev.neuro.28.061604.135718
Moree, B., Yin, G., Lázaro, D. F., Munari, F., Strohäker, T., Giller, K., et al. (2015). Small molecules detected by second-harmonic generation modulate the conformation of monomeric α-synuclein and reduce its aggregation in cells. J. Biol. Chem. 290, 27582–27593. doi: 10.1074/jbc.M114.636027
Morgan, L. A., and Grundmann, O. (2017). Preclinical and potential applications of common western herbal supplements as complementary treatment in Parkinson's disease. J. Diet. Suppl. 14, 453–466. doi: 10.1080/19390211.2016.1263710
Morshedi, D., Aliakbari, F., Tayaranian-Marvian, A., Fassihi, A., Pan-Montojo, F., and Pérez-Sánchez, H. (2015). Cuminaldehyde as the major component of Cuminum cyminum, a natural aldehyde with inhibitory effect on alpha-synuclein fibrillation and cytotoxicity. J. Food Sci. 80, H2336–2345. doi: 10.1111/1750-3841.13016
Morshedi, D., and Nasouti, M. (2016). Essential oils may lead α-synuclein towards toxic fibrils formation. Parkinsons. Dis. 2016:6219249. doi: 10.1155/2016/6219249
Noorwez, S. M., Kuksa, V., Imanishi, Y., Zhu, L., Filipek, S., Palczewski, K., et al. (2003). Pharmacological chaperone-mediated in vivo folding and stabilization of the p23h-opsin mutant associated with autosomal dominant retinitis pigmentosa. J. Biol. Chem. 278, 14442–14450. doi: 10.1074/jbc.M300087200
Ono, K., and Yamada, M. (2006). Antioxidant compounds have potent anti-fibrillogenic and fibril-destabilizing effects for alpha-synuclein fibrils in vitro. J. Neurochem. 97, 105–115. doi: 10.1111/j.1471-4159.2006.03707.x
Ortiz-Ortiz, M. A., Morán, J. M., Ruiz-Mesa, L. M., Niso-Santano, M., Bravo-SanPedro, J. M., Gómez-Sánchez, R., et al. (2010). Curcumin exposure induces expression of the Parkinson's disease-associated leucine-rich repeat kinase 2 (LRRK2) in rat mesencephalic cells. Neurosci. Lett. 468, 120–124. doi: 10.1016/j.neulet.2009.10.081
Pandareesh, M. D., Mythri, R. B., and Srinivas Bharath, M. M. (2015). Bioavailability of dietary polyphenols: Factors contributing to their clinical application in CNS diseases. Neurochem. Int. 89, 198–208. doi: 10.1016/j.neuint.2015.07.003
Pandey, N., Strider, J., Nolan, W. C., Yan, S. X., and Galvin, J. E. (2008). Curcumin inhibits aggregation of alpha-synuclein. Acta Neuropathol. 115, 479–489. doi: 10.1007/s00401-007-0332-4
Patel, M. Y., Panchal, H. V., Ghribi, O., and Benzeroual, K. E. (2012). The neuroprotective effect of fisetin in the MPTP model of Parkinson's disease. J. Parkinsons Dis. 2, 287–302. doi: 10.3233/JPD-012110
Pervin, M., Unno, K., Ohishi, T., Tanabe, H., Miyoshi, N., and Nakamura, Y. (2018). Beneficial effects of green tea catechins on neurodegenerative diseases. Molecules. 23:E1297. doi: 10.3390/molecules23061297
Pogacnik, L., Pirc, K., Palmela, I., Skrt, M., Kim, K. S., Brites, D., et al. (2016). Potential for brain accessibility and analysis of stability of selected flavonoids in relation to neuroprotection in vitro. Brain Res. 1651, 17–26. doi: 10.1016/j.brainres.2016.09.020
Pujols, J., Peña-Díaz, S., Conde-Giménez, M., Pinheiro, F., Navarro, S., Sancho, J., et al. (2017). High-throughput screening methodology to identify alpha-synuclein aggregation inhibitors. Int. J. Mol. Sci. 18:E478. doi: 10.3390/ijms18030478
Rekha, K. R., Selvakumar, G. P., Santha, K., and Inmozhi Sivakamasundari, R. (2013). Geraniol attenuates α-synuclein expression and neuromuscular impairment through increase dopamine content in MPTP intoxicated mice by dose dependent manner. Biochem. Biophys. Res. Commun. 440, 664–670. doi: 10.1016/j.bbrc.2013.09.122
Ren, R., Shi, C., Cao, J., Sun, Y., Zhao, X., Guo, Y., et al. (2016). Neuroprotective effects of a standardized flavonoid extract of safflower against neurotoxin-induced cellular and animal models of Parkinson's disease. Sci. Rep. 6:22135. doi: 10.1038/srep22135
Reyes, J. F., Olsson, T. T., Lamberts, J. T., Devine, M. J., Kunath, T., and Brundin, P. (2015). A cell culture model for monitoring α-synuclein cell-to-cell transfer. Neurobiol. Dis. 77, 266–275. doi: 10.1016/j.nbd.2014.07.003
Ringe, D., and Petsko, G. A. (2009). What are pharmacological chaperones and why are they interesting? J. Biol. 8:80. doi: 10.1186/jbiol186
Rogers, J. T., Mikkilineni, S., Cantuti-Castelvetri, I., Smith, D. H., Huang, X., Bandyopadhyay, S., et al. (2011). The alpha-synuclein 5′untranslated region targeted translation blockers: anti-alpha synucleinefficacy of cardiac glycosides and Posiphen. J. Neural Transm. (Vienna) 118, 493–507. doi: 10.1007/s00702-010-0513-5
Ruipérez, V., Darios, F., and Davletov, B. (2010). Alpha-synuclein, lipids and Parkinson's disease. Prog. Lipid Res. 49, 420–428. doi: 10.1016/j.plipres.2010.05.004
Sarrafchi, A., Bahmani, M., Shirzad, H., and Rafieian-Kopaei, M. (2016). Oxidative stress and Parkinson's disease: new hopes in treatment with herbal antioxidants. Curr. Pharm. Des. 22, 238–246. doi: 10.2174/1381612822666151112151653
Sashourpour, M., Zahri, S., Radjabian, T., Ruf, V., Pan-Montojo, F., and Morshedi, D. (2017). A study on the modulation of alpha-synuclein fibrillation by Scutellaria pinnatifida extracts and its neuroprotective properties. PLoS ONE 12:e0184483. doi: 10.1371/journal.pone.0184483
Sawkar, A. R., Cheng, W. C., Beutler, E., Wong, C. H., Balch, W. E., and Kelly, J. W. (2002). Chemical chaperones increase the cellular activity of n370s beta-glucosidase: a therapeutic strategy for gaucher disease. Proc. Natl. Acad. Sci. U.S.A. 99, 15428–15433. doi: 10.1073/pnas.192582899
Schenk, D. B., Koller, M., Ness, D. K., Griffith, S. G., Grundman, M., Zago, W., et al. (2017). First-in-human assessment of PRX002, an anti-α-synuclein monoclonal antibody, in healthy volunteers. Mov. Disord. 32, 211–218. doi: 10.1002/mds.26878
Scholz, S., and Williamson, G. (2007). Interactions affecting the bioavailability of dietary polyphenols in vivo. Int. J. Vitam. Nutr. Res. 77, 224–235. doi: 10.1024/0300-9831.77.3.224
Sekowski, S., Ionov, M., Abdulladjanova, N., Makhmudov, R., Mavlyanov, S., Milowska, K., et al. (2017). Interaction of α-synuclein with Rhus typhina tannin–implication for Parkinson's disease. Colloids Surf. B Biointerfaces 155, 159–165. doi: 10.1016/j.colsurfb.2017.04.007
Shaltiel-Karyo, R., Davidi, D., Frenkel-Pinter, M., Ovadia, M., Segal, D., and Gazit, E. (2012). Differential inhibition of α-synuclein oligomeric and fibrillar assembly in parkinson's disease model by cinnamon extract. Biochim. Biophys. Acta 1820, 1628–1635. doi: 10.1016/j.bbagen.2012.04.021
Sharma, N., Kapoor, M., and Nehru, B. (2016). Apocyanin, NADPH oxidase inhibitor prevents lipopolysaccharide induced α-synucleinaggregation and ameliorates motor function deficits in rats: possible role of biochemical and inflammatory alterations. Behav. Brain Res. 296, 177–190. doi: 10.1016/j.bbr.2015.09.012
Sharma, N., and Nehru, B. (2018). Curcumin affords neuroprotection and inhibits α-synuclein aggregation in lipopolysaccharide-induced Parkinson's disease model. Inflammopharmacology 26, 349–360. doi: 10.1007/s10787-017-0402-8
Shashikumar, S., Pradeep, H., Chinnu, S., Rajini, P. S., and Rajanikant, G. K. (2015). Alpha-linolenic acid suppresses dopaminergic neurodegeneration induced by 6-OHDA in Caenorhabditis elegans. Physiol. Behav. 151, 563–569. doi: 10.1016/j.physbeh.2015.08.025
Shen, C., Sun, F. L., Zhang, R. Y., Zhang, L., Li, Y. L., Zhang, L., et al. (2015). Tetrahydroxystilbene glucoside ameliorates memory and movement functions, protects synapses and inhibits α-synuclein aggregation in hippocampus and striatum in aged mice. Restor. Neurol. Neurosci. 33, 531–541. doi: 10.3233/RNN-150514
Shoval, H., Weiner, L., Gazit, E., Levy, M., Pinchuk, I., and Lichtenberg, D. (2008). Polyphenol-induced dissociation of various amyloid fibrils results in a methionine-independent formation of ROS. Biochim. Biophys. Acta 1784, 1570–1577. doi: 10.1016/j.bbapap.2008.08.007
Shukla, V., Phulara, S. C., Yadav, D., Tiwari, S., Kaur, S., Gupta, M. M., et al. (2012). Iridoid compound 10-O-trans-p-coumaroylcatalpol extends longevity and reduces α synucleinaggregation in Caenorhabditis elegans. CNS Neurol. Disord. Drug Targets 11, 984–992. doi: 10.2174/1871527311211080007
Siddique, Y. H., Ara, G., Jyoti, S., and Afzal, M. (2012). The dietary supplementation of nordihydroguaiaretic acid (NDGA) delayed the loss of climbing ability in Drosophila model of Parkinson's disease. J. Diet. Suppl. 9, 1–8. doi: 10.3109/19390211.2011.630716
Siddique, Y. H., Jyoti, S., and Naz, F (2014). Effect of epicatechin gallate dietary supplementation on transgenic Drosophila model of Parkinson's disease. J. Diet. Suppl. 11, 121–130. doi: 10.3109/19390211.2013.859207
Siddique, Y. H., Khan, W., Singh, B. R., and Naqvi, A. H. (2013b). Synthesis of alginate-curcumin nanocomposite and its protective role in transgenic Drosophila model of Parkinson's disease. ISRN Pharmacol. 2013:794582. doi: 10.1155/2013/794582
Siddique, Y. H., Mujtaba, S. F., Jyoti, S., and Naz, F (2013a). GC-MS analysis of Eucalyptus citriodora leaf extract and its role on the dietary supplementation in transgenic Drosophila model of Parkinson's disease. Food Chem Toxicol. 55, 29–35. doi: 10.1016/j.fct.2012.12.028
Simon-Sanchez, J., Schulte, C., Bras, J. M., Sharma, M., Gibbs, J. R., Berg, D., et al. (2009). Genome-wide association study reveals genetic risk underlying Parkinson's disease. Nat. Genet. 41, 1308–1312. doi: 10.1038/ng.487
Singh, P. K., Kotia, V., Ghosh, D., Mohite, G. M., Kumar, A., and Maji, S. K. (2013). Curcumin modulates α-synuclein aggregation and toxicity. ACS Chem. Neurosci. 4, 393–407. doi: 10.1021/cn3001203
Singleton, A. B., Farrer, M., Johnson, J., Singleton, A., Hague, S., Kachergus, J., et al. (2003). α-Synuclein locus triplication causes Parkinson's disease. Science 302:841. doi: 10.1126/science.1090278
Sivanesam, K., and Andersen, N. H. (2016). Modulating the amyloidogenesis of α-synuclein. Curr. Neuropharmacol. 14, 226–237. doi: 10.2174/1570159X13666151030103153
Skibinski, G., and Finkbeiner, S. (2011). Drug discovery in Parkinson's disease–update and developments in the use of cellular models. Int. J. High Throughput Screen. 2011, 15–25. doi: 10.2147/IJHTS.S8681
Smita, S. S., Raj Sammi, S., Laxman, T. S., Bhatta, R. S., and Pandey, R. (2017). Shatavarin IV elicits lifespan extension and alleviates Parkinsonism in Caenorhabditis elegans. Free Radic. Res. 51, 954–969. doi: 10.1080/10715762.2017.1395419
Šneideris, T., Baranauskiene, L., Cannon, J.G., Rutkiene, R., Meškys, R., and Smirnovas, V. (2015). Looking for a generic inhibitor of amyloid-like fibril formation among flavone derivatives. Peer J. 3:e1271. doi: 10.7717/peerj.1271
Spillantini, M. G., Crowther, R. A., Jakes, R., Hasegawa, M., and Goedert, M. (1998). Alpha-synuclein in filamentous inclusions of Lewy bodies from Parkinson's disease and dementia with lewy bodies. Proc. Natl. Acad. Sci. U.S.A. 95, 6469–6473. doi: 10.1073/pnas.95.11.6469
Spillantini, M. G., Schmidt, M. L., Lee, V. M., Trojanowski, J. Q., Jakes, R., Goedert, M., et al. (1997). Alpha-synuclein in Lewy bodies. Nature 388, 839–840. doi: 10.1038/42166
Spinelli, K. J., Osterberg, V. R., Meshul, C. K., Soumyanath, A., and Unni, V. K. (2015). Curcumin treatment improves motor behavior in α-synuclein transgenic mice. PLoS ONE 10:e0128510. doi: 10.1371/journal.pone.0128510
Srinivasan, R., Henderson, B. J., Lester, H. A., and Richards, C. I. (2014). Pharmacological chaperoning of nAChRs: a therapeutic target for Parkinson's disease. Pharmacol. Res. 83, 20–29. doi: 10.1016/j.phrs.2014.02.005
Sun, A., Xu, X., Lin, J., Cui, X., and Xu, R. (2015). Neuroprotection by saponins. Phytother. Res. 29,187–200. doi: 10.1002/ptr.5246
Sun, X., Cao, Y. B., Hu, L. F., Yang, Y. P., Li, J., Wang, F., et al. (2011). ASICs mediate the modulatory effect by paeoniflorin on α-synuclein autophagic degradation. Brain Res. 1396,77–87. doi: 10.1016/j.brainres.2011.04.011
Tachibana, H., Koga, K., Fujimura, Y., and Yamada, K. (2004). A receptor for green tea polyphenol EGCG. Nat. Struct. Mol. Biol. 11, 380–381. doi: 10.1038/nsmb743
Taebnia, N., Morshedi, D., Yaghmaei, S., Aliakbari, F., Rahimi, F., and Arpanaei, A. (2016). Curcumin-loaded amine-functionalized mesoporous silica nanoparticles inhibit α-synuclein fibrillation and reduce its cytotoxicity-associated effects. Langmuir 32, 13394–13402. doi: 10.1021/acs.langmuir.6b02935
Takahashi, R., Ono, K., Takamura, Y., Mizuguchi, M., Ikeda, T., Nishijo, H., et al. (2015). Phenolic compounds prevent the oligomerization of α-synuclein and reduce synaptic toxicity. J. Neurochem. 134, 943–955. doi: 10.1111/jnc.13180
Tamarappoo, B. K., and Verkman, A. S. (1998). Defective aquaporin-2 trafficking in nephrogenic diabetes insipidus and correction by chemical chaperones. J. Clin. Invest. 101, 2257–2567. doi: 10.1172/JCI2303
Tanji, K., Miki, Y., Maruyama, A., Mimura, J., Matsumiya, T., Mori, F., et al. (2015). Trehalose intake induces chaperone molecules along with autophagy in a mouse model of Lewy body disease. Biochem. Biophys. Res. Commun. 465, 746–752. doi: 10.1016/j.bbrc.2015.08.076
Tavassoly, O., Kakish, J., Nokhrin, S., Dmitriev, O., and Lee, J. S. (2014). The use of nanopore analysis for discovering drugs which bind to α-synuclein for treatment of Parkinson's disease. Eur. J. Med. Chem. 88, 42–54. doi: 10.1016/j.ejmech.2014.07.090
Temsamani, H., Krisa, S., Decossas-Mendoza, M., Lambert, O., Mérillon, J.M., and Richard, T. (2016). Piceatannol and other wine stilbenes: a pool of inhibitors against α-synuclein aggregation and cytotoxicity. Nutrients 8:E367. doi: 10.3390/nu8060367
Teraoka, M., Nakaso, K., Kusumoto, C., Katano, S., Tajima, N., Yamashita, A., et al. (2012). Cytoprotective effect of chlorogenic acid against α-synuclein-related toxicity in catecholaminergic PC12 cells. J. Clin. Biochem. Nutr. 51, 122–127. doi: 10.3164/jcbn.D-11-00030
Tompa, P. (2005). The interplay between structure and function in intrinsically unstructured proteins. FEBS Lett. 579, 3346–3354. doi: 10.1016/j.febslet.2005.03.072
Török, N., Majláth, Z., Szalárdy, L., and Vécsei, L. (2016). Investigational α-synuclein aggregation inhibitors: hope for Parkinson's disease. Expert Opin. Investig. Drugs 25, 1281–1294. doi: 10.1080/13543784.2016.1237501
Ur Rasheed, M. S., Tripathi, M. K., Mishra, A. K., Shukla, S., and Singh, M. P. (2016). Resveratrol protects from toxin-induced Parkinsonism: a plethora of proofs hitherto petty translational value. Mol. Neurobiol. 53, 2751–2760. doi: 10.1007/s12035-015-9124-3
Uversky, V. N., and Dunker, A. K. (2010). Understanding protein non-folding. Biochim. Biophys. Acta 1804, 1231–1264. doi: 10.1016/j.bbapap.2010.01.017
Van Kampen, J., Robertson, H. A., Hagg, T., and Drobitch, R. (2003). Neuroprotective actions of the ginseng extract G115 in two rodent models of Parkinson's disease. Exp. Neurol. 184, 521–529. doi: 10.1016/j.expneurol.2003.08.002
Van Kampen, J. M., Baranowski, D. B., Shaw, C. A., and Kay, D. G. (2014). Panax ginseng is neuroprotective in a novel progressive model of Parkinson's disease. Exp. Gerontol. 50, 95–105. doi: 10.1016/j.exger.2013.11.012
Velander, P., Wu, L., Henderson, F., Zhang, S., Bevan, D. R., and Xu, B. (2017). Natural product-based amyloid inhibitors. Biochem. Pharmacol. 139, 40–55. doi: 10.1016/j.bcp.2017.04.004
Visanji, N. P., Brotchie, J. M., Kalia, L. V., Koprich, J. B., Tandon, A., and Watts, J. C. (2016). α-synuclein based animal models of parkinson's disease: challenges and opportunities in a new era. Trends Neurosci. 39, 750–762. doi: 10.1016/j.tins.2016.09.003
Wagner, H., and Ulrich-Merzenich, G. (2009). Synergy research: approaching a new generation of phytopharmaceuticals. Phytomedicine 16, 97–110. doi: 10.1016/j.phymed.2008.12.018
Wakabayashi, K., Engelender, S., Yoshimoto, M., Tsuji, S., Ross, C. A., and Takahashi, H. (2000). Synphilin-1 is present in Lewy bodies in Parkinson's disease. Ann. Neurol. 47, 521–523. doi: 10.1002/1531-8249(200004)47:4<521::AID-ANA18>3.0.CO;2-B
Wang, M. S., Boddapati, S., Emadi, S., and Sierks, M. R. (2010). Curcumin reduces alpha-synuclein induced cytotoxicity in Parkinson's disease cell model. BMC Neurosci. 11:57. doi: 10.1186/1471-2202-11-57
Wang, S., He, H., Chen, L., Zhang, W., Zhang, X., and Chen, J (2015b). Protective effects of salidroside in the MPTP/MPP(+)-induced model of Parkinson's disease through ROS-NO-related mitochondrion pathway. Mol Neurobiol. 51, 718–728. doi: 10.1007/s12035-014-8755-0
Wang, X. C., Wang, X., and Li, Q. L. (2014). Effect of chaperone-mediated autophagy in MPP+ -induced SH-SY5Y cells and interventional effect of puerarin. Zhongguo Zhong Yao Za Zhi. 39, 106–112.
Wang, Z. H., Zhang, J. L., Duan, Y. L., Zhang, Q. S., Li, G. F., and Zheng, D. L. (2015a). MicroRNA-214 participates in the neuroprotective effect of resveratrol via inhibiting α-synuclein expression in MPTP-induced Parkinson's disease mouse. Biomed. Pharmacother. 74, 252–256. doi: 10.1016/j.biopha.2015.08.025
Wang, Z. Y., Liu, J. Y., Yang, C. B., Malampati, S., Huang, Y. Y., Li, M. X., et al. (2017). Neuroprotective natural products for the treatment of Parkinson's disease by targeting the autophagy-lysosome pathway: a systematic review. Phytother. Res. 31, 1119–1127. doi: 10.1002/ptr.5834
Weinreb, O., Mandel, S., Youdim, M. B. H., and Amit, T. (2013). Targeting dysregulation of brain iron homeostasis in Parkinson's disease by iron chelators. Free Radic. Biol. Med. 62, 52–64. doi: 10.1016/j.freeradbiomed.2013.01.017
Winner, B., Jappelli, R., Maji, S. K., Desplats, P. A., Boyer, L., Aigner, S., et al. (2011). In vivo demonstration that α-synuclein oligomers are toxic. Proc. Natl. Acad. Sci. U.S.A. 108, 4194–4199. doi: 10.1073/pnas.1100976108
Wong, V. K., Wu, A. G., Wang, J. R., Liu, L., and Law, B. Y. (2015). Neferine attenuates the protein level and toxicity of mutant huntingtin in PC-12 cells via induction of autophagy. Molecules 20, 3496–3514. doi: 10.3390/molecules20033496
Wu, A. G., Wong, V. K., Xu, S. W., Chan, W. K., Ng, C. I., Liu, L., et al. (2013). Onjisaponin B derived from Radix polygalae enhances autophagy and accelerates the degradation of mutant α-synuclein and huntingtin in PC-12 Cells. Int. J. Mol. Sci. 14, 22618–22641. doi: 10.3390/ijms141122618
Wu, Y., Jiang, X., Yang, K., Xia, Y., Cheng, S., Tang, Q., et al. (2017). Inhibition of α-Synuclein contributes to the ameliorative effects of dietary flavonoids luteolin on arsenite-induced apoptotic cell death in the dopaminergic PC12 cells. Toxicol. Mech. Methods 27, 598–608. doi: 10.1080/15376516.2017.1339155
Wu, Y., Li, X., Zhu, J. X., Xie, W., Le, W., and Fan, Z. (2011). Resveratrol-activated AMPK/SIRT1/autophagy in cellular models of Parkinson's disease. Neurosignals 19, 163–174. doi: 10.1159/000328516
Xu, Q., Langley, M., Kanthasamy, A. G., and Reddy, M. B. (2017). Epigallocatechin gallate has a neurorescue effect in a mouse model of Parkinson disease. J. Nutr. 147, 1926–1931. doi: 10.3945/jn.117.255034
Xu, Y., Zhang, P., Wang, C., Shan, Y., Wang, D., Qian, F., et al. (2013). Effect of ginsenoside Rg3 on tyrosine hydroxylase and related mechanisms in the forced swimming-induced fatigue rats. J. Ethnopharmacol. 150, 138–147. doi: 10.1016/j.jep.2013.08.016
Xu, Y., Zhang, Y., Quan, Z., Wong, W., Guo, J., Zhang, R., et al. (2016). Epigallocatechin gallate (EGCG) inhibits alpha-synuclein aggregation: a potential agent for Parkinson's disease. Neurochem. Res. 41, 2788–2796. doi: 10.1007/s11064-016-1995-9
Yamaguchi, Y., Masuda, M., Sasakawa, H., Nonaka, T., Hanashima, S., Hisanaga, S., et al. (2010). Characterization of inhibitor-bound alpha-synuclein dimer: role of alpha-synuclein N-terminal region in dimerization and inhibitor binding. J. Mol. Biol. 395, 445–456. doi: 10.1016/j.jmb.2009.10.068
Yang, F., Yang, Y. P., Mao, C. J., Cao, B. Y., Cai, Z. L., Shi, J. J., et al. (2009). Role of autophagy and proteasome degradation pathways in apoptosis of PC12 cells overexpressing human alpha-synuclein. Neurosci. Lett. 454, 203–218. doi: 10.1016/j.neulet.2009.03.027
Yang, J. E., Rhoo, K. Y., Lee, S., Lee, J. T., Park, J. H., Bhak, G., et al. (2017). EGCG-mediated protection of the membrane disruption and cytotoxicity caused by the ‘active oligomer’ of α-synuclein. Sci. Rep. 7:17945. doi: 10.1038/s41598-017-18349-z
Yoshida, W., Kobayashi, N., Sasaki, Y., Ikebukuro, K., and Sode, K. (2013). Partial peptide of α-synuclein modified with small-molecule inhibitors specifically inhibits amyloid fibrillation of α-synuclein. Int. J. Mol. Sci. 14, 2590–2600. doi: 10.3390/ijms14022590
Yuan, J., Ren, J., Wang, Y., He, X., and Zhao, Y. (2016). Acteoside binds to caspase-3 and exerts neuroprotection in the rotenone rat model of Parkinson's disease. PLoS ONE 11:e0162696. doi: 10.1371/journal.pone.0162696
Zhang, H., Bai, L., He, J., Zhong, L., Duan, X., Ouyang, L., et al. (2017). Recent advances in discovery and development of natural products as source for anti-Parkinson's disease lead compounds. Eur. J. Med. Chem. 141, 257–272. doi: 10.1016/j.ejmech.2017.09.068
Zhang, H., Rochet, J. C., and Stanciu, L. A. (2015). Cu(II) promotes amyloid pore formation. Biochem. Biophys. Res. Commun. 464, 342–347. doi: 10.1016/j.bbrc.2015.06.156
Zhang, L., Yu, S., Zhang, R., Xing, Y., Li, Y., and Li, L. (2013). Tetrahydroxystilbene glucoside antagonizes age-related α-synuclein overexpression in the hippocampus of APP transgenic mouse model of Alzheimer's disease. Restor. Neurol. Neurosci. 31, 41–52. doi: 10.3233/RNN-120260
Zhang, N., Yan, F., Liang, X., Wu, M., Shen, Y., Chen, M., et al. (2018). Localized delivery of curcumin into brain with polysorbate 80-modified cerasomes by ultrasound-targeted microbubble destruction for improved Parkinson's disease therapy. Theranostics 8, 2264–2277. doi: 10.7150/thno.23734
Zhao, J., Xu, L., Liang, Q., Sun, Q., Chen, C., Zhang, Y., et al. (2017). Metal chelator EGCG attenuates Fe(III)-induced conformational transition of α-synuclein and protects AS-PC12 cells against Fe(III)-induced death. J. Neurochem. 143, 136–146. doi: 10.1111/jnc.14142
Zheng, M., Xin, Y., Li, Y., Xu, F., Xi, X., Guo, H., et al. (2018). Ginsenosides: a potential neuroprotective agent. Biomed. Res. Int. 2018:8174345. doi: 10.1155/2018/8174345
Zhou, J. X., Zhang, H. B., Huang, Y., He, Y., Zheng, Y., Anderson, J. P., et al. (2013). Tenuigenin attenuates α-synuclein-induced cytotoxicity by down-regulating polo-like kinase 3. CNS Neurosci. Ther. 19, 688–694. doi: 10.1111/cns.12124
Zhu, M., Han, S., and Fink, A. L. (2013). Oxidized quercetin inhibits α-synuclein fibrillization. Biochim. Biophys. Acta 1830, 2872–2881. doi: 10.1016/j.bbagen.2012.12.027
Keywords: α-synuclein, plants, phytochemicals, Parkinson's disease, neuroprotective, natural products, neurotoxicity, bioactive agents
Citation: Javed H, Nagoor Meeran MF, Azimullah S, Adem A, Sadek B and Ojha SK (2019) Plant Extracts and Phytochemicals Targeting α-Synuclein Aggregation in Parkinson's Disease Models. Front. Pharmacol. 9:1555. doi: 10.3389/fphar.2018.01555
Received: 17 April 2018; Accepted: 20 December 2018;
Published: 19 March 2019.
Edited by:
Muhammad Ayaz, University of Malakand, PakistanReviewed by:
Carlos M. Opazo, The University of Melbourne, AustraliaBazbek Davletov, University of Sheffield, United Kingdom
Copyright © 2019 Javed, Nagoor Meeran, Azimullah, Adem, Sadek and Ojha. This is an open-access article distributed under the terms of the Creative Commons Attribution License (CC BY). The use, distribution or reproduction in other forums is permitted, provided the original author(s) and the copyright owner(s) are credited and that the original publication in this journal is cited, in accordance with accepted academic practice. No use, distribution or reproduction is permitted which does not comply with these terms.
*Correspondence: Abdu Adem, YWJkdS5hZGVtQHVhZXUuYWMuYWU=
Shreesh Kumar Ojha, c2hyZWVzaG9qaGFAdWFldS5hYy5hZQ==