- 1Departamento de Fisiología y Farmacología, Centro de Ciencias Básicas, Universidad Autónoma de Aguascalientes, Aguascalientes, Mexico
- 2School of Behavioral and Brain Sciences and Center for Advanced Pain Studies, University of Texas at Dallas, Richardson, TX, United States
- 3Departamento de Neurobiología del Desarrollo y Neurofisiología, Instituto de Neurobiología, Universidad Nacional Autónoma de México, Santiago de Querétaro, Mexico
- 4Cátedras CONACYT, Departamento de Cirugía, Centro de Ciencias Biomédicas, Universidad Autónoma de Aguascalientes, Aguascalientes, Mexico
- 5Neurobiology of Pain Laboratory, Departamento de Farmacobiología, Cinvestav, Mexico City, Mexico
Background: Marijuana extracts (cannabinoids) have been used for several millennia for pain treatment. Regarding the site of action, cannabinoids are highly promiscuous molecules, but only two cannabinoid receptors (CB1 and CB2) have been deeply studied and classified. Thus, therapeutic actions, side effects and pharmacological targets for cannabinoids have been explained based on the pharmacology of cannabinoid CB1/CB2 receptors. However, the accumulation of confusing and sometimes contradictory results suggests the existence of other cannabinoid receptors. Different orphan proteins (e.g., GPR18, GPR55, GPR119, etc.) have been proposed as putative cannabinoid receptors. According to their expression, GPR18 and GPR55 could be involved in sensory transmission and pain integration.
Methods: This article reviews select relevant information about the potential role of GPR18 and GPR55 in the pathophysiology of pain.
Results: This work summarized novel data supporting that, besides cannabinoid CB1 and CB2 receptors, GPR18 and GPR55 may be useful for pain treatment.
Conclusion: There is evidence to support an antinociceptive role for GPR18 and GPR55.
Physiology of Pain
Adaptive Function of Pain
Pain involves unpleasant sensations in response to real or potential tissue damage (Basbaum et al., 2009). Usually, pain unleashes a signal alert to prevent extensive injury by promoting defensive (passive and/or active) actions against the noxious (nociceptive) stimuli. Thus, pain is considered a protective and adaptive mechanism. However, pain may become persistent and pathological without a recognized protective or adaptive mechanism. When this happens, it affects the quality of life of patients and their social environment. Hence, pathological pain is an important medical problem causing distress and disability that requires prompt clinical investigation and treatment (Julius and Basbaum, 2001; Moffat and Rae, 2011). On the other hand, considering that tissue damage is not always the main origin of pain, cognitive perception and somatic sensation should be considered as related but different phenomena. Cognitive perception involves a psychological component frequently related with emotional experiences. Therefore, pain may be cataloged as a subjective event that requires patient awareness (Basbaum and Woolf, 1999; Julius and Basbaum, 2001; Walker and Hohmann, 2005).
Sensory System: Anatomical and Functional View
The terminal endings of primary afferent neurons whose cell bodies are located in the dorsal root ganglia (DRG) and trigeminal ganglia (TG) are responsible for the transmission of multiple peripheral stimuli (proprioceptive or nociceptive) to the central nervous system (Julius and Basbaum, 2001; Walker and Hohmann, 2005). In the case of nociceptive transmission, two main types of pseudo-unipolar nociceptive neurons are found in those ganglia: (1) non-myelinated small diameter and multimodal C-fibers, which conduct electrical impulses at low speed (∼1 m/s), sensing and transducing thermal, chemical and mechanical stimuli; and (2) thinly myelinated Aδ-fibers that show fast conduction velocity (∼5–30 m/s), sensing mechanical and thermal stimuli (Moffat and Rae, 2011). These primary afferent nociceptive fibers sense the peripheral nociceptive environment and send the nociceptive information to the spinal dorsal horn where they make a synapse with second order neurons, which convey neuronal firing to supraspinal sites where the action potentials are decoded and perceived as pain. At the peripheral level, there are several channels and receptors involved in the initiation of nociceptive transmission, such as the transient receptor potential vanilloid type 1 (TRPV1) channel, tetrodotoxin-resistant (Na+-TTXr) voltage-gated sodium (Na+) channels, purinergic P2X receptors, serotonin (5-HT3) channel receptor, and calcium (Ca2+) channels, among others.
The nociceptive signal from the peripheral nociceptive fibers is directed toward a second order neuron into the spinal cord, and then the electrical signal is conducted to the brain cortex mainly through the antero-lateral pathway tract where the signal is interpreted as a painful sensation (Snider and McMahon, 1998; Steeds, 2009; Fabbro and Crescentini, 2014). In fact, several sensorial components such as stimuli identification, location, and emotional components are codified in the cortex (Albe-Fessard et al., 1985). The diversity of peripheral and central regions and mechanisms implicated made the control of nociception and pain a complex challenge. Finally, we must keep in mind that nociceptive transmission could be endogenously modulated. For instance, the spinal cord, which is the first relay of nociceptive transmission, could be modulated by diverse neuromodulators (noradrenergic, serotonergic, opioidergic, and oxytocinergic) (for references see Mason, 2001; Vanegas and Schaible, 2004; Loyd and Murphy, 2009; Condés-Lara et al., 2015; Llorca-Torralba et al., 2016) that may diminish or increase the noxious sensation. Nevertheless, these modulatory systems exist along the noxious pathways, including the cortical station. So, the modulation of nociceptive transmission is complex and involves an array of neurotransmitters, neuromodulators and a wide variety of specific and non-specific receptors, which are dysregulated during pathological pain states (Heinricher, 2016).
Classic Treatments for Pain
Pain treatment can be categorized as pharmacologic and non-pharmacologic. In the first case, there are a variety of druggable targets in both central and peripheral nervous system commonly used for pain treatment. Analgesics are classified as: (i) non-opioid analgesics; (ii) opioid analgesics; and (iii) adjuvant analgesics (Figure 1). The most frequently non-opioid analgesics used are non-steroidal anti-inflammatory drugs (NSAIDs), such as aspirin, ibuprofen and celecoxib. The primary mechanism of action of NSAIDs is through the inhibition of the cyclooxygenase enzymes (COX) by consequently decreasing the action of prostaglandins and their sensitizing properties. Opioid-like drugs, such as morphine, ameliorate pain by modulating the cellular excitability at the supraspinal, spinal and peripheral level through activation of opioid receptors (μ-, δ-, and κ-opioid receptors). Furthermore, opioids could enhance descending inhibitory pathways and modify the sensory and affective components of pain. In the case of adjuvants, local anesthetics (e.g., lidocaine) stop the electrical impulse by blocking voltage-gated sodium (Na+) channels. Tricyclic and noradrenaline-reuptake inhibitors act by maintaining and/or augmenting the monoamine levels in descending tracts and anticonvulsants decrease the synaptic transmission affecting neuronal excitability (Basbaum and Woolf, 1999; Sinha et al., 2017).
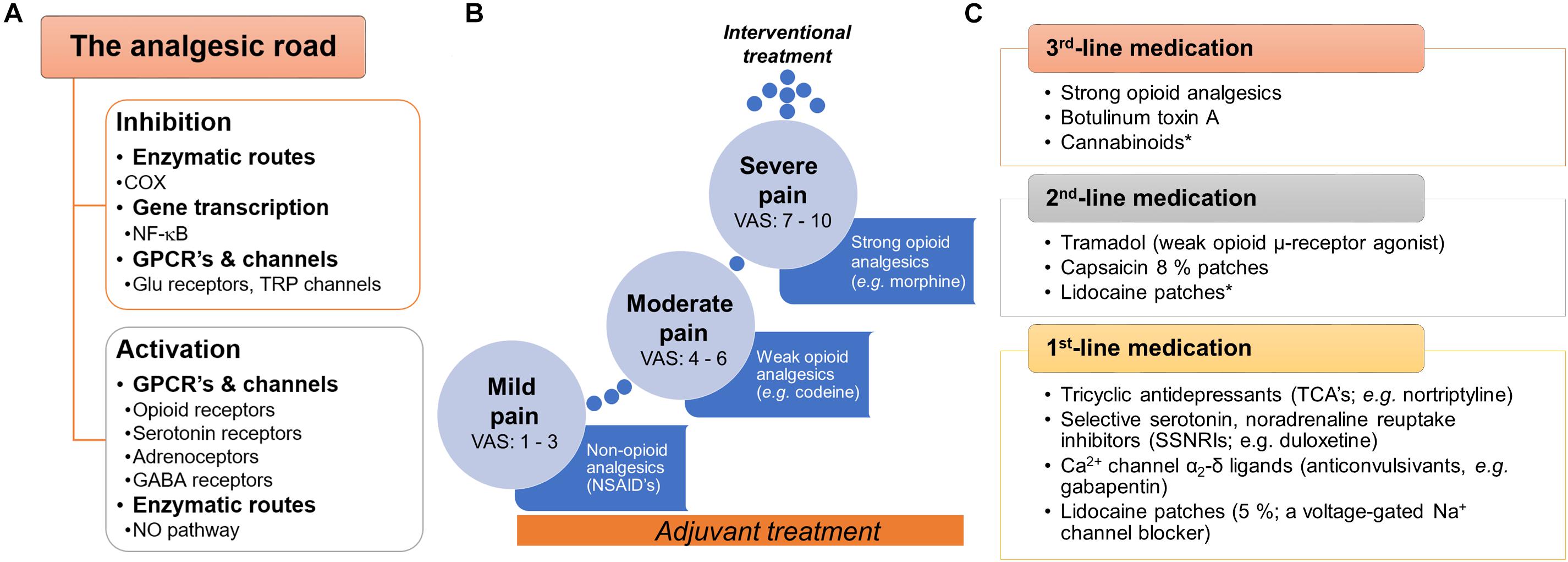
Figure 1. General view of the current pharmacotherapy and guidelines used to treat pain. (A) Snapshot of the two main pathways (inhibition or activation) by which analgesic drugs induce pain relief at peripheral, spinal and supraspinal levels. (B) To treat pain, the WHO proposed the three-step analgesic ladder. Although primarily for the management of cancer pain, it is also used as a general guideline for the management of acute and chronic non-malignant pain. A key characteristic in this approach is the use of adjuvants∗∗ with the primary drug along the pain treatment. (C) In the case of neuropathic pain, specific clinical guidelines have been proposed by several international and regional professional associations. Although several recent clinical trials support these guidelines, we need to keep in mind that several factors could limit the applicability in real-world settings (i.e., neuropathic pain is a syndrome caused by diverse etiologies and different clinical manifestations). In general terms, three-line medication has been proposed by several professional associations (including the IASP, EFNS, NICE, and CPS). In all cases, the use of strong opioids is recommended as a 3rd-line medication agent considering the potential risk for abuse, overdose, mortality or misuse. ∗No conclusive efficacy on neuropathic pain treatment. ∗∗Adjuvants or co-analgesics are drugs non-specifically designed (or marketed) to treat pain; some examples: glucocorticoids, antidepressants (some SNRIs and TCAs), α2-adrenergic agonists (e.g., clonidine) and cannabinoids (including cannabis). COX, cyclooxygenase or prostaglandin-endoperoxide synthase; CPS, Canadian Pain Society; EFNS, European Federation of Neurological Sciences; GPCRs, G protein-coupled receptors, IASP, International Association for the Study of Pain; NICE, National Institute for Health and Care Excellence (of the United Kingdom); WHO, World Health Organization.
Non-steroidal Anti-inflammatory Drugs
Non-steroidal anti-inflammatory drugs are substances that inhibit a component of the inflammatory cascade and, thence, are an important therapeutic option for non-steroid-based pain treatment. Briefly, these compounds (with exception of acetaminophen) have anti-inflammatory, antipyretic, and analgesic effects by inhibiting COX activity. At this point, we must keep in mind that the COX enzymes have at least three isoforms (COX-1, COX-2 and COX-3) and the non-selective NSAIDs act to block COX-1 and COX-2 indistinctly, favoring gastrointestinal and renal side effects (mediated by COX-1 inhibition). These side effects are particularly common in the elderly, who are most likely to experience chronic pain (Griffin et al., 1991; Buffum and Buffum, 2000; Horl, 2010). To minimize the side effects, selective COX-2 inhibitors have arrived at clinical practice. Unfortunately, several clinical trials have shown that these inhibitors also increase harmful cardiovascular effects (Bhosale et al., 2015).
Opioid-Based Treatments
Opioid analgesics act in the central nervous system and are typically prescribed to patients suffering chronic pain refractory to non-opioid treatment. Despite their well-known side effects (sedation, nausea, vomiting, constipation, pruritus and respiratory depression), opioids are widely accepted as effective for acute pain as well as cancer pain. This group of drugs have high abuse liability and are also toxic in elevated doses. For instance, from 1999 to 2014, more than 165,000 persons died of overdose related to opioids in the Unites States. In 2013, an estimated of 1.9 million people abused or were dependent on opioid pain medication (Dowell et al., 2016). Moreover, placebo-controlled trials indicate that, on average, opioids do not result in a clinically significant reduction of chronic pain symptoms (Martell et al., 2007), and even in cases where opioid analgesia is adequate for the individual patient, analgesic effects are typically not maintained during the long-term opioid pharmacotherapy due to pharmacokinetic or pharmacodynamic tolerance (Ballantyne and Shin, 2008; Dumas and Pollack, 2008). Eventually, chronic exposure to opioids results in hyperalgesia (Chu et al., 2008).
Antidepressants
Antidepressant drugs have been used as analgesics in chronic pain disorders for decades (Mico et al., 2006). Their pharmacological mechanisms have been associated with the ability to block 5-hydroxytriptamine (serotonin or 5-HT) and noradrenaline re-uptake and consequently with an increase of the activity of the endogenous analgesic system. Tricyclic antidepressants (TCAs) (e.g., amitriptyline and imipramine), tetracyclic antidepressants (TeCAs) (e.g., amoxapine, maprotiline) and the selective serotonin-norepinephrine reuptake inhibitors (SNRIs) (e.g., duloxetine and venlafaxine) are traditionally used to treat chronic pain (Mika et al., 2013). TCAs have been shown to be effective for different neuropathic pain conditions in randomized controlled trials (Finnerup et al., 2010). TCAs are generally reasonably well-tolerated but high doses are associated with a high risk of sudden cardiac death (Ray et al., 2004). The SNRIs duloxetine and venlafaxine have a well-documented efficacy in painful poly-neuropathy (Finnerup et al., 2010). SNRIs are generally well tolerated. However, the most common side-effects reported are nausea, somnolence, dizziness, constipation, anorexia, dry mouth, hyperhidrosis, and sexual dysfunction (Stahl et al., 2005).
Anticonvulsants
Gabapentin and pregabalin are anticonvulsants with therapeutic activity against neuropathic pain (Rajapakse et al., 2015). Their analgesic mechanism has been associated to their binding to the α2δ1 subunit, which in turn blocks voltage-gated calcium (Ca2+)-channels at presynaptic sites (Gee et al., 1996) or NMDA receptors at post-synaptic neurons (Chen et al., 2018; Ma et al., 2018). Both drugs are well tolerated but the most common side-effects are somnolence and dizziness, peripheral edema, weight gain, nausea, vertigo, asthenia, dry mouth, and ataxia (Quintero, 2017). Other anticonvulsants used for pain relief are carbamazepine and its analog oxcarbazepine, lamotrigine and valproate. Lamotrigine is effective for central post-stroke pain (Vestergaard et al., 2001) and diabetic neuropathy (Eisenberg et al., 2001), but has failed to relieve pain in patients with multiple sclerosis (Breuer et al., 2007) and neuropathic pain (Silver et al., 2007). Valproate also has a limited role in the treatment of neuropathic pain (Drewes et al., 1994; Otto et al., 2004; Agrawal et al., 2009).
Cannabinoids
One alternative for pain treatment came from Asia more than 3000 years ago: marijuana extracts (Li, 1974; Touw, 1981; Jensen et al., 2015). The utility of marijuana-based drugs for treating pain is explained by the existence of an ancient system of cellular control named the endocannabinoid system (ECS). Unfortunately, our knowledge about the physiology of the ECS is only partial (see below). In this review, we summarized novel data supporting that, apart from cannabinoid type-1 (CB1) and cannabinoid type-2 (CB2) receptors, some putative cannabinoid receptors (i.e., GPR18 and GPR55) may be useful for pain treatment. This should allow researchers to focus their studies on developing endocannabinoid-based options as analgesics and anti-inflammatory drugs.
Endocannabinoids and Pain
Endocannabinoid System: Generalities
Despite the ancient and well-known use of cannabis derivatives for pain management, medically recognized use of these compounds has largely subsided due to the lack of knowledge of its molecular pharmacology, its abuse for recreational purposes and additional undesirable effects, such as hypomotility and hypothermia (Crawley et al., 1993), impairments in executive function (Crean et al., 2011) and memory consolidation (Ranganathan and D’Souza, 2006). However, the identification of the major psychoactive component Δ9-tetrahydrocannabinol (Δ9-THC) (Gaoni and Mechoulam, 1964), and the subsequent isolation of cannabinoid receptors (CB1 and CB2 receptors, both G-proteins-coupled receptors linked to Gi/o proteins) with high expression levels in the nervous system, led to an explosion of studies exploring the ECS and its regulatory functions in health and disease. Briefly, the ECS consists of endogenous cannabinoids (endocannabinoids, eCBs), cannabinoid receptors, enzymes responsible for synthesis and degradation of eCBs and all genes related to them (Mackie, 2008a,b).
In this context, although several cannabinoids are available, current literature about their potential use for pain treatment remains controversial (Davis, 2014). Indeed, as reviewed by Nurmikko et al. (2007) and Martin-Sanchez et al. (2009), Δ9-THC or Δ9-THC plus cannabidiol induced relief in only one among six to nine patients (number needed to treat, NNT = 6–9). Moreover, the number needed to harm (NNH) (motor and cognitive dysfunction and altered perception) ranged between five and eight. These data suggest that, apart from its low efficacy, Δ9THC could have a narrow therapeutic index. Nevertheless, the above cannabimimetic effects seem to be mainly mediated by CB1 receptor activation, suggesting that other parts of the ECS could be druggable to treat pain. In addition, one of the physiological functions attributed to the eCBs is to suppress pain (Walker and Huang, 2002).
Endogenous Cannabinoids
The first eCB isolated in the brain was N-arachidonoyl ethanolamide (AEA), or anandamide (a name taken from the Sanskrit word Ananda, which means “bliss, joy,” and amide) (Devane et al., 1992; Figure 2). AEA is a fatty acid neuromodulator derived from the non-oxidative metabolism of arachidonic acid (AA). The second endocannabinoid identified was 2-arachidonoyl glycerol (2-AG) (Mechoulam et al., 1995; Sugiura et al., 1995). As the search for endogenous Δ9-THC-like compounds continued, other bioactive lipids were extracted from animal tissues. These include noladin ether (Hanus et al., 2001), virodhamine (Porter et al., 2002) and N-arachidonoyl dopamine (NADA) (Huang et al., 2001).
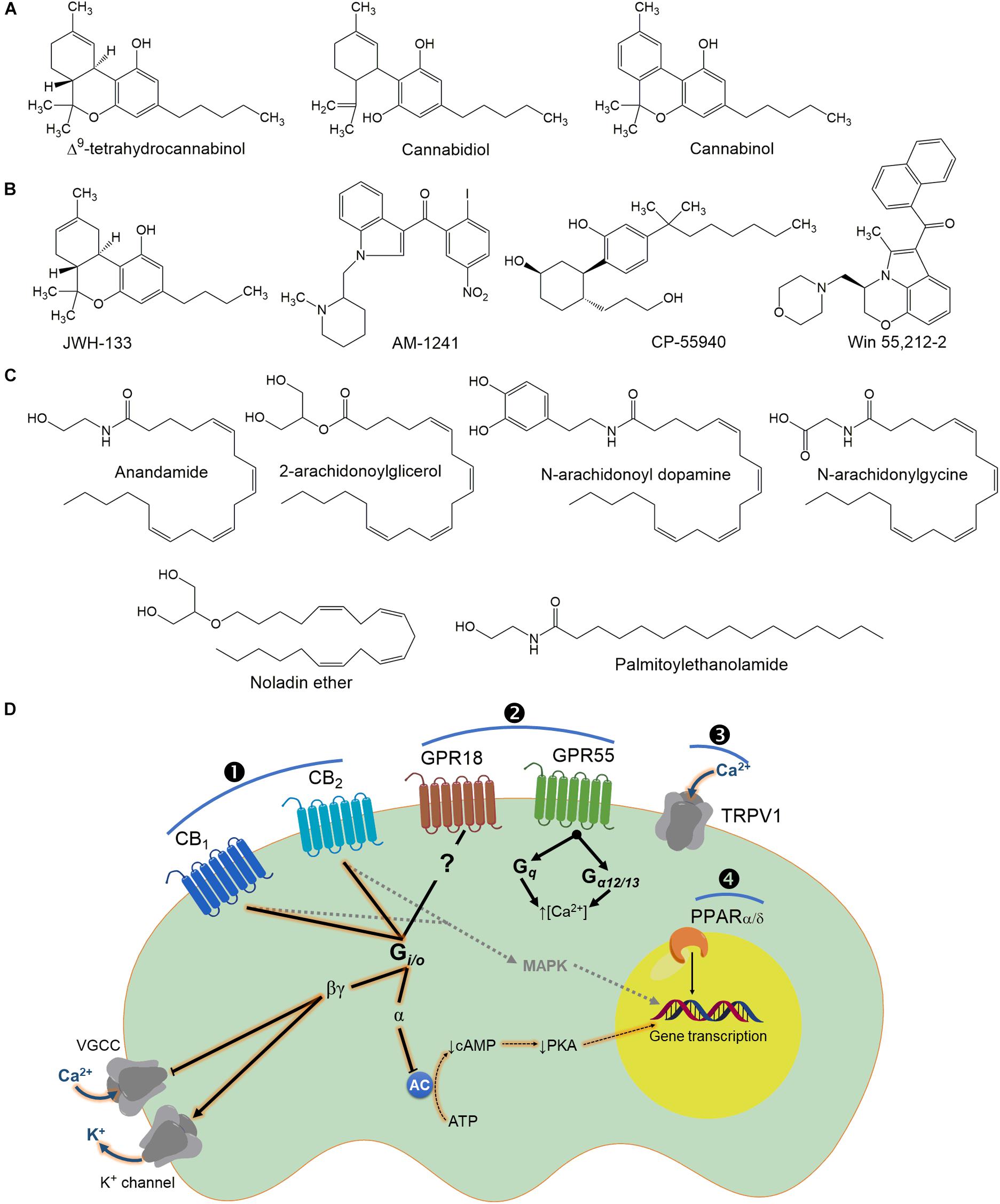
Figure 2. Chemical structures of some plant (A), synthetic cannabinoids (B) and endocannabinoids (C) that bind to cannabinoid receptors (D). It is interesting to note that cannabinoids could activate intracellular pathways by direct activation of its receptors (\protect❶ and ❷) or modulate other family receptors (\protect❸ and ❹), which contribute to the biological effect of these molecules (particularly for the endocannabinoids). In general terms, classic cannabinoid receptors (CB1 and CB2) are GPCRs, which are canonically coupled to Gi/o proteins. Consequently, under CB1/2 receptors: (i) a decrease of adenylyl cyclase (AC) activity; (ii) an inactivation of Ca2+ channels; and (iii) activation of inwardly rectifying K+ channels are achieved. These are signal transduction systems associated with inhibition of neurotransmitter release. The inhibition of AC occurs via activation of Gαi-mediated signaling whereas Gαo-activation results in inhibition of voltage-dependent Ca2+ channels (VDCCs) through the release of associated βγ subunits (apparently CB2 receptors are ineffective, compared with CB1, for shifting ionic currents via βγ subunits). In addition to PKA inhibition, CB1/2 receptor signaling also leads to the downstream activation of MAPK which can regulate nuclear transcription factors and consequently expression of several genes. Note that GPR18 seems to be coupled to Gi/o proteins, whereas GPR55 has been associated with an increase of intracellular Ca2+ via Gα12/13. In the case of TRPV1 channels (a non-selective cation channel for Ca2+, Mg2+, and Na+ ions), it is well-known that agonist can be used rationally for the treatment of pain considering that this channel under constant activation desensitizes the nociceptive neuron. Finally, although not fully investigated, cannabinoid compounds could also activate PPARα/δ, which are involved in pain modulation and transmission.
The most widely investigated eCBs are anandamide and 2-AG. Indeed, anandamide is present in about 170-fold lower levels of brain tissue than 2-AG (Stella et al., 1997), and both lipidic derivatives activate cannabinoid CB1 and CB2 receptors. Certainly, anandamide shows preferential affinity for CB1 (Ki = 89 nM) compared to CB2 (Ki = 371 nM) receptors (Gauldie et al., 2001), whereas 2-AG is considered a full agonist at both CB1 and CB2 receptors (Sugiura and Waku, 2000). Nevertheless, it has been shown that AEA could activate the vanilloid type-1 receptor (TRPV1), which contributes to the many non-CB1-mediated effects (Zygmunt et al., 1999; Smart et al., 2000). Furthermore, AEA and other eCBs (palmitoylethanolamide [PEA] and oleylethanolamide [OEA]) also are agonists of the peroxisome proliferator-activated receptor α (PPARα) (Fu et al., 2003; Bouaboula et al., 2005; Lo Verme et al., 2005). PEA also has a well-established role in pain modulation and inflammation in rodents (Jaggar et al., 1998; Calignano et al., 2001; Lo Verme et al., 2005; D’Agostino et al., 2007; González-Hernández et al., 2015), whereas in humans PEA treatment seems to relieve neuropathic pain (Calabro et al., 2010; Conigliaro et al., 2011; Gatti et al., 2012).
The eCBs are atypical neurotransmitters and/or neuromodulators. They are not stored in synaptic vesicles and are not released from presynaptic terminals via an exocytotic mechanism. In fact, their precursors exist in the cell membrane, are cleaved by specific enzymes “on demand” depending on intracellular calcium increase and are released from cells immediately after their production. The synthesis, release and deactivation of the endogenous cannabinoids are tightly regulated processes. As discussion of these processes is beyond the scope of this review, the interested reader is referred to several reviews on the topic (Howlett, 2002; Piomelli, 2003; Simon and Cravatt, 2006; Okamoto et al., 2007; Ueda et al., 2011; Luchicchi and Pistis, 2012).
Cannabinoid Receptors
To date, there are two known cannabinoid receptors that are part of the ECS, the CB1 and CB2 receptors. These receptors belong to the 7-transmembrane G-protein coupled receptors (GPCRs) primarily coupled to Gi/o proteins that inhibit adenylyl cyclase (AC) and increase mitogen-activated protein kinase (MAPK) activity downstream of β-arrestin (Howlett, 2002; Vasileiou et al., 2013). Activation of these receptors triggers the inwardly rectifying potassium (K+)-channels and A-type potassium (K+)-channel currents and inhibits N-Type and P/Q type calcium (Ca2+)-channel activity (Demuth and Molleman, 2006). The CB2 receptor is also negatively coupled to adenylyl cyclase but it seems not to be coupled to calcium (Ca2+)-channels (Felder et al., 1995). However, CB1 receptors can also interact with Gs and Gq/11 under certain conditions and with certain agonists (Mackie, 2005, 2008b). In addition, a pair of orphan-related receptors (GPR18 and GPR55) is also described as cannabinoid putative receptors.
CB1 receptor expression
The CB1 receptor is highly expressed in the cortex, cerebellum and associational cortical regions of neocortex (Glass et al., 1997). It is also expressed in the spinal dorsal horn (Sanudo-Pena et al., 1999) and in DRG neurons (Hohmann and Herkenham, 1999; Salio et al., 2002; Walker and Hohmann, 2005). Autonomic nerve terminals express CB1 receptors (Ishac et al., 1996; Vizi et al., 2001), which negatively modulate the sympathetic tone (Marichal-Cancino et al., 2013). Low levels of these receptors have been reported in the adrenal gland, thymus, heart, bone marrow, tonsils, prostrate, uterus, ovary and lung (Galiegue et al., 1995; Rice et al., 1997). A key characteristic of this receptor is the formation of heterodimers, suggesting that intracellular signaling could change under different conditions (Callen et al., 2012; Laprairie et al., 2012; Straiker et al., 2012).
CB2 receptor expression
The CB2 receptor is mostly expressed on cells of the immune system and spleen (Munro et al., 1993; Galiegue et al., 1995; Di Marzo et al., 2004). A few studies have found CB2 immunoreactivity expression in glial and neuronal cells in some areas of the rodent brain (Gong et al., 2006; Onaivi et al., 2006), but this expression remains controversial (Hohmann and Herkenham, 1999; Salio et al., 2002; Walker and Hohmann, 2005). Notably, nerve injury and inflammation upregulate expression of CB2 receptors in neurons and microglia (Beltramo et al., 2006; Rahn and Hohmann, 2009; Sagar et al., 2009; Hsieh et al., 2011). Furthermore, some studies have demonstrated the presence of CB2 receptors in the DRG and afferent fibers in the spinal dorsal horn (Ross et al., 2001; Anand et al., 2008).
Role of CB1 and CB2 Receptors on Primary Afferent Neurons
DRG neurons express CB1 receptors (Hohmann and Herkenham, 1999; Ross et al., 2001; Price et al., 2003). This receptor is synthesized in the cell neuronal bodies and inserted on both central and peripheral terminals (Hohmann and Herkenham, 1999; Hohmann et al., 1999). CB1 receptors are mainly expressed in myelinated fibers of DRG neurons (Hohmann and Herkenham, 1999; Salio et al., 2002; Bridges et al., 2003) and also co-localize with CGRP, TRPV1 and IB4 (Hohmann and Herkenham, 1999; Hohmann et al., 1999; Ahluwalia et al., 2000; Bridges et al., 2003; Veress et al., 2013).
Nerve injury enhances CB1 receptor expression in the DRG and spinal cord (Lim et al., 2003; Wang et al., 2007; Shiue et al., 2017) and other brain areas related with the emotional component of pain (Knerlich-Lukoschus et al., 2011). These data give an anatomical basis for the involvement of CB1 receptors in modulating neuropathic pain. In this regard, it has been shown that systemic and local administration of CB1 receptor agonists produce anti-nociceptive effects in neuropathic pain models (Herzberg et al., 1997; Fox et al., 2001; Bridges et al., 2003; Yu et al., 2010). Moreover, deletion of CB1 receptors in peripheral (but not at spinal or supraspinal level) nociceptors reduced analgesia by local or systemic (but no intrathecal) CB1 receptor agonists (Agarwal et al., 2007). Thus, CB1 receptors located at primary afferent neurons constitute the prime target for producing cannabinoid analgesia.
Some of the peripheral antinociceptive effects of cannabinoids may occur through interaction with another receptor system. In this regard, an early work in rat nodose ganglion neurons showed that cannabinoid agonists inhibited 5-HT-induced currents in a concentration-dependent manner. The inward current was sensitive to the serotonin (5-HT3) receptor antagonist MDL72222, suggesting a cannabinoid-mediated inhibition of serotonin (5-HT3) currents (Fan, 1995). Later, in vivo experiments demonstrated that application of CB1 and CB2 receptor agonists attenuated the activity of rat peripheral (5-HT3) receptors on the terminals of cardiopulmonary afferent C-fibers (Godlewski et al., 2003) through an allosteric interaction at a (5-HT3) modulatory site (Barann et al., 2002). Moreover, the inhibitory effects of cannabinoids may occur through a synergistic action with opioid receptors and their signal transduction pathways (Pugh et al., 1996; Smith et al., 1998; Manzanares et al., 1999; Massi et al., 2003; Scavone et al., 2013) or by a cannabinoid-mediated increase in opioid peptide synthesis and release of endogenous opioids such as enkephalins and dynorphins (Corchero et al., 1997a,b; Valverde et al., 2001).
The use of cannabinoid agonists as analgesic drugs is limited due to adverse effects in the CNS (Clermont-Gnamien et al., 2002; Attal et al., 2004; Turcotte et al., 2010). However, since it has been demonstrated that CB1 receptors are expressed at primary afferent neurons (Agarwal et al., 2007), the synthesis of CB1 receptor agonists with limited CNS penetration is under development (Clapper et al., 2010; Yu et al., 2010).
The molecular mechanisms by which the CB1 receptor has peripheral antinociceptive effects are not completely understood. It is known that CB1 receptor, coupled to Gi/o protein, can modulate several cellular mechanisms, all of which can reduce the excitability of neurons (e.g., opening of inward rectifying potassium (K+)-channels and A-type potassium (K+)-channels, and inhibiting N-Type and P/Q type calcium (Ca2+)-channels) (Demuth and Molleman, 2006). Moreover, there are several studies showing that cannabinoids can modulate the activity of transient receptor potential (TRP) channels, which are implicated in the modulation of pain processing. For example, multiple studies have shown that activation of the CB1 receptor suppresses capsaicin-induced hyperalgesia in afferent neurons (Ko and Woods, 1999; Li et al., 1999; Johanek et al., 2001; Millns et al., 2001; Johanek and Simone, 2004; Santha et al., 2010). However, there are controversial findings regarding the effects of CB1 receptor agonists on TRPV1 channels, because the CB1 receptor agonist anandamide exerts dual effects on afferent neurons, depending on the concentration used (Ross, 2003; Evans et al., 2004; Sousa-Valente et al., 2014). Specifically, anandamide produces a CB1-mediated inhibitory effect at nM concentration, while it exerts a TRPV1-mediated stimulatory effect at higher concentrations (μM) in primary afferent neurons (Tognetto et al., 2001; Roberts et al., 2002; Ross, 2003; Fischbach et al., 2007). A recent study using mouse afferent neurons has shown that activation of CB1 receptors inhibit nerve growth factor (NGF)-induced sensitization of TRPV1 (Wang et al., 2014), possibly through multiple signaling pathways, including ERK1/2 and PI3K (Zhuang et al., 2004; Stein et al., 2006; Zhu and Oxford, 2007).
The analgesic action of cannabinoids may be mediated by the presynaptic inhibition of neurotransmitter release in sensory neurons. For example, presynaptic CB1 receptors inhibit CGRP and substance P (SP) release from trigeminal sensory nerves (Akerman et al., 2004; Oshita et al., 2005). Moreover, CB1 receptor agonists reduce voltage-activated Ca2+ current in DRG neurons (Ross et al., 2001). On the other hand, it is possible that even more important than peripheral actions, cannabinoids induce analgesia by interfering with circuitry in the rostral ventromedial medulla (RVM) (Meng et al., 1998).
CB2 receptors have also been found in nociceptive sensory neurons of rodents (Ross et al., 2001; Merriam et al., 2008; Schuelert et al., 2010) and humans (Anand et al., 2008). Like with CB1 receptors, nerve damage upregulates CB2 receptors in the superficial laminae of the dorsal horn of the spinal cord and isolated DRG of mice (Wotherspoon et al., 2005) and human beings (Anand et al., 2008).
Although the specific role of the CB2 receptor in sensory neurons remains unclear, several functional studies in sensory neurons point to an antinociceptive role (Burston and Woodhams, 2014). For instance, the putative CB2 receptor agonist JWH-133 inhibits capsaicin-induced depolarization of the vagus sensory nerve in guinea pigs and humans (Patel et al., 2003). Moreover, JWH-133 reduces the response of wide dynamic range dorsal horn neurons to both innocuous and noxious intensities of mechanical stimuli (Elmes et al., 2004). This compound also attenuates the capsaicin-evoked Ca2+ response in DRG neurons in neuropathic rats (Sagar et al., 2009), while GW818646X (other CB2 receptor agonist) diminishes capsaicin-induced inward cation currents and elevation of cytoplasmic Ca2+ (Anand et al., 2008). Another CB2 receptor agonist, A-836339, inhibits von Frey-evoked activity of WDR neurons in neuropathic rats (McGaraughty et al., 2009). Local peripheral injection of the selective CB2 receptor agonist AM1241 into the hind paw produces antinociception to thermal stimulation (Malan et al., 2001). AM1241 also inhibits bradykinin-induced mesenteric afferent nerve activity (Hillsley et al., 2007). This effect was absent in CB2 knock-out mice and blocked by AM630, a CB2 receptor inverse agonist. Local injection of the PEA analog N-(4-methoxy-2-nitrophenyl)hexadecanamide induces CB1- and CB2-dependent antinociception in rats (Roa-Coria et al., 2012). Similar results were observed with GW833972A, another putative CB2 receptor agonist (Belvisi et al., 2008). Interestingly, repeated systemic administration of the CB2 receptor selective agonist AM1710 suppresses paclitaxel-induced allodynia (Deng et al., 2015). Taken together, the data strongly suggest that CB1 and CB2 receptors have an antinociceptive role. Despite this evidence, there are few cannabinoid-based drugs currently available for clinical use (see below).
CB1 and CB2-Based Treatment for Pain
A randomized, placebo-controlled, double-blinded crossover design was used to examine the effect of cannabinoids on pain. Low, medium, and high doses of smoked cannabis (respectively 2, 4, and 8% Δ9-THC by weight) did not modify capsaicin-induced pain assessed in 15 healthy volunteers 5 min after exposure (Wallace et al., 2007). In contrast, the medium dose of Δ9-THC diminished capsaicin-induced pain 45 min after cannabis exposure. Of note, these authors found that a high dose of cannabis increased capsaicin-induced pain (Wallace et al., 2007). Similar results have been reported with a high dose of nabilone (an oral synthetic cannabinoid Δ9-THC analog) on 41 patients with postoperative pain (Beaulieu, 2006). Another study evaluated cannabis extract capsules (20 mg of Δ9-THC) in 18 healthy female volunteers (Kraft et al., 2008). Treatment with Δ9-THC was not able to reduce pain induced by capsaicin, electrical stimulation or sunburn. Taken together, it seems that Δ9-THC is not effective for acute pain. A similar conclusion was reached after analyzing a total of 611 patients in seven well-designed studies (Stevens and Higgins, 2017).
Although the effects of cannabinoids in the acute pain setting seem to be disappointing, results of clinical trials evaluating cannabinoids in chronic pain are much more promising (see Table 1). The conditions causing chronic pain varied between studies and included neuropathy (chemotherapy, diabetes, human immunodeficiency virus [HIV]), cancer, fibromyalgia, multiple sclerosis and rheumatoid arthritis (Whiting et al., 2015). Sativex (containing Δ9-THC:cannabidiol [CBD] in an approximate 1:1 ratio [oral spray]) reduced neuropathic pain in patients with unilateral neuropathic pain (Berman et al., 2004; Nurmikko et al., 2007; Langford et al., 2013; Serpell et al., 2014). Likewise, treatment with smoked cannabis diminished pain in patients with multiple sclerosis (Rog et al., 2005; Corey-Bloom et al., 2012), neuropathic pain (Wilsey et al., 2013) and diabetic neuropathy (Wallace et al., 2015). In contrast, sativex was ineffective in relieving chemotherapy-induced neuropathic pain (Lynch et al., 2014). Oral administration of dronabinol, a synthetic Δ9-THC analog, modestly reduced central pain in patients with multiple sclerosis (Svendsen et al., 2004). Nabilone, another synthetic Δ9-THC analog, diminished neuropathic pain in diabetic patients (Toth et al., 2012). Oral administration of Δ9-THC (ECP002A) reduced pain in patients with progressive multiple sclerosis. Drug dosage was well tolerated and had a stable pharmacokinetic profile (van Amerongen et al., 2017). Nabilone is also effective in patients with medication overuse headache (Pini et al., 2012). In contrast, nabilone did not reduce pain in patients with fibromyalgia (Skrabek et al., 2008).
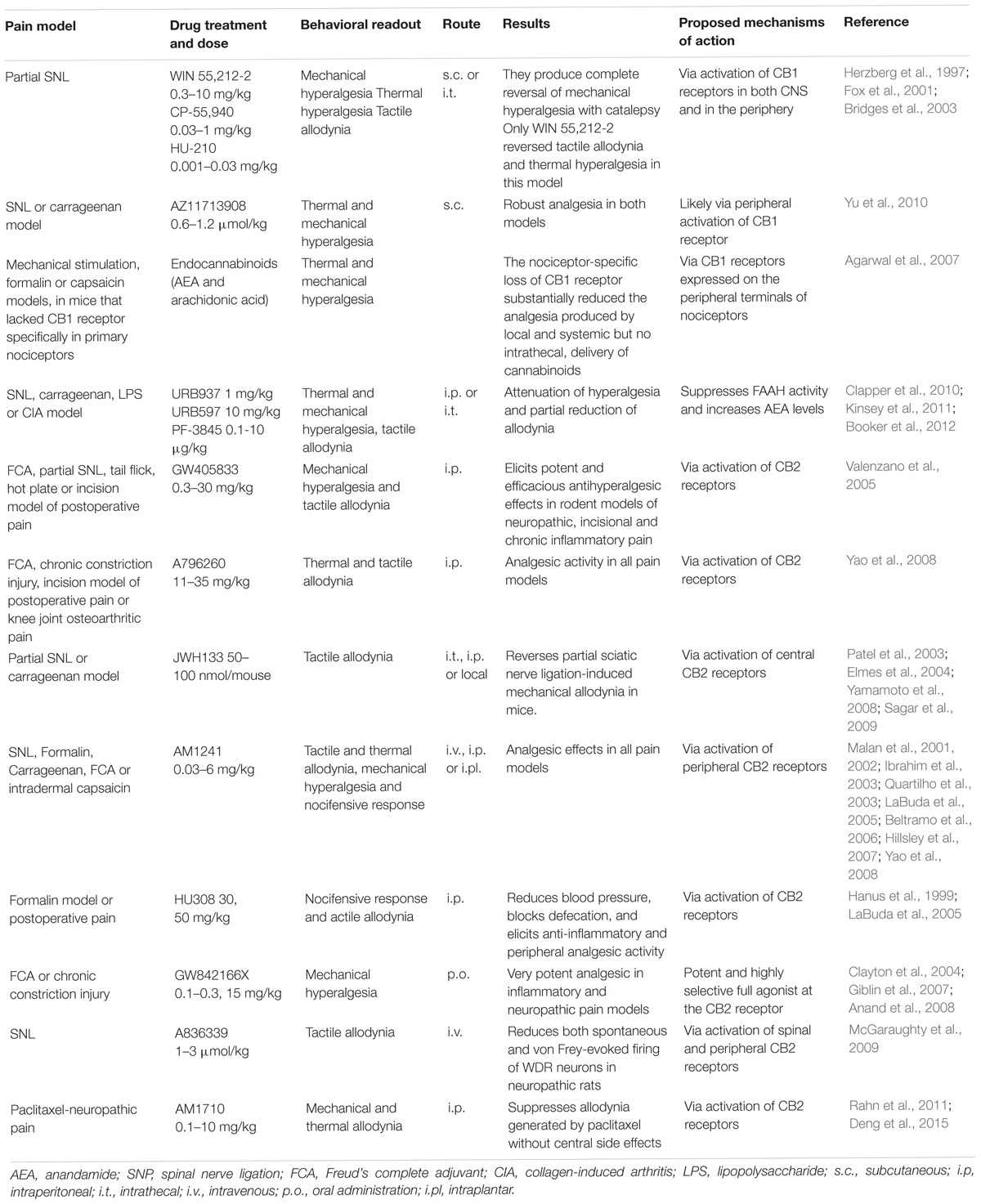
Table 1. Studies about the antinociceptive effects of CB1 and CB2 receptor agonists in different pain models.
A limitation to clinical use of cannabinoids for pain is their unfavorable side-effect profile, such as drowsiness, dizziness, speech impediments, memory impairment and confusion. Results of clinical trials with these agents indicate that high dosages are required to attain therapeutic effects and it is difficult to reach these dosages in clinical practice (Turcotte et al., 2010). At doses that prevent subjective effects, some cannabinoids seem to be ineffective for controlling acute pain (Kalliomäki et al., 2013). Several peripherally restricted CB1 and CB2 receptor agonists have been developed to avoid these side effects (Pertwee, 2009; Yu et al., 2010; Rahn et al., 2011; Yrjola et al., 2013). However, additional research is needed to improve study methodologies including the use of standard formulations and/or dosages, the increase in the number of subjects involved, and the general determination of the safe and effective use of cannabis for the treatment of human pain.
Another interesting area of research has recently focused on the evaluation of the possible synergy between cannabinoids and opioids in the management of pain. A combination of Δ9-THC and morphine diminished experimental pain in healthy volunteers (Roberts et al., 2006). Furthermore, dronabinol combined with opioids relieved chronic pain in patients (Narang et al., 2008).
In the last years, pain research has focused on the inhibition of the enzymes playing a role in EC metabolism and the elevation of the EC tonus locally. Special emphasis is given on multi-target analgesia compounds, where one of the targets is the EC degrading enzyme. Dual FAAH1 /TRPV1 blockers, such as N-arachidonoyl-serotonin (AA-5-HT) and OMDM198, are effective in animal studies, but this multi-target strategy has not yet reached the clinic (Maione et al., 2007, 2013; Morera et al., 2009; Costa et al., 2010; Malek et al., 2015).
Importantly, cannabinoids interact (apart from CB1 and CB2) with several other pharmacological receptors, including the cannabinoid putative receptors GPR18 and GPR55 (which have been even suggested as CBx and CB3 receptors). It is likely that the contradictory effects observed in clinical trials using Cannabis sp.-based treatments (e.g., Δ9-THC) may be due to the high promiscuity of cannabinoids for their receptors. Before achieving a clinical benefit from an EC system-based therapy in pain (and other alterations), it is mandatory to detect and understand the physiological and/or pathophysiological role of the cellular targets involved. In this context, we provide an analysis of the potential participation of the putative cannabinoid receptors GPR18 and GPR55 in pain (see below).
Gpr18 and Gpr55: Potential Targets for Pain Treatment
GPR55 and GPR18: Generalities
Cannabinoids interact with multiple orphan receptors (Alexander, 2012). Different groups have discussed if G protein-coupled receptor 18 (GPR18) and 55 (GPR55) should be considered as novel cannabinoid receptors (Alexander, 2012; Alexander et al., 2017). Nevertheless, the nomenclature suggested by the Nomenclature Committee of the Union of Basic and Clinical Pharmacology (NC-IUPHAR) Subcommittee on Cannabinoid Receptors (Pertwee et al., 2010) decided that all criteria to consider these as novel cannabinoid receptors remain incomplete and, accordingly, they were classified again as orphan receptors (Alexander et al., 2017). Independently of the official decision, these receptors clearly interact with cannabinoids directly or indirectly. Expression of GPR18 seems to be rich in the testis, spleen, peripheral blood leucocytes and lymph nodes (Gantz et al., 1997; Vassilatis et al., 2003; Rosenkilde et al., 2006). Its expression suggests a potential role in the control of immune system activity (e.g., leucocytes migration) (Burstein et al., 2011) and accordingly inflammation. Moreover, activation of GPR18 by N-arachidonoylglycine leads to apoptosis of inflammatory leukocytes (Burstein et al., 2011; Takenouchi et al., 2012), which in turn reduces local inflammation. There is also evidence that activation of GPR18 lowers intraocular pressure in mice (Miller et al., 2016). All these findings suggest a physiological function of NAGly via GPR18 in different inflammatory processes.
Knowledge about GPR55 physiology in the nervous system has increased recently (Marichal-Cancino et al., 2017). This receptor has been suggested as a potential therapeutic target in Parkinson’s disease due to a possible alteration on its expression in the basal nuclei (Celorrio et al., 2017), where it is related to procedural memories (Marichal-Cancino et al., 2016). GPR55 is also expressed in the hippocampus, where it has a role in spatial navigation (Marichal-Cancino et al., 2018). Furthermore, it is possible that some antiepileptic actions observed with phytocannabinoids involve the blocking of GPR55 (Kaplan et al., 2017). However, the above is a topic under study and findings are preliminary. Despite all advances in the physiology of GPR55, several actions in different areas of the CNS remain obscure (Marichal-Cancino et al., 2017). Interestingly, PEA (a cannabinoid related compound) is currently used to treat pain and inflammation. Like other cannabinoid related molecules, PEA has a very complex mechanism of action, which includes direct and/or indirect interaction with CB1, TRPV1, PPAR, GPR55 and GPR18, among other receptors (Keppel Hesselink et al., 2014). Certainly, PEA has high affinity for GPR55 as a full agonist (Ryberg et al., 2007). Thus, it is necessary to investigate whether GPR55 is involved in the analgesic and anti-inflammatory actions of PEA.
Actions of GPR18 and GPR55 and Their Potential Role in the Pharmacology of Pain
GPR18 and GPR55 are differentially expressed in the central and peripheral nociceptive systems of rodents and humans, suggesting a potential role in the modulation of nociceptive pathways (DRG TXome Database)2(Ray et al., 2018). In general, GPR18 is less studied compared to GPR55 (see below). This is partly due to the fact that signaling mechanisms and endogenous ligands are still controversial (Alexander et al., 2017). GPR18 has been suggested to modulate, depending on the ligand, both Gαi/o and Gαq/11 transduction pathways (Console-Bram et al., 2014). In this sense, NAGly is proposed as the endogenous GPR18 ligand (Kohno et al., 2006; McHugh et al., 2010). However, a recent study suggests that NAGly increases Ca2+ mobilization and MAPK activity in HAGPR55/CHO cells (Console-Bram et al., 2017). This response is attenuated by ML193 (GPR55 receptor antagonist) suggesting that NAGly-mediated effects depend on GPR55 activation. Moreover, an independent study reported that NAGly does not activate GPR18 receptors (Lu et al., 2013). In support of this, there is a previous observation showing that NAGly does not activate GPR18 (Yin et al., 2009). These discrepancies could be partially explained by the fact that NAGly is also a reversible and non-competitive inhibitor of the glycine transporter type 2 (GlyT2) (Wiles et al., 2006). In line with this, it has been shown that NAGly enhances inhibitory glycinergic transmission synaptic within the superficial dorsal horn by blocking glycine uptake via GlyT2 and decreasing excitatory NMDA-mediated synaptic transmission (Jeong et al., 2010).
It has been proposed that both GPR18 and GPR55 could play a role in the modulation of acute and chronic pain (Table 2). In animal models of inflammatory pain, intraplantar NAGly administration attenuates formalin-induced pain (Huang et al., 2001). Moreover, intrathecal administration of NAGly reduces complete Freund’s adjuvant (CFA)-induced mechanical allodynia and thermal hyperalgesia by a CB1-independent mechanism (Succar et al., 2007). Additionally, NAGly increases the production of 15-deoxy-Δ13,14-prostaglandin J2 and lipoxin A4, leading to a reduction in the migration of inflammatory cells into the area of acute inflammation (Burstein et al., 2011). GPR18 is expressed on human leukocytes, including polymorphonuclear neutrophils (PMN), monocytes, and macrophages and, furthermore, its activation regulates leukocyte trafficking during acute inflammation (Chiang et al., 2015). GPR18 and TRPV1 are expressed in chondrocytes within the deep zone of cartilage in patients with osteoarthritis (OA) (Dunn et al., 2016), suggesting that GPR18 presence in degenerate tissues could be a target for treatment with cannabinoids.
Nerve injury enhances expression of GPR18 mRNA in spinal cord and/or the DRG of rats, suggesting a potential role of GPR18 in the modulation of neuropathic pain (Malek et al., 2016). Accordingly, intrathecal administration of NAGly reduces mechanical allodynia in rats subjected to spinal nerve ligation and this effect is not prevented by pretreatment with either the CB1 or CB2 receptor antagonists AM251 and SR144528, respectively (Vuong et al., 2008). Although NAGly has been proposed as an endogenous GPR18 ligand, recent studies have found that resolvin D2 (RvD2) also activates GPR18 receptors (Chiang et al., 2015; Zhang et al., 2016). RvD2 activates recombinant human GPR18 in a receptor- and ligand-dependent manner and promotes the resolution of bacterial infections and organ protection (Chiang et al., 2015). Moreover, RvD2 enhances endothelial cell migration in a Rac-dependent manner via GPR18, and GPR18-deficient mice have an endogenous defect in perfusion recovery following hind limb ischemia (Zhang et al., 2016). In rodents, intrathecal administration of RvD2 reverses CFA-induced inflammatory pain, prevents formalin-induced spontaneous pain, and also reverses C-fiber stimulation-evoked long-term potentiation in the spinal cord (Park et al., 2011). However, RvD2 antinociceptive effects seem to be mediated by additional mechanisms involving the inhibition of transient receptor potential (TRPV1 and TRPA1) channels (Park et al., 2011). Undoubtedly, more studies to redefine the signaling pathways, ligands and physiological functions of GPR18 are needed.
GPR55 has been found highly expressed in large-diameter neurons, but present at low levels in small-diameter neurons of the mouse DRG (Lauckner et al., 2008). Indeed, reports suggest that GPR55 plays a role in modulating nociceptor excitability. Activation of GPR55 with lysophosphatidylinositol (LPI) promotes excitability in cultured large DRG neurons by increasing intracellular Ca2+ (Lauckner et al., 2008) and also produces mechanical hypersensitivity in mice after local peripheral administration (Gangadharan et al., 2013). Although there is a general consensus that LPI acts as an agonist for GPR55, it has been also reported that LPI modulates large-conductance Ca2+-activated potassium (K+) channels (BKCa) (Bondarenko et al., 2011a,b), 2-pore domain potassium (K+)-channels (TREK-1) (Maingret et al., 2000; Danthi et al., 2003) and the potassium (K+) channel subfamily K member 4 (KCNK4 or TRAAK) (Maingret et al., 2000), transient receptor potential (TRPV2; Monet et al., 2009; Harada et al., 2017), and transient receptor potential (TRPM8; Vanden Abeele et al., 2006; Andersson et al., 2007) channels. All these channels are expressed in the primary nociceptive pathway and their activation either modulates or amplifies sensory information (Basbaum et al., 2009). Therefore, the pharmacological data with LPI should be taken with caution. Furthermore, LPI is not the sole GPR55 activator. The hydrophilic glycerophospholipid lyso-phosphatidyl-β-D-glucoside (LysoPtdGlc) was recently reported as a regulator of the nociceptive central axon projections by activating GPR55 with high affinity (Guy et al., 2015). This indicates that glycerophospholipids could play a role modulating nociceptive inputs in vivo.
Nerve damage increases GPR55 mRNA expression in the spinal cord and DRG of rats (Malek et al., 2016) suggesting the participation of these receptors in neuropathic pain. It has been shown that the synthetic GPR55 agonist O-1602 reduces movement-evoked firing of nociceptive C fibers in a rat model of acute joint inflammation, and this effect is blocked by the GPR55 receptor antagonist O-1918 (Schuelert and McDougall, 2011). O-1602 also has protective effects in a murine model of experimentally induced colitis, but this anti-inflammatory effect could not be mediated by GPR55 (Schicho et al., 2011).
On the other hand, other studies have reported that GPR55 knockout mice show a reduced tumor-induced mechanical hypersensitivity (Gangadharan et al., 2013). GPR55 agonist O-1602 produces pronociceptive effects in neuropathic rats (Breen et al., 2012). At the central nervous system, local injection of the GPR55 putative inverse agonist CID16020046 into the anterior cingulated cortex (ACC) produces antinociception in the formalin test by decreasing the extracellular signal-regulated kinase 1/2 (ERK1/2) phosphorylation in the ACC and c-fos mRNA expression in the spinal cord (Okine et al., 2016). Moreover, LPI administration into the periaqueductal gray (PAG) attenuates nociceptive latencies in a hot-plate test and also produces a concentration-dependent increase in intracellular Ca2+ levels in dissociated rat PAG neurons expressing GPR55 mRNA (Deliu et al., 2015). Although the exact mechanisms underlying the GPR55-mediated antinociceptive effects remain to be elucidated, it has been suggested that some cytokines (e.g., IL-4 and IL-10) are responsible for the modulatory effects observed during inflammatory pain conditions (Staton et al., 2008).
Using cell lines, other studies have shown that GPR55 couples to Gα13 and activates GTPases RhoA, Cdc42 and Rac1 (Ryberg et al., 2007; Henstridge et al., 2009). Some efforts have tried to elucidate the G-protein signaling pathway activated by GPR55 agonists in vivo. Using pharmacological and conditional genetic tools in mice, the research group headed by Rohini Kuner showed that LPI-mediated hypersensitivity depends on the activation of Gα13 and Gαq/11, which in turn activate ERK1/2 (Gangadharan et al., 2013). In support of these results, it has been shown that LPI produces β-arrestin trafficking, MAPK, ERK1/2 phosphorylation and activates the G-protein signaling by a PKCβII-independent mechanism (Oka et al., 2007; Kapur et al., 2009). Interestingly, the effects on β-arrestin GPR55 complex formation, ERK1/2 phosphorylation and internalization of GPR55 are blocked by the GPR55 antagonist/partial agonist CP55,940 (Kapur et al., 2009), suggesting that a complex mechanism triggered upon GPR55 activation modulates G-coupled signaling pathways. Moreover, it has been documented that activation of GPR55 leads to additional p38 MAPK (Oka et al., 2010) and AKT phosphorylation (Pineiro et al., 2011). These events are related to the subsequent activation of several major transcription factors such as the nuclear factor of activated T-cells (NFAT) (Waldeck-Weiermair et al., 2008; Henstridge et al., 2009, 2010), CREB (Henstridge et al., 2010), NF-kB (Waldeck-Weiermair et al., 2008; Henstridge et al., 2010), and ATF2 (Oka et al., 2010).
Certainly, there is extensive literature indicating that signaling pathways involving MAPK and transcription factors such as NF-κB play an important role in pain (Niederberger and Geisslinger, 2008; Ji et al., 2009). However, it is worth emphasizing that most of the signaling mechanisms reported for GPR55 receptors have been obtained in vitro using cell lines and may not be completely translated to in vivo models. This is particularly important due to the recent discrepancies in the pain field using GPR55 knock-out mice. It was originally reported that mice lacking GPR55 show no differences in baseline pain responses compared to wild-type mice, but mechanical hyperalgesia is absent following either intraplantar CFA injection or partial nerve ligation (Staton et al., 2008). However, a recent study using knock-out mice suggests that GPR55 is dispensable for the development of inflammatory and neuropathic pain (Carey et al., 2017). According to these authors, GPR55 knock-out mice have no differences in mechanical, cold or heat hypersensitivity after intraplantar capsaicin, formalin or CFA injection. Likewise, development and maintenance of neuropathic pain after paclitaxel administration or partial nerve ligation is undistinguishable between GPR55 knock-out and wild-type mice. While the explanation for this discrepancy is not clear, Carey et al. have suggested that these differences could be due to multiple factors, including the way the GPR55 knock-out mice were made, the battery of tests used, freely moving animals versus restrained animals during the test, sex differences, body weight, and age of animals. Evidently, more behavioral studies using controlled experimental conditions will be necessary to define the importance of GPR55 receptors in modulating pain responses.
Conclusion
Cannabinoids, via CB1 receptors, mainly induce inhibition of pain integration that seems to be useful particularly in the treatment of chronic pain, whereas CB2 stimulation mainly causes antiinflammation via negative modulation of the immune system. GPR18 and GPR55 have a role in integrating, transmitting and/or alleviating pain. However, further studies using more selective pharmacological tools combined with genetic tools to generate cell-specific ablation or reactivation of GPR18/GPR55 receptors in specific cell populations will help to clarify the functional role of these receptors to take advantage of them in therapeutics.
Author Contributions
RG-A, PB-I, and EV-M developed the manuscript and discussed central ideas of it. AG-H and MC-L adapted the manuscript, designed graphs, and discussed central ideas of it. VG-S corrected the style and reviewed and edited the manuscript. MR supervised the project, worked on the conceptualization and acquired funding. BM-C conceived of the presented idea, integrated and edited information, and developed some central themes.
Funding
This work was supported by “Dirección General de Investigación y Posgrado” from Autonomous University of Aguascalientes. It was also supported by CONACyT through Postdoctoral Fellowship no. 274414 to PB-I.
Conflict of Interest Statement
The authors declare that the research was conducted in the absence of any commercial or financial relationships that could be construed as a potential conflict of interest.
Acknowledgments
We especially thank Prof. Dr. Theodore J. Price for revising the manuscript. We also thank to PRODEP’s program for supporting this work.
Footnotes
- ^FAAH, Fatty Acid Amide Hydrolase Enzyme. FAAH is an integral membrane enzyme that hydrolyzes the endocannabinoid anandamide and related amidated signaling lipids. FAAH KO mice display elevated anandamide levels, showing reduced nociceptive transmission in several pain models. Journal of Neurobiology 61: 149–60.
- ^http://www.utdallas.edu/bbs/painneurosciencelab/DRGtranscriptome/search.php
References
Agarwal, N., Pacher, P., Tegeder, I., Amaya, F., Constantin, C. E., Brenner, G. J., et al. (2007). Cannabinoids mediate analgesia largely via peripheral type 1 cannabinoid receptors in nociceptors. Nat. Neurosci. 10, 870–879. doi: 10.1038/nn1916
Agrawal, R. P., Goswami, J., Jain, S., and Kochar, D. K. (2009). Management of diabetic neuropathy by sodium valproate and glyceryl trinitrate spray: a prospective double-blind randomized placebo-controlled study. Diabetes Res. Clin. Pract. 83, 371–378. doi: 10.1016/j.diabres.2008.12.018
Ahluwalia, J., Urban, L., Capogna, M., Bevan, S., and Nagy, I. (2000). Cannabinoid 1 receptors are expressed in nociceptive primary sensory neurons. Neuroscience 100, 685–688. doi: 10.1016/S0306-4522(00)00389-4
Akerman, S., Kaube, H., and Goadsby, P. J. (2004). Anandamide is able to inhibit trigeminal neurons using an in vivo model of trigeminovascular-mediated nociception. J. Pharmacol. Exp. Ther. 309, 56–63. doi: 10.1124/jpet.103.059808
Albe-Fessard, D., Berkley, K. J., Kruger, L., Ralston, H. J. III, and Willis, W. D. Jr. (1985). Diencephalic mechanisms of pain sensation. Brain Res. 356, 217–296. doi: 10.1016/0165-0173(85)90013-X
Alexander, S. P. (2012). 2012 cannabinoid themed section. Br. J. Pharmacol. 167, 1573–1574. doi: 10.1111/j.1476-5381.2012.02238.x
Alexander, S. P., Christopoulos, A., Davenport, A. P., Kelly, E., Marrion, N. V., Peters, J. A., et al. (2017). The concise guide to pharmacology 2017/18: G protein-coupled receptors. Br. J. Pharmacol. 174(Suppl. 1), S17–S129. doi: 10.1111/bph.13878
Anand, U., Otto, W. R., Sanchez-Herrera, D., Facer, P., Yiangou, Y., Korchev, Y., et al. (2008). Cannabinoid receptor CB2 localisation and agonist-mediated inhibition of capsaicin responses in human sensory neurons. Pain 138, 667–680. doi: 10.1016/j.pain.2008.06.007
Andersson, D. A., Nash, M., and Bevan, S. (2007). Modulation of the cold-activated channel TRPM8 by lysophospholipids and polyunsaturated fatty acids. J. Neurosci. 27, 3347–3355. doi: 10.1523/JNEUROSCI.4846-06.2007
Attal, N., Brasseur, L., Guirimand, D., Clermond-Gnamien, S., Atlami, S., and Bouhassira, D. (2004). Are oral cannabinoids safe and effective in refractory neuropathic pain? Eur. J. Pain 8, 173–177.
Ballantyne, J. C., and Shin, N. S. (2008). Efficacy of opioids for chronic pain: a review of the evidence. Clin. J. Pain 24, 469–478. doi: 10.1097/AJP.0b013e31816b2f26
Barann, M., Molderings, G., Bruss, M., Bonisch, H., Urban, B. W., and Gothert, M. (2002). Direct inhibition by cannabinoids of human 5-HT3A receptors: probable involvement of an allosteric modulator site. Br. J. Pharmacol. 137, 589–596. doi: 10.1038/sj.bjp.0704829
Basbaum, A. I., Bautista, D. M., Scherrer, G., and Julius, D. (2009). Cellular and molecular mechanisms of pain. Cell 139, 267–284. doi: 10.1016/j.cell.2009.09.028
Basbaum, A. I., and Woolf, C. J. (1999). Pain. Curr. Biol. 9, R429–R431. doi: 10.1016/S0960-9822(99)80273-5
Beaulieu, P. (2006). Effects of nabilone, a synthetic cannabinoid, on postoperative pain. Can. J. Anaesth. 53, 769–775. doi: 10.1007/BF03022793
Beltramo, M., Bernardini, N., Bertorelli, R., Campanella, M., Nicolussi, E., Fredduzzi, S., et al. (2006). CB2 receptor-mediated antihyperalgesia: possible direct involvement of neural mechanisms. Eur. J. Neurosci. 23, 1530–1538. doi: 10.1111/j.1460-9568.2006.04684.x
Belvisi, M. G., Patel, H. J., Freund-Michel, V., Hele, D. J., Crispino, N., and Birrell, M. A. (2008). Inhibitory activity of the novel CB2 receptor agonist, GW833972A, on guinea-pig and human sensory nerve function in the airways. Br. J. Pharmacol. 155, 547–557. doi: 10.1038/bjp.2008.298
Berman, J. S., Symonds, C., and Birch, R. (2004). Efficacy of two cannabis based medicinal extracts for relief of central neuropathic pain from brachial plexus avulsion: results of a randomised controlled trial. Pain 112, 299–306. doi: 10.1016/j.pain.2004.09.013
Bhosale, U. A., Quraishi, N., Yegnanarayan, R., and Devasthale, D. (2015). A comparative study to evaluate the cardiovascular risk of selective and nonselective cyclooxygenase inhibitors (COX-Is) in arthritic patients. J. Basic Clin. Physiol. Pharmacol. 26, 73–79. doi: 10.1515/jbcpp-2014-0005
Bondarenko, A. I., Malli, R., and Graier, W. F. (2011a). The GPR55 agonist lysophosphatidylinositol acts as an intracellular messenger and bidirectionally modulates Ca2+ -activated large-conductance K+ channels in endothelial cells. Pflugers Arch. 461, 177–189. doi: 10.1007/s00424-010-0898-x
Bondarenko, A. I., Malli, R., and Graier, W. F. (2011b). The GPR55 agonist lysophosphatidylinositol directly activates intermediate-conductance Ca2+ -activated K+ channels. Pflugers Arch. 462, 245–255. doi: 10.1007/s00424-011-0977-7
Booker, L., Kinsey, S. G., Abdullah, R. A., Blankman, J. L., Long, J. Z., Ezzili, C., et al. (2012). The fatty acid amide hydrolase (FAAH) inhibitor PF-3845 acts in the nervous system to reverse LPS-induced tactile allodynia in mice. Br. J. Pharmacol. 165, 2485–2496. doi: 10.1111/j.1476-5381.2011.01445.x
Bouaboula, M., Hilairet, S., Marchand, J., Fajas, L., Le Fur, G., and Casellas, P. (2005). Anandamide induced PPARgamma transcriptional activation and 3T3-L1 preadipocyte differentiation. Eur. J. Pharmacol. 517, 174–181. doi: 10.1016/j.ejphar.2005.05.032
Breen, C., Brownjohn, P. W., and Ashton, J. C. (2012). The atypical cannabinoid O-1602 increases hind paw sensitisation in the chronic constriction injury model of neuropathic pain. Neurosci. Lett. 508, 119–122. doi: 10.1016/j.neulet.2011.12.039
Breuer, B., Pappagallo, M., Knotkova, H., Guleyupoglu, N., Wallenstein, S., and Portenoy, R. K. (2007). A randomized, double-blind, placebo-controlled, two-period, crossover, pilot trial of lamotrigine in patients with central pain due to multiple sclerosis. Clin. Ther. 29, 2022–2030. doi: 10.1016/j.clinthera.2007.09.023
Bridges, D., Rice, A. S., Egertova, M., Elphick, M. R., Winter, J., and Michael, G. J. (2003). Localisation of cannabinoid receptor 1 in rat dorsal root ganglion using in situ hybridisation and immunohistochemistry. Neuroscience 119, 803–812. doi: 10.1016/S0306-4522(03)00200-8
Buffum, M., and Buffum, J. C. (2000). Nonsteroidal anti-inflammatory drugs in the elderly. Pain Manag. Nurs. 1, 40–50. doi: 10.1053/jpmn.2000.7779
Burstein, S. H., Mcquain, C. A., Ross, A. H., Salmonsen, R. A., and Zurier, R. E. (2011). Resolution of inflammation by N-arachidonoylglycine. J. Cell. Biochem. 112, 3227–3233. doi: 10.1002/jcb.23245
Burston, J. J., and Woodhams, S. G. (2014). Endocannabinoid system and pain: an introduction. Proc. Nutr. Soc. 73, 106–117. doi: 10.1017/S0029665113003650
Calabro, R. S., Gervasi, G., Marino, S., Mondo, P. N., and Bramanti, P. (2010). Misdiagnosed chronic pelvic pain: pudendal neuralgia responding to a novel use of palmitoylethanolamide. Pain Med. 11, 781–784. doi: 10.1111/j.1526-4637.2010.00823.x
Calignano, A., La Rana, G., and Piomelli, D. (2001). Antinociceptive activity of the endogenous fatty acid amide, palmitylethanolamide. Eur. J. Pharmacol. 419, 191–198. doi: 10.1016/S0014-2999(01)00988-8
Callen, L., Moreno, E., Barroso-Chinea, P., Moreno-Delgado, D., Cortes, A., Mallol, J., et al. (2012). Cannabinoid receptors CB1 and CB2 form functional heteromers in brain. J. Biol. Chem. 287, 20851–20865. doi: 10.1074/jbc.M111.335273
Carey, L. M., Gutierrez, T., Deng, L., Lee, W. H., Mackie, K., and Hohmann, A. G. (2017). Inflammatory and neuropathic nociception is preserved in GPR55 knockout mice. Sci. Rep. 7:944. doi: 10.1038/s41598-017-01062-2
Celorrio, M., Rojo-Bustamante, E., Fernandez-Suarez, D., Saez, E., Estella-Hermoso De Mendoza, A., Muller, C. E., et al. (2017). GPR55: a therapeutic target for Parkinson’s disease? Neuropharmacology 125, 319–332. doi: 10.1016/j.neuropharm.2017.08.017
Chen, J., Li, L., Chen, S. R., Chen, H., Xie, J. D., Sirrieh, R. E., et al. (2018). The alpha2delta-1-NMDA receptor complex is critically involved in neuropathic pain development and gabapentin therapeutic actions. Cell Rep. 22, 2307–2321. doi: 10.1016/j.celrep.2018.02.021
Chiang, N., Dalli, J., Colas, R. A., and Serhan, C. N. (2015). Identification of resolvin D2 receptor mediating resolution of infections and organ protection. J. Exp. Med. 212, 1203–1217. doi: 10.1084/jem.20150225
Chu, L. F., Angst, M. S., and Clark, D. (2008). Opioid-induced hyperalgesia in humans: molecular mechanisms and clinical considerations. Clin. J. Pain 24, 479–496. doi: 10.1097/AJP.0b013e31816b2f43
Clapper, J. R., Moreno-Sanz, G., Russo, R., Guijarro, A., Vacondio, F., Duranti, A., et al. (2010). Anandamide suppresses pain initiation through a peripheral endocannabinoid mechanism. Nat. Neurosci. 13, 1265–1270. doi: 10.1038/nn.2632
Clayton, N. M., Wilson, A. W., Collins, S. D., Giblin, G. M., Mitchell, B. L., Goldsmith, P., et al. (2004). Anti-hypersensitive and anti-inflammatory activity of the potent and selective CB2 agonist GW842166X. Proc. Br. Pharmacol. Soc. 21:050P. Available at: http://www.pa2online.org/Vol2Issue4abst050P.html
Clermont-Gnamien, S., Atlani, S., Attal, N., Le Mercier, F., Guirimand, F., and Brasseur, L. (2002). [The therapeutic use of D9-tetrahydrocannabinol (dronabinol) in refractory neuropathic pain]. Presse Med. 31, 1840–1845.
Condés-Lara, M., Martínez-Lorenzana, G., Rubio-Beltrán, E., Rodríguez-Jiménez, J., Rojas-Piloni, G., and González-Hernández, A. (2015). Hypothalamic paraventricular nucleus stimulation enhances c-Fos expression in spinal and supraspinal structures related to pain modulation. Neurosci. Res. 98, 59–63. doi: 10.1016/j.neures.2015.04.004
Conigliaro, R., Drago, V., Foster, P. S., Schievano, C., and Di Marzo, V. (2011). Use of palmitoylethanolamide in the entrapment neuropathy of the median in the wrist. Minerva Med. 102, 141–147.
Console-Bram, L., Brailoiu, E., Brailoiu, G. C., Sharir, H., and Abood, M. E. (2014). Activation of GPR18 by cannabinoid compounds: a tale of biased agonism. Br. J. Pharmacol. 171, 3908–3917. doi: 10.1111/bph.12746
Console-Bram, L., Ciuciu, S. M., Zhao, P., Zipkin, R. E., Brailoiu, E., and Abood, M. E. (2017). N-arachidonoyl glycine, another endogenous agonist of GPR55. Biochem. Biophys. Res. Commun. 490, 1389–1393. doi: 10.1016/j.bbrc.2017.07.038
Corchero, J., Avila, M. A., Fuentes, J. A., and Manzanares, J. (1997a). delta-9-Tetrahydrocannabinol increases prodynorphin and proenkephalin gene expression in the spinal cord of the rat. Life Sci. 61, 39–43.
Corchero, J., Fuentes, J. A., and Manzanares, J. (1997b). delta 9-Tetrahydrocannabinol increases proopiomelanocortin gene expression in the arcuate nucleus of the rat hypothalamus. Eur. J. Pharmacol. 323, 193–195. doi: 10.1016/S0014-2999(97)00144-1
Corey-Bloom, J., Wolfson, T., Gamst, A., Jin, S., Marcotte, T. D., Bentley, H., et al. (2012). Smoked cannabis for spasticity in multiple sclerosis: a randomized, placebo-controlled trial. CMAJ 184, 1143–1150. doi: 10.1503/cmaj.110837
Costa, B., Bettoni, I., Petrosino, S., Comelli, F., Giagnoni, G., and Di Marzo, V. (2010). The dual fatty acid amide hydrolase/TRPV1 blocker, N-arachidonoyl-serotonin, relieves carrageenan-induced inflammation and hyperalgesia in mice. Pharmacol. Res. 61, 537–546. doi: 10.1016/j.phrs.2010.02.001
Crawley, J. N., Corwin, R. L., Robinson, J. K., Felder, C. C., Devane, W. A., and Axelrod, J. (1993). Anandamide, an endogenous ligand of the cannabinoid receptor, induces hypomotility and hypothermia in vivo in rodents. Pharmacol. Biochem. Behav. 46, 967–972. doi: 10.1016/0091-3057(93)90230-Q
Crean, R. D., Tapert, S. F., Minassian, A., Macdonald, K., Crane, N. A., and Mason, B. J. (2011). Effects of chronic, heavy cannabis use on executive functions. J. Addict. Med. 5, 9–15. doi: 10.1097/ADM.0b013e31820cdd57
D’Agostino, G., La Rana, G., Russo, R., Sasso, O., Iacono, A., Esposito, E., et al. (2007). Acute intracerebroventricular administration of palmitoylethanolamide, an endogenous peroxisome proliferator-activated receptor-alpha agonist, modulates carrageenan-induced paw edema in mice. J. Pharmacol. Exp. Ther. 322, 1137–1143. doi: 10.1124/jpet.107.123265
Deliu, E., Sperow, M., Console-Bram, L., Carter, R. L., Tilley, D. G., Kalamarides, D. J., et al. (2015). The lysophosphatidylinositol receptor GPR55 modulates pain perception in the periaqueductal gray. Mol. Pharmacol. 88, 265–272. doi: 10.1124/mol.115.099333
Danthi, S., Enyeart, J. A., and Enyeart, J. J. (2003). Modulation of native TREK-1 and Kv1.4 K+ channels by polyunsaturated fatty acids and lysophospholipids. J. Membr. Biol. 195, 147–164. doi: 10.1007/s00232-003-0616-0
Davis, M. P. (2014). Cannabinoids in pain management: CB1, CB2 and non-classic receptor ligands. Expert Opin. Investig. Drugs 23, 1123–1140. doi: 10.1517/13543784.2014.918603
Demuth, D. G., and Molleman, A. (2006). Cannabinoid signalling. Life Sci. 78, 549–563. doi: 10.1016/j.lfs.2005.05.055
Deng, L., Guindon, J., Cornett, B. L., Makriyannis, A., Mackie, K., and Hohmann, A. G. (2015). Chronic cannabinoid receptor 2 activation reverses paclitaxel neuropathy without tolerance or cannabinoid receptor 1-dependent withdrawal. Biol. Psychiatry 77, 475–487. doi: 10.1016/j.biopsych.2014.04.009
Devane, W. A., Hanus, L., Breuer, A., Pertwee, R. G., Stevenson, L. A., Griffin, G., et al. (1992). Isolation and structure of a brain constituent that binds to the cannabinoid receptor. Science 258, 1946–1949. doi: 10.1126/science.1470919
Di Marzo, V., Bifulco, M., and De Petrocellis, L. (2004). The endocannabinoid system and its therapeutic exploitation. Nat. Rev. Drug Discov. 3, 771–784. doi: 10.1038/nrd1495
Dowell, D., Haegerich, T. M., and Chou, R. (2016). CDC guideline for prescribing opioids for chronic pain–united states, 2016. JAMA 315, 1624–1645. doi: 10.1001/jama.2016.1464
Drewes, A. M., Andreasen, A., and Poulsen, L. H. (1994). Valproate for treatment of chronic central pain after spinal cord injury. A double-blind cross-over study. Paraplegia 32, 565–569.
Dumas, E. O., and Pollack, G. M. (2008). Opioid tolerance development: a pharmacokinetic/pharmacodynamic perspective. AAPS J. 10, 537–551. doi: 10.1208/s12248-008-9056-1
Dunn, S. L., Wilkinson, J. M., Crawford, A., Bunning, R. A. D., and Le Maitre, C. L. (2016). Expression of cannabinoid receptors in human osteoarthritic cartilage: implications for future therapies. Cannabis Cannabinoid Res. 1, 3–15. doi: 10.1089/can.2015.0001
Eisenberg, E., Lurie, Y., Braker, C., Daoud, D., and Ishay, A. (2001). Lamotrigine reduces painful diabetic neuropathy: a randomized, controlled study. Neurology 57, 505–509. doi: 10.1212/WNL.57.3.505
Elmes, S. J., Jhaveri, M. D., Smart, D., Kendall, D. A., and Chapman, V. (2004). Cannabinoid CB2 receptor activation inhibits mechanically evoked responses of wide dynamic range dorsal horn neurons in naive rats and in rat models of inflammatory and neuropathic pain. Eur. J. Neurosci. 20, 2311–2320. doi: 10.1111/j.1460-9568.2004.03690.x
Evans, R. M., Scott, R. H., and Ross, R. A. (2004). Multiple actions of anandamide on neonatal rat cultured sensory neurones. Br. J. Pharmacol. 141, 1223–1233. doi: 10.1038/sj.bjp.0705723
Fabbro, F., and Crescentini, C. (2014). Facing the experience of pain: a neuropsychological perspective. Phys. Life Rev. 11, 540–552. doi: 10.1016/j.plrev.2013.12.010
Fan, P. (1995). Cannabinoid agonists inhibit the activation of 5-HT3 receptors in rat nodose ganglion neurons. J. Neurophysiol. 73, 907–910. doi: 10.1152/jn.1995.73.2.907
Felder, C. C., Joyce, K. E., Briley, E. M., Mansouri, J., Mackie, K., Blond, O., et al. (1995). Comparison of the pharmacology and signal transduction of the human cannabinoid CB1 and CB2 receptors. Mol. Pharmacol. 48, 443–450.
Finnerup, N. B., Sindrup, S. H., and Jensen, T. S. (2010). The evidence for pharmacological treatment of neuropathic pain. Pain 150, 573–581. doi: 10.1016/j.pain.2010.06.019
Fischbach, T., Greffrath, W., Nawrath, H., and Treede, R. D. (2007). Effects of anandamide and noxious heat on intracellular calcium concentration in nociceptive DRG neurons of rats. J. Neurophysiol. 98, 929–938. doi: 10.1152/jn.01096.2006
Fox, A., Kesingland, A., Gentry, C., Mcnair, K., Patel, S., Urban, L., et al. (2001). The role of central and peripheral Cannabinoid1 receptors in the antihyperalgesic activity of cannabinoids in a model of neuropathic pain. Pain 92, 91–100. doi: 10.1016/S0304-3959(00)00474-7
Fu, J., Gaetani, S., Oveisi, F., Lo Verme, J., Serrano, A., Rodriguez De Fonseca, F., et al. (2003). Oleylethanolamide regulates feeding and body weight through activation of the nuclear receptor PPAR-alpha. Nature 425, 90–93. doi: 10.1038/nature01921
Galiegue, S., Mary, S., Marchand, J., Dussossoy, D., Carriere, D., Carayon, P., et al. (1995). Expression of central and peripheral cannabinoid receptors in human immune tissues and leukocyte subpopulations. Eur. J. Biochem. 232, 54–61. doi: 10.1111/j.1432-1033.1995.tb20780.x
Gangadharan, V., Selvaraj, D., Kurejova, M., Njoo, C., Gritsch, S., Skoricova, D., et al. (2013). A novel biological role for the phospholipid lysophosphatidylinositol in nociceptive sensitization via activation of diverse G-protein signalling pathways in sensory nerves in vivo. Pain 154, 2801–2812. doi: 10.1016/j.pain.2013.08.019
Gantz, I., Muraoka, A., Yang, Y. K., Samuelson, L. C., Zimmerman, E. M., Cook, H., et al. (1997). Cloning and chromosomal localization of a gene (GPR18) encoding a novel seven transmembrane receptor highly expressed in spleen and testis. Genomics 42, 462–466. doi: 10.1006/geno.1997.4752
Gaoni, Y., and Mechoulam, R. (1964). Isolation, structure, and partial synthesis of an active constituent of hashish. J. Am. Chem. Soc. 86, 1646–1647. doi: 10.1021/ja01062a046
Gatti, A., Lazzari, M., Gianfelice, V., Di Paolo, A., Sabato, E., and Sabato, A. F. (2012). Palmitoylethanolamide in the treatment of chronic pain caused by different etiopathogenesis. Pain Med. 13, 1121–1130. doi: 10.1111/j.1526-4637.2012.01432.x
Gauldie, S. D., Mcqueen, D. S., Pertwee, R., and Chessell, I. P. (2001). Anandamide activates peripheral nociceptors in normal and arthritic rat knee joints. Br. J. Pharmacol. 132, 617–621. doi: 10.1038/sj.bjp.0703890
Gee, N. S., Brown, J. P., Dissanayake, V. U., Offord, J., Thurlow, R., and Woodruff, G. N. (1996). The novel anticonvulsant drug, gabapentin (Neurontin), binds to the alpha2delta subunit of a calcium channel. J. Biol. Chem. 271, 5768–5776. doi: 10.1074/jbc.271.10.5768
Giblin, G. M. P., O’shaughnessy, C. T., Naylor, A., Mitchell, W. L., Eatherton, A. J., Slingsby, B. P., et al. (2007). Discovery of 2-[(2,4-dichlorophenyl)amino]-N-[(tetrahydro- 2H-pyran-4-yl)methyl]-4-(trifluoromethyl)- 5-pyrimidinecarboxamide, a selective CB2 receptor agonist for the treatment of inflammatory pain. J. Med. Chem. 50, 2597–2600. doi: 10.1021/jm061195+
Glass, M., Dragunow, M., and Faull, R. L. (1997). Cannabinoid receptors in the human brain: a detailed anatomical and quantitative autoradiographic study in the fetal, neonatal and adult human brain. Neuroscience 77, 299–318. doi: 10.1016/S0306-4522(96)00428-9
Godlewski, G., Gothert, M., and Malinowska, B. (2003). Cannabinoid receptor-independent inhibition by cannabinoid agonists of the peripheral 5-HT3 receptor-mediated von Bezold-Jarisch reflex. Br. J. Pharmacol. 138, 767–774. doi: 10.1038/sj.bjp.0705114
Gong, J. P., Onaivi, E. S., Ishiguro, H., Liu, Q. R., Tagliaferro, P. A., Brusco, A., et al. (2006). Cannabinoid CB2 receptors: immunohistochemical localization in rat brain. Brain Res. 1071, 10–23. doi: 10.1016/j.brainres.2005.11.035
González-Hernández, A., Martínez-Lorenzana, G., Rodríguez-Jiménez, J., Rojas-Piloni, G., and Condés-Lara, M. (2015). Intracisternal injection of palmitoylethanolamide inhibits the peripheral nociceptive evoked responses of dorsal horn wide dynamic range neurons. J. Neural Transm. 122, 369–374. doi: 10.1007/s00702-014-1255-6
Griffin, M. R., Piper, J. M., Daugherty, J. R., Snowden, M., and Ray, W. A. (1991). Nonsteroidal anti-inflammatory drug use and increased risk for peptic ulcer disease in elderly persons. Ann. Intern. Med. 114, 257–263. doi: 10.7326/0003-4819-114-4-257
Guy, A. T., Nagatsuka, Y., Ooashi, N., Inoue, M., Nakata, A., Greimel, P., et al. (2015). Glycerophospholipid regulation of modality-specific sensory axon guidance in the spinal cord. Science 349, 974–977. doi: 10.1126/science.aab3516
Hanus, L., Abu-Lafi, S., Fride, E., Breuer, A., Vogel, Z., Shalev, D. E., et al. (2001). 2-arachidonyl glyceryl ether, an endogenous agonist of the cannabinoid CB1 receptor. Proc. Natl. Acad. Sci. U.S.A. 98, 3662–3665. doi: 10.1073/pnas.061029898
Hanus, L., Breuer, A., Tchilibon, S., Shiloah, S., Goldenberg, D., Horowitz, M., et al. (1999). HU-308: a specific agonist for CB2, a peripheral cannabinoid receptor. Proc. Natl. Acad. Sci. U.S.A. 96, 14228–14233. doi: 10.1073/pnas.96.25.14228
Harada, K., Kitaguchi, T., Kamiya, T., Aung, K. H., Nakamura, K., Ohta, K., et al. (2017). Lysophosphatidylinositol-induced activation of the cation channel TRPV2 triggers glucagon-like peptide-1 secretion in enteroendocrine L cells. J. Biol. Chem. 292, 10855–10864. doi: 10.1074/jbc.M117.788653
Heinricher, M. M. (2016). Pain modulation and the transition from acute to chronic pain. Adv. Exp. Med. Biol. 904, 105–115. doi: 10.1007/978-94-017-7537-3_8
Henstridge, C. M., Balenga, N. A., Ford, L. A., Ross, R. A., Waldhoer, M., and Irving, A. J. (2009). The GPR55 ligand L-alpha-lysophosphatidylinositol promotes RhoA-dependent Ca2+ signaling and NFAT activation. FASEB J. 23, 183–193. doi: 10.1096/fj.08-108670
Henstridge, C. M., Balenga, N. A., Schroder, R., Kargl, J. K., Platzer, W., Martini, L., et al. (2010). GPR55 ligands promote receptor coupling to multiple signalling pathways. Br. J. Pharmacol. 160, 604–614. doi: 10.1111/j.1476-5381.2009.00625.x
Herzberg, U., Eliav, E., Bennett, G. J., and Kopin, I. J. (1997). The analgesic effects of R(+)-WIN 55,212-2 mesylate, a high affinity cannabinoid agonist, in a rat model of neuropathic pain. Neurosci. Lett. 221, 157–160. doi: 10.1016/S0304-3940(96)13308-5
Hillsley, K., Mccaul, C., Aerssens, J., Peeters, P. J., Gijsen, H., Moechars, D., et al. (2007). Activation of the cannabinoid 2 (CB2) receptor inhibits murine mesenteric afferent nerve activity. Neurogastroenterol. Motil. 19, 769–777. doi: 10.1111/j.1365-2982.2007.00950.x
Hohmann, A. G., Briley, E. M., and Herkenham, M. (1999). Pre- and postsynaptic distribution of cannabinoid and mu opioid receptors in rat spinal cord. Brain Res. 822, 17–25. doi: 10.1016/S0006-8993(98)01321-3
Hohmann, A. G., and Herkenham, M. (1999). Localization of central cannabinoid CB1 receptor messenger RNA in neuronal subpopulations of rat dorsal root ganglia: a double-label in situ hybridization study. Neuroscience 90, 923–931. doi: 10.1016/S0306-4522(98)00524-7
Horl, W. H. (2010). Nonsteroidal anti-inflammatory drugs and the kidney. Pharmaceuticals 3, 2291–2321. doi: 10.3390/ph3072291
Howlett, A. C. (2002). The cannabinoid receptors. Prostaglandins Other Lipid Mediat. 68–69, 619–631. doi: 10.1016/S0090-6980(02)00060-6
Hsieh, G. C., Pai, M., Chandran, P., Hooker, B. A., Zhu, C. Z., Salyers, A. K., et al. (2011). Central and peripheral sites of action for CB2 receptor mediated analgesic activity in chronic inflammatory and neuropathic pain models in rats. Br. J. Pharmacol. 162, 428–440. doi: 10.1111/j.1476-5381.2010.01046.x
Huang, S. M., Bisogno, T., Petros, T. J., Chang, S. Y., Zavitsanos, P. A., Zipkin, R. E., et al. (2001). Identification of a new class of molecules, the arachidonyl amino acids, and characterization of one member that inhibits pain. J. Biol. Chem. 276, 42639–42644. doi: 10.1074/jbc.M107351200
Ibrahim, M. M., Deng, H., Zvonok, A., Cockayne, D. A., Kwan, J., Mata, H. P., et al. (2003). Activation of CB2 cannabinoid receptors by AM1241 inhibits experimental neuropathic pain: pain inhibition by receptors not present in the CNS. Proc. Natl. Acad. Sci. U.S.A. 100, 10529–10533. doi: 10.1073/pnas.1834309100
Ishac, E. J., Jiang, L., Lake, K. D., Varga, K., Abood, M. E., and Kunos, G. (1996). Inhibition of exocytotic noradrenaline release by presynaptic cannabinoid CB1 receptors on peripheral sympathetic nerves. Br. J. Pharmacol. 118, 2023–2028. doi: 10.1111/j.1476-5381.1996.tb15639.x
Jaggar, S. I., Hasnie, F. S., Sellaturay, S., and Rice, A. S. (1998). The anti-hyperalgesic actions of the cannabinoid anandamide and the putative CB2 receptor agonist palmitoylethanolamide in visceral and somatic inflammatory pain. Pain 76, 189–199. doi: 10.1016/S0304-3959(98)00041-4
Jensen, B., Chen, J., Furnish, T., and Wallace, M. (2015). Medical marijuana and chronic pain: a review of basic science and clinical evidence. Curr. Pain Headache Rep. 19:50. doi: 10.1007/s11916-015-0524-x
Jeong, H. J., Vandenberg, R. J., and Vaughan, C. W. (2010). N-arachidonyl-glycine modulates synaptic transmission in superficial dorsal horn. Br. J. Pharmacol. 161, 925–935. doi: 10.1111/j.1476-5381.2010.00935.x
Ji, R. R., Gereau, R. W. T., Malcangio, M., and Strichartz, G. R. (2009). MAP kinase and pain. Brain Res. Rev. 60, 135–148. doi: 10.1016/j.brainresrev.2008.12.011
Johanek, L. M., Heitmiller, D. R., Turner, M., Nader, N., Hodges, J., and Simone, D. A. (2001). Cannabinoids attenuate capsaicin-evoked hyperalgesia through spinal and peripheral mechanisms. Pain 93, 303–315. doi: 10.1016/S0304-3959(01)00336-0
Johanek, L. M., and Simone, D. A. (2004). Activation of peripheral cannabinoid receptors attenuates cutaneous hyperalgesia produced by a heat injury. Pain 109, 432–442. doi: 10.1016/j.pain.2004.02.020
Julius, D., and Basbaum, A. I. (2001). Molecular mechanisms of nociception. Nature 413, 203–210. doi: 10.1038/35093019
Kalliomäki, J., Segerdahl, M., Webster, L., Reimfelt, A., Huizar, K., Annas, P., et al. (2013). Evaluation of the analgesic efficacy of AZD1940, a novel cannabinoid agonist, on post-operative pain after lower third molar surgical removal. Scand. J. Pain 4, 17–22. doi: 10.1016/j.sjpain.2012.08.004
Kaplan, J. S., Stella, N., Catterall, W. A., and Westenbroek, R. E. (2017). Cannabidiol attenuates seizures and social deficits in a mouse model of Dravet syndrome. Proc. Natl. Acad. Sci. U.S.A. 114, 11229–11234. doi: 10.1073/pnas.1711351114
Kapur, A., Zhao, P., Sharir, H., Bai, Y., Caron, M. G., Barak, L. S., et al. (2009). Atypical responsiveness of the orphan receptor GPR55 to cannabinoid ligands. J. Biol. Chem. 284, 29817–29827. doi: 10.1074/jbc.M109.050187
Keppel Hesselink, J. M., Kopsky, D. J., and Sajben, N. L. (2014). Vulvodynia and proctodynia treated with topical baclofen 5 % and palmitoylethanolamide. Arch. Gynecol. Obstet. 290, 389–393. doi: 10.1007/s00404-014-3218-4
Kinsey, S. G., Naidu, P. S., Cravatt, B. F., Dudley, D. T., and Lichtman, A. H. (2011). Fatty acid amide hydrolase blockade attenuates the development of collagen-induced arthritis and related thermal hyperalgesia in mice. Pharmacol. Biochem. Behav. 99, 718–725. doi: 10.1016/j.pbb.2011.06.022
Knerlich-Lukoschus, F., Noack, M., Von Der Ropp-Brenner, B., Lucius, R., Mehdorn, H. M., and Held-Feindt, J. (2011). Spinal cord injuries induce changes in CB1 cannabinoid receptor and C-C chemokine expression in brain areas underlying circuitry of chronic pain conditions. J. Neurotrauma 28, 619–634. doi: 10.1089/neu.2010.1652
Ko, M. C., and Woods, J. H. (1999). Local administration of delta9-tetrahydrocannabinol attenuates capsaicin-induced thermal nociception in rhesus monkeys: a peripheral cannabinoid action. Psychopharmacology 143, 322–326. doi: 10.1007/s002130050955
Kohno, M., Hasegawa, H., Inoue, A., Muraoka, M., Miyazaki, T., Oka, K., et al. (2006). Identification of N-arachidonylglycine as the endogenous ligand for orphan G-protein-coupled receptor GPR18. Biochem. Biophys. Res. Commun. 347, 827–832. doi: 10.1016/j.bbrc.2006.06.175
Kraft, B., Frickey, N. A., Kaufmann, R. M., Reif, M., Frey, R., Gustorff, B., et al. (2008). Lack of analgesia by oral standardized cannabis extract on acute inflammatory pain and hyperalgesia in volunteers. Anesthesiology 109, 101–110. doi: 10.1097/ALN.0b013e31817881e1
LaBuda, C. J., Koblish, M., and Little, P. J. (2005). Cannabinoid CB2 receptor agonist activity in the hindpaw incision model of postoperative pain. Eur. J. Pharmacol. 527, 172–174. doi: 10.1016/j.ejphar.2005.10.020
Langford, R. M., Mares, J., Novotna, A., Vachova, M., Novakova, I., Notcutt, W., et al. (2013). A double-blind, randomized, placebo-controlled, parallel-group study of THC/CBD oromucosal spray in combination with the existing treatment regimen, in the relief of central neuropathic pain in patients with multiple sclerosis. J. Neurol. 260, 984–997. doi: 10.1007/s00415-012-6739-4
Laprairie, R. B., Kelly, M. E., and Denovan-Wright, E. M. (2012). The dynamic nature of type 1 cannabinoid receptor (CB1) gene transcription. Br. J. Pharmacol. 167, 1583–1595. doi: 10.1111/j.1476-5381.2012.02175.x
Lauckner, J. E., Jensen, J. B., Chen, H. Y., Lu, H. C., Hille, B., and Mackie, K. (2008). GPR55 is a cannabinoid receptor that increases intracellular calcium and inhibits M current. Proc. Natl. Acad. Sci. U.S.A. 105, 2699–2704. doi: 10.1073/pnas.0711278105
Li, H.-L. (1974). An archaeological and historical account of cannabis in China. Econ. Bot. 28, 437–448. doi: 10.1007/BF02862859
Li, J., Daughters, R. S., Bullis, C., Bengiamin, R., Stucky, M. W., Brennan, J., et al. (1999). The cannabinoid receptor agonist WIN 55,212-2 mesylate blocks the development of hyperalgesia produced by capsaicin in rats. Pain 81, 25–33. doi: 10.1016/S0304-3959(98)00263-2
Lim, G., Sung, B., Ji, R. R., and Mao, J. (2003). Upregulation of spinal cannabinoid-1-receptors following nerve injury enhances the effects of Win 55,212-2 on neuropathic pain behaviors in rats. Pain 105, 275–283. doi: 10.1016/S0304-3959(03)00242-2
Llorca-Torralba, M., Borges, G., Neto, F., Mico, J. A., and Berrocoso, E. (2016). Noradrenergic Locus coeruleus pathways in pain modulation. Neuroscience 338, 93–113. doi: 10.1016/j.neuroscience.2016.05.057
Lo Verme, J., Fu, J., Astarita, G., La Rana, G., Russo, R., Calignano, A., et al. (2005). The nuclear receptor peroxisome proliferator-activated receptor-alpha mediates the anti-inflammatory actions of palmitoylethanolamide. Mol. Pharmacol. 67, 15–19. doi: 10.1124/mol.104.006353
Loyd, D. R., and Murphy, A. Z. (2009). The role of the periaqueductal gray in the modulation of pain in males and females: are the anatomy and physiology really that different? Neural Plast. 2009:462879. doi: 10.1155/2009/462879
Lu, V. B., Puhl, H. L. III, and Ikeda, S. R. (2013). N-Arachidonyl glycine does not activate G protein-coupled receptor 18 signaling via canonical pathways. Mol. Pharmacol. 83, 267–282. doi: 10.1124/mol.112.081182
Luchicchi, A., and Pistis, M. (2012). Anandamide and 2-arachidonoylglycerol: pharmacological properties, functional features, and emerging specificities of the two major endocannabinoids. Mol. Neurobiol. 46, 374–392. doi: 10.1007/s12035-012-8299-0
Lynch, M. E., Cesar-Rittenberg, P., and Hohmann, A. G. (2014). A double-blind, placebo-controlled, crossover pilot trial with extension using an oral mucosal cannabinoid extract for treatment of chemotherapy-induced neuropathic pain. J. Pain Symptom Manage. 47, 166–173. doi: 10.1016/j.jpainsymman.2013.02.018
Ma, H., Chen, S. R., Chen, H., Li, L., Li, D. P., Zhou, J. J., et al. (2018). Alpha2delta-1 is essential for sympathetic output and NMDA receptor activity potentiated by angiotensin II in the hypothalamus. J. Neurosci. 38, 6388–6398. doi: 10.1523/JNEUROSCI.0447-18.2018
Mackie, K. (2005). Cannabinoid receptor homo- and heterodimerization. Life Sci. 77, 1667–1673. doi: 10.1016/j.lfs.2005.05.011
Mackie, K. (2008a). Cannabinoid receptors: where they are and what they do. J. Neuroendocrinol. 20(Suppl. 1), 10–14. doi: 10.1111/j.1365-2826.2008.01671.x
Mackie, K. (2008b). Signaling via CNS cannabinoid receptors. Mol. Cell. Endocrinol. 286, S60–S65. doi: 10.1016/j.mce.2008.01.022
Maingret, F., Patel, A. J., Lesage, F., Lazdunski, M., and Honore, E. (2000). Lysophospholipids open the two-pore domain mechano-gated K+ channels TREK-1 and TRAAK. J. Biol. Chem. 275, 10128–10133. doi: 10.1074/jbc.275.14.10128
Maione, S., Costa, B., Piscitelli, F., Morera, E., De Chiaro, M., Comelli, F., et al. (2013). Piperazinyl carbamate fatty acid amide hydrolase inhibitors and transient receptor potential channel modulators as =“dual-target” analgesics. Pharmacol. Res. 76, 98–105. doi: 10.1016/j.phrs.2013.07.003
Maione, S., De Petrocellis, L., De Novellis, V., Moriello, A. S., Petrosino, S., Palazzo, E., et al. (2007). Analgesic actions of N-arachidonoyl-serotonin, a fatty acid amide hydrolase inhibitor with antagonistic activity at vanilloid TRPV1 receptors. Br. J. Pharmacol. 150, 766–781. doi: 10.1038/sj.bjp.0707145
Malan, T. P. Jr., Ibrahim, M. M., Vanderah, T. W., Makriyannis, A., and Porreca, F. (2002). Inhibition of pain responses by activation of CB2 cannabinoid receptors. Chem. Phys. Lipids 121, 191–200. doi: 10.1016/S0009-3084(02)00155-X
Malan, T. P. Jr., Ibrahim, M. M., Deng, H., Liu, Q., Mata, H. P., Vanderah, T., et al. (2001). CB2 cannabinoid receptor-mediated peripheral antinociception. Pain 93, 239–245. doi: 10.1016/S0304-3959(01)00321-9
Malek, N., Kostrzewa, M., Makuch, W., Pajak, A., Kucharczyk, M., Piscitelli, F., et al. (2016). The multiplicity of spinal AA-5-HT anti-nociceptive action in a rat model of neuropathic pain. Pharmacol. Res. 111, 251–263. doi: 10.1016/j.phrs.2016.06.012
Malek, N., Mrugala, M., Makuch, W., Kolosowska, N., Przewlocka, B., Binkowski, M., et al. (2015). A multi-target approach for pain treatment: dual inhibition of fatty acid amide hydrolase and TRPV1 in a rat model of osteoarthritis. Pain 156, 890–903. doi: 10.1097/j.pain.0000000000000132
Manzanares, J., Corchero, J., Romero, J., Fernandez-Ruiz, J. J., Ramos, J. A., and Fuentes, J. A. (1999). Pharmacological and biochemical interactions between opioids and cannabinoids. Trends Pharmacol. Sci. 20, 287–294. doi: 10.1016/S0165-6147(99)01339-5
Marichal-Cancino, B. A., Fajardo-Valdez, A., Ruiz-Contreras, A. E., Méndez-Díaz, M., and Prospero-García, O. (2017). Advances in the physiology of GPR55 in the central nervous system. Curr. Neuropharmacol. 15, 771–778. doi: 10.2174/1570159X14666160729155441
Marichal-Cancino, B. A., Fajardo-Valdez, A., Ruiz-Contreras, A. E., Méndez-Díaz, M., and Prospero-García, O. (2018). Possible role of hippocampal GPR55 in spatial learning and memory in rats. Acta Neurobiol. Exp. 78, 41–50. doi: 10.21307/ane-2018-001
Marichal-Cancino, B. A., Manrique-Maldonado, G., Altamirano-Espinoza, A. H., Ruiz-Salinas, I., González-Hernández, A., Maassenvandenbrink, A., et al. (2013). Analysis of anandamide- and lysophosphatidylinositol-induced inhibition of the vasopressor responses produced by sympathetic stimulation or noradrenaline in pithed rats. Eur. J. Pharmacol. 721, 168–177. doi: 10.1016/j.ejphar.2013.09.039
Marichal-Cancino, B. A., Sánchez-Fuentes, A., Méndez-Díaz, M., Ruiz-Contreras, A. E., and Prospero-García, O. (2016). Blockade of GPR55 in the dorsolateral striatum impairs performance of rats in a T-maze paradigm. Behav. Pharmacol. 27, 393–396. doi: 10.1097/FBP.0000000000000185
Martell, B. A., O’connor, P. G., Kerns, R. D., Becker, W. C., Morales, K. H., Kosten, T. R., et al. (2007). Systematic review: opioid treatment for chronic back pain: prevalence, efficacy, and association with addiction. Ann. Intern. Med. 146, 116–127. doi: 10.7326/0003-4819-146-2-200701160-00006
Martin-Sanchez, E., Furukawa, T. A., Taylor, J., and Martin, J. L. (2009). Systematic review and meta-analysis of cannabis treatment for chronic pain. Pain Med. 10, 1353–1368. doi: 10.1111/j.1526-4637.2009.00703.x
Mason, P. (2001). Contributions of the medullary raphe and ventromedial reticular region to pain modulation and other homeostatic functions. Annu. Rev. Neurosci. 24, 737–777. doi: 10.1146/annurev.neuro.24.1.737
Massi, P., Vaccani, A., Rubino, T., and Parolaro, D. (2003). Cannabinoids and opioids share cAMP pathway in rat splenocytes. J. Neuroimmunol. 145, 46–54. doi: 10.1016/j.jneuroim.2003.09.006
McGaraughty, S., Chu, K. L., Dart, M. J., Yao, B. B., and Meyer, M. D. (2009). A CB2 receptor agonist, A-836339, modulates wide dynamic range neuronal activity in neuropathic rats: contributions of spinal and peripheral CB2 receptors. Neuroscience 158, 1652–1661. doi: 10.1016/j.neuroscience.2008.11.015
McHugh, D., Hu, S. S., Rimmerman, N., Juknat, A., Vogel, Z., Walker, J. M., et al. (2010). N-arachidonoyl glycine, an abundant endogenous lipid, potently drives directed cellular migration through GPR18, the putative abnormal cannabidiol receptor. BMC Neurosci. 11:44. doi: 10.1186/1471-2202-11-44
Mechoulam, R., Ben-Shabat, S., Hanus, L., Ligumsky, M., Kaminski, N. E., Schatz, A. R., et al. (1995). Identification of an endogenous 2-monoglyceride, present in canine gut, that binds to cannabinoid receptors. Biochem. Pharmacol. 50, 83–90. doi: 10.1016/0006-2952(95)00109-D
Meng, I. D., Manning, B. H., Martin, W. J., and Fields, H. L. (1998). An analgesia circuit activated by cannabinoids. Nature 395, 381–383. doi: 10.1038/26481
Merriam, F. V., Wang, Z. Y., Guerios, S. D., and Bjorling, D. E. (2008). Cannabinoid receptor 2 is increased in acutely and chronically inflamed bladder of rats. Neurosci. Lett. 445, 130–134. doi: 10.1016/j.neulet.2008.08.076
Mico, J. A., Ardid, D., Berrocoso, E., and Eschalier, A. (2006). Antidepressants and pain. Trends Pharmacol. Sci. 27, 348–354. doi: 10.1016/j.tips.2006.05.004
Mika, J., Zychowska, M., Makuch, W., Rojewska, E., and Przewlocka, B. (2013). Neuronal and immunological basis of action of antidepressants in chronic pain - clinical and experimental studies. Pharmacol. Rep. 65, 1611–1621. doi: 10.1016/S1734-1140(13)71522-6
Miller, S., Leishman, E., Oehler, O., Daily, L., Murataeva, N., Wager-Miller, J., et al. (2016). Evidence for a GPR18 role in diurnal Regulation of intraocular pressure. Invest. Ophthalmol. Vis. Sci. 57, 6419–6426. doi: 10.1167/iovs.16-19437
Millns, P. J., Chapman, V., and Kendall, D. A. (2001). Cannabinoid inhibition of the capsaicin-induced calcium response in rat dorsal root ganglion neurones. Br. J. Pharmacol. 132, 969–971. doi: 10.1038/sj.bjp.0703919
Moffat, R., and Rae, C. P. (2011). Anatomy, physiology and pharmacology of pain. Anaesth. Intensive Care Med. 12, 12–15. doi: 10.1016/j.mpaic.2010.10.011
Monet, M., Gkika, D., Lehen’kyi, V., Pourtier, A., Vanden Abeele, F., Bidaux, G., et al. (2009). Lysophospholipids stimulate prostate cancer cell migration via TRPV2 channel activation. Biochim. Biophys. Acta 1793, 528–539. doi: 10.1016/j.bbamcr.2009.01.003
Morera, E., De Petrocellis, L., Morera, L., Moriello, A. S., Ligresti, A., Nalli, M., et al. (2009). Synthesis and biological evaluation of piperazinyl carbamates and ureas as fatty acid amide hydrolase (FAAH) and transient receptor potential (TRP) channel dual ligands. Bioorg. Med. Chem. Lett. 19, 6806–6809. doi: 10.1016/j.bmcl.2009.09.033
Munro, S., Thomas, K. L., and Abu-Shaar, M. (1993). Molecular characterization of a peripheral receptor for cannabinoids. Nature 365, 61–65. doi: 10.1038/365061a0
Narang, S., Gibson, D., Wasan, A. D., Ross, E. L., Michna, E., Nedeljkovic, S. S., et al. (2008). Efficacy of dronabinol as an adjuvant treatment for chronic pain patients on opioid therapy. J. Pain 9, 254–264. doi: 10.1016/j.jpain.2007.10.018
Niederberger, E., and Geisslinger, G. (2008). The IKK-NF-κB pathway: a source for novel molecular drug targets in pain therapy? FASEB J. 22, 3432–3442. doi: 10.1096/fj.08-109355
Nurmikko, T. J., Serpell, M. G., Hoggart, B., Toomey, P. J., Morlion, B. J., and Haines, D. (2007). Sativex successfully treats neuropathic pain characterised by allodynia: a randomised, double-blind, placebo-controlled clinical trial. Pain 133, 210–220. doi: 10.1016/j.pain.2007.08.028
Oka, S., Kimura, S., Toshida, T., Ota, R., Yamashita, A., and Sugiura, T. (2010). Lysophosphatidylinositol induces rapid phosphorylation of p38 mitogen-activated protein kinase and activating transcription factor 2 in HEK293 cells expressing GPR55 and IM-9 lymphoblastoid cells. J. Biochem. 147, 671–678. doi: 10.1093/jb/mvp208
Oka, S., Nakajima, K., Yamashita, A., Kishimoto, S., and Sugiura, T. (2007). Identification of GPR55 as a lysophosphatidylinositol receptor. Biochem. Biophys. Res. Commun. 362, 928–934. doi: 10.1016/j.bbrc.2007.08.078
Okamoto, Y., Wang, J., Morishita, J., and Ueda, N. (2007). Biosynthetic pathways of the endocannabinoid anandamide. Chem. Biodivers. 4, 1842–1857. doi: 10.1002/cbdv.200790155
Okine, B. N., Madasu, M. K., Mcgowan, F., Prendergast, C., Gaspar, J. C., Harhen, B., et al. (2016). N-palmitoylethanolamide in the anterior cingulate cortex attenuates inflammatory pain behaviour indirectly via a CB1 receptor-mediated mechanism. Pain 157, 2687–2696. doi: 10.1097/j.pain.0000000000000687
Onaivi, E. S., Ishiguro, H., Gong, J. P., Patel, S., Perchuk, A., Meozzi, P. A., et al. (2006). Discovery of the presence and functional expression of cannabinoid CB2 receptors in brain. Ann. N. Y. Acad. Sci. 1074, 514–536. doi: 10.1196/annals.1369.052
Oshita, K., Inoue, A., Tang, H. B., Nakata, Y., Kawamoto, M., and Yuge, O. (2005). CB(1) cannabinoid receptor stimulation modulates transient receptor potential vanilloid receptor 1 activities in calcium influx and substance P Release in cultured rat dorsal root ganglion cells. J. Pharmacol. Sci. 97, 377–385. doi: 10.1254/jphs.FP0040872
Otto, M., Bach, F. W., Jensen, T. S., and Sindrup, S. H. (2004). Valproic acid has no effect on pain in polyneuropathy: a randomized, controlled trial. Neurology 62, 285–288. doi: 10.1212/WNL.62.2.285
Park, C. K., Xu, Z. Z., Liu, T., Lu, N., Serhan, C. N., and Ji, R. R. (2011). Resolvin D2 is a potent endogenous inhibitor for transient receptor potential subtype V1/A1, inflammatory pain, and spinal cord synaptic plasticity in mice: distinct roles of resolvin D1, D2, and E1. J. Neurosci. 31, 18433–18438. doi: 10.1523/JNEUROSCI.4192-11.2011
Patel, H. J., Birrell, M. A., Crispino, N., Hele, D. J., Venkatesan, P., Barnes, P. J., et al. (2003). Inhibition of guinea-pig and human sensory nerve activity and the cough reflex in guinea-pigs by cannabinoid (CB2) receptor activation. Br. J. Pharmacol. 140, 261–268. doi: 10.1038/sj.bjp.0705435
Pertwee, R. G. (2009). Emerging strategies for exploiting cannabinoid receptor agonists as medicines. Br. J. Pharmacol. 156, 397–411. doi: 10.1111/j.1476-5381.2008.00048.x
Pertwee, R. G., Howlett, A. C., Abood, M. E., Alexander, S. P. H., Di Marzo, V., Elphick, M. R., et al. (2010). International Union of Basic and Clinical Pharmacology. LXXIX. Cannabinoid receptors and their ligands: beyond CB1 and CB2. Pharmacol. Rev. 62, 588–631. doi: 10.1124/pr.110.003004
Pineiro, R., Maffucci, T., and Falasca, M. (2011). The putative cannabinoid receptor GPR55 defines a novel autocrine loop in cancer cell proliferation. Oncogene 30, 142–152. doi: 10.1038/onc.2010.417
Pini, L. A., Guerzoni, S., Cainazzo, M. M., Ferrari, A., Sarchielli, P., Tiraferri, I., et al. (2012). Nabilone for the treatment of medication overuse headache: results of a preliminary double-blind, active-controlled, randomized trial. J. Headache Pain 13, 677–684. doi: 10.1007/s10194-012-0490-1
Piomelli, D. (2003). The molecular logic of endocannabinoid signalling. Nat. Rev. Neurosci. 4, 873–884. doi: 10.1038/nrn1247
Porter, A. C., Sauer, J. M., Knierman, M. D., Becker, G. W., Berna, M. J., Bao, J., et al. (2002). Characterization of a novel endocannabinoid, virodhamine, with antagonist activity at the CB1 receptor. J. Pharmacol. Exp. Ther. 301, 1020–1024. doi: 10.1124/jpet.301.3.1020
Price, T. J., Helesic, G., Parghi, D., Hargreaves, K. M., and Flores, C. M. (2003). The neuronal distribution of cannabinoid receptor type 1 in the trigeminal ganglion of the rat. Neuroscience 120, 155–162. doi: 10.1016/S0306-4522(03)00333-6
Pugh, G. Jr., Smith, P. B., Dombrowski, D. S., and Welch, S. P. (1996). The role of endogenous opioids in enhancing the antinociception produced by the combination of delta 9-tetrahydrocannabinol and morphine in the spinal cord. J. Pharmacol. Exp. Ther. 279, 608–616.
Quartilho, A., Mata, H. P., Ibrahim, M. M., Vanderah, T. W., Porreca, F., Makriyannis, A., et al. (2003). Inhibition of inflammatory hyperalgesia by activation of peripheral CB2 cannabinoid receptors. Anesthesiology 99, 955–960. doi: 10.1097/00000542-200310000-00031
Quintero, G. C. (2017). Review about gabapentin misuse, interactions, contraindications and side effects. J. Exp. Pharmacol. 9, 13–21. doi: 10.2147/JEP.S124391
Rahn, E. J., and Hohmann, A. G. (2009). Cannabinoids as pharmacotherapies for neuropathic pain: from the bench to the bedside. Neurotherapeutics 6, 713–737. doi: 10.1016/j.nurt.2009.08.002
Rahn, E. J., Thakur, G. A., Wood, J. A., Zvonok, A. M., Makriyannis, A., and Hohmann, A. G. (2011). Pharmacological characterization of AM1710, a putative cannabinoid CB2 agonist from the cannabilactone class: antinociception without central nervous system side-effects. Pharmacol. Biochem. Behav. 98, 493–502. doi: 10.1016/j.pbb.2011.02.024
Rajapakse, C. S., Lisai, M., Deregnaucourt, C., Sinou, V., Latour, C., Roy, D., et al. (2015). Synthesis of new 4-aminoquinolines and evaluation of their in vitro activity against chloroquine-sensitive and chloroquine-resistant plasmodium falciparum. PLoS One 10:e0140878. doi: 10.1371/journal.pone.0140878
Ranganathan, M., and D’Souza, D. C. (2006). The acute effects of cannabinoids on memory in humans: a review. Psychopharmacology 188, 425–444. doi: 10.1007/s00213-006-0508-y
Ray, P., Torck, A., Quigley, L., Wangzhou, A., Neiman, M., Rao, C., et al. (2018). Comparative transcriptome profiling of the human and mouse dorsal root ganglia: an RNA-seq-based resource for pain and sensory neuroscience research. Pain 159, 1325–1345. doi: 10.1097/j.pain.0000000000001217
Ray, W. A., Meredith, S., Thapa, P. B., Hall, K., and Murray, K. T. (2004). Cyclic antidepressants and the risk of sudden cardiac death. Clin. Pharmacol. Ther. 75, 234–241. doi: 10.1016/j.clpt.2003.09.019
Rice, W., Shannon, J. M., Burton, F., and Fiedeldey, D. (1997). Expression of a brain-type cannabinoid receptor (CB1) in alveolar Type II cells in the lung: regulation by hydrocortisone. Eur. J. Pharmacol. 327, 227–232. doi: 10.1016/S0014-2999(97)89665-3
Roa-Coria, J. E., Navarrete-Vázquez, G., Fowler, C. J., Flores-Murrieta, F. J., Déciga-Campos, M., and Granados-Soto, V. (2012). N-(4-Methoxy-2-nitrophenyl)hexadecanamide, a palmitoylethanolamide analogue, reduces formalin-induced nociception. Life Sci. 91, 1288–1294. doi: 10.1016/j.lfs.2012.09.024
Roberts, J. D., Gennings, C., and Shih, M. (2006). Synergistic affective analgesic interaction between delta-9-tetrahydrocannabinol and morphine. Eur. J. Pharmacol. 530, 54–58. doi: 10.1016/j.ejphar.2005.11.036
Roberts, L. A., Christie, M. J., and Connor, M. (2002). Anandamide is a partial agonist at native vanilloid receptors in acutely isolated mouse trigeminal sensory neurons. Br. J. Pharmacol. 137, 421–428. doi: 10.1038/sj.bjp.0704904
Rog, D. J., Nurmikko, T. J., Friede, T., and Young, C. A. (2005). Randomized, controlled trial of cannabis-based medicine in central pain in multiple sclerosis. Neurology 65, 812–819. doi: 10.1212/01.wnl.0000176753.45410.8b
Rosenkilde, M. M., Benned-Jensen, T., Andersen, H., Holst, P. J., Kledal, T. N., Luttichau, H. R., et al. (2006). Molecular pharmacological phenotyping of EBI2. An orphan seven-transmembrane receptor with constitutive activity. J. Biol. Chem. 281, 13199–13208. doi: 10.1074/jbc.M602245200
Ross, R. A. (2003). Anandamide and vanilloid TRPV1 receptors. Br. J. Pharmacol. 140, 790–801. doi: 10.1038/sj.bjp.0705467
Ross, R. A., Coutts, A. A., Mcfarlane, S. M., Anavi-Goffer, S., Irving, A. J., Pertwee, R. G., et al. (2001). Actions of cannabinoid receptor ligands on rat cultured sensory neurones: implications for antinociception. Neuropharmacology 40, 221–232. doi: 10.1016/S0028-3908(00)00135-0
Ryberg, E., Larsson, N., Sjogren, S., Hjorth, S., Hermansson, N. O., Leonova, J., et al. (2007). The orphan receptor GPR55 is a novel cannabinoid receptor. Br. J. Pharmacol. 152, 1092–1101. doi: 10.1038/sj.bjp.0707460
Sagar, D. R., Jhaveri, M., and Chapman, V. (2009). Targeting the cannabinoid system to produce analgesia. Curr. Top. Behav. Neurosci. 1, 275–287. doi: 10.1007/978-3-540-88955-7_11
Salio, C., Fischer, J., Franzoni, M. F., and Conrath, M. (2002). Pre- and postsynaptic localizations of the CB1 cannabinoid receptor in the dorsal horn of the rat spinal cord. Neuroscience 110, 755–764. doi: 10.1016/S0306-4522(01)00584-X
Santha, P., Jenes, A., Somogyi, C., and Nagy, I. (2010). The endogenous cannabinoid anandamide inhibits transient receptor potential vanilloid type 1 receptor-mediated currents in rat cultured primary sensory neurons. Acta Physiol. Hung. 97, 149–158. doi: 10.1556/APhysiol.97.2010.2.1
Sanudo-Pena, M. C., Strangman, N. M., Mackie, K., Walker, J. M., and Tsou, K. (1999). CB1 receptor localization in rat spinal cord and roots, dorsal root ganglion, and peripheral nerve. Zhongguo Yao Li Xue Bao 20, 1115–1120.
Scavone, J. L., Sterling, R. C., and Van Bockstaele, E. J. (2013). Cannabinoid and opioid interactions: implications for opiate dependence and withdrawal. Neuroscience 248, 637–654. doi: 10.1016/j.neuroscience.2013.04.034
Schicho, R., Bashashati, M., Bawa, M., Mchugh, D., Saur, D., Hu, H. M., et al. (2011). The atypical cannabinoid O-1602 protects against experimental colitis and inhibits neutrophil recruitment. Inflamm. Bowel Dis. 17, 1651–1664. doi: 10.1002/ibd.21538
Schuelert, N., and McDougall, J. J. (2011). The abnormal cannabidiol analogue O-1602 reduces nociception in a rat model of acute arthritis via the putative cannabinoid receptor GPR55. Neurosci. Lett. 500, 72–76. doi: 10.1016/j.neulet.2011.06.004
Schuelert, N., Zhang, C., Mogg, A. J., Broad, L. M., Hepburn, D. L., Nisenbaum, E. S., et al. (2010). Paradoxical effects of the cannabinoid CB2 receptor agonist GW405833 on rat osteoarthritic knee joint pain. Osteoarthritis Cartilage 18, 1536–1543. doi: 10.1016/j.joca.2010.09.005
Serpell, M., Ratcliffe, S., Hovorka, J., Schofield, M., Taylor, L., Lauder, H., et al. (2014). A double-blind, randomized, placebo-controlled, parallel group study of THC/CBD spray in peripheral neuropathic pain treatment. Eur. J. Pain 18, 999–1012. doi: 10.1002/j.1532-2149.2013.00445.x
Shiue, S. J., Peng, H. Y., Lin, C. R., Wang, S. W., Rau, R. H., and Cheng, J. K. (2017). Continuous intrathecal infusion of cannabinoid receptor agonists attenuates nerve ligation-induced pain in rats. Reg. Anesth. Pain Med. 42, 499–506. doi: 10.1097/AAP.0000000000000601
Silver, M., Blum, D., Grainger, J., Hammer, A. E., and Quessy, S. (2007). Double-blind, placebo-controlled trial of lamotrigine in combination with other medications for neuropathic pain. J. Pain Symptom Manage. 34, 446–454. doi: 10.1016/j.jpainsymman.2006.12.015
Simon, G. M., and Cravatt, B. F. (2006). Endocannabinoid biosynthesis proceeding through glycerophospho-N-acyl ethanolamine and a role for alpha/beta-hydrolase 4 in this pathway. J. Biol. Chem. 281, 26465–26472. doi: 10.1074/jbc.M604660200
Sinha, S., Schreiner, A. J., Biernaskie, J., Nickerson, D., and Gabriel, V. A. (2017). Treating pain on skin graft donor sites: review and clinical recommendations. J. Trauma Acute Care Surg. 83, 954–964. doi: 10.1097/TA.0000000000001615
Skrabek, R. Q., Galimova, L., Ethans, K., and Perry, D. (2008). Nabilone for the treatment of pain in fibromyalgia. J. Pain 9, 164–173. doi: 10.1016/j.jpain.2007.09.002
Smart, D., Gunthorpe, M. J., Jerman, J. C., Nasir, S., Gray, J., Muir, A. I., et al. (2000). The endogenous lipid anandamide is a full agonist at the human vanilloid receptor (hVR1). Br. J. Pharmacol. 129, 227–230. doi: 10.1038/sj.bjp.0703050
Smith, F. L., Fujimori, K., Lowe, J., and Welch, S. P. (1998). Characterization of delta9-tetrahydrocannabinol and anandamide antinociception in nonarthritic and arthritic rats. Pharmacol. Biochem. Behav. 60, 183–191. doi: 10.1016/S0091-3057(97)00583-2
Snider, W. D., and McMahon, S. B. (1998). Tackling pain at the source: new ideas about nociceptors. Neuron 20, 629–632. doi: 10.1016/S0896-6273(00)81003-X
Sousa-Valente, J., Varga, A., Ananthan, K., Khajuria, A., and Nagy, I. (2014). Anandamide in primary sensory neurons: too much of a good thing? Eur. J. Neurosci. 39, 409–418. doi: 10.1111/ejn.12467
Stahl, S. M., Grady, M. M., Moret, C., and Briley, M. (2005). SNRIs: their pharmacology, clinical efficacy, and tolerability in comparison with other classes of antidepressants. CNS Spectr. 10, 732–747. doi: 10.1017/S1092852900019726
Staton, P. C., Hatcher, J. P., Walker, D. J., Morrison, A. D., Shapland, E. M., Hughes, J. P., et al. (2008). The putative cannabinoid receptor GPR55 plays a role in mechanical hyperalgesia associated with inflammatory and neuropathic pain. Pain 139, 225–236. doi: 10.1016/j.pain.2008.04.006
Stein, A. T., Ufret-Vincenty, C. A., Hua, L., Santana, L. F., and Gordon, S. E. (2006). Phosphoinositide 3-kinase binds to TRPV1 and mediates NGF-stimulated TRPV1 trafficking to the plasma membrane. J. Gen. Physiol. 128, 509–522. doi: 10.1085/jgp.200609576
Stella, N., Schweitzer, P., and Piomelli, D. (1997). A second endogenous cannabinoid that modulates long-term potentiation. Nature 388, 773–778. doi: 10.1038/42015
Stevens, A. J., and Higgins, M. D. (2017). A systematic review of the analgesic efficacy of cannabinoid medications in the management of acute pain. Acta Anaesthesiol. Scand. 61, 268–280. doi: 10.1111/aas.12851
Straiker, A., Wager-Miller, J., Hutchens, J., and Mackie, K. (2012). Differential signalling in human cannabinoid CB1 receptors and their splice variants in autaptic hippocampal neurones. Br. J. Pharmacol. 165, 2660–2671. doi: 10.1111/j.1476-5381.2011.01744.x
Succar, R., Mitchell, V. A., and Vaughan, C. W. (2007). Actions of N-arachidonyl-glycine in a rat inflammatory pain model. Mol. Pain 3:24. doi: 10.1186/1744-8069-3-24
Sugiura, T., Kondo, S., Sukagawa, A., Nakane, S., Shinoda, A., Itoh, K., et al. (1995). 2-Arachidonoylglycerol: a possible endogenous cannabinoid receptor ligand in brain. Biochem. Biophys. Res. Commun. 215, 89–97. doi: 10.1006/bbrc.1995.2437
Sugiura, T., and Waku, K. (2000). 2-arachidonoylglycerol: a possible multifunctional lipid mediator in the nervous and immune systems. Ann. N. Y. Acad. Sci. 905, 344–346. doi: 10.1111/j.1749-6632.2000.tb06575.x
Svendsen, K. B., Jensen, T. S., and Bach, F. W. (2004). Does the cannabinoid dronabinol reduce central pain in multiple sclerosis? Randomised double blind placebo controlled crossover trial. BMJ 329:253. doi: 10.1136/bmj.38149.566979.AE
Takenouchi, R., Inoue, K., Kambe, Y., and Miyata, A. (2012). N-arachidonoyl glycine induces macrophage apoptosis via GPR18. Biochem. Biophys. Res. Commun. 418, 366–371. doi: 10.1016/j.bbrc.2012.01.027
Tognetto, M., Amadesi, S., Harrison, S., Creminon, C., Trevisani, M., Carreras, M., et al. (2001). Anandamide excites central terminals of dorsal root ganglion neurons via vanilloid receptor-1 activation. J. Neurosci. 21, 1104–1109. doi: 10.1523/JNEUROSCI.21-04-01104.2001
Toth, C., Mawani, S., Brady, S., Chan, C., Liu, C., Mehina, E., et al. (2012). An enriched-enrolment, randomized withdrawal, flexible-dose, double-blind, placebo-controlled, parallel assignment efficacy study of nabilone as adjuvant in the treatment of diabetic peripheral neuropathic pain. Pain 153, 2073–2082. doi: 10.1016/j.pain.2012.06.024
Touw, M. (1981). The religious and medicinal uses of Cannabis in China, India and Tibet. J. Psychoactive Drugs 13, 23–34. doi: 10.1080/02791072.1981.10471447
Turcotte, D., Le Dorze, J. A., Esfahani, F., Frost, E., Gomori, A., and Namaka, M. (2010). Examining the roles of cannabinoids in pain and other therapeutic indications: a review. Expert Opin. Pharmacother. 11, 17–31. doi: 10.1517/14656560903413534
Ueda, N., Tsuboi, K., Uyama, T., and Ohnishi, T. (2011). Biosynthesis and degradation of the endocannabinoid 2-arachidonoylglycerol. Biofactors 37, 1–7. doi: 10.1002/biof.131
Valenzano, K. J., Tafesse, L., Lee, G., Harrison, J. E., Boulet, J. M., Gottshall, S. L., et al. (2005). Pharmacological and pharmacokinetic characterization of the cannabinoid receptor 2 agonist, GW405833, utilizing rodent models of acute and chronic pain, anxiety, ataxia and catalepsy. Neuropharmacology 48, 658–672. doi: 10.1016/j.neuropharm.2004.12.008
Valverde, O., Noble, F., Beslot, F., Dauge, V., Fournie-Zaluski, M. C., and Roques, B. P. (2001). Delta9-tetrahydrocannabinol releases and facilitates the effects of endogenous enkephalins: reduction in morphine withdrawal syndrome without change in rewarding effect. Eur. J. Neurosci. 13, 1816–1824. doi: 10.1046/j.0953-816x.2001.01558.x
van Amerongen, G., Kanhai, K., Baakman, A. C., Heuberger, J., Klaassen, E., Beumer, T. L., et al. (2017). Effects on spasticity and neuropathic pain of an oral formulation of delta9-tetrahydrocannabinol in patients with progressive multiple sclerosis. Clin. Ther. 40, 1467–1482. doi: 10.1016/j.clinthera.2017.01.016
Vanden Abeele, F., Zholos, A., Bidaux, G., Shuba, Y., Thebault, S., Beck, B., et al. (2006). Ca2+-independent phospholipase A2-dependent gating of TRPM8 by lysophospholipids. J. Biol. Chem. 281, 40174–40182. doi: 10.1074/jbc.M605779200
Vanegas, H., and Schaible, H. G. (2004). Descending control of persistent pain: inhibitory or facilitatory? Brain Res. Brain Res. Rev. 46, 295–309. doi: 10.1016/j.brainresrev.2004.07.004
Vasileiou, I., Fotopoulou, G., Matzourani, M., Patsouris, E., and Theocharis, S. (2013). Evidence for the involvement of cannabinoid receptors’ polymorphisms in the pathophysiology of human diseases. Expert Opin. Ther. Targets 17, 363–377. doi: 10.1517/14728222.2013.754426
Vassilatis, D. K., Hohmann, J. G., Zeng, H., Li, F., Ranchalis, J. E., Mortrud, M. T., et al. (2003). The G protein-coupled receptor repertoires of human and mouse. Proc. Natl. Acad. Sci. U.S.A. 100, 4903–4908. doi: 10.1073/pnas.0230374100
Veress, G., Meszar, Z., Muszil, D., Avelino, A., Matesz, K., Mackie, K., et al. (2013). Characterisation of cannabinoid 1 receptor expression in the perikarya, and peripheral and spinal processes of primary sensory neurons. Brain Struct. Funct. 218, 733–750. doi: 10.1007/s00429-012-0425-2
Vestergaard, K., Andersen, G., Gottrup, H., Kristensen, B. T., and Jensen, T. S. (2001). Lamotrigine for central poststroke pain: a randomized controlled trial. Neurology 56, 184–190. doi: 10.1212/WNL.56.2.184
Vizi, E. S., Katona, I., and Freund, T. F. (2001). Evidence for presynaptic cannabinoid CB1 receptor-mediated inhibition of noradrenaline release in the guinea pig lung. Eur. J. Pharmacol. 431, 237–244. doi: 10.1016/S0014-2999(01)01413-3
Vuong, L. A., Mitchell, V. A., and Vaughan, C. W. (2008). Actions of N-arachidonyl-glycine in a rat neuropathic pain model. Neuropharmacology 54, 189–193. doi: 10.1016/j.neuropharm.2007.05.004
Waldeck-Weiermair, M., Zoratti, C., Osibow, K., Balenga, N., Goessnitzer, E., Waldhoer, M., et al. (2008). Integrin clustering enables anandamide-induced Ca2+ signaling in endothelial cells via GPR55 by protection against CB1-receptor-triggered repression. J. Cell Sci. 121, 1704–1717. doi: 10.1242/jcs.020958
Walker, J. M., and Hohmann, A. G. (2005). Cannabinoid mechanisms of pain suppression. Handb. Exp. Pharmacol. 168, 509–554. doi: 10.1007/3-540-26573-2_17
Walker, J. M., and Huang, S. M. (2002). Cannabinoid analgesia. Pharmacol. Ther. 95, 127–135. doi: 10.1016/S0163-7258(02)00252-8
Wallace, M., Schulteis, G., Atkinson, J. H., Wolfson, T., Lazzaretto, D., Bentley, H., et al. (2007). Dose-dependent effects of smoked cannabis on capsaicin-induced pain and hyperalgesia in healthy volunteers. Anesthesiology 107, 785–796. doi: 10.1097/01.anes.0000286986.92475.b7
Wallace, M. S., Marcotte, T. D., Umlauf, A., Gouaux, B., and Atkinson, J. H. (2015). Efficacy of inhaled cannabis on painful diabetic neuropathy. J. Pain 16, 616–627. doi: 10.1016/j.jpain.2015.03.008
Wang, S., Lim, G., Mao, J., Sung, B., Yang, L., and Mao, J. (2007). Central glucocorticoid receptors regulate the upregulation of spinal cannabinoid-1 receptors after peripheral nerve injury in rats. Pain 131, 96–105. doi: 10.1016/j.pain.2006.12.019
Wang, Z. Y., Mcdowell, T., Wang, P., Alvarez, R., Gomez, T., and Bjorling, D. E. (2014). Activation of CB1 inhibits NGF-induced sensitization of TRPV1 in adult mouse afferent neurons. Neuroscience 277, 679–689. doi: 10.1016/j.neuroscience.2014.07.041
Whiting, P. F., Wolff, R. F., Deshpande, S., Di Nisio, M., Duffy, S., Hernandez, A. V., et al. (2015). Cannabinoids for medical use: a systematic review and meta-analysis. JAMA 313, 2456–2473. doi: 10.1001/jama.2015.6358
Wiles, A. L., Pearlman, R. J., Rosvall, M., Aubrey, K. R., and Vandenberg, R. J. (2006). N-Arachidonyl-glycine inhibits the glycine transporter, GLYT2a. J. Neurochem. 99, 781–786. doi: 10.1111/j.1471-4159.2006.04107.x
Wilsey, B., Marcotte, T., Deutsch, R., Gouaux, B., Sakai, S., and Donaghe, H. (2013). Low-dose vaporized cannabis significantly improves neuropathic pain. J. Pain 14, 136–148. doi: 10.1016/j.jpain.2012.10.009
Wotherspoon, G., Fox, A., Mcintyre, P., Colley, S., Bevan, S., and Winter, J. (2005). Peripheral nerve injury induces cannabinoid receptor 2 protein expression in rat sensory neurons. Neuroscience 135, 235–245. doi: 10.1016/j.neuroscience.2005.06.009
Yamamoto, W., Mikami, T., and Iwamura, H. (2008). Involvement of central cannabinoid CB2 receptor in reducing mechanical allodynia in a mouse model of neuropathic pain. Eur. J. Pharmacol. 583, 56–61. doi: 10.1016/j.ejphar.2008.01.010
Yao, B. B., Hsieh, G. C., Frost, J. M., Fan, Y., Garrison, T. R., Daza, A. V., et al. (2008). In vitro and in vivo characterization of A-796260: a selective cannabinoid CB2 receptor agonist exhibiting analgesic activity in rodent pain models. Br. J. Pharmacol. 153, 390–401. doi: 10.1038/sj.bjp.0707568
Yin, H., Chu, A., Li, W., Wang, B., Shelton, F., Otero, F., et al. (2009). Lipid G protein-coupled receptor ligand identification using beta-arrestin PathHunter assay. J. Biol. Chem. 284, 12328–12338. doi: 10.1074/jbc.M806516200
Yrjola, S., Kalliokoski, T., Laitinen, T., Poso, A., Parkkari, T., and Nevalainen, T. (2013). Discovery of novel cannabinoid receptor ligands by a virtual screening approach: further development of 2,4,6-trisubstituted 1,3,5-triazines as CB2 agonists. Eur. J. Pharm. Sci. 48, 9–20. doi: 10.1016/j.ejps.2012.10.020
Yu, X. H., Cao, C. Q., Martino, G., Puma, C., Morinville, A., St-Onge, S., et al. (2010). A peripherally restricted cannabinoid receptor agonist produces robust anti-nociceptive effects in rodent models of inflammatory and neuropathic pain. Pain 151, 337–344. doi: 10.1016/j.pain.2010.07.019
Zhang, M. J., Sansbury, B. E., Hellmann, J., Baker, J. F., Guo, L., Parmer, C. M., et al. (2016). Resolvin D2 enhances postischemic revascularization while resolving inflammation. Circulation 134, 666–680. doi: 10.1161/CIRCULATIONAHA.116.021894
Zhu, W., and Oxford, G. S. (2007). Phosphoinositide-3-kinase and mitogen activated protein kinase signaling pathways mediate acute NGF sensitization of TRPV1. Mol. Cell. Neurosci. 34, 689–700. doi: 10.1016/j.mcn.2007.01.005
Zhuang, Z. Y., Xu, H., Clapham, D. E., and Ji, R. R. (2004). Phosphatidylinositol 3-kinase activates ERK in primary sensory neurons and mediates inflammatory heat hyperalgesia through TRPV1 sensitization. J. Neurosci. 24, 8300–8309. doi: 10.1523/JNEUROSCI.2893-04.2004
Keywords: GPR18, GPR55, endocannabinoid system, cannabinoid receptors, pain
Citation: Guerrero-Alba R, Barragán-Iglesias P, González-Hernández A, Valdez-Moráles EE, Granados-Soto V, Condés-Lara M, Rodríguez MG and Marichal-Cancino BA (2019) Some Prospective Alternatives for Treating Pain: The Endocannabinoid System and Its Putative Receptors GPR18 and GPR55. Front. Pharmacol. 9:1496. doi: 10.3389/fphar.2018.01496
Received: 27 August 2018; Accepted: 07 December 2018;
Published: 08 January 2019.
Edited by:
Luis F. Callado, Universidad del País Vasco, SpainReviewed by:
Styliani Vlachou, Dublin City University, IrelandThomas Heinbockel, Howard University, United States
Copyright © 2019 Guerrero-Alba, Barragán-Iglesias, González-Hernández, Valdez-Moráles, Granados-Soto, Condés-Lara, Rodríguez and Marichal-Cancino. This is an open-access article distributed under the terms of the Creative Commons Attribution License (CC BY). The use, distribution or reproduction in other forums is permitted, provided the original author(s) and the copyright owner(s) are credited and that the original publication in this journal is cited, in accordance with accepted academic practice. No use, distribution or reproduction is permitted which does not comply with these terms.
*Correspondence: Bruno A. Marichal-Cancino, bruno.marichal@edu.uaa.mx; br.marichal@gmail.com