- Centre de la Recherche Nationale Scientifique, Institut des Neurosciences Cellulaires et Intégratives, Université de Strasbourg, Strasbourg, France
Mu opioid receptors modulate a large number of physiological functions. They are in particular involved in the control of pain perception and reward properties. They are also the primary molecular target of opioid drugs and mediate their beneficial analgesic effects, euphoric properties as well as negative side effects such as tolerance and physical dependence. Importantly, mu opioid receptors can physically associate with another receptor to form a novel entity called heteromer that exhibits specific ligand binding, signaling, and trafficking properties. As reviewed here, in vivo physical proximity has now been evidenced for several receptor pairs, subsequent impact of heteromerization on native mu opioid receptor signaling and trafficking identified and a link to behavioral changes established. Selective targeting of heteromers as a tool to modulate mu opioid receptor activity is therefore attracting growing interest and raises hopes for innovative therapeutic strategies.
Introduction
The mu opioid (mu) receptor is a G protein-coupled receptor (GPCR) that neuromodulates several physiological functions, in particular nociception (Kieffer and Evans, 2009). This receptor also mediates the reinforcing properties of natural stimuli. In addition, mu receptors are the primary molecular target of opioid drugs used in the clinic (e.g., morphine, codeine, oxycodone, fentanyl, tramadol), and are responsible for their analgesic properties but also for the side effects associated with their acute (e.g., respiratory depression, nausea, dizziness, sedation, constipation) (Kieffer, 1999) and chronic use (tolerance, hyperalgesia, and physical dependence) (Matthes et al., 1996; Williams et al., 2013). Moreover, mu receptors mediate opioid rewarding and euphoric properties that underlie their abuse potential (Matthes et al., 1996). The latter is at the root of the epidemic that has developed in North America upon misuse and/or abuse of prescription opioid drugs after an initial therapeutic use or in patients that self-medicate (Vowles et al., 2015). It underscores the need for designing effective opioid analgesics devoid of side effects and has prompted considerable efforts to better understand the molecular and cellular mechanisms underlying mu receptor activity. In this context, functional consequences elicited by physical association of the mu receptor with another GPCR attracted attention. Here, we review evidence of molecular, cellular, and behavioral modulation induced by mu receptor heteromerization in native tissue.
Mu Receptor Heteromers in Native Tissue
Heteromers involving the mu receptor have been extensively studied in heterologous systems (Fujita et al., 2014a). Receptor physical proximity has now been established in native tissue for several receptor pairs using receptor co-immunoprecipitation, co-localization by electron microscopy or in situ proximity ligation assay (PLA), and/or disruption of physical contact by an interfering peptide. The use of interfering peptide and/or mice deficient for one receptor also significantly contributed to demonstrate the specificity of the functional changes associated with heteromer formation and to establish a causal link with behavioral outputs. In native tissue, the mu receptor heteromerizes with the delta opioid (delta) or kappa opioid (kappa) receptors or with the non-opioid receptors ORL1, cannabinoid CB1, galanin Gal1, adrenergic α2a, somatostatin sst2, dopamine D1, chemokine CCR5, and vasopressin V1b. Association between the mu receptor splice variant MOR1D and the gastrin-releasing peptide receptor (GRPR) has also been reported as well as mu physical proximity with the ion channel NMDA (Table 1). Heteromerization with a Gαi/o coupled GPCR is thus the most frequently reported to date but association with the Gαq coupled GRPR and vasopressin V1B receptors or the Gαs coupled dopamine D1 receptor indicates no specific requirement. Additional heteromers likely exist in vivo since mu receptor heteromerizes with serotonin 5HT1a (Cussac et al., 2012), neuropeptide FF NPFF2 (Roumy et al., 2007), melanocortin MC3 (Rediger et al., 2009), neurokinin NK1 (Pfeiffer et al., 2003), and possibly, dopamine D4 (Qian et al., 2018) receptors in co-transfected cells, and neuronal co-localization with chemokine CXCR4 (Patel et al., 2006; Heinisch et al., 2011), metabotropic glutamate mGluR5 (Schröder et al., 2009) and serotonin 5HT2a (Lopez-Gimenez et al., 2008), and dopamine D4 (Rivera et al., 2017) receptors has been reported (see also http://www.gpcr-hetnet.com for further information on the GPCR interaction network, and interacting or non-interacting receptor pairs; Borroto-Escuela et al., 2014).
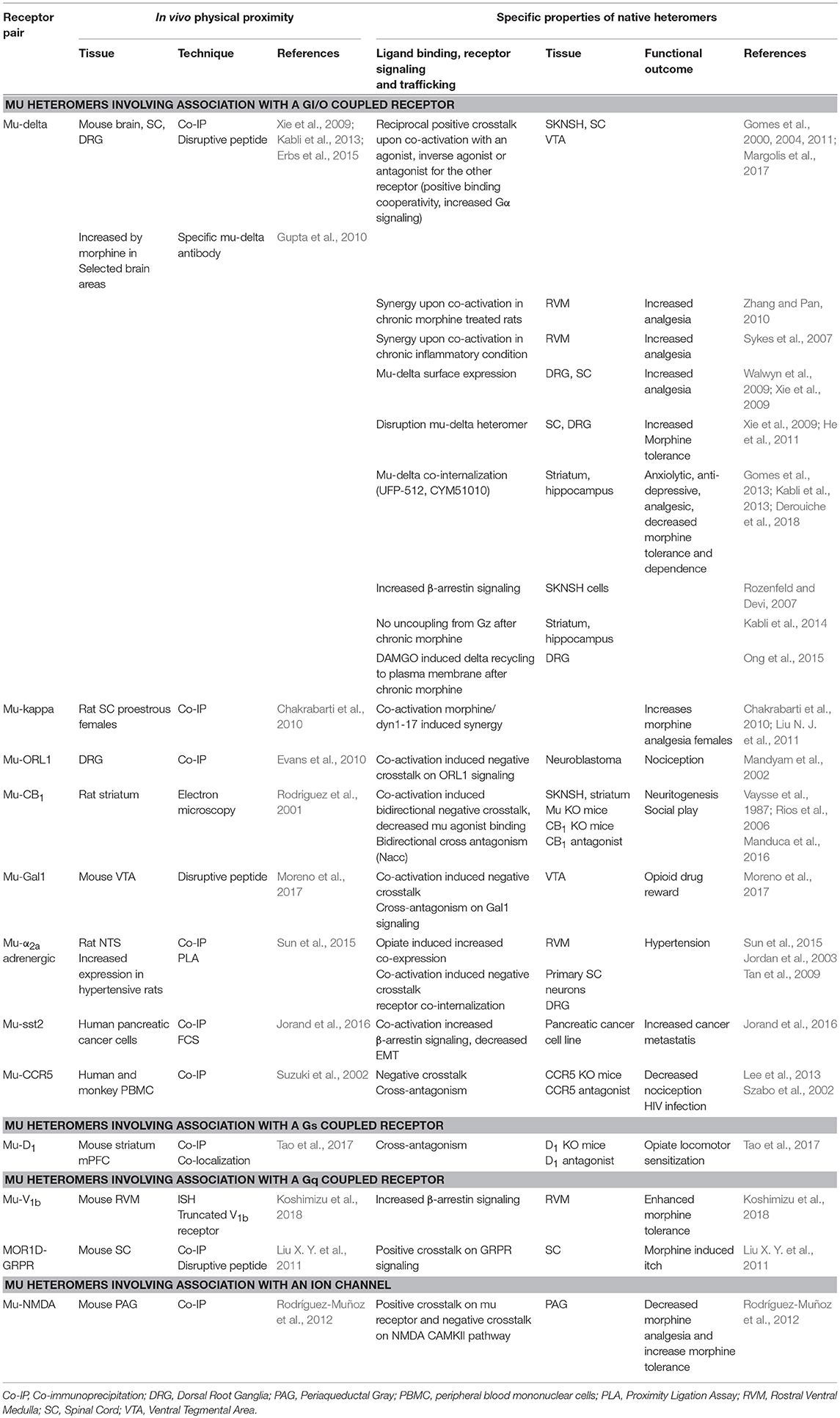
Table 1. Identification, properties, and functional outcome of native heteromers involving the mu opioid receptor.
Expression of native heteromers is dynamic. Chronic morphine treatment enhances mu-delta heteromer density in brain regions associated with the reward pathway (Gupta et al., 2010). Concomitant increase in delta receptor localization at the cell surface is observed and is mu receptor dependent (Gendron et al., 2015; Ong et al., 2015; Erbs et al., 2016). Heteromers form intracellularly in native tissue. In the mouse dorsal root ganglia (DRG), mu and delta opioid receptors associate in the endoplasmic reticulum (ER), which requires phosphorylation of the delta receptor at threonine 161 by the cdk5 kinase (Walwyn et al., 2009; Xie et al., 2009). Mu-delta density could also be affected in other pathological conditions enhancing delta receptor presence at the cell surface such as inflammatory pain conditions (Cahill et al., 2003) or voluntary alcohol consumption (van Rijn et al., 2012). In addition, expression of mu-α2a heteromers in the nucleus of the solitary tract (NTS) is dynamically regulated and increased in hypertensive rats (Sun et al., 2015). In human peripheral blood mononuclear cells (PBMC), the mu agonist DAMGO induced CCR5 receptor synthesis through a TGFβ1 dependent mechanism (Happel et al., 2008), suggesting a role for mu-CCR5 heteromers in HIV1 entry in opiate abusers.
Modulation of G Protein Signaling in Native Mu Heteromers
In SK-N-SH neuroblastoma cells co-expressing mu and delta receptors, occupancy of the binding site of one receptor by a non-signaling concentration of ligand increased binding and Gα signaling of the other receptor (Gomes et al., 2000, 2004, 2011). The nature of the first ligand did not seem important since agonist, antagonist or inverse agonist induced similar effects. Data therefore suggest that mu-delta heteromerization induces cross-allosteric modulation with a positive cooperativity promoted upon binding of the first ligand (Figure 1). Accordingly, co-application of the delta antagonist TIPPψ and mu agonist DAMGO or co-application of the mu antagonist CTAP and delta agonists deltorphin II or DPDPE increased hyperpolarization in a subset of neurons in the ventral tegmental area (VTA) (Margolis et al., 2017). Similarly, co-injection of subthreshold doses of the mu agonist DAMGO and the delta agonist deltorphin II in the rostral ventromedial medulla (RVM) of rats chronically treated with morphine increased γ aminobutyric acid (GABA)ergic inhibition through synergistic activation of the phospholipase A2 and cyclic adenosine monophosphate (cAMP)/protein kinase A (PKA) dependent pathways (Zhang and Pan, 2010). Moreover, mu-delta preferential coupling to the pertussis toxin insensitive Gαz subunit would not be desensitized by chronic morphine administration in the rat striatum and hippocampus (George et al., 2000; Kabli et al., 2014). Altogether, mu-delta positive crosstalk reinforces the inhibition of neuronal activity.
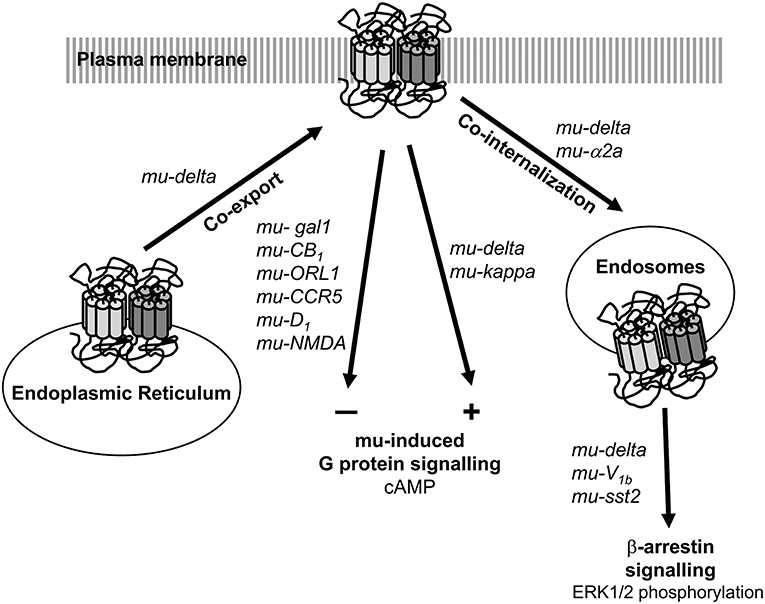
Figure 1. Impact of heteromerization on mu opioid receptor signaling and trafficking. Heteromerization can take place in the endoplasmic reticulum. Association with another opioid receptor positively modulates mu opioid receptor G protein-dependent signaling whereas association with a non-opioid receptor negatively regulates it. Heteromerization also favors the recruitment the β-arrrestin dependent pathway upon internalization in the endosomal compartments. Native receptor pairs for which information is available are indicated.
In contrast, heteromers formed with a non-opioid receptor appear to negatively modulate mu receptor G protein dependent signaling (Figure 1). In the VTA, co-activation of mu-Gal1 heteromers by galanin and endomorphin 1 decreased extracellular signal-regulated kinase ERK1/2, protein kinase B (AKT), and cyclic AMP response element binding protein (CREB) phosphorylation (Moreno et al., 2017). Accordingly, galanin could not prevent dopamine release promoted by local infusion of endomorphin 1 in the presence of an interfering peptide that disrupt mu-Gal1 physical interaction (Moreno et al., 2017). In addition, acute morphine administration enhanced ERK1/2 activation in the nucleus accumbens (Nacc) and amygdala of galanin knock-out mice compared to wild type mice (Hawes et al., 2008). These data suggest a negative crosstalk mediated by mu-Gal1 heteromers by which galanin dampens mu receptor signaling. In addition, the mu antagonist CTOP counteracted galanin induced ERK1/2, AKT and CREB phosphorylation indicative of a cross-antagonism on Gal1 receptor signaling (Moreno et al., 2017).
In BE(2)-C neuroblastoma cells co-expressing mu and ORL1 receptors, pretreatment with nociceptin decreased DAMGO potency and efficacy to inhibit adenylate cyclase (Mandyam et al., 2002). This effect was abolished in HEK293 cells co-transfected with receptor pairs unable to physically associate, which supports heteromer specificity (Wang et al., 2005). Additional examples of negative crosstalk on mu receptor signaling can be linked to heteromerization. Co-activation of mu and cannabinoid CB1 receptors by the mu agonist morphine and a non-signaling dose of the CB1 agonist WIN 55,212-2 decreased [35S]guanosine 5′-[γ-thio]triphosphate (GTPγS) binding and ERK1/2 signaling in SK-N-SH neuroblastoma cells (Rios et al., 2006) and Δ9-tetrahydrocannabinol (THC) allosterically decreased dihydromorphine binding at the mu receptor in rat striatal membranes (Vaysse et al., 1987). Similarly, co-activation of mu and adrenergic α2a receptors decreased ERK1/2 phosphorylation in primary spinal cord neurons (Jordan et al., 2003). Also, the chemokine CCL5 induced phosphorylation of the mu receptor in human PBMC indicating cross-desensitization (Szabo et al., 2002). Finally, the dopamine D1 antagonist SCH233390 decreased G protein activation and ERK1/2 phosphorylation induced by the mu agonist DAMGO in mouse striatal membrane from wild type but not mice deficient for the D1 receptor (Tao et al., 2017). Activation of mu-NMDA heteromers by N-methyl-D-aspartate (NMDA) in the periaqueductal gray (PAG) also negatively regulated mu receptor activity by promoting PKA-dependent dissociation of the heteromer and subsequent mu receptor phosphorylation. This in turn promoted G protein uncoupling and receptor desensitization (Rodríguez-Muñoz et al., 2012).
Interestingly, constitutive activity has been reported for delta opioid (Costa and Herz, 1989), kappa opioid (Sirohi and Walker, 2015), ORL1 (Beedle et al., 2004), cannabinoid CB1 (Fioravanti et al., 2008), adrenergic α2a (Pauwels et al., 2000), and mu opioid receptors (Wang et al., 2004). Since heteromers involving the mu receptor form in the ER in a ligand independent manner, receptor constitutive activity could represent an important determinant of the allosteric modulation and could contribute to the basal homeostasis of the cell in the absence of receptor stimulation. The release of endogenous peptides would however further modulate their functional impact because these receptors can still be activated by agonists (Canals and Milligan, 2008).
Activation of β-Arrestin Signaling in Native Mu Heteromers
In SK-N-SH neuroblastoma cells co-expressing mu and delta receptors, activation by the mu agonist DAMGO changed the spatio-temporal profile of ERK1/2 phosphorylation (Rozenfeld and Devi, 2007) (Figure 1). This was abolished in the presence of a β-arrestin 2 small interfering ribonucleic acid (siRNA) or in the presence of the delta selective antagonist TIPPψ (Rozenfeld and Devi, 2007) suggesting that activation of heteromers involving the mu receptor can promote β-arrestin dependent signaling. Mice deficient for β-arrestin 2 developed less tolerance to morphine (Bohn et al., 2002), data thus suggest that the recruitment of the β-arrestin pathway by mu heteromers contributes to morphine tolerance. This hypothesis is also supported by the observation that tolerance to morphine develops more slowly in mice deficient for the vasopressin V1B receptor or in the presence of a V1B selective antagonist (Koshimizu et al., 2018). In the mouse RVM, vasopressin V1B receptors constitutively associate with β-arrestin 2 through a leucine rich motif present in the V1B C-terminus (Koshimizu et al., 2018). This suggests that physical association with the V1B receptor facilitates the recruitment of the β-arrestin pathway by the mu receptor, and contributes to the development of morphine tolerance. Accordingly, removal by genome editing with the clustered regularly interspaced short palindromic repeats-CRISPR associated protein 9 (CRISPR-Cas9) system of the leucine rich motif responsible for the receptor V1B-β-arrestin interaction increased morphine-induced analgesia and reduced adenylate cyclase supersensitization and morphine-induced tolerance and physical dependence (Koshimizu et al., 2018).
Of note, co-activation of mu and somatostatin sst2 receptors by dermorphin and L-054,264 in pancreatic cancer lines similarly altered the spatio-temporal profile of ERK1/2 phosphorylation, potentiating the epithelial to mesenchymal transition (Jorand et al., 2016).
Receptor Co-Internalization in Native Mu Heteromers
Co-internalization of endogenous heteromers is less documented largely due to the lack of appropriate tools (Figure 1). Receptor internalization contributes to desensitize G protein dependent signaling and favors β-arrestin dependent signaling (Calebiro et al., 2010). Accordingly, co-internalization of mu and adrenergic α2a receptors was dependent on β-arrestin 2 recruitment and mitogen-activated protein kinase (MAPK) p38 activation in the mouse DRGs (Tan et al., 2009).
Mu-delta co-internalization was observed following activation by the mu-delta biased agonist CYM51010 in primary hippocampal neurons from fluorescent double knock-in mice (Derouiche et al., 2018) but could not be detected in the spinal cord following SNC80 application (Wang et al., 2018). Since SNC80 promoted mu-delta co-internalization in co-transfected HEK293 cells (He et al., 2011), this observation highlights the influence of the cellular environment.
Mu Heteromers Modulate Nociception, Morphine Analgesia and Tolerance
Several observations support the implication of mu heteromers in the control of the nociceptive threshold. The lower response to inflammatory or chemical stimuli in CCR5 knock-out mice or upon injection of a CCR5 antagonist indicate that mu-CCR5 heteromers contribute to dampen the basal nociceptive threshold by exerting a negative crosstalk on mu receptor signaling (Lee et al., 2013). Mapping mu and delta receptors in the central and peripheral nervous systems using double fluorescent knock-in mice revealed mu-delta co-expression in discrete neuronal populations located in networks involved in the perception and processing of nociceptive stimuli (Erbs et al., 2015). Accordingly, disrupting mu-delta physical interaction with an interfering peptide in naïve mice increased morphine-induced thermal analgesia (He et al., 2011). In rats chronically treated with morphine or with persistent inflammatory pain, co-administration of low doses of mu and delta agonists in the RVM enhanced mechanical and thermal analgesia (Sykes et al., 2007; Zhang and Pan, 2010). Since delta receptor expression is increased in both pathological conditions (Cahill et al., 2003; Gendron et al., 2015; Ong et al., 2015; Erbs et al., 2016), this synergistic effect can be explained by the positive crosstalk at mu-delta heteromer elicited by receptor co-activation (Gomes et al., 2000, 2004, 2011). Similarly, co-activation by morphine and the subsequently released dynorphin 1–17 acted synergistically at mu-kappa heteromers to increase spinal morphine analgesia (Chakrabarti et al., 2010). However, this effect is sex-dependent and more pronounced in proestrous female mice where mu-kappa heteromers are most abundant (Chakrabarti et al., 2010; Liu N. J. et al., 2011).
Activation of mu-delta heteromers by the mu agonist DAMGO (Rozenfeld and Devi, 2007) or co-activation of mu-V1b heteromers by vasopressin and morphine (Koshimizu et al., 2018) increased β-arrestin 2 recruitment and signaling. Importantly, this pathway participates to the development of morphine tolerance (Bohn et al., 2000), which suggests a contribution from mu heteromers. Accordingly, disruption of the physical contact between the mu and delta opioid receptors (Xie et al., 2009; He et al., 2011) or between the mu and vasopressin V1b receptors (Koshimizu et al., 2018) decreased morphine tolerance. In addition, activation of mu-NMDA heteromers in the PAG reduces morphine efficacy through a dual mechanism. Indeed, stimulation by NMDA decreases the analgesic effect of morphine by exerting a negative crosstalk on mu signaling whereas morphine binding to the mu receptor potentiates the NMDA-Ca2+/calmodulin-dependent protein kinase (CAMKII) pathway and contributes to morphine tolerance (Rodríguez-Muñoz et al., 2012).
Other roles for mu heteromers include morphine-induced itch generated by cross-activation of the GRPR signaling in MORD1-GRPR heteromers (Liu X. Y. et al., 2011). Moreover, mu-α2a, mu-CB1, or mu-ORL1 heteromers very likely represent additional key players since all four receptors modulate nociception but, to date, a direct link to heteromerization with the mu receptor is still lacking.
Mu Heteromers Modulate Reward Processing and Addiction to Opioid Drugs
Modulation of mu receptor signaling by heteromer formation in the mesocorticolimbic pathway is bound to have a profound impact on the rewarding properties of opioid drugs and natural stimuli. Accordingly, galanin-dependent dampening of opiate reinforcing and rewarding properties was abolished upon disruption of mu-Gal1 heteromers in the VTA (Moreno et al., 2017) or in galanin knock-out mice (Hawes et al., 2008). Also, chronic morphine treatment increased mu-delta heteromer expression in several brain regions including the VTA and Nacc (Gupta et al., 2010). Therefore, the positive cross talk at mu-delta heteromers observed in a subset of VTA neurons could contribute to increased dopamine release in the Nacc and opiate reinforcing properties (Margolis et al., 2017).
Also, systemic injection of the endocannabinoid 2-arachidonoyl (2-AG) hydrolysis inhibitor JZL184 increased the concentration of the endogenous ligand and enhanced social play behavior in adolescent rodents (Manduca et al., 2016). This effect was blocked by infusing the mu antagonist CTAP in the Nacc and was absent in mu receptor knock-out mice (Manduca et al., 2016). Reciprocally, systemic injection of the mu agonist morphine increased social play and was abolished by the CB1 antagonist SR1417-16 or in CB1 receptor knock-out mice (Manduca et al., 2016). This bidirectional cross-antagonism suggests that mu-CB1 heteromers in the Nacc modulate the strong rewarding value of social play.
Mu receptors are also involved in other aspects of opiate addiction such as locomotor sensitization and could achieve their modulatory control through heteromerization with dopamine D1 receptors. Indeed, opiate hyperlocomotion and locomotor sensitization were abolished in dopamine D1 receptor in knock-out mice or following local injection of the D1 antagonist SCH23390 in the Nacc (Tao et al., 2017).
Mu Heteromers Modulate Anxiety and Depression
Pharmacological and knock-out based studies linked an anxiogenic and depressant phenotype to mu receptor activation and, on the opposite, associated an anxiolytic and antidepressant phenotype with delta receptor activation (Lutz et al., 2014).
Systemic administration or local micro-infusion in the Nacc of the delta agonist UFP512 promoted anxiolytic- and anti-depressant-like activity (Vergura et al., 2008; Kabli et al., 2013). These effects were abolished by pretreatment with the mu antagonist CTOP or the delta antagonist naltrindole or following disruption of mu-delta physical contact in the Nacc (Kabli et al., 2013). These data therefore suggest that accumbal mu-delta heteromers participate to the modulation of anxio-depressive states.
Mu Heteromers Modulate Metabolic Disorders
Mu receptors are known to control autonomous functions. Higher levels of mu-α2a heteromers in the NTS were correlated with increased blood pressure in hypertensive rats (Sun et al., 2015). In normotensive rats, mu-α2a heteromerization induced by the mu agonist DAMGO was paralleled by increased blood pressure. Treatment with the mu antagonist CTAP antagonized DAMGO changes in normotensive rats and reduced mu-α2a heteromerization and blood pressure in hypertensive rats (Sun et al., 2015). Thus, activation of the mu receptor by endogenous opioid peptides dampens the activity of the α2a adrenergic receptors thereby potentiating hypertension.
Interactions between mu and somatostatin receptors have been postulated to influence tumor cell growth (Hatzoglou et al., 2005). Recently, mu-sst2 heteromers were identified in pancreatic cancer lines and in tissue from patients with pancreatic ductal adenocarcinoma. Co-activation of the receptors initiated the epithelial to mesenchymal transition, which is associated with increased metastatic potential (Jorand et al., 2016).
Mu Heteromers as a Novel Therapeutic Target
The bivalent ligand MDAN-21 composed of the mu agonist oxymorphone and the delta antagonist naltrindole tethered by a 21 amino acid long linker was developed to selectively target mu-delta heteromers (Daniels et al., 2005). The length of the linker was designed to enable simultaneous binding of the two ligand moieties to the orthosteric binding pockets of two GPCRs in physical contact. MDAN-21 induced analgesia with low tolerance, low physical dependence and no reinforcing properties (Daniels et al., 2005; Lenard et al., 2007; Aceto et al., 2012) providing a proof of concept that selective targeting of mu-delta heteromers may represent a valid therapeutic strategy, in particular for patients on opiate maintenance treatment.
More recently, the bivalent ligand MCC22 linking the mu agonist oxymorphone to the CCR5 antagonist TAK220 has been proposed to inhibit inflammatory and neuropathic pain by targeting mu-CCR5 heteromers (Akgün et al., 2015). This is in line with the enhanced nociception observed in CCR5 receptor knock-out mice or in the presence of a CCR5 antagonist (Lee et al., 2013).
A major limitation to the therapeutic use of bivalent ligands is their poor capacity to cross the blood brain barrier (Le Naour et al., 2013; Jörg et al., 2015). Therefore, monovalent bifunctional ligands that would selectively target mu heteromers have been developed (Schiller, 2010; Günther et al., 2018). Eluxadoline is a mixed mu agonist delta antagonist recently been approved by the FDA for the treatment of the irritable bowel syndrome (FDA application N°206940). Arguments in favor of binding to mu-delta heteromers include lower efficacy in mice deficient for the delta receptor and reduced signaling in the presence of mu-delta selective antibodies (Fujita et al., 2014b). Eluxadoline thus represents the first drug on the market designed to target heteromers. In preclinical models, other ligands further support preferential activation of mu heteromers as a valuable therapeutic approach. The mu-delta biased agonist CYM51010 induced potent thermal analgesia comparable to morphine but less tolerance and physical dependence (Gomes et al., 2013) and the mu-kappa agonist NNTA produced strong analgesia devoid of tolerance, physical dependence, or reinforcing properties upon intrathecal injection in mice (Yekkirala et al., 2011).
Conclusion
Our current appreciation of the role of mu heteromer is still in its infancy and their contribution to mu receptor-dependent behavior likely underestimated. So far, physical proximity has only been validated for a limited number of receptor pairs in vivo and their functional interactions addressed in a handful of tissue or brain areas. Moreover, heteromer expression is dynamically regulated depending on physiopathological conditions. No doubt that both novel functions and receptor pairs will be uncovered in the future, which further emphasizes their potential as innovative therapeutic targets.
Author Contributions
All authors listed have made a substantial, direct and intellectual contribution to the work, and approved it for publication.
Conflict of Interest Statement
The authors declare that the research was conducted in the absence of any commercial or financial relationships that could be construed as a potential conflict of interest.
Acknowledgments
The work was performed thanks to the financial support of the Fondation pour la Recherche Médicale (DPA20140129364), the CNRS and the University of Strasbourg. LD was the recipient of an IDEX post-doctoral fellowship of the University of Strasbourg and MU was a fellow of the Neurotime Erasmus Mundus Joint Doctorate program.
References
Aceto, M. D., Harris, L. S., Negus, S. S., Banks, M. L., Hughes, L. D., Akgun, E., et al. (2012). MDAN-21: a bivalent opioid ligand containing mu-agonist and delta-antagonist pharmacophores and its effects in rhesus monkeys. Int. J. Med. Chem. 2012:327257. doi: 10.1155/2012/327257
Akgün, E., Javed, M. I., Lunzer, M. M., Powers, M. D., Sham, Y. Y., Watanabe, Y., et al. (2015). Inhibition of inflammatory and neuropathic pain by targeting a Mu Opioid Receptor/Chemokine Receptor5 Heteromer (MOR-CCR5). J. Med. Chem. 58, 8647–8657. doi: 10.1021/acs.jmedchem.5b01245
Beedle, A. M., McRory, J. E., Poirot, O., Doering, C. J., Altier, C., Barrere, C., et al. (2004). Agonist-independent modulation of N-type calcium channels by ORL1 receptors. Nat. Neurosci. 7, 118–125. doi: 10.1038/nn1180
Bohn, L. M., Gainetdinov, R. R., Lin, F. T., Lefkowitz, R. J., and Caron, M. G. (2000). Mu-opioid receptor desensitization by beta-arrestin-2 determines morphine tolerance but not dependence. Nature 408, 720–723. doi: 10.1038/35047086
Bohn, L. M., Lefkowitz, R. J., and Caron, M. G. (2002). Differential mechanisms of morphine antinociceptive tolerance revealed in (beta)arrestin-2 knock-out mice. J. Neurosci. 22, 10494–10500. doi: 10.1523/JNEUROSCI.22-23-10494.2002
Borroto-Escuela, D. O., Brito, I., Romero-Fernandez, W., Di Palma, M., Oflijan, J., Skieterska, K., et al. (2014). The G protein-coupled receptor heterodimer network (GPCR-HetNet) and its hub components. Int. J. Mol. Sci. 15, 8570–8590. doi: 10.3390/ijms15058570
Cahill, C. M., Morinville, A., Hoffert, C., O'donnell, D., and Beaudet, A. (2003). Up-regulation and trafficking of delta opioid receptor in a model of chronic inflammation: implications for pain control. Pain 101, 199–208. doi: 10.1016/S0304-3959(02)00333-0
Calebiro, D., Nikolaev, V. O., Persani, L., and Lohse, M. J. (2010). Signaling by internalized G-protein-coupled receptors. Trends Pharmacol. Sci. 31, 221–228. doi: 10.1016/j.tips.2010.02.002
Canals, M., and Milligan, G. (2008). Constitutive activity of the cannabinoid CB1 receptor regulates the function of co-expressed Mu opioid receptors. J. Biol. Chem. 283, 11424–11434. doi: 10.1074/jbc.M710300200
Chakrabarti, S., Liu, N. J., and Gintzler, A. R. (2010). Formation of mu-/kappa-opioid receptor heterodimer is sex-dependent and mediates female-specific opioid analgesia. Proc. Natl. Acad. Sci. U.S.A. 107, 20115–20119. doi: 10.1073/pnas.1009923107
Costa, T., and Herz, A. (1989). Antagonists with negative intrinsic activity at d opioid receptors coupled to GTP-binding proteins. Proc. Natl. Acad. Sci. U.S.A. 86, 7321–7325. doi: 10.1073/pnas.86.19.7321
Cussac, D., Rauly-Lestienne, I., Heusler, P., Finana, F., Cathala, C., Bernois, S., et al. (2012). mu-Opioid and 5-HT1A receptors heterodimerize and show signalling crosstalk via G protein and MAP-kinase pathways. Cell. Signal. 24, 1648–1657. doi: 10.1016/j.cellsig.2012.04.010
Daniels, D. J., Lenard, N. R., Etienne, C. L., Law, P. Y., Roerig, S. C., and Portoghese, P. S. (2005). Opioid-induced tolerance and dependence in mice is modulated by the distance between pharmacophores in a bivalent ligand series. Proc. Natl. Acad. Sci. U.S.A. 102, 19208–19213. doi: 10.1073/pnas.0506627102
Derouiche, L., Ory, S., and Massotte, D. (2018). “Double fluorescent knock-in mice to investigate endogenous mu-delta opioid heteromer subscellular distribution,” in Receptor-Receptor Interactions in the Central Nervous System, Neuromethods, eds K. Fuxe and D. Borroto-Escuela (New York, NY: The Humana Press; Springer Nature), 149–162.
Erbs, E., Faget, L., Ceredig, R. A., Matifas, A., Vonesch, J. L., Kieffer, B. L., et al. (2016). Impact of chronic morphine on delta opioid receptor-expressing neurons in the mouse hippocampus. Neuroscience 313, 46–56. doi: 10.1016/j.neuroscience.2015.10.022
Erbs, E., Faget, L., Scherrer, G., Matifas, A., Filliol, D., Vonesch, J. L., et al. (2015). A mu-delta opioid receptor brain atlas reveals neuronal co-occurrence in subcortical networks. Brain Struct. Funct. 220, 677–702. doi: 10.1007/s00429-014-0717-9
Evans, R. M., You, H., Hameed, S., Altier, C., Mezghrani, A., Bourinet, E., et al. (2010). Heterodimerization of ORL1 and opioid receptors and its consequences for N-type calcium channel regulation. J. Biol. Chem. 285, 1032–1040. doi: 10.1074/jbc.M109.040634
Fioravanti, B., De Felice, M., Stucky, C. L., Medler, K. A., Luo, M. C., Gardell, L. R., et al. (2008). Constitutive activity at the cannabinoid CB1 receptor is required for behavioral response to noxious chemical stimulation of TRPV1: antinociceptive actions of CB1 inverse agonists. J. Neurosci. 28, 11593–11602. doi: 10.1523/JNEUROSCI.3322-08.2008
Fujita, W., Gomes, I., and Devi, L. A. (2014a). Revolution in GPCR Signaling: Opioid receptor heteromers as novel therapeutic targets. Br. J. Pharmacol. 171, 4155–4176. doi: 10.1111/bph.12798
Fujita, W., Gomes, I., Dove, L. S., Prohaska, D., Mcintyre, G., and Devi, L. A. (2014b). Molecular characterization of eluxadoline as a potential ligand targeting mu-delta opioid receptor heteromers. Biochem. Pharmacol. 92, 448–456. doi: 10.1016/j.bcp.2014.09.015
Gendron, L., Mittal, N., Beaudry, H., and Walwyn, W. (2015). Recent advances on the delta opioid receptor: from trafficking to function. Br. J. Pharmacol. 172, 403–419. doi: 10.1111/bph.12706
George, S. R., Fan, T., Xie, Z., Tse, R., Tam, V., Varghese, G., et al. (2000). Oligomerization of mu- and delta-opioid receptors. Generation of novel functional properties. J. Biol. Chem. 275, 26128–26135. doi: 10.1074/jbc.M000345200
Gomes, I., Fujita, W., Gupta, A., Saldanha, S. A., Negri, A., Pinello, C. E., et al. (2013). Identification of a mu-delta opioid receptor heteromer-biased agonist with antinociceptive activity. Proc. Natl. Acad. Sci. U.S.A. 110, 12072–12077. doi: 10.1073/pnas.1222044110
Gomes, I., Gupta, A., Filipovska, J., Szeto, H. H., Pintar, J. E., and Devi, L. A. (2004). A role for heterodimerization of mu and delta opiate receptors in enhancing morphine analgesia. Proc. Natl. Acad. Sci. U.S.A. 101, 5135–5139. doi: 10.1073/pnas.0307601101
Gomes, I., Ijzerman, A. P., Ye, K., Maillet, E. L., and Devi, L. A. (2011). G protein-coupled receptor heteromerization: a role in allosteric modulation of ligand binding. Mol. Pharmacol. 79, 1044–1052. doi: 10.1124/mol.110.070847
Gomes, I., Jordan, B. A., Gupta, A., Trapaidze, N., Nagy, V., and Devi, L. A. (2000). Heterodimerization of mu and delta opioid receptors: a role in opiate synergy. J. Neurosci. 20, 1–5. doi: 10.1523/JNEUROSCI.20-22-j0007.2000
Günther, T., Dasgupta, P., Mann, A., Miess, E., Kliewer, A., Fritzwanker, S., et al. (2018). Targeting multiple opioid receptors - improved analgesics with reduced side effects? Br. J. Pharmacol. 175, 2857–2868. doi: 10.1111/bph.13809
Gupta, A., Mulder, J., Gomes, I., Rozenfeld, R., Bushlin, I., Ong, E., et al. (2010). Increased abundance of opioid receptor heteromers after chronic morphine administration. Sci. Signal. 3:ra54. doi: 10.1126/scisignal.2000807
Happel, C., Steele, A. D., Finley, M. J., Kutzler, M. A., and Rogers, T. J. (2008). DAMGO-induced expression of chemokines and chemokine receptors: the role of TGF-beta1. J. Leukoc. Biol. 83, 956–963. doi: 10.1189/jlb.1007685
Hatzoglou, A., Kampa, M., and Castanas, E. (2005). Opioid-somatostatin interactions in regulating cancer cell growth. Front. Biosci. 10, 244–256. doi: 10.2741/1524
Hawes, J. J., Brunzell, D. H., Narasimhaiah, R., Langel, U., Wynick, D., and Picciotto, M. R. (2008). Galanin protects against behavioral and neurochemical correlates of opiate reward. Neuropsychopharmacology 33, 1864–1873. doi: 10.1038/sj.npp.1301579
He, S. Q., Zhang, Z. N., Guan, J. S., Liu, H. R., Zhao, B., Wang, H. B., et al. (2011). Facilitation of mu-opioid receptor activity by preventing delta-opioid receptor-mediated codegradation. Neuron 69, 120–131. doi: 10.1016/j.neuron.2010.12.001
Heinisch, S., Palma, J., and Kirby, L. G. (2011). Interactions between chemokine and mu-opioid receptors: anatomical findings and electrophysiological studies in the rat periaqueductal grey. Brain Behav. Immun. 25, 360–372. doi: 10.1016/j.bbi.2010.10.020
Jorand, R., Biswas, S., Wakefield, D. L., Tobin, S. J., Golfetto, O., Hilton, K., et al. (2016). Molecular signatures of mu opioid receptor and somatostatin receptor 2 in pancreatic cancer. Mol. Biol. Cell 27, 3659–3672. doi: 10.1091/mbc.e16-06-0427
Jordan, B. A., Gomes, I., Rios, C., Filipovska, J., and Devi, L. A. (2003). Functional interactions between mu opioid and alpha 2A-adrenergic receptors. Mol. Pharmacol. 64, 1317–1324. doi: 10.1124/mol.64.6.1317
Jörg, M., May, L. T., Mak, F. S., Lee, K. C., Miller, N. D., Scammells, P. J., et al. (2015). Synthesis and pharmacological evaluation of dual acting ligands targeting the adenosine A2A and dopamine D2 receptors for the potential treatment of Parkinson's disease. J. Med. Chem. 58, 718–738. doi: 10.1021/jm501254d
Kabli, N., Fan, T., O'dowd, B. F., and George, S. R. (2014). mu-delta opioid receptor heteromer-specific signaling in the striatum and hippocampus. Biochem. Biophys. Res. Commun. 450, 906–911. doi: 10.1016/j.bbrc.2014.06.099
Kabli, N., Nguyen, T., Balboni, G., O'dowd, B. F., and George, S. R. (2013). Antidepressant-like and anxiolytic-like effects following activation of the mu-delta opioid receptor heteromer in the nucleus accumbens. Mol. Psychiatry 19, 968–994. doi: 10.1038/mp.2013.115
Kieffer, B. L. (1999). Opioids: first lessons from knockout mice. Trends Pharmacol. 20, 19–26. doi: 10.1016/S0165-6147(98)01279-6
Kieffer, B. L., and Evans, C. J. (2009). Opioid receptors: from binding sites to visible molecules in vivo. Neuropharmacology 56(Suppl. 1), 205–212. doi: 10.1016/j.neuropharm.2008.07.033
Koshimizu, T. A., Honda, K., Nagaoka-Uozumi, S., Ichimura, A., Kimura, I., Nakaya, M., et al. (2018). Complex formation between the vasopressin 1b receptor, beta-arrestin-2, and the mu-opioid receptor underlies morphine tolerance. Nat. Neurosci. 21, 820–833. doi: 10.1038/s41593-018-0144-y
Le Naour, M., Akgun, E., Yekkirala, A., Lunzer, M. M., Powers, M. D., Kalyuzhny, A. E., et al. (2013). Bivalent ligands that target mu opioid (MOP) and cannabinoid1 (CB1) receptors are potent analgesics devoid of tolerance. J. Med. Chem. 56, 5505–5513. doi: 10.1021/jm4005219
Lee, Y. K., Choi, D. Y., Jung, Y. Y., Yun, Y. W., Lee, B. J., Han, S. B., et al. (2013). Decreased pain responses of C-C chemokine receptor 5 knockout mice to chemical or inflammatory stimuli. Neuropharmacology 67, 57–65. doi: 10.1016/j.neuropharm.2012.10.030
Lenard, N. R., Daniels, D. J., Portoghese, P. S., and Roerig, S. C. (2007). Absence of conditioned place preference or reinstatement with bivalent ligands containing mu-opioid receptor agonist and delta-opioid receptor antagonist pharmacophores. Eur. J. Pharmacol. 566, 75–82. doi: 10.1016/j.ejphar.2007.02.040
Liu, N. J., Chakrabarti, S., Schnell, S., Wessendorf, M., and Gintzler, A. R. (2011). Spinal synthesis of estrogen and concomitant signaling by membrane estrogen receptors regulate spinal kappa- and mu-opioid receptor heterodimerization and female-specific spinal morphine antinociception. J. Neurosci. 31, 11836–11845. doi: 10.1523/JNEUROSCI.1901-11.2011
Liu, X. Y., Liu, Z. C., Sun, Y. G., Ross, M., Kim, S., Tsai, F. F., et al. (2011). Unidirectional cross-activation of GRPR by MOR1D uncouples itch and analgesia induced by opioids. Cell 147, 447–458. doi: 10.1016/j.cell.2011.08.043
Lopez-Gimenez, J. F., Vilaro, M. T., and Milligan, G. (2008). Morphine desensitization, internalization, and down-regulation of the mu opioid receptor is facilitated by serotonin 5-hydroxytryptamine2A receptor coactivation. Mol. Pharmacol. 74, 1278–1291. doi: 10.1124/mol.108.048272
Lutz, P. E., Ayranci, G., Chu-Sin-Chung, P., Matifas, A., Koebel, P., Filliol, D., et al. (2014). Distinct mu, delta, and kappa opioid receptor mechanisms underlie low sociability and depressive-like behaviors during heroin abstinence. Neuropsychopharmacology 39, 2694–2705. doi: 10.1038/npp.2014.126
Manduca, A., Lassalle, O., Sepers, M., Campolongo, P., Cuomo, V., Marsicano, G., et al. (2016). Interacting cannabinoid and opioid receptors in the nucleus accumbens core control adolescent social play. Front. Behav. Neurosci. 10:211. doi: 10.3389/fnbeh.2016.00211
Mandyam, C. D., Thakker, D. R., Christensen, J. L., and Standifer, K. M. (2002). Orphanin FQ/nociceptin-mediated desensitization of opioid receptor-like 1 receptor and mu opioid receptors involves protein kinase C: a molecular mechanism for heterologous cross-talk. J. Pharmacol. Exp. Ther. 302, 502–509. doi: 10.1124/jpet.102.033159
Margolis, E. B., Fujita, W., Devi, L. A., and Fields, H. L. (2017). Two delta opioid receptor subtypes are functional in single ventral tegmental area neurons, and can interact with the mu opioid receptor. Neuropharmacology 123, 420–432. doi: 10.1016/j.neuropharm.2017.06.019
Matthes, H. W., Maldonado, R., Simonin, F., Valverde, O., Slowe, S., Kitchen, I., et al. (1996). Loss of morphine-induced analgesia, reward effect and withdrawal symptoms in mice lacking the mu-opioid-receptor gene. Nature 383, 819–823. doi: 10.1038/383819a0
Moreno, E., Quiroz, C., Rea, W., Cai, N. S., Mallol, J., Cortes, A., et al. (2017). Functional mu-opioid-galanin receptor heteromers in the ventral tegmental area. J. Neurosci. 37, 1176–1186. doi: 10.1523/JNEUROSCI.2442-16.2016
Ong, E. W., Xue, L., Olmstead, M. C., and Cahill, C. M. (2015). Prolonged morphine treatment alters delta opioid receptor post-internalization trafficking. Br. J. Pharmacol. 172, 615–629. doi: 10.1111/bph.12761
Patel, J. P., Sengupta, R., Bardi, G., Khan, M. Z., Mullen-Przeworski, A., and Meucci, O. (2006). Modulation of neuronal CXCR4 by the micro-opioid agonist DAMGO. J. Neurovirol. 12, 492–500. doi: 10.1080/13550280601064798
Pauwels, P. J., Tardif, S., Wurch, T., and Colpaert, F. C. (2000). Facilitation of constitutive alpha(2A)-adrenoceptor activity by both single amino acid mutation (Thr(373)Lys) and g(alphao) protein coexpression: evidence for inverse agonism. J. Pharmacol. Exp. Ther. 292, 654–663.
Pfeiffer, M., Kirscht, S., Stumm, R., Koch, T., Wu, D., Laugsch, M., et al. (2003). Heterodimerization of substance P and mu-opioid receptors regulates receptor trafficking and resensitization. J. Biol. Chem. 278, 51630–51637. doi: 10.1074/jbc.M307095200
Qian, M., Vasudevan, L., Huysentruyt, J., Risseeuw, M. D. P., Stove, C., Vanderheyden, P. M. L., et al. (2018). Design, synthesis, and biological evaluation of bivalent ligands targeting dopamine D2 -like receptors and the mu-opioid receptor. ChemMedChem 13, 944–956. doi: 10.1002/cmdc.201700787
Rediger, A., Tarnow, P., Bickenbach, A., Schaefer, M., Krude, H., Gruters, A., et al. (2009). Heterodimerization of hypothalamic G-protein-coupled receptors involved in weight regulation. Obes. Facts 2, 80–86. doi: 10.1159/000209862
Rios, C., Gomes, I., and Devi, L. A. (2006). mu opioid and CB1 cannabinoid receptor interactions: reciprocal inhibition of receptor signaling and neuritogenesis. Br. J. Pharmacol. 148, 387–395. doi: 10.1038/sj.bjp.0706757
Rivera, A., Gago, B., Suarez-Boomgaard, D., Yoshitake, T., Roales-Bujan, R., Valderrama-Carvajal, A., et al. (2017). Dopamine D4 receptor stimulation prevents nigrostriatal dopamine pathway activation by morphine: relevance for drug addiction. Addict. Biol. 22, 1232–1245. doi: 10.1111/adb.12407
Rodriguez, J. J., Mackie, K., and Pickel, V. M. (2001). Ultrastructural localization of the CB1 cannabinoid receptor in μ-opioid receptor patches of the rat Caudate putamen nucleus. J. Neurosci. 21, 823–833. doi: 10.1523/JNEUROSCI.21-03-00823.2001
Rodríguez-Muñoz, M., Sanchez-Blazquez, P., Vicente-Sanchez, A., Berrocoso, E., and Garzon, J. (2012). The mu-opioid receptor and the NMDA receptor associate in PAG neurons: implications in pain control. Neuropsychopharmacology 37, 338–349. doi: 10.1038/npp.2011.155
Roumy, M., Lorenzo, C., Mazeres, S., Bouchet, S., Zajac, J. M., and Mollereau, C. (2007). Physical association between neuropeptide FF and micro-opioid receptors as a possible molecular basis for anti-opioid activity. J. Biol. Chem. 282, 8332–8342. doi: 10.1074/jbc.M606946200
Rozenfeld, R., and Devi, L. A. (2007). Receptor heterodimerization leads to a switch in signaling: beta-arrestin2-mediated ERK activation by mu-delta opioid receptor heterodimers. FASEB J. 21, 2455–2465. doi: 10.1096/fj.06-7793com
Schiller, P. W. (2010). Bi- or multifunctional opioid peptide drugs. Life Sci. 86, 598–603. doi: 10.1016/j.lfs.2009.02.025
Schröder, H., Wu, D. F., Seifert, A., Rankovic, M., Schulz, S., Hollt, V., et al. (2009). Allosteric modulation of metabotropic glutamate receptor 5 affects phosphorylation, internalization, and desensitization of the micro-opioid receptor. Neuropharmacology 56, 768–778. doi: 10.1016/j.neuropharm.2008.12.010
Sirohi, S., and Walker, B. M. (2015). Maturational alterations in constitutive activity of medial prefrontal cortex kappa-opioid receptors in Wistar rats. J. Neurochem. 135, 659–665. doi: 10.1111/jnc.13279
Sun, G. C., Ho, W. Y., Chen, B. R., Cheng, P. W., Cheng, W. H., Hsu, M. C., et al. (2015). GPCR dimerization in brainstem nuclei contributes to the development of hypertension. Br. J. Pharmacol. 172, 2507–2518. doi: 10.1111/bph.13074
Suzuki, S., Chuang, L. F., Yau, P., Doi, R. H., and Chuang, R. Y. (2002). Interactions of opioid and chemokine receptors: oligomerization of mu, kappa, and delta with CCR5 on immune cells. Exp. Cell Res. 280, 192–200. doi: 10.1006/excr.2002.5638
Sykes, K. T., White, S. R., Hurley, R. W., Mizoguchi, H., Tseng, L. F., and Hammond, D. L. (2007). Mechanisms responsible for the enhanced antinociceptive effects of micro-opioid receptor agonists in the rostral ventromedial medulla of male rats with persistent inflammatory pain. J. Pharmacol. Exp. Ther. 322, 813–821. doi: 10.1124/jpet.107.121954
Szabo, I., Chen, X. H., Xin, L., Adler, M. W., Howard, O. M., Oppenheim, J. J., et al. (2002). Heterologous desensitization of opioid receptors by chemokines inhibits chemotaxis and enhances the perception of pain. Proc. Natl. Acad. Sci. U.S.A. 99, 10276–10281. doi: 10.1073/pnas.102327699
Tan, M., Walwyn, W. M., Evans, C. J., and Xie, C. W. (2009). p38 MAPK and beta-arrestin 2 mediate functional interactions between endogenous micro-opioid and alpha2A-adrenergic receptors in neurons. J. Biol. Chem. 284, 6270–6281. doi: 10.1074/jbc.M806742200
Tao, Y. M., Yu, C., Wang, W. S., Hou, Y. Y., Xu, X. J., Chi, Z. Q., et al. (2017). Heteromers of mu opioid and dopamine D1 receptors modulate opioid-induced locomotor sensitization in a dopamine-independent manner. Br. J. Pharmacol. 174, 2842–2861. doi: 10.1111/bph.13908
van Rijn, R. M., Brissett, D. I., and Whistler, J. L. (2012). Distinctive modulation of ethanol place preference by delta opioid receptor-selective agonists. Drug Alcohol Depend. 122, 156–159. doi: 10.1016/j.drugalcdep.2011.09.024
Vaysse, P. J., Gardner, E. L., and Zukin, R. S. (1987). Modulation of rat brain opioid receptors by cannabinoids. J. Pharmacol. Exp. Ther. 241, 534–539.
Vergura, R., Balboni, G., Spagnolo, B., Gavioli, E., Lambert, D. G., Mcdonald, J., et al. (2008). Anxiolytic- and antidepressant-like activities of H-Dmt-Tic-NH-CH(CH2-COOH)-Bid (UFP-512), a novel selective delta opioid receptor agonist. Peptides 29, 93–103. doi: 10.1016/j.peptides.2007.10.012
Vowles, K. E., Mcentee, M. L., Julnes, P. S., Frohe, T., Ney, J. P., and Van Der Goes, D. N. (2015). Rates of opioid misuse, abuse, and addiction in chronic pain: a systematic review and data synthesis. Pain 156, 569–576. doi: 10.1097/01.j.pain.0000460357.01998.f1
Walwyn, W., John, S., Maga, M., Evans, C. J., and Hales, T. G. (2009). Delta receptors are required for full inhibitory coupling of mu-receptors to voltage-dependent Ca(2+) channels in dorsal root ganglion neurons. Mol. Pharmacol. 76, 134–143. doi: 10.1124/mol.109.055913
Wang, D., Raehal, K. M., Lin, E. T., Lowery, J. J., Kieffer, B. L., Bilsky, E. J., et al. (2004). Basal signaling activity of mu opioid receptor in mouse brain: role in narcotic dependence. J. Pharmacol. Exp. Ther. 308, 512–520. doi: 10.1124/jpet.103.054049
Wang, D., Tawfik, V. L., Corder, G., Low, S. A., Francois, A., Basbaum, A. I., et al. (2018). Functional divergence of delta and mu opioid receptor organization in CNS pain circuits. Neuron 98, 90-108.e105. doi: 10.1016/j.neuron.2018.03.002
Wang, H. L., Hsu, C. Y., Huang, P. C., Kuo, Y. L., Li, A. H., Yeh, T. H., et al. (2005). Heterodimerization of opioid receptor-like 1 and mu-opioid receptors impairs the potency of micro receptor agonist. J. Neurochem. 92, 1285–1294. doi: 10.1111/j.1471-4159.2004.02921.x
Williams, J. T., Ingram, S. L., Henderson, G., Chavkin, C., Von Zastrow, M., Schulz, S., et al. (2013). Regulation of mu-opioid receptors: desensitization, phosphorylation, internalization, and tolerance. Pharmacol. Rev. 65, 223–254. doi: 10.1124/pr.112.005942
Xie, W. Y., He, Y., Yang, Y. R., Li, Y. F., Kang, K., Xing, B. M., et al. (2009). Disruption of Cdk5-associated phosphorylation of residue threonine-161 of the delta-opioid receptor: impaired receptor function and attenuated morphine antinociceptive tolerance. J. Neurosci. 29, 3551–3564. doi: 10.1523/JNEUROSCI.0415-09.2009
Yekkirala, A. S., Lunzer, M. M., Mccurdy, C. R., Powers, M. D., Kalyuzhny, A. E., Roerig, S. C., et al. (2011). N-naphthoyl-beta-naltrexamine (NNTA), a highly selective and potent activator of mu/kappa-opioid heteromers. Proc. Natl. Acad. Sci. U.S.A. 108, 5098–5103. doi: 10.1073/pnas.1016277108
Keywords: mu opioid receptor, heteromer, GPCR, delta opioid receptor, morphine, hypertension, addiction, pain
Citation: Ugur M, Derouiche L and Massotte D (2018) Heteromerization Modulates mu Opioid Receptor Functional Properties in vivo. Front. Pharmacol. 9:1240. doi: 10.3389/fphar.2018.01240
Received: 26 July 2018; Accepted: 11 October 2018;
Published: 13 November 2018.
Edited by:
Francisco Ciruela, University of Barcelona, SpainReviewed by:
Mariana Spetea, Universität Innsbruck, AustriaDasiel Oscar Borroto-Escuela, Department of Neuroscience, Karolinska Institute (KI), Sweden
Copyright © 2018 Ugur, Derouiche and Massotte. This is an open-access article distributed under the terms of the Creative Commons Attribution License (CC BY). The use, distribution or reproduction in other forums is permitted, provided the original author(s) and the copyright owner(s) are credited and that the original publication in this journal is cited, in accordance with accepted academic practice. No use, distribution or reproduction is permitted which does not comply with these terms.
*Correspondence: Dominique Massotte, d.massotte@unistra.fr