- 1Department of Pharmacognosy and Molecular Basis of Phytotherapy, Medical University of Warsaw, Warsaw, Poland
- 2Institute of Pharmacy, Freie Universtaet Berlin, Berlin, Germany
Among 65 species belonging to the genus Cornus only two, Cornus mas L. and Cornus officinalis Sieb. et Zucc. (Cornaceae), have been traditionally used since ancient times. Cornus mas (cornelian cherry) is native to southern Europe and southwest Asia, whereas C. officinalis (Asiatic dogwood, cornel dogwood) is a deciduous tree distributed in eastern Asia, mainly in China, as well as Korea and Japan. Based on the different geographic distribution of the closely related species but clearly distinct taxa, the ethnopharmacological use of C. mas and C. officinalis seems to be independently originated. Many reports on the quality of C. mas fruits were performed due to their value as edible fruits, and few reports compared their physicochemical properties with other edible fruits. However, the detailed phytochemical profiles of C. mas and C. officinalis, in particular fruits, have never been compared. The aim of this review was highlighting the similarities and differences of phytochemicals found in fruits of C. mas and C. officinalis in relation to their biological effects as well as compare the therapeutic use of fruits from both traditional species. The fruits of C. mas and C. officinalis are characterized by the presence of secondary metabolites, in particular iridoids, anthocyanins, phenolic acids and flavonoids. However, much more not widely known iridoids, such as morroniside, as well as tannins were detected particularly in fruits of C. officinalis. The referred studies of biological activity of both species indicate their antidiabetic and hepatoprotective properties. Based on the available reports antihyperlipidemic and anticoagulant activity seems to be unique for extracts of C. mas fruits, whereas antiosteoporotic and immunomodulatory activities were assigned to preparations of C. officinalis fruits. In conclusion, the comparison of phytochemical composition of fruits from both species revealed a wide range of similarities as well as some constituents unique for cornelian cherry or Asiatic dogwood. Thus, these phytochemicals are considered the important factor determining the biological activity and justifying the use of C. mas and C. officinalis in the traditional European and Asiatic medicine.
Introduction
Recently published ethnopharmacological studies regarding the genus Cornus (Cornaceae) have shown that among 65 species only two of them have a long tradition in a medicinal use. Cornus mas L. native to southern Europe and southwest Asia, known as cornelian cherry, is used since ancient times (Dragendorff, 1898). The fruits of this plant were used for a broad variety of diseases and complaints in all areas of its geographical distribution. The preparations from C. mas (European cornel, cornelian cherry) were considered as astringent, tonic and antipyretic remedies (Fournier, 1948). Cornus officinalis Sieb. et Zucc., also named Asiatic dogwood (cornel dogwood, Japanese cornel), is a deciduous tree distributed in eastern Asia, mainly in China, as well as Korea and Japan (Ma et al., 2014). It was used as a traditional Chinese medicine for more than 2,000 years to promote a healthy liver and kidney, and is a common ingredient of many TCM prescriptions (Liu X. et al., 2011).
The dogwood genus Cornus L. is a member of Cornales in the Asterids clade (The Angiosperm Phylogeny Group; APG IV, 2016). The dogwood genus is morphologically heterogenous, variable in inflorescences, fruits and chromosomes (Eyde, 1988; Xiang et al., 2005; Ma et al., 2017). Four major lineages of the dogwood genus Cornus L., such as the blue- or white-fruited group, the cornelian cherry group, the big-bracted group and the dwarf dogwood group, have been drawn (Xiang et al., 2006, 2008). However, the systematical classification of flowering plants from Cornales clade and relationships within this taxon seems to be problematic for many years (Xiang et al., 1996, 1998, 2002, 2006; Fan and Xiang, 2001; APG IV, 2016). Despite these controversies, phylogenetic analyses of this clade based on four DNA regions, such as rbcL and matK from chloroplast DNA genome as well as 26S rDNA and ITS (internal transcribed spacer) 1–5. 8S rDNA from the nuclear genome, classified both C. mas and C. officinalis in the group of cornelian cherries (Xiang et al., 1998, 2002, 2006; Fan and Xiang, 2001). In addition, the subgenera Afrocarnia (Harms) Wangerin, Cornus L. and Sinocornus Q.Y. Xiang were included into the group of cornelian cherries. Cornus mas and C. officinalis were classified in the subgenus Cornus L. (Fan and Xiang, 2001; Xiang et al., 2006). Both of them are temperate deciduous small shrubs or trees blooming before leaf development. The morphological characters, such as umbellate cymes terminal subtended by four not showy scale-like bracts, red or purple black fruits and the wall of fruit stone riddled with cavities, supported the membership of these species in the monophyletic group of cornelian cherries. All these data strongly supported the sister phylogenetic relationship of C. mas and C. officinalis (Xiang et al., 2005). The genus Cornus is widely distributed in the northern hemisphere. Fossil fruit stones similar to the contemporary fruit stones of C. mas are likely to derive from the late Miocene of Poland, as well as from Pliocene and Pleistocene of France, Germany and Netherlands. Evidence from analyses of fossils, phylogeny and biogeographic distribution support an origin and early diversification of Cornus likely in Europe. It is suggested that the ancestor of the living cornelian cherries spread from Europe to eastern Asia around the mid-Oligocene and the mid-Miocene. Cornus mas is a single representative species of cornelian cherry in Europe. On the other hand, C. officinalis in eastern Asia represents the Miocene migrant from Europe (Xiang et al., 2005). Based on the different geographic distribution of the closely related species but clearly distinct taxa, the ethnopharmacological use of C. mas and C. officinalis seems to be independently originated. In the traditional medicine of Europe and Middle East only fruits of C. mas were used and in the traditional medicine of China reports describe only preparations of fruits from C. officinalis for medicinal application.
Plant materials belonging to the genus Cornus are characterized by the presence of secondary metabolites, in particular iridoids, anthocyanins, tannins and flavonoids. Although many reports on the quality of fruits of C. mas were performed due to the fact of their value as edible fruits, as well as few studies compared their properties with other edible fruits used for a production of wines, liquors or jams (Tarko et al., 2014; Cosmulescu et al., 2017), the detailed phytochemical profiles of C. mas and C. officinalis, in particular fruits, have never been compared. The chemical composition of plant materials obtained from C. mas and C. officinalis can be significantly different depending on the geographic location, climatic factors, season, as well as cultivation practice. For this reason, the phytochemical composition is considered an important factor determining the biological activity and justifying the traditional use of these species.
All these facts taking together make interesting to compare the therapeutic use of both traditional species based on their chemical composition. This review particularly highlights the similarities and differences in phytochemicals found in fruits of C. mas and C. officinalis in relation to their biological effects based on reports available in this field.
Similarities and Differences in the Chemical Composition of Fruits From C. mas and C. officinalis
General
The main secondary metabolites found in dogwoods seem to be iridoid and non-flavonoid glucosides. Even though the blue-fruited dogwoods contain non-flavonoid glucoside salidroside derived from shikimic acid, iridoid glucosides were found to be unique in all red-fruited dogwoods, and they are derived from mevalonic acid (Bate-Smith et al., 1975; Jensen et al., 1975). The dogwoods iridoids were classified into three groups, including cornin and its precursor dihydrocornin, monotropein and its precursor geniposide as well as secoiridoids, secologanin and morroniside (Bate-Smith et al., 1975). Thus, the presence of iridoids (Figures 1, 2) in red-fruited dogwoods is considered a symplesiomorphy (Xiang et al., 1996), and confirms the close taxonomical relationship of C. mas and C. officinalis. The representative compounds of this group, such as loganin, loganic acid, cornin, sweroside, and cornuside, have been found in both species.
Furthermore, the polyphenolic compounds are represented by anthocyanins, flavonoids and phenolic acids in fruits of C. mas and C. officinalis. Pelargonidin 3-O-galactoside followed by cyanidin 3-O-galactoside and delphinidin 3-O-galactoside were the most abundant anthocyanins in fruits of both species. Among flavonoids, quercetin, and kaempferol glycosides were detected in both species. Taking into consideration that flavonoids are widely distributed in plants, their occurrence was considered the similar characteristic for both species. Nevertheless, some discrepancy in the flavonoid composition occurs. Even more flavonoids have been referred by Huang et al. (2018) but full availability of the studies concerning their identification or isolation is rather limited.
Last but not least, the similar constituents of C. mas and C. officinalis are considered triterpenoids, in particular represented by ursolic acid detected in both species, and carbohydrates. It is worth to note that neither in fruits of C. mas nor in fruits of C. officinalis phenylpropanoid esters have been reported to date, even though they usually co-exist with iridoids as it is observed in some plants from Oleaceae, Scrophulariaceae, Verbenaceae, Plantaginaceae, and Lamiaceae. This might be justified by the less significant role of shikimate pathway in biosynthesis of compounds in red-fruited dogwoods. Table 1 summarizes the key phytochemicals of fruits from C. mas and C. officinalis that can be found in the literature. The names of compounds have been provided as they were described in the referred literature. Due to the fact that HPLC-MS is currently one of the most popular method for elucidation of chemical composition of plant preparations, the molecular weights of phytochemicals were also listed (Table 1) to help reader with comparing experimental data in order to preliminary identification detected constituents.
Similarities Of Chemical Composition
Iridoids
It is believed that particularly loganin (1) plays a crucial role in the formation sequences of highly oxidized glucosides of iridoids as well as in the biosynthesis of secoiridoids glucosides and indole alkaloids. The cyclization to a hemiacetal is likely to take place through the incorporation of an oxygen function into the C-8 position of secologanin (27) derived from loganin (1) to generate further iridoid derivatives (Inouye et al., 1974). Secoiridoids are believed to be even more advanced due to the more complicated biostynthetic steps (Xiang et al., 1996). Thus, the most closely related in the iridoids transformation pathway compounds, such as loganin (1), loganic acid (3), cornin (4), sweroside (5), and representative of secoiridoids, cornuside (6), have been detected both in fruits of C. mas and C. officinalis (Deng et al., 2013; Ma et al., 2014; Perova et al., 2014; Sochor et al., 2014; Kucharska et al., 2015; Jiang et al., 2016). However, it should be noted that in the 26 cultivars of C. mas it was shown that the most abundant iridoid was loganic acid (3) (81.5–461.1 mg/100 g fw) constituting 88–96% of total iridoids (86.9–493.7 mg/100 g fw). Cornuside (6) has already followed loganic acid (3) but its content was much lower than loganic acid (3) and maximally reached 36.2 mg/100 g fw (West et al., 2012a; Kucharska et al., 2015). Only two reports describe the identification of loganin (1) and sweroside (5) in fruit of C. mas (Deng et al., 2013; Perova et al., 2014). Nevertheless, the reason of limited data on identification of loganin and sweroside in fruits of cornelian cherry is due to their low concentration compared to loganic acid. More detailed studies are needed in this field.
Anthocyanins
The mature fruits from both C. mas and C. officinalis are dark red colored and for this reason they might be easily confused. Thus, in addition to iridoids (Figures 1, 2), anthocyanins (Figure 3), red colorant compounds, are widely distributed in fruits of these dogwoods. The content of anthocyanins increases when the fruits change their color from light yellow to dark red (Gunduz et al., 2013). Fruits of cornelian cherry and Asiatic dogwood contain in particular pelargonidin (52) and cyanidin (57) derivatives. Both in C. mas and C. officinalis pelargonidin 3-O-galactoside (53), cyanidin 3-O-galactoside (58), and delphinidin 3-O-galactoside (64) were detected and quantified. Pelargonidin 3-O-galactoside (53) (1.62 mg/g) and cyanidin 3-O-galactoside (58) (1.66 mg/g) have been noted as the most abundant anthocyanins of C. mas fresh fruit (Jayaprakasam et al., 2006; Vareed et al., 2006; Sozanski et al., 2014; Kucharska et al., 2015). The amount of pelargonidin 3-O-galactoside (53) in fruit of C. officinalis (0.78 mg/g) was approximately half of the concentration determined in fruit of C. mas. The other detected anthocyanins of C. officinalis fruit were present even at lower concentrations (0.15–0.21 mg/g; Vareed et al., 2006). In the literature, more attention has been focused on anthocyanins of C. mas (Pantelidis et al., 2007; Popović et al., 2012; Bilejić et al., 2015; Kucharska et al., 2015). These pigments were identified using methods such as HPLC, LC-ES/MS and NMR (Seeram et al., 2002; Kucharska et al., 2015), HPLC (Tural and Koca, 2008; Capanoglu et al., 2011) and LC-PDA-MS (Pawlowska et al., 2010). In some cultivars of the southeastern Europe peonidin 3-O-glucoside (62) followed by cyanidin 3-O-galactoside (58) was established as the major constituent of C. mas fruits (Drkenda et al., 2014).
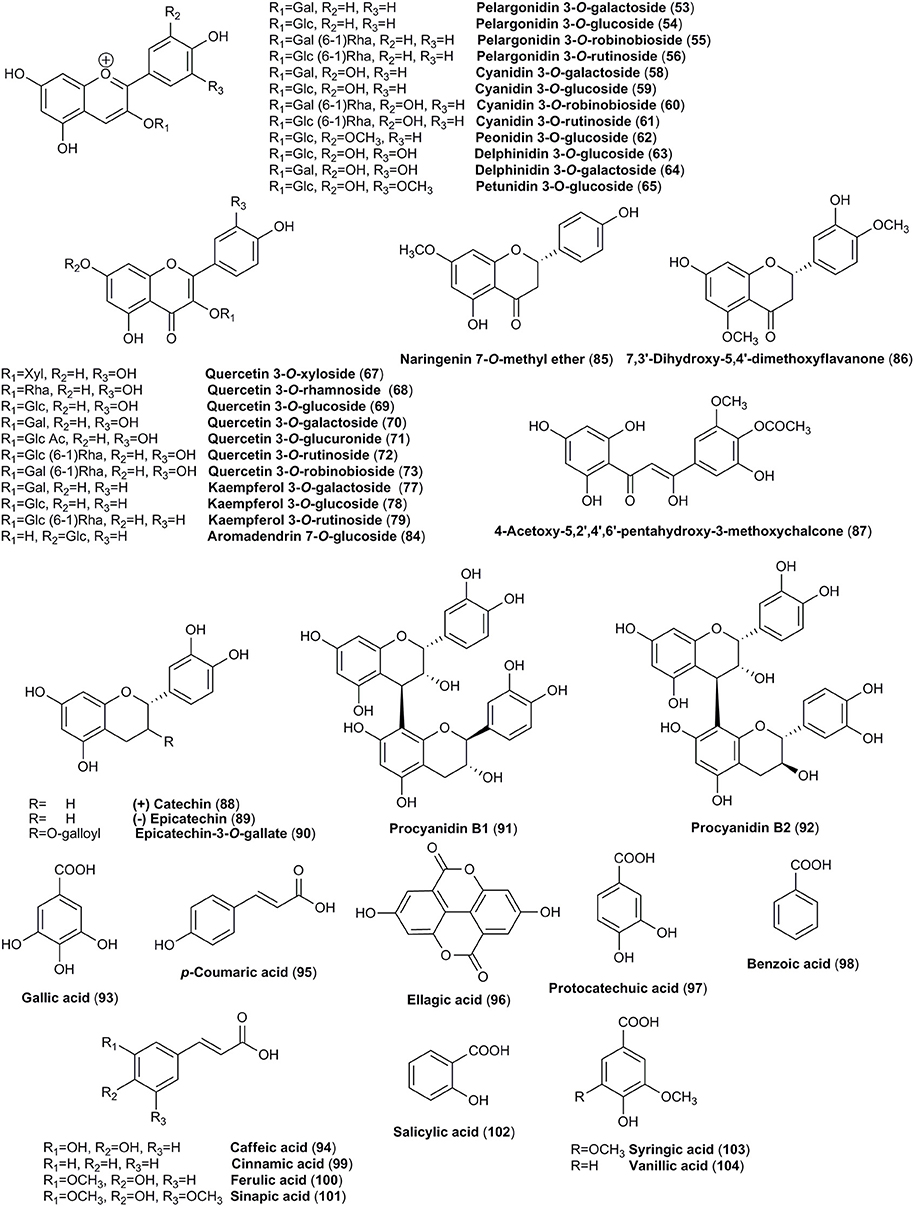
Figure 3. The chemical structures of anthocyanins, flavonoids, and phenolic acids identified in fruits of C. mas and/or C. officinalis.
Flavonoids
Cornus species have been proven to contain flavonols mostly quercetin (66) and kaempferol (76) derivatives (Figure 3). Most of reported compounds were identified as monoglycosides containing glucose, galactose, rhamnose, and glucuronic acid as a sugar moiety. The quercetin glycosides (69–71) as well as kaempferol 3-O-β-D-galactoside (77) were determined in both species (Pawlowska et al., 2010; Xie et al., 2012; Ma et al., 2014). The trace amounts of aglycone of flavonols such as quercetin (66) was found in fruits of both C. mas and C. officinalis (Xie et al., 2012; Rudrapaul et al., 2015).
Phenolic Acids
Three different groups of phenolic acids (Figure 3), including benzoic acid, trans-cinnamic acid and ellagic acid derivatives have been described in the fruits of C. mas and C. officinalis. The typical representatives of each group such as gallic acid (93), caffeic acid (94), p-coumaric acid (95), ellagic acid (96), and protocatechuic acid (97) have been found in both dogwoods (Liu Z. et al., 2011; Cao et al., 2012; Radovanović et al., 2013; Milenković-Andjelković et al., 2015; Rudrapaul et al., 2015; Antolak et al., 2017; Cosmulescu et al., 2017). Ellagic acid (96), which is a marker of ellagitannins occurrence, detected in the fruits of cornelian cherry suggests the presence of ellagitannins in this plant material. However, this hypothesis requires further investigation.
Triterpenoids
Additionally, some other compounds such as triterpenoids (Figure 4) and carbohydrates have been identified in both species. Among terpenoids only ursolic acid (112) has been reported in the fruits of cornelian cherry and Asiatic dogwood (Jayaprakasam et al., 2006; Wang H. et al., 2008; Jang et al., 2014; He et al., 2016). This compound was often reported as an active constituent found in ethanolic and methanolic extracts from fruits of C. officinalis (Gao et al., 2008). In addition, other three triterpenoid acids (113–115), including oleanolic acid, have been identified in C. officinalis (Jayaprakasam et al., 2006; Wang H. et al., 2008; Jang et al., 2014; He et al., 2016).
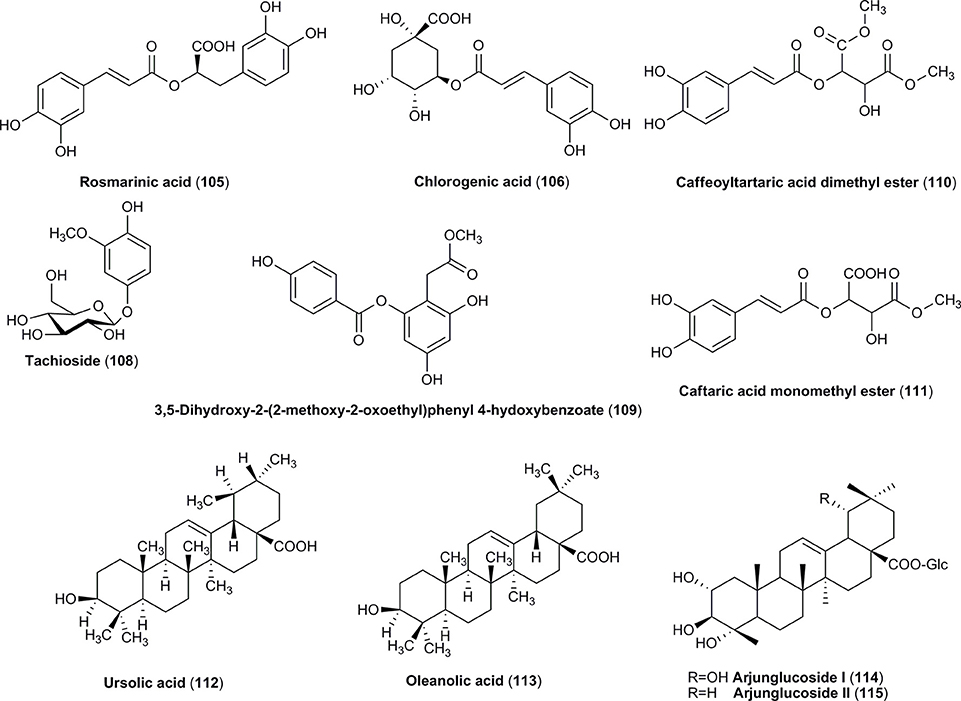
Figure 4. The chemical structures of phenolic compounds and terpenoids identified in fruits of C. mas and/or C. officinalis.
Carbohydrates
Carbohydrates are the crucial compounds determining the quality of fruits due to the fact that the sugar content is a major parameter affecting a direct consumption of fruits in addition to food preservation aspects (Çopur et al., 2003; Brindza, 2007; Bilejić et al., 2011, 2015). Thus, monosaccharides and polysaccharides are present in the fruits of both species. The fruits of C. mas contain the atypical fibers such as calcium pectate (116) (1.31%; Bilejić et al., 2011; Jaćimović et al., 2015). The crude fiber (0.36–0.76%) and total pectin (0.26–1.04%) contents were evaluated in C. mas fruits grown in different regions of Europe (Çopur et al., 2003; Sochor et al., 2014). Therefore, the juice of C. mas is considered an alternative fermentation substrate for the production of functional beverage. The simultaneous decreasing total sugars content and increasing contents of lactic and acetic acids were studied in the juice as well as unripe cornelian cherries fruits fermented by Lactobacillus sp. (Nouska et al., 2016; Czyzowska et al., 2017). It is worth to note that fermented cornelian cherry-derived products of iridoid glycosides and phenolic acids may complement gut microbiota and in this manner play a role of new probiotic functional products (Czyzowska et al., 2017). Additionally, the fibers and particularly oligosaccharides, which are generally found in fruits, are considered the non-digestible food ingredient stimulating the growth or selective activity of gut microbiota (Gibson, 2008). The dietary fiber in both cornelian cherries (Nawirska-Olszanska et al., 2010) and cornel dogwood fruits (Shao, 2011) is the important constituent of a diet determining human well-being due to a wide range of metabolic health benefits such as reduction of cholesterol level, blood pressure and body weight. Thus, the potential prebiotic activity of constituents such as dietary fiber from fruits of both dogwoods should not be excluded. On the other hand, a purification and isolation of bioactive polysaccharides of C. officinalis fruit (Yang et al., 2010; Li et al., 2012; You et al., 2013; Yin et al., 2016) have been also shown. The list of polysaccharides (117–119) identified in the fruits of C. officinalis is presented in Table 1. The most abundant monosaccharide components of these polysaccharides were glucose, rhamnose and arabinose (Yang et al., 2010; Li et al., 2012). The monosaccharide profile of C. mas fruits have been represented by glucose, fructose and sucrose (Bilejić et al., 2011; Perova et al., 2014; Tarko et al., 2014; Antolak et al., 2017).
Differences In Chemical Composition
General
Despite a wide range of similarities and close phylogenetic relationship of C. mas and C. officinalis, few differences in chemical composition of fruits can be found. The most valuable characteristic established only in fruits of C. officinalis seems to be the presence of tannins (120–139) as well as unique iridoids. Morroniside (8) is one of the most important iriodid glucoside reported in the fruits of C. officinalis. Additionally, 43 other iridoids (9–51) have been identified in this plant material. It should be underlined that co-existence of iridoids and tannins seems to be very rare in plants. Furthermore, 5-hydroxymethylfurfural (163) and steroids, such as β-sitosterol (165) and daucosterol-6′-malate (166) are unique for C. officinalis.
On the other hand, flavan-3-ols derivatives, such as procyanidin B1 (91) and B2 (92), carotenoids (140–149) and fatty acids (150–162) have been identified only in fruits of C. mas. It is worth to note that many reports provide data on ascorbic acid or pectin content in fruits of C. mas due to their dietary role. As far as the Asiatic dogwood is concerned, these data are completely missed.
The one comparative study on quantities of secondary metabolites in aqueous extracts from leaves of C. mas and C. officinalis have revealed that extracts of C. mas are characterized by lower content of flavonoids, hydroxycinnamic acids, total polyphenols and tannins than extracts of C. officinalis (Forman et al., 2015). On the other hand, no comparative quantitation of major secondary metabolites, except for anthocyanins (Seeram et al., 2002; Vareed et al., 2006) of dogwood fruits is available to date. Thus, further comparative elucidation of chemical composition as well as quantification of phytochemicals in fruits of both species still seem to be a challenge.
Unique Iridoids of C. officinalis
Although iridoids were classified as similar characteristic for both species, much more not widely known iridoids have been found only in fruits of C. officinalis. In particular, in contrary to the cornelian cherry fruits the major iridoid constituents of Asiatic dogwood fruits were isomers of α and β of morroniside (8) as well as their methyl and ethyl derivatives (9–15) (Du et al., 2008; West et al., 2012a,b; Xie et al., 2012; Jiang et al., 2016; Park et al., 2016; Ji et al., 2017). Additionally, apart from typical iridoids described previously, less-known compounds such as logmalicids A and B (19–20) as well as cornusfurosides A-D (22–24) were found in fruits of C. officinalis (Ma et al., 2014; He et al., 2017). Fifteen rare iridoid glucoside dimers, named cornusides A-O (31–45) (Figure 2), have been isolated from an aqueous extract of C. officinalis fruits (Ye et al., 2017).
Tannins of C. officinalis
Similarly, tannins are attributed only to the fruits of C. officinalis and have been widely described. Therefore, tannins (Figures 5, 6) in the fruits of C. officinalis constitute the most important difference between both plant materials. Despite the mean content of tannins in fruits of C. mas was established as 0.34% (Gunduz et al., 2013), the detailed reports on the identification of tannins in the fruits of C. mas have been limited in contrary to C. officinalis. The Asiatic dogwood is particularly considered a rich source of hydrolyzable tannins (Okuda et al., 1981, 1984). Twenty tannins (120–139), including ellagitannins and gallotannins, have been described in C. officinalis. The derivatives of galloylglucose (121–125), particularly digalloylglucose, have been identified to date. Tellimagrandin I (126) and II (127) as well as gemin D (137) and oenothein C (138) are representative of ellagitannins (Okuda et al., 1981, 1984; Lee et al., 1989; Cao et al., 2012; Bhakta et al., 2017). Most of unique dimeric and trimeric hydrolyzable tannins named cornusiins A–G (128–134) have been found only in the fruits of C. officinalis to date (Okuda et al., 1984; Hatano et al., 1989a,b, 1990). One of the bioactive constituents which should be mentioned due to the fact of potential biological activity is also 7-O-galloyl-D-sedoheptulose (120) (Yamabe et al., 2009; Yokozawa et al., 2010; Liu Z. et al., 2011; Park et al., 2015). It is worth to note that some tannins were detected in the fruits of cornelian cherry (Kucharska, 2012). However, their detailed identification was not conducted, and in fact the data concerning tannins in C. mas are not available.
Carotenoids and Fatty Acids Of C. mas
Some lipophilic compounds such as carotenoids as well as fatty acids have been identified in the fruits of C. mas in contrast to C. officinalis. One report showed the determination of ten carotenoids (140–149) in the fruits of C. mas (Horváth et al., 2007), in addition to their quantitation in fresh and dried fruits (Rosu et al., 2011; Lofti et al., 2014). Carotenoids are believed to be regulators of lipid metabolism and antioxidants. Among carotenoids the most abundant in a diet is β-carotene (147), known singlet oxygen quencher and precursor for vitamin A (Grune et al., 2010). Despite limited data on this group of compounds in fruits of C. mas, their potential role in biological effectiveness of C. mas fruits should be considered. To the best of our knowledge there is no report on carotenoids in C. officinalis. Similarly, the available data on fatty acids in the fruits of Asiatic dogwood have been limited and the detailed analysis seems to be necessary. Fatty acids (150–162) such as linoleic acid (152), oleic acid (155) and palmitic acid (156) have been the most abundant in the fruits of C. mas (Krivoruchko, 2014).
Diversity of Phenolic Compounds In C. mas and C. officinalis
Nine quercetin derivatives (67–75) have been identified in the fruits of C. mas (Pawlowska et al., 2010). Simultaneously, kaempferol 3-O-β-D-galactoside (77), myricetin (81), and naringenin 7-O-methylether (85) have been detected in the fruits of C. mas along with 7,3′-dihydroxy-5,4′-dimethoxyflavanone (86) (Pawlowska et al., 2010; Rudrapaul et al., 2015). It is worth to note that 2R, 3S-trans-aromadendrin (83) and its 7-O-β-D-glucoside (84), which are not so widely distributed in plants compared to quercetin and kaempferol glycosides, were determined in the fruits of C. mas (Pawlowska et al., 2010; Rudrapaul et al., 2015). On the other hand, some glycosides, such as quercetin 3-O-β-D-glucuronide methyl ester (74), quercetin 3-O-β-D-(6″-n-butyl glucuronide) (75), kaempferol 3-O-β-D-rutinoside (79) as well as kaempferide (80) have been reported in fruits of C. officinalis according to the available literature (Lin et al., 2011; Xie et al., 2012; Ma et al., 2014).
Flavan-3-ols such as catechin (88) and epicatechin (89) have been identified and quantified in the fruits of C. mas (Capanoglu et al., 2011; Milenković-Andjelković et al., 2015). In the study of Milenković-Andjelković et al. (2015), catechin (88) was the predominant favan-3-ol in the fruit extracts of C. mas (Figure 3). Additionally, procyanidin B2 (92) was found only in the fruit extracts of C. mas (Radovanović et al., 2013; Milenković-Andjelković et al., 2015). The degree of polymerization in the fruits of cornelian cherry was assessed at the level of 63 total bound (epi)catechin units/end units (Capanoglu et al., 2011).
The mixture of benzoic acid (98) and cinnamic acid (99) derived phenolic acids, including ferulic acid (100), sinapic acid (101) as well as vanillic acid (104), was identified in the fruits of C. mas (Radovanović et al., 2013; Milenković-Andjelković et al., 2015; Rudrapaul et al., 2015; Cosmulescu et al., 2017). In addition, the esters formed between phenolic acids' particles, such as rosmarinic acid (105) and chlorogenic acid (106) have been found only in the fruits of cornelian cherry (Figure 4; Deng et al., 2013; Antolak et al., 2017; Popović et al., 2017; Bajić-Ljubičić et al., 2018). The unique derivatives of phenolic acids such as tachioside (108), caffeoyltartaric acid dimethyl ester (110), and caftaric acid monomethyl ester (111) are characteristic for Asiatic dogwood's fruits (Figure 4; Lin et al., 2011; Ma et al., 2014; Park et al., 2016).
Other Constituents
Due to the fact of the usage of C. mas for a production of concentrates, jams, and even more often fruit juice and alcoholic beverages, rheological and physicochemical properties related to the quality of liquid foods have been reported (Güleryüz et al., 1998; Brindza, 2009; Kalyoncu et al., 2009; Karadeniz et al., 2009; Yilmaz et al., 2009; Hassanpour et al., 2012; Gastoł et al., 2013; Bilejić et al., 2015; Rad et al., 2016; Bozdogan, 2017). In particular, apart from titrable acidity, soluble solid content as well as total sugars and pectin content, vitamin C was often quantified in the fruits of C. mas. They were characterized by the high content of ascorbic acid ranging from 43.6 to 76.7 mg/100 g, or even reaching 112 mg/100 g in Turkish cultivars (Güleryüz et al., 1998; Çopur et al., 2003; Demir and Kalyoncu, 2003; Brindza, 2007, 2009; Yalcinkaya et al., 2009; Yilmaz et al., 2009; Rop et al., 2010; Dokoupil and Rezníček, 2012; Hassanpour et al., 2012; Bilejić et al., 2015; Kostecka et al., 2017). However, it was noted that cornelian cherry fruits from Greece were characterized by higher content of vitamin C than these ones from Poland (Kostecka et al., 2017). On the other hand, some physicochemical properties of cornel dogwood wine during its fermentation and storage were studied once (Zhang Q. -A. et al., 2013).
Few reports considered the potential bioactivity of 5-hydroxymethylfurfural (163) isolated from fruits of C. officinalis (Figure 7; Du et al., 2008; Cao et al., 2011, 2012; Jiang et al., 2016). Additionally, steroid compounds including β-sitosterol (165) and daucosterol-6′-malate (166) have been identified only in fruits of C. officinalis (Figure 7; Lee et al., 1989; Lin et al., 2011; Xie et al., 2012).
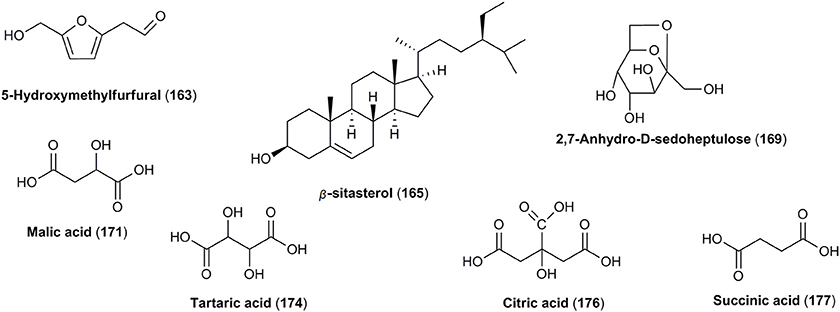
Figure 7. The chemical structures of other compounds identified in fruits of C. mas and/or C. officinalis.
Similarities and Differences of Pharmacological Effects or Biological Activities
General
Taking into account different medical approaches in the traditional medicine in ancient Europe and Asia, in particular China, it is rather interesting that there are some similar indications reported for both species. They have been traditionally used to improve liver and kidney functions and are considered therapeutically usefull due to their antidiabetic and antimicrobial properties. Considering the phytochemical similarities between both species the comparable use of both species is scientifically reasonable. For this reason, the antidiabetic effect as well as renal and hepatic protective activities of both species were particularly considered in the reported studies. In addition, the positive effects on central nervous and cardiovascular systems of both species have been noted. The background of these effects seems to be the antioxidant activity as well as an amelioration of inflammatory processes through regulation of NF-κB and MAPK signaling pathways by constituents of extracts from fruits of both species. The isolated constituents of fruits, such as cornuside (6), loganin (1), ursolic acid (112), anthocyanins, and more specific for C. officinalis morroniside (8), and 7-O-galloyl-D-seduheptulose (120), demonstrated the biological impact. However, the pharmacological effect of other phytochemicals found in fruits of both species should not be excluded in the provided reports. Thus, the accurate qualitative and quantitative characterization of phytochemicals in extracts is required in the studies, and the beneficial role of complex phytochemical formulation providing the synergistic or additive effect should be underlined.
The antimicrobial and antitumor activities of both species seem to be mostly related to the presence of polyphenolic compounds in fruit extracts. However, these activities of both species do not seem to be well-established.
Table 2 demonstrates similarities and differences of the biological or pharmacological activities between the preparations from fruits of both species. The biologically or pharmacologically active groups of compounds or isolated constituents of fruit extracts were included in Table 2.
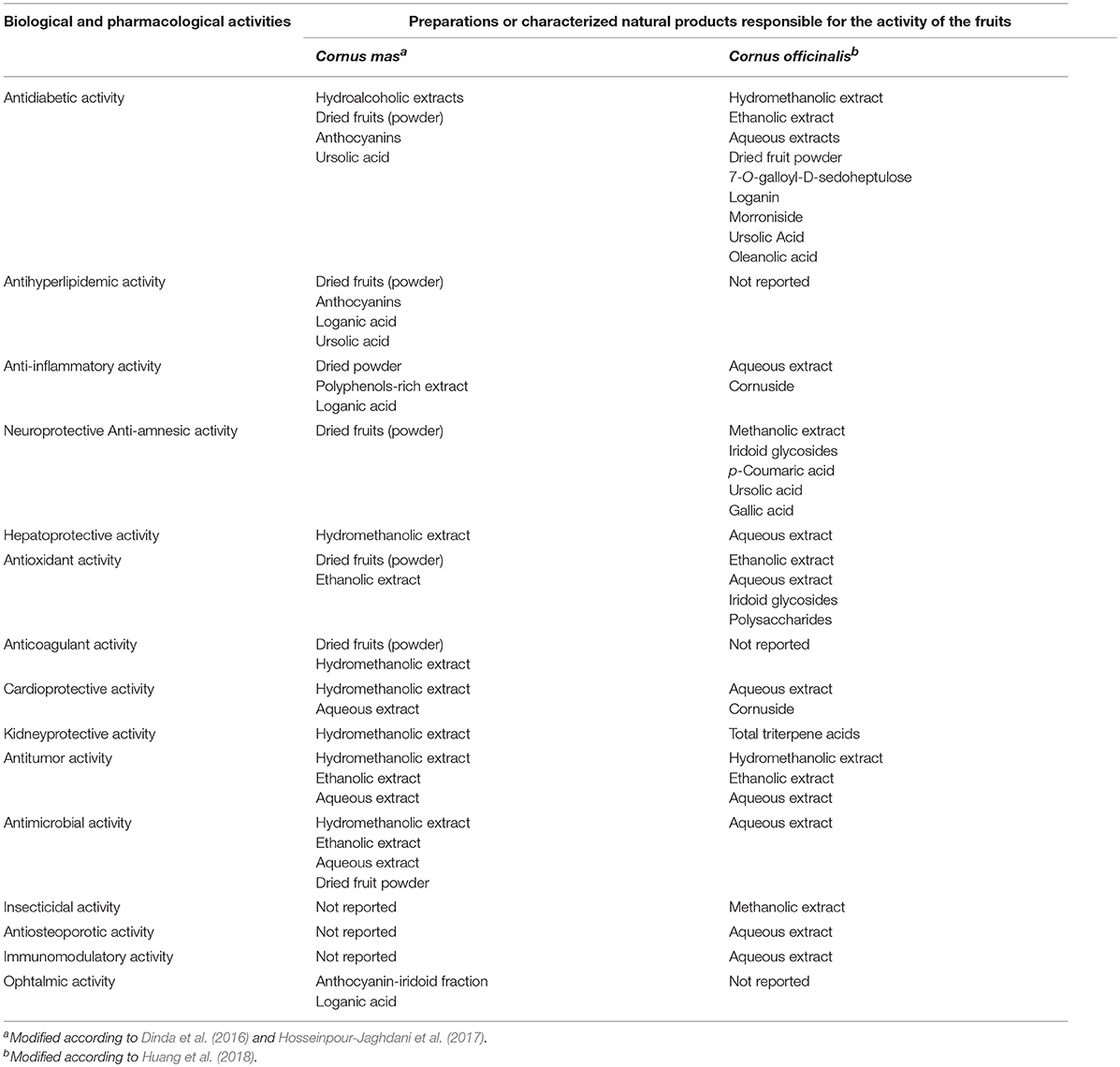
Table 2. Similarities and differences of the biological or pharmacological activities of fruits from C. mas and C. officinalis.
Similar Biological or Pharmacological Activities of C. mas and C. officinalis
Antidiabetic Effect
Comparing the reported traditional therapeutic use of fruits from C. mas and C. officinalis it is obvious that the treatment of metabolic disorders played an important role. Based on different fundamentals of ancient medicine in China and Europe or western Asia and despite missing modern diagnostic tools for precise detection of diabetes, the physicians recognized definitely metabolic disease in the distant past and tried to treat their accompanying symptoms. Under this point of view the use of fruits from both species should be discussed in order to their potential usefulness in the treatment of metabolic diseases in different geographic regions. Due to the wide spectrum of natural compounds and modern investigations of their biological activity an antidiabetic effect of cornelian cherry seems to be proven. Based on some animal experiments investigating the effect of preparations made from C. mas fruits the blood glucose decreasing activity and enhancement of insulin sensitivity were demonstrated (Jayaprakasam et al., 2006; Mirbadalzadeh and Shirdel, 2012; Narimani-Rad et al., 2013; Asgary et al., 2014). The same findings were reported also for fruits of C. officinalis (Park et al., 2011; Gao et al., 2012; Liu et al., 2012; Han et al., 2014). Data from clinical studies evaluating the therapeutic effect of the herbal preparation are available only for C. mas. One study on the fruit extract showed its antidiabetic effect associated with the reduction of risk factors for secondary diseases (Soltani et al., 2015). Comparing the chemical constituents present in both species and their biochemical or pharmacological effects some similarities can be seen. In the extract of both species polyphenolic compounds (e.g., flavonoids, anthocyanins), which inhibit α-glucosidase activity combined with decreasing postprandial blood glucose level, are present (Park et al., 2011; Asgary et al., 2014; Shishehbor et al., 2016). Ursolic acid (112) also present in both species is capable to stimulate the glucose uptake through the phosphorylation of insulin receptor (Zhang et al., 2006). Specific antidiabetic effects were reported for 7-O-galloyl-D-seduheptulose (120) and iridoids [e.g., loganin (1), morroniside (8)] present in C. officinalis (Yamabe et al., 2009; Park et al., 2010, 2013; He et al., 2016) as well as for cyanidin 3-O-galactoside (58) and cyanidin 3-O-glucoside (59) present in C. mas (Jayaprakasam et al., 2005). On the other hand, loganin (1) and ursolic acid (112) were not able to affect an expression of phosphoenolpyruvate carboxykinase (PEPCK) in rat hepatoma H4IIE cell line on the contrary methanolic extract of C. officinalis fruit (50 μg/ml) which exerted insulin mimetic effect in this manner, and one of its fraction enhanced insulin secretion by rat insulin-secreting BRIN-BD11 cells in response to high glucose concentration (16.7 mM; Chen et al., 2008). Although there is only one published clinical study of the fruit extract from C. mas the antidiabetic effect seems to be proven (Soltani et al., 2015).
The prolonged high blood glucose concentration leads to progressive lesions in heart, kidney, blood vessels and other organs. Moreover, increased intracellular reducing sugars and their derivatives may participate in glycation and formation of advanced glycation endproducts (AGEs) which play an important role in further development of chronic diabetic complications, atherosclerosis and aging process. The phytochemicals of C. officinalis fruits such as gallotannins, particularly 7-O-galloyl-D-sedopheptulose (120), and morroniside (8) inhibited AGE formation or inhibited expression of receptors for AGEs (Yamabe et al., 2009; Lee et al., 2011; Park et al., 2012b, 2015; Lv et al., 2016). Due to the series of diabetes type II complications, the inhibition of AGE formation seems to be a promising target for therapeutic intervention supporting the AGE-related disorders.
Antioxidant Activity
In addition to the studies of total phenolic, flavonoid, and anthocyanins content, antioxidant properties of the extracts from cornelian cherries were determined in a correlation with these phytochemicals. Apart from polyphenols, ascorbic acid and carotenoids in C. mas play a significant antioxidant role. Thus, both antioxidants content and antioxidant capacity of dogwoods fruits are factors determining the quality of cultivars and basic physicochemical properties. In vitro assays such as free radical scavenging with DPPH (2,2-diphenyl-1-picrylhydrazyl radical), ferric ion reducing antioxidant power (FRAP), total antioxidant capacity with ABTS+ (2,2-azinobis(3-ethylbenzothiazoline-6-sulfonic acid) radical cation) and oxygen radical absorbance capacity (ORAC) were the most frequently used methods in a wide range of studies on C. mas (Dragović-Uzelac et al., 2007; Pantelidis et al., 2007; Hamid et al., 2011; Celep et al., 2012; Popović et al., 2012; Gunduz et al., 2013; Pyrkosz-Biardzka et al., 2014; Stankovic et al., 2014; Milenković-Andjelković et al., 2015; Hosu et al., 2016; Moldovan et al., 2016) and C. officinalis (Lee et al., 2006; West et al., 2012a; Hwang et al., 2016). Moreover, the hydromethanolic extracts of some cornelian cherry fruit cultivars from Turkey demonstrated significant H2O2 scavenging activity (79.1%; Ersoy et al., 2011). Galactosides of cyanidin (58) and pelargonidin (53) detected in both species inhibited lipid peroxidation by 70.2 and 40.3% in an iron-catalyzed liposomal model, respectively (Seeram et al., 2002). On the other hand, the antioxidant properties, including protection of catalase and glutathione peroxidase, of C. officinalis fruits are also assigned to polysaccharides (Li et al., 2012; Wu et al., 2013) and ursolic acid (Yu et al., 2009).
Anti-inflammatory Activity
Anti-inflammatory and analgesic activities of extracts are reported for both species. As mentioned before most of the results were obtained in cell culture or biochemical experiments. The beneficial effect of aqueous extract from fruits of C. officinalis and its iridoid constituent cornuside (6) on protein expression of COX-1 and COX-2 as well as PGE2 production in LPS-induced macrophages RAW 264.7 was shown (Sung et al., 2009; Choi et al., 2011). Similarly, ursolic acid (112), which was isolated from an ethanolic extract of C. officinalis inhibited NF-κB activation in LPS-stimulated peritoneal macrophages. Oral administration of ursolic acid (112) significantly inhibited 2,4,6-trinitrobenzenesulfonic acid (TNBS)-induced colon shortening and MPO activity in mice, and also suppressed TNBS-induced COX-2 and iNOS expression as well as NF-κB activation in colon tissues. Ursolic acid (112) may ameliorate colitis by regulating NF-κB and MAPK signaling pathways via the inhibition of LPS binding to toll-like receptors 4 (TLR4) on immune cells (Jang et al., 2014). Taking into consideration a wide range of ingredients and general mechanisms responsible for induction or prevention of inflammation, in vitro results fit to conclusions obtained from animal experiments and a clinical study (Sung et al., 2009; Sozanski et al., 2014, 2016; Moldovan et al., 2016). In particular, cornuside (6) was indicated as an immunomodulatory dogwood-derived compound in a model of sepsis in rats (Jiang et al., 2009). The link between chronic inflammation and cancer involves cytokines and mediators of inflammatory pathways. Plants contain numerous secondary metabolites shown to modulate inflammatory pathways. Studies have shown that these metabolites, among which some are used in traditional medicine, can reduce inflammation and carcinogenesis (Desai et al., 2018). Phenolic and other compounds present in the extracts of the dogwood fruits target various inflammation-related molecules and pathways associated with cancer (Choi et al., 2011). Polyphenols modulate important cellular signaling processes such as NF-κB (nuclear factor κ light-chain enhancer of activated B cells) activation, chromatin structure, glutathione biosynthesis, Nrf2 (nuclear redox factor) activation, direct ROS scavenging or antioxidant effect via glutathione peroxidase activity. Finally, they regulate inflammatory genes in macrophages and lung epithelial cells. Thus, recent data suggest that polyphenols can play a role of modifiers of signal transduction pathways to elicit their beneficial effects. However, to correlate this with beneficial effects in humans their metabolism and bioavailability need to be considered (Rahman et al., 2006).
Renal and Hepatic Protective Activities
The metabolism of xenobiotics is often linked with the production of harmful radicals or metabolites damaging such organs like liver and kidney resulting in inflammation or carcinogenic transformation. As mentioned before natural products present in fruits of both species are able to inhibit both damage generated by radicals and inflammatory processes in vitro. Animal experiments performed with methanolic extracts of C. mas fruits are reported to show hepatoprotective activities against chemically induced hepatotoxicity after oral application. The dosage used in the investigations with rats ranging from 200 to 700 mg/kg body weight (Abbasi et al., 2014; Alavian et al., 2014; Saei et al., 2016). Similar reports concerning C officinalis are also published (Lee N. H. et al., 2012). The protective effect on kidneys is described for both species using comparable animal experimental settings (Yokozawa et al., 2008; Jiang et al., 2012; Abbasi et al., 2014; Es Haghi et al., 2014). In particular, the iridoids [loganin (1) and morroniside (8)] present mainly in the fruits of C. officinalis as well as 7-O-galloyl-D-sedoheptulose (120) are able to prevent renal damage in diabetic rats (Xu et al., 2006; Park et al., 2012a).
Antimicrobial Effect
Antibacterial effects are also reported for extracts of the fruits from both species without remarkable activity (Mau et al., 2001; Krzyściak et al., 2011; Turker et al., 2012; Radovanović et al., 2013; Kyriakopoulos and Dinda, 2015; Milenković-Andjelković et al., 2015; Hosseinpour-Jaghdani et al., 2017). Due to the presence of a complex of different phenolic compounds in dogwood fruits the antimicrobial activity is not surprising. All phenolic compounds to a certain extent express this activity (Radulović et al., 2013). In addition, it is worth to note that some phytochemicals of plant materials may reduce the adhesion of bacteria (Asaia spp.) found in food products/beverages, as it was shown in the case of juices of cornelian cherry (Antolak et al., 2017). Thanks to this, the antiadhesive activity of fruit-derived constituents prevents the formation of biofilm on the solid surface, which can be potential source of product contamination. Thus, they are likely to protect both food and plant preparations used in the phytotherapy against loss of organoleptic quality manifested by turbidity and flock formation.
Effects on Memory and Brain Functions
The phytotherapy of diseases or complaints related to the central nervous systems, including memory retention, has a long tradition in Asian and also in European medicine. The reported anti-amnestic and neuroprotective activities of both species seem to be based on similar constituents such as flavonoids and terpenoids which express antioxidant or more precisely radical scavenging effects (Dinda et al., 2016; Cooper and Ma, 2017; Huang et al., 2018). Animal experiments as well as biochemical investigations showed that the dried fruit powder and fruit extracts were able to increase level of physiological antioxidants such as glutathione and antioxidative enzymes, including superoxide dismutase or catalase, as well as to prevent cells and tissues against free radicals in this manner (Lee et al., 2006; Kim et al., 2011; Celep et al., 2012; Lee N. H. et al., 2012; Sozanski et al., 2014). In particular, morroniside (8) has been proved to protect human neuroblastoma cells against H2O2-induced stress, inhibit the apoptosis and accumulation of intracellular Ca2+ as well as restore the mitochondrial potential in SH-SY5Y cell line (Wang W. et al., 2008; Wang et al., 2009). The results of in vitro studies in cellular models were confirmed in cerebral ischemia model in rats. Morroniside (8) at the concentration range from 30 to 270 mg/kg body weight significantly reduced infarct volume, alleviated neurological impairment caused by ischemia/reperfusion-induced brain injury as well as enhanced progenitor cell proliferation (Wang et al., 2010; Sun et al., 2014a,b). Some special reports indicate the improvement of neurological functions in rats after feeding with iridoid glycosides from C. officinalis (Yao et al., 2009). Additionally, in the experimental autoimmune encephalomyelitis in animal models the administration of cornel iridoid glycoside significantly attenuated the symptoms of multiple sclerosis via an increased expression of brain-derived neurotrophic factor (BDNF) and nerve growth factor (NGF) as well as inhibition of JAK/STAT1 and JAK/STAT3 phosphorylation and reduction of proinflammatory mediators, such as TNF-α, IL-1β, IFN-γ, IL-17 (Yin et al., 2014; Qu et al., 2016). Iridoids of C. officinalis also protect glutamate-injured hippocampal cells (Jeong et al., 2012). A treatment of rats with a polyphenolic-rich dried fruits of C. mas resulted in increased activity of catalase in brain tissue, while in plasma it caused the opposite effect. On the other hand, the increased activity of paraoxonase-1 (PON1) was observed both in rats brain and plasma what potentiate a protective effect of PON1 with regard to the LDL and prevention of oxidative stress. Polyphenols and ascorbic acid of C. mas fruits were linked with this effect. Therefore, both neuroprotective and cardioprotective effects were assumed (Francik et al., 2014).
On the other hand, cornuside (6) as a representative for secoiridoid glycosides turned out to be an inhibitor of β-site amyloid precursor protein cleaving enzyme 1 (BACE1; IC50 = 55.8 μg/ml) but was a weak inhibitor of acetylocholinesterase (IC50 > 100 μg/ml) and butyrylocholinesterase (IC50 > 500 μg/ml). Other polyphenolic compounds isolated from fruits of C. officinalis such as tellimagrandin I (126), tellimagrandin II (127) and isoterchebin (139) were significant inhibitors of all enzymes engaged in the progression of neurodegeneration in Alzheimer's disease (Bhakta et al., 2017). Morroniside (8) was found to inhibit tau hyperphosphorylation, another Alzheimer's disease marker, via protein phosphatase 2A activation in human neuroblastoma SK-N-SH cells (Yang et al., 2016). The downregulation of mRNA expression of STIM1 and the inhibition of extracellular Ca2+ influx in PC12 cell line by an aqueous extract from fruits of C. officinalis were proposed as the potential inducing mechanisms of neurite generation and differentiation (Wang et al., 2015).
Cardioprotective Activity
In close relation to neuroprotection linked with antioxidant properties, antihyperlipidemic and cardioprotective activity of both herbal preparations were demonstrated by in vitro as well as in vivo experiments. As mentioned before these protective effects seem to be based on the same chemical constituents. In animal studies for both plant materials evidence was shown for antihypertensive effects, decreasing blood pressure and reduction of circulating cholesterol concentration (Park et al., 2009; Rafieian-Kopaei et al., 2011; Asgary et al., 2014; Sozanski et al., 2014, 2016; Hosseinpour et al., 2017). The ethanolic extract of C. officinalis fruits has significantly inhibited adipogenesis (87.1%) in 3T3-L1 preadipocytes (Roh and Jung, 2012; Kang et al., 2014). In addition, it is believed that the metabolic diseases potentially correlate with endocrine hormones due to considerable role of cortisol in cholesterol metabolism (Fraser et al., 1999). One report demonstrated reduction of cortisol concentration in plasma of hamsters after supplementation of C. mas fruits (Lofti et al., 2014). In relation to lipid metabolism, the ability of morroniside (8) and 7-O-galloyl-D-sedoheptulose (120) to decrease the expression of proteins associated with lipid homeostasis SREBP-1 and SREBP-2 (sterol regulatory element binding proteins) in kidneys of mice (db/db) and rats has been shown (Yokozawa et al., 2009; Park et al., 2010). The direct cardioprotective role of cornuside present in both species has been established. The decrease of infarct volume after myocardial ischemia/reperfusion injury in rats treated with cornuside (6) has been assigned to the reduction of polymorphonuclear leukocytes infiltration, myeloperoxidase (MPO) activity and malondialdehyde (MDA) production in myocardial tissue as well as lower serum-levels of pro-inflammatory mediators (Jiang et al., 2011). Furthermore, this compound dilated vascular smooth muscles through endothelium-dependent NO/cGMP signaling pathway (Kang et al., 2007). Unfortunately, no convincing human studies addressing these biological activities have been published so far. Although there is one published clinical study of a fruit extract from C. mas demonstrating its antidiabetic effect associated with the decreasing risk factors of cardiovascular diseases (Soltani et al., 2015). These results revealed a trend in another clinical trial toward amelioration of lipid profile and vascular inflammation following addition of C. mas extracts to the daily diet of dyslipidemic children and adolescents but these findings need to be verified by larger scale trials (Asgary et al., 2013). However, human studies with extracts of C. officinalis fruits have not been published so far. Discussing these effects with respect to the ethnopharmacological reports regarding the traditional use of both species in the past some evidence for beneficial effects on heart and circulation system may exist. This concerns especially the traditional use of C. mas to treat disorders or complaints related to aging processes like diabetes, ischemia or cardiovascular diseases (Dinda et al., 2016; Hosseinpour-Jaghdani et al., 2017). Due to the use of C. officinalis in TCM as a component of complex drug mixtures (Chen et al., 2008; Liu et al., 2013; Dai et al., 2016), a clear assignment to effects on heart and circulation system is rather difficult. However, in preparations of TCM used for treatment of diseases often related to aging processes the fruits of C. officinalis are abundant constituents (Chinese Pharmacopoeia, 2015).
Antitumor Activity
Despite the reports of antitumor activity of C. mas and C. officinalis fruits, the data do not provide any evidence that the fruits of both species show any real antitumor activity. All results from the literature refer to cytotoxic activities in different tumor cell lines (A549, MCF-7, SKOV3, PC-3, HepG2, U937) but not to animal experiments or human studies (Chang et al., 2004; Yousefi et al., 2015). Even though a cytotoxic effect on tumor cells is observed, the antitumor activity is not fully addressed and cannot be interpreted. This kind of over-interpretation of in vitro studies occurs very often but has no pharmacological relevance. All polyphenolic compounds, including the most abundant constituents of dogwood fruit extracts, bind to cells in culture leading to the induction of the biological effect. Consequently, the cytotoxicity or inhibition of proliferation associated with changes in signaling pathways occur. Only a clear selectivity in cytotoxicity between tumor and non-tumor cells corresponding with appropriate animal in vivo experiments opens up the possibility for the statement of antitumor activity. The reported antioxidant activity for both species (Dinda et al., 2016; Hosseinpour-Jaghdani et al., 2017; Huang et al., 2018) might be a hint for protective effects against tumor-inducing free radicals but for both species only in vitro models have been used to demonstrate such effects (Chang et al., 2004; Chu et al., 2009; Turker et al., 2012; Yousefi et al., 2015). In particular, the flavonoids are regarded as safe and easily obtainable. For this reason, they are considered ideal candidates for cancer chemoprevention or associated agents in clinical treatment (Sak, 2014).
Unique Biological and Pharmacological Activities of C. mas and C. officinalis
General
The unique anticoagulant and ophthalmic effects of dried fruits and extracts or anthocyanin-iridoid fractions from fruits of C. mas were assessed in the available reports. On the other hand, the antiosteoporotic, immunomodulatory and insecticidal properties of C. officinalis fruits, their extracts or constituents, such as morroniside (8), polysaccharides or 5-hydroxymethylfurfural (163), and dimethyl malate (173) were only found. Moreover, there is no available report on the antihyperlipidemic activity of C. officinalis. The circumstances of needs in the traditional eastern or western medicine requiring scientific confirmation are a pivotal factor determining scientific research. For this reason, these differences between C. mas and C. officinalis might arise from the lack of dedicated studies or even from different cultural needs resulting from the geographical distribution of both species.
Cornus mas L.
Anticoagulant Effect
Historical reports about an anticoagulant effect of fruits from C. mas do not exist. According to the interpretation of diseases of the circulation system, their etiology was rather assumed in disturbances of the digestion tract based on the theory of the four humors. The knowledge of functions and pathological significance of platelets or the blood coagulation system is the result of research in the twentieth century. For this reason, single reports about pharmacological effects of traditionally used herbal remedies on blood coagulation or platelet function are rather remarkable. Thus, it is worth to note that the dried fruit powder and hydromethanolic extracts of the fruits of C. mas showed an anticoagulant effect in animal experiments. In hypercholesterolemic rabbits the cornelian cherry powder reduced the plasma fibrinogen level after feeding over 60 days. The results indicated that consumption of this dried plant material is likely to be beneficial in atherosclerotic patients due to its reducing effects on fibrinogen (Asgary et al., 2010; Rafieian-Kopaei et al., 2011). A significant decrease in the platelet distribution width (PDW) was found in male rats treated with 50, 200, and 400 mg/kg body weight of a hydromethanolic extract over 3 weeks. The changes in the platelet-related parameters suggested platelet activity inhibition without bone marrow suppression in the treated groups in comparison with the control groups. Regarding to the secondary products present in the drug, it is evidence that antioxidants reduce the platelet activity through stimulation of prostacyclin synthesis and scavenging the synthesis-inhibiting peroxides. Recent studies have shown that polyphenols such as quercetin (66) and catechin (88), stop NADPH oxidase enzyme (present in platelets). Therefore, inhibiting the production of superoxide anion (·) and increasing the biological power of NO consequently regulate glycoprotein pine receptors (GPIIb-IIIa) on platelet membrane surface, that in turn inhibits platelets' activation and their adherence during inflammation and thrombosis processes. Decreasing effect of the fruit extract on platelet activity might classify it as an alternative for antiplatelet therapy in cardiovascular diseases (Abdollahi et al., 2014). Unfortunately, clinical studies investigating this aspect of activity have not been published to date. Despite some similarities in the phytochemical composition regarding the phenolic compounds between fruits from C. mas and C. officinalis anticoagulant effects of C. officinalis preparations are not reported in the traditional Chinese medicine. According to the theoretical fundamentals of Chinese medicine the blood coagulation system did not play any role in TCM. Nevertheless, a biochemical study also proposed an antiplatelet activity of this drug based on malic acid (171), citric acid (176), and succinic acid (177) identified in the fruits of C. officinalis (Zhang Q. -A. et al., 2013).
Ophthalmic Activity
It is believed that anthocyanins as well as other antioxidants may improve the visual functions and the visual field of patients with normal-tension glaucoma via neuroprotective effect, inhibition of lipid peroxidation and improvement of vascular blood flow into the optic nerve. The progressive optic nerve damage accompanied by morphological changes, loss of the field of vision and even increased intraocular pressure are the symptoms of so called glaucoma. An interesting study on the effect of an anthocyanin-iridoid fraction derived from fruits of C. mas on intraocular pressure was conducted in rabbits. Intraocular pressure was decreased by 19 and 25% after 2–3 h intraconjunctival administration of the fraction or loganic acid (3) solution (0.7%), respectively (Szumny et al., 2015). This activity of cornelian cherries polyphenols is likely to provide beneficial effects on vascular flow in diabetic and hypertensive retinopathy.
Cornus officinalis Sieb. et Zucc.
Antiosteoporotic Activity
Osteoporosis as a chronic disease is closely related with aging processes in the elderly. Chinese herbal medicines have been used to treat this disease for a long time and among the preparations listed in Chinese Pharmacopoeia fruits of C. officinalis are also included (Huang et al., 2018). Based on the modern findings in pathogenesis and treatment of osteoporosis a variety of results from biochemical and cell biological experiments are published which support the idea that natural products present in the plant preparation play a beneficial role.
Extract of C. officinalis significantly inhibits RANKL-mediated osteoclast differentiation in a dose-dependent manner and RANKL-induced phosphorylation of p38 and c-JUN N-terminal kinase. In the same way, the protein expression of c-Fos and NFATc1 is also suppressed as well as the RANKL-induced degradation of IκB kinase (Kim J. Y. et al., 2012). Morroniside (8), one the main constituent of extracts from C. officinalis fruits, has been studied on osteoarthritis in human chondrocytes. This compound increased the levels of type II collagen, and aggrecan as well as improved the level of proteoglycans in cartilage matrix. In this manner, it supported the survival of chondrocytes with articular cartilage thickness (Cheng et al., 2015). Summarizing, the extracts of C. officinalis or individual compounds inhibit osteoclast differentiation not only in in vitro cellular models but also in osteoporosis animal models (Xia et al., 2014). Detailed animal studies or clinical trials are not published.
Immunomodulatory Activity
The pivotal characteristics of immunomodulators in the maintenance of the human well-being and health cannot be overlooked. The sources of these medicinal remedies are part of long traditions in different regions of the world, such as traditional Chinese medicine, which have been developed through empirical experience. Traditional medicine employs a holistic approach to the prevention of disease and traditional herbal medicines are a source of components with therapeutic value (Tiwari et al., 2018). Many studies have been conducted also on preparations of C. officinalis and have revealed constituents that influence the innate immune system through the stimulation of macrophages and lymphocytes, and modulation of the cytokine profile, which leads to a reduction of the incidence of infections or allergic diseases.
In addition, allergic asthma is a chronic inflammatory disease of the airways, demonstrated by symptoms such as wheezing, coughing, breathlessness, and airways inflammation. Investigations in a mouse model of allergic asthma suggest that the therapeutic effects of Corni fructus are mediated by reduced production of Th2 cytokines (IL-5), eotaxin, and specific IgE as well as a reduced eosinophil infiltration (Kim S. H. et al., 2012). These findings are supported by animal experiments with polysaccharides from crude and processed C. officinalis fruits which have an enhanced effect on non-specific immunity, specific humoral immunity and specific cellular immunity in immuno-depressed mice (Du et al., 2008).
Along with the anti-inflammatory effects mentioned before, these results provide some evidence that the fruits of C. officinalis show immune-protective activity. Nevertheless, the evidence by human studies is still missing.
Insecticidal Activity
An interesting biological activity is the insecticidal effect of 5-hydroxymethylfurfural (163) and dimethyl malate (173) determined in a bioassay-guided fractionation of methanolic extract from the fruits of C. officinalis against larvae of D. melanogaster. Acute toxicity against adults of D. melanogaster was determined showing LD50 value of 34.0 and 21.5 μg/adult for 5-hydroxymethylfurfural (168) and dimethyl malate (173), respectively (Miyazawa et al., 2003). Such investigations were reported rather infrequently. Nevertheless, they are important with respect to protect health and food quality in the past and presently. The use of such experiences could help to develop ecological strategies for modern food storage.
Conclusion
The utilization of plant resources for nutrition and medicinal use in the past belongs to the process of civilization. The investigation of such remarkable fruits from both species is therefore not surprising. According to the different ideas regarding the origin of diseases and methods for therapeutic treatment, the different use of fruits from C. mas und C. officinalis in the West and the East could be explained. Despite all these differences it is rather surprising that such a level of consistency is evident. The chemical composition of C. mas and C. officinalis seems to be well-established. However, the availability of missing information on some groups of compounds, such as fatty acids, carotenoids and ascorbic acid in the fruits of C. officinalis, is still needed. Nevertheless, fruits of both species are rich in iridoids, anthocyanins, and flavonoids. The main difference in phytochemicals concern tannins, which were found only in C. officinalis and may potentially determine the biological activity in a significant manner. However, the role of tannins, except for 7-O-galloyl-D-sedoheptulose, was not widely considered in the biological activity of C. officinalis fruits. Taking into consideration the close phylogenetic relationship of both species the question about tannins in fruits of C. mas is open. On the other hand, tannins do not rather occur in the iridoid-rich plant materials but this hypothesis requires detailed elucidation in this case. Nevertheless, the transfer of medicinal experiences from the West to the East or vice versa cannot be excluded but is not documented in the literature for this two species. The explanation for this consistency seems to be rather the result of testing and empirical observation. Analyzing the ethnopharmacological data available under modern scientific aspects and correlating the phytochemical composition of both species, the results show that nearly the same ingredients are also attributed for the same activity or presumed therapeutic use. However, some data concerning the phytochemical composition as well as pharmacological activity of, particularly C. officinalis, are still missed or limited due to their availability in Chinese. Thus, only dedicated comparative investigations of C. mas and C. officinalis fruit preparations could really provide evidence of similarities or differences in the field of their phytochemical composition and biological or pharmacological properties. Last but not least is the assignation of extracts constituents potentially responsible for the specific activity. It means that the accordance between ethnopharmacological use of both plant materials should be based on the well-established chemical composition as well as simultaneous in vitro and in vivo studies, which may facilitate the identification of active constituents. Only these studies could validate careful observations of the users or physicians in the past.
Author Contributions
MC carried out a literature review in the field of phytochemistry and biological activity. MM designed the draft of the manuscript and carried out a literature review in the field of pharmacological activity. Both authors wrote the manuscript, revised and approved the final version of the manuscript.
Conflict of Interest Statement
The authors declare that the research was conducted in the absence of any commercial or financial relationships that could be construed as a potential conflict of interest.
References
Abbasi, M., Abdollahi, B., Milani, P., Mohajeri, D., and Nourdadgar, A. (2014). Effects of hydro-methanolic extract of Cornus mas on histopathological and biochemical parameters of rats' liver and kidney. Bothalia J. 44, 250–259.
Abdollahi, B., Mesgari Abbasi, M., Zakeri Milani, P., Nourdadgar, A. S., Banan Khojasteh, S. M., and Nejati, V. (2014). Hydro-methanolic extract of Cornus mas L. and blood glucose, lipid profile and hematological parameters of male rats. Iran. Red Crescent Med. J. 16:e17784. doi: 10.5812/ircmj.17784
Alavian, S. M., Banihabib, N., Es Haghi, M., and Panahi, F. (2014). Protective effect of Cornus mas fruits extract on serum biomarkers in CCl4-induced hepatotoxicity in male rats. Hepat. Mon. 14:e10330. doi: 10.5812/hepatmon.10330
Antolak, H., Czyzowska, A., Sakac, M., Mišan, A., Duragić, O., and Kregiel, D. (2017). Phenolic compounds contained in little-known wild fruits as antiadhesive agents against the beverage-spoiling bacteria Asaia spp. Molecules 22:E1256. doi: 10.3390/molecules22081256
APG IV (2016). An update of the Angiosperm Phylogeny Group classification for the orders and families of flowering plants: APG IV. Bot. J. Linnean Soc. 181, 1–20. doi: 10.1111/boj.12385
Asgary, S., Kelishadi, R., Rafieian-Kopaei, M., Najafi, S., Najafi, M., and Sahebkar, A. (2013). Investigation of the lipid-modifying and antiinflammatory effects of Cornus mas L. supplementation on dyslipidemic children and adolescents. Pediatr. Cardiol. 34, 1729–1735. doi: 10.1007/s00246-013-0693-5
Asgary, S., Rafieian-Kopaei, M., Adelnia, A., Kazemi, S., and Shamsi, F. (2010). Comparing the effects of lovastatin and Cornus mas fruit on fibrinogen level in hypercholesterolemic rabbits. ARYA Atheroscler. 6, 1–5.
Asgary, S., Rafieian-Kopaei, M., Shamsi, F., Najafi, S., and Sahebkar, A. (2014). Biochemical and histopathological study of the anti-hyperglycemic and anti-hyperlipidemic effects of cornelian cherry (Cornus mas L.) in alloxan-induced diabetic rats. J. Complement. Integr. Med. 11, 63–69. doi: 10.1515/jcim-2013-0022
Bajić-Ljubičić, J., Popović, Z., Matić, R., and Bojović, S. (2018). Selected phenolic compounds in fruits of wild growing Cornus mas L. Ind. J. Tradit. Know. 17, 91–96.
Bate-Smith, E., Ferguson, I., Hutson, K., Jensen, S., Nielsen, B., and Swain, T. (1975). Phytochemical interrelationships in the Cornaceae. Biochem. Sys. Ecol. 3, 79–89. doi: 10.1016/0305-1978(75)90046-0
Begic-Akagic, A., Drkenda, P., Vranac, A., Orazem, P., and Hudina, M. (2013). Influence of growing region and storage time on phenolic profile of cornelian cherry jam and fruit. Eur. J. Hort. Sci. 78, 30–39.
Bhakta, H. K., Park, C. H., Yokozawa, T., Tanaka, T., Jung, H. A., and Choi, J. S. (2017). Potential anti-cholinesterase and beta-site amyloid precursor protein cleaving enzyme 1 inhibitory activities of cornuside and gallotannins from Cornus officinalis fruits. Arch. Pharm. Res. 40, 836–853. doi: 10.1007/s12272-017-0924-z
Bilejić, S., Golšin, B., Cerović, S., and Bogdanovć, B. (2015). Pomological characteristics of cornelian cherry (Cornus mas L.) selections in Serbia and the possibility of growing in intensive organic orchards. Acta Univ. Agric. Silvic. Mendel. Brun. 63, 1101–1104. doi: 10.11118/actaun201563041101
Bilejić, S. M., Golšin, B. R., Ninić Todorović, J., Cerović, S. B., and Popović, B. M. (2011). Physicochemical fruit characteristics of cornelian cherry (Cornus mas L.) genotypes from Serbia. Hort. Sci. 46, 849–853.
Bozdogan, A. (2017). Viscosity and physicochemical properties of cornlian cherry (Cornus mas L.) concentrate. Food Measure. 11, 1326–1332. doi: 10.1007/s11694-017-9510-9
Brindza, P. (2007). Slovakian cornelian cherry (Cornus mas L.) potential for cultivation. Acta Hort. 760, 433–438. doi: 10.17660/ActaHortic.2007.760.59
Brindza, P. (2009). Biological and commercial characteristics of cornelian cherry (Cornus mas L.) population in the Gemer region og Slovakia. Acta Hort. 818, 85–94. doi: 10.17660/ActaHortic.2009.818.11
Cai, H., Cao, G., and Cai, B. (2013). Rapid simultaneous identification and determination of the multiple compounds in crude Fructus Corni and its processed products by HPLC-MS/MS with multiple reaction monitoring mode. Pharm. Biol. 51, 273–278. doi: 10.3109/13880209.2012.720689
Cao, G., Zhang, C., Zhang, Y., Cong, X., Cai, H., and Cai, B. (2012). Screening and identification of potential active components in crude Fructus Corni using solid-phase extraction and LC-LTQ-linear ion trap mass spectrometry. Pharm. Biol. 50, 278–283. doi: 10.3109/13880209.2011.599036
Cao, G., Zhang, Y., Feng, J., Cai, H., Zhang, C., Ding, M., et al. (2011). A rapid and sensitive assay for determining the main components in processed Fructus Corni by UPLC-Q-TOF-MS. Chromatographia 73, 135–141. doi: 10.1007/s10337-010-1825-1
Capanoglu, E., Boyacioglu, D., De Vos, R. C. H., Hall, R., and Beekwilder, J. (2011). Procyanidins in fruit from Sour cherry (Prunus cerasus) differ strongly in chainlength from those in Laurel cherry (Prunus lauracerasus) and Cornelian cherry (Cornus mas). J. Berry Res. 1, 137–146. doi: 10.3233/BR-2011-015
Celep, E., Aydin, A., and Yesilada, E. (2012). A comparative study on the in vitro antioxidant potentials of three edible fruits: cornelian cherry, Japanese persimmon and cherry laurel. Food Chem. Toxicol. 50, 3329–3335. doi: 10.1016/j.fct.2012.06.010
Chang, J., Chiang, L., Hsu, F., and Lin, C. (2004). Chemoprevention against hepatocellular carcinoma of Cornus officinalis in vitro. Am. J. Chin. Med. 32, 717–725. doi: 10.1142/S0192415X04002296
Chen, C. C., Hsu, C. Y., Chen, C. Y., and Liu, H. K. (2008). Fructus Corni suppresses hepatic gluconeogenesis related gene transcription, enhances glucose responsiveness of pancreatic beta-cells, and prevents toxin induced beta-cell death. J. Ethnopharmacol. 117, 483–490. doi: 10.1016/j.jep.2008.02.032
Cheng, L., Zeng, G., Liu, Z., Zhang, B., Cui, X., Zhao, H., et al. (2015). Protein kinase B and extracellular signal-regulated kinase contribute to the chondroprotective effect of morroniside on osteoarthritis chondrocytes. J. Cell. Mol. Med. 19, 1877–1886. doi: 10.1111/jcmm.12559
Chinese Pharmacopoeia (2015). Pharmacopoeia of the People's Republic of China. Beijing: People's Medical Publishing House.
Choi, Y. H., Jin, G. Y., Li, G. Z., and Yan, G. H. (2011). Cornuside suppresses lipopolysaccharide-induced inflammatory mediators by inhibiting nuclear factor-kappa B activation in RAW 264.7 macrophages. Biol. Pharm. Bull. 34, 959–966. doi: 10.1248/bpb.34.959
Chu, Q., Satoh, K., Kanamoto, T., Terakubo, S., Nakashima, H., Wang, Q., et al. (2009). Antitumor potential of three herbal extracts against human oral squamous cell lines. Anticancer Res. 29, 3211–3219.
Cooper, E. L., and Ma, M. J. (2017). Alzheimer disease: clues from traditional and complementary medicine. J. Tradit. Complement. Med. 7, 380–385. doi: 10.1016/j.jtcme.2016.12.003
Çopur, U., Soylu, A., Gürbüz, N., Degirmencioglu, N., and Ertürk, Ü. (2003). Suitability of Cornus mas (cornelian cherry) genotypes and cultivars for fruit juice. Biotechnol. Biotechnol. Eq. 17, 176–182. doi: 10.1080/13102818.2003.10819217
Cosmulescu, S., Trandafir, I., and Nour, V. (2017). Phenolic acids and flavonoids profiles of extracts from edible wild fruits and their antioxidant properties. Int. J. Food Prop. 20, 3124–3134. doi: 10.1080/10942912.2016.1274906
Czyzowska, A., Kucharska, A. Z., Nowak, A., Sokół-Łetowska, A., Motyl, I., and Piórecki, N. (2017). Suitability of the probiotic lactic acid bacteria strains as the starter cultures in unripe cornelian cherry (Cornus mas L.) fermentation. J. Food Sci. Technol. 54, 2936–2946. doi: 10.1007/s13197-017-2732-3
Dai, B., Wu, Q., Zeng, C., Zhang, J., Cao, L., Xiao, Z., et al. (2016). The effect of Liuwei Dihuang decoction on PI3K/Akt signaling pathway in liver of type 2 diabetes mellitus (T2DM) rats with insulin resistance. J. Ethnopharmacol. 192, 382–389. doi: 10.1016/j.jep.2016.07.024
Demir, F., and Kalyoncu, I. H. (2003). Some nutritional, pomological and physical properties of cornelian cherry (Cornus mas L.). J. Food Eng. 60, 335–341. doi: 10.1016/S0260-8774(03)00056-6
Deng, S., West, B. J., and Jensen, C. J. (2013). UPLC-TOF-MS characterization and identification of bioactive iridoids in Cornus mas fruit. J. Anal. Methods Chem. 2013:710972. doi: 10.1155/2013/710972
Desai, S. J., Prickril, B., and Rasooly, A. (2018). Mechanisms of phytonutrient modulation of cyclooxygenase-2 (COX-2) and inflammation related to cancer. Nutr. Cancer 70, 350–375. doi: 10.1080/01635581.2018.1446091
Dinda, B., Kyriakopoulos, A. M., Dinda, S., Zoumpourlis, V., Thomaidis, N. S., Velegraki, A., et al. (2016). Cornus mas L. (cornelian cherry), an important European and Asian traditional food and medicine: ethnomedicine, phytochemistry and pharmacology for its commercial utilization in drug industry. J. Ethnopharmacol. 193, 670–690. doi: 10.1016/j.jep.2016.09.042
Dokoupil, L., and Rezníček, V. (2012). Production and use of the cornelian cherry–Cornus mas L. Acta Univ. Agric. Silvic. Mendel. Brun. 60, 49–58. doi: 10.11118/actaun201260080049
Dragendorff, G. (1898). Die Heilpflanzen der verschiedenen Völker und Zeiten. Stuttgart: Ferdinand Enke Verlag.
Dragović-Uzelac, V., Levaj, B., Bursać, D., Pedisić, S., Radojčić, I., and Biško, A. (2007). Total phenolics and antioxidant capacity assays of selected fruits. Agric. Conspec. Sci. 72, 279–284.
Drkenda, P., Spahic, A., Begic-Akagic, A., Gasi, F., Vranac, A., Hudina, M., et al. (2014). Pomological characteristics of some autochthnous genotypes of cornelian cherry (Cornus mas L.) in Bosnia and Herzegovina. Erwerbs-Obstbau 56, 59–66. doi: 10.1007/s10341-014-0203-9
Du, C.-T., and Francis, F. J. (1973). Anthocyanins from Cornus mas. Phytochemistry 12, 2487–2489. doi: 10.1016/0031-9422(73)80460-1
Du, W., Cai, H., Wang, M., Ding, X., Yang, H., and Cai, B. (2008). Simultaneous determination of six active components in crude and processed Fructus Corni by high performance liquid chromatography. J. Pharm. Biomed. Anal. 48, 194–197. doi: 10.1016/j.jpba.2008.04.021
Ersoy, N., Bagci, Y., and Gok, V. (2011). Antioxidant properties of 12 cornelian cherry fruit types (Cornus mas L.) slected from Turkey. Sci. Res. Essays 6, 98–102. doi: 10.5897/SRE10.740
Es Haghi, M., Dehghan, G., Banihabib, N., Zare, S., Mikaili, P., and Panahi, F. (2014). Protective effects of Cornus mas fruit extract on carbon tetrachloride induced nephrotoxicity in rats. Ind. J. Nephrol. 24, 291–296. doi: 10.4103/0971-4065.133000
Eyde, R. (1988). Comprehending Cornus: puzzles and progress in the systematics of the dogwoods. Bot. Rev. (Lancaster) 54, 233–351. doi: 10.1007/BF02868985
Fan, C., and Xiang, Q.-Y. (2001). Phylogenetic relationships within Cornus (Cornaceae) based on 26S rDNA sequences. Am. J. Bot. 88, 1131–1138. doi: 10.2307/2657096
Forman, V., Haladová, M., Grančai, D., and Ficková, M. (2015). Antiproliferative activities of water infusions from leaves of five Cornus L. species. Molecules 20, 22546–22552. doi: 10.3390/molecules201219786
Fournier, P. (ed.). (1948). Le Livre des Plantes Médicinales et Vénéneuses de France. Paris: Paul Lechevalier.
Francik, R., Krośniak, J., Krosniak, M., Berköz, M., Sanocka, I., and Francik, S. (2014). The neuroprotective effect of Cornus mas on brain tissue of Wistar rats. Sci. World J. 2014:847368. doi: 10.1155/2014/847368
Fraser, R., Ingram, M. C., Anderson, N. H., Morrison, C., Davies, E., and Connell, J. M. (1999). Cortisol effects on body mass, blood pressure, and cholesterol in the general population. Hypertension 33, 1364–1368. doi: 10.1161/01.HYP.33.6.1364
Gao, D., Li, N., Li, Q., Li, J., Han, Z., Fan, Y., et al. (2008). Study of the extraction, purification and antidiabetic potential of ursolic acid from Cornus officinalis Sieb. et Zucc. Therapy 5, 697–705. doi: 10.2217/14750708.5.5.697
Gao, D., Li, Q., Gao, Z., and Wang, L. (2012). Antidiabetic effects of Corni Fructus extract in streptozotocin-induced diabetic rats. Yonsei Med. J. 53, 691–700. doi: 10.3349/ymj.2012.53.4.691
Gastoł, M., Krośniak, M., Derwisz, M., and Dobrowolska-Iwanek, J. (2013). Cornelian cherry (Cornus mas L.) juice as a potential source of biological compounds. J. Med. Food 16, 728–732. doi: 10.1089/jmf.2012.0248
Gibson, G. (2008). Prebiotics as gut microflora management tools. J. Clin. Gastroenterol. 42, S75–79. doi: 10.1097/MCG.0b013e31815ed097
Grune, T., Lietz, G., Palou, A., Ross, A., Stahl, W., Tang, G., et al. (2010). β-Carotene is an important vitamin A source for humans. J. Nutr. 140, 2268S−2285S. doi: 10.3945/jn.109.119024
Güleryüz, M., Bolat, I., and Pirlak, L. (1998). Selection of table cornelian cherry (Cornus mas L.) types of Çoruh Valley. Turk. J. Agric. For. 22, 357–364.
Gunduz, K., Saracoglu, O., Özgen, M., and Serce, S. (2013). Antioxidant, physical and chemical characteristics of cornelian cherry fruits (Cornus mas L.) at different stages of ripeness. Acta Sci. Pol. Hortorum Cultus 12, 59–66.
Hamid, H., Yousef, H., Jafar, H., and Mohammad, A. (2011). Antioxidant capacity and phytochemical properties of cornelian cherry (Cornus mas L.) genotypes in Iran. Sci. Hortic. 129, 459–463. doi: 10.1016/j.scienta.2011.04.017
Han, Y., Jung, H. W., and Park, Y. K. (2014). Selective therapeutic effect of Cornus officinalis fruits on the damage of different organs in STZ-induced diabetic rats. Am. J. Chin. Med. 42, 1169–1182. doi: 10.1142/S0192415X14500736
Hassanpour, H., Hamidoghli, Y., and Samizadeh, H. (2012). Some fruit characeteristics of Iranian cornelian cherries (Cornus mas L.). Not. Bot. Horti. Agrobo 40, 247–252. doi: 10.15835/nbha4017385
Hatano, T., Ogawa, N., Kira, R., Yasuhara, T., and Okuda, T. (1989a). Tannins of Cornaceous plants. I. Cornusiins A, B and C, dimeric monomeric and trimeric hydrolyzable tannins from Cornus officinalis, and orientation of valoneoyl group in related tannins. Chem. Pharm. Bull. (Tokyo) 37, 2083–2090.
Hatano, T., Yasuhara, T., Abe, R., and Okuda, T. (1990). A galloylated monoterpene glucoside and a dimeric hydrolysable tannin from Cornus officinalis. Phytochemistry 29, 2975–2978. doi: 10.1016/0031-9422(90)87118-E
Hatano, T., Yasuhara, T., and Okuda, T. (1989b). Tannins of Cornaceous plants. II. Cornusiins D, E and F, new dimeric and trimeric hydrolyzable tannins from Cornus officinalis. Chem. Pharm. Bull. (Tokyo) 37, 2665–2669.
He, J., Ye, X. S., Wang, X. X., Yang, Y. N., Zhang, P. C., Ma, B. Z., et al. (2017). Four new iridoid glucosides containing the furan ring from the fruit of Cornus officinalis. Fitoterapia 120, 136–141. doi: 10.1016/j.fitote.2017.06.003
He, K., Song, S., Zou, Z., Feng, M., Wang, D., Wang, Y., et al. (2016). The hypoglycemic and synergistic effect of loganin, morroniside, and ursolic acid isolated from the fruits of Cornus officinalis. Phytother. Res. 30, 283–291. doi: 10.1002/ptr.5529
Horváth, G., Turcsi, E., Molnár, P., Szabó, L. G., and Deli, J. (2007). “Isolation and identification of carotenoids in the fruit of cornelian cherry (Cornus mas L.),” in 55th International Congress and Annual Meeting of the Society for Medicinal Plant Research. ed P. Medica (Graz, Austria: Thieme Publisher).
Hosseinpour, F., Shomali, T., and Rafieian-Kopaei, M. (2017). Hypocholesterolemic activity of cornelian cherry (Cornus mas L.) fruits. J. Complement. Integr. Med. 14:20170007. doi: 10.1515/jcim-2017-0007
Hosseinpour-Jaghdani, F., Shomali, T., Gholipour-Shahraki, A., Rahimi-Madiseh, M., and Rafieian-Kopaei, M. (2017). Cornus mas: a review on traditional uses and pharmacological properties. J. Complement. Integr. Med. 14:20160137. doi: 10.1515/jcim-2016-0137
Hosu, A., Cimpoiu, C., David, L., and Moldovan, B. (2016). Study of the antioxidant property variation of cornelian cherry fruits during storage using HPTLC and spectrophotometric assays. J. Anal. Methods Chem. 2016:2345375. doi: 10.1155/2016/2345375
Huang, J., Zhang, Y., Dong, L., Gao, Q., Yin, L., Quan, H., et al. (2018). Ethnopharmacology, phytochemistry, and pharmacology of Cornus officinalis Sieb. et Zucc. J. Ethnopharmacol. 213, 280–301. doi: 10.1016/j.jep.2017.11.010
Hwang, K. A., Hwang, Y. J., and Song, J. (2016). Antioxidant activities and oxidative stress inhibitory effects of ethanol extracts from Cornus officinalis on raw 264.7 cells. BMC Complement. Altern. Med. 16:196. doi: 10.1186/s12906-016-1172-3
Inouye, H., Shinichi, U., Inoue, K., and Takeda, Y. (1974). Studies on monoterpene glucosides and related natural products. XXIII. Biosynthesis of the secoiridoid glucosides, gentiopicroside, morroniside, oleuropein, and jasminin. Chem. Pharm. Bull. 22, 676–686. doi: 10.1248/cpb.22.676
Jaćimović, V., BoŽović, D., Ercisli, S., Ognjanov, V., and Bosančić, B. (2015). Some fruit characteristics of selected cornelian cherries (Cornus mas L.) from Montenegro. Erwerbs-Obstbau 57, 119–124. doi: 10.1007/s10341-015-0238-6
Jang, S. E., Jeong, J. J., Hyam, S. R., Han, M. J., and Kim, D. H. (2014). Ursolic acid isolated from the seed of Cornus officinalis ameliorates colitis in mice by inhibiting the binding of lipopolysaccharide to Toll-like receptor 4 on macrophages. J. Agric. Food Chem. 62, 9711–9721. doi: 10.1021/jf501487v
Jayaprakasam, B., Olson, L. K., Schutzki, R. E., Tai, M. H., and Nair, M. G. (2006). Amelioration of obesity and glucose intolerance in high-fat-fed C57BL/6 mice by anthocyanins and ursolic acid in Cornelian cherry (Cornus mas). J. Agric. Food Chem. 54, 243–248. doi: 10.1021/jf0520342
Jayaprakasam, B., Vareed, S. K., Olson, L. K., and Nair, M. G. (2005). Insulin secretion by bioactive anthocyanins and anthocyanidins present in fruits. J. Agric. Food Chem. 53, 28–31. doi: 10.1021/jf049018+
Jensen, S., Kjaer, A., and Nielsen, B. (1975). The genus Cornus: non-flabonoid glucosides as taxonomic markers. Biochem. Sys. Ecol. 3, 75–78. doi: 10.1016/0305-1978(75)90045-9
Jensen, S. R., Kjaer, A., and Nielsen, B. J. (1973). Loniceroside (secologanin) in Cornus officinalis and C. mas. Phytochemistry 12, 2064–2065. doi: 10.1016/S0031-9422(00)91544-9
Jeong, E. J., Kim, T. B., Yang, H., Kang, S. Y., Kim, S. Y., Sung, S. H., et al. (2012). Neuroprotective iridoid glycosides from Cornus officinalis fruits against glutamate-induced toxicity in HT22 hippocampal cells. Phytomedicine 19, 317–321. doi: 10.1016/j.phymed.2011.08.068
Ji, W., Wang, T., Liu, W., Liu, F., Guo, L., Geng, Y., et al. (2017). Water-compatible micron-sized monodisperse molecularly imprinted beads for selective extraction of five iridoid glycosides from Cornus officinalis fructus. J. Chromatogr. A 1504, 1–8. doi: 10.1016/j.chroma.2017.05.003
Jiang, W. L., Chen, X. G., Zhu, H. B., and Tian, J. W. (2009). Effect of cornuside on experimental sepsis. Planta Med. 75, 614–619. doi: 10.1055/s-0029-1185383
Jiang, W. L., Zhang, S. M., Tang, X. X., and Liu, H. Z. (2011). Protective roles of cornuside in acute myocardial ischemia and reperfusion injury in rats. Phytomedicine 18, 266–271. doi: 10.1016/j.phymed.2010.07.009
Jiang, W. L., Zhang, S. P., Hou, J., and Zhu, H. B. (2012). Effect of loganin on experimental diabetic nephropathy. Phytomedicine 19, 217–222. doi: 10.1016/j.phymed.2011.08.064
Jiang, Y., Chen, H., Wang, L., Zou, J., Zheng, X., and Liu, Z. (2016). Quality evaluation of polar and active components in crude and processed Fructus Corni by quantitative analysis of multicomponents with single marker. J. Anal. Methods Chem. 2016:6496840. doi: 10.1155/2016/6496840
Kalyoncu, I. H., Ersoy, N., and Yilmaz, M. (2009). Physico-chemical and nutritional properties of cornelian cherry fruits (Cornus mas L.) grown in Turkey. Asian J. Chem. 21, 6555–6561.
Kang, C., Kwon, Y., and So, J. (2014). Anti-adipogenic effects of Corni fructus in 3T3-L1 preadipocytes. Biotechnol. Bioprocess Eng. 19, 52–57. doi: 10.1007/s12257-013-0444-8
Kang, D. G., Choi, D. H., Lee, J. K., Lee, Y. J., Moon, M. K., Yang, S. N., et al. (2007). Endothelial NO/cGMP-dependent vascular relaxation of cornuside isolated from the fruit of Cornus officinalis. Planta Med. 73, 1436–1440. doi: 10.1055/s-2007-990243
Karadeniz, T., Deligöz, M. S., Çorumlu, M., Senyurt, M., and Bak, T. (2009). Selection of native cornelian cherries grown in Çorum (Turkey). Acta Hort. 825, 83–88. doi: 10.17660/ActaHortic.2009.825.9
Kim, D. K., and Kwak, J. H. (1998). A furan derivative from Cornus officinalis. Arch. Pharm. Res. 21, 787–789. doi: 10.1007/BF02976779
Kim, H. J., Kim, B. H., and Kim, Y. C. (2011). Antioxidative action of corni fructus aqueous extract on kidneys of diabetic mice. Toxicol. Res. 27, 37–41. doi: 10.5487/TR.2011.27.1.037
Kim, J. Y., Kim, Y. K., Choi, M. K., Oh, J., Kwak, H. B., and Kim, J. J. (2012). Effect of Cornus officinalis on receptor activator of nuclear factor-kappaB ligand (RANKL)-induced osteoclast differentiation. J. Bone Metab. 19, 121–127. doi: 10.11005/jbm.2012.19.2.121
Kim, S. H., Kim, B. K., and Lee, Y. C. (2012). Effects of Corni fructus on ovalbumin-induced airway inflammation and airway hyper-responsiveness in a mouse model of allergic asthma. J. Inflamm. (Lond). 9:9. doi: 10.1186/1476-9255-9-9
Kostecka, M., Szot, I., Czernecki, T., and Szot, P. (2017). Vitamin C content of new ecotypes of cornelian cherry (Cornus mas L.) determined by various analytical methods. Acta Sci. Hortorum Cultus 16, 53–61. doi: 10.24326/asphc.2017.4.6
Krivoruchko, E. V. (2014). Carboxylic acids from Cornus mas. Chem. Nat. Comp. 50:112. doi: 10.1007/s10600-014-0879-y
Krzyściak, P., Kośniak, M., Gastoł, M., Ochonska, D., and Krzyściak, W. (2011). Antimicrobial activity of Cornelian cherry (Cornus mas L.). Post Fitoter. 4, 227–231.
Kucharska, A. Z. (2012). Zwiazki aktywne Owoców Derenia (Cornus mas L.). Wrocław: Wrocław University of Environmental and Life Sciences.
Kucharska, A. Z., Szumny, A., Sokół-Łetowska, A., Piórecki, N., and Klymenko, S. V. (2015). Iridoids and anthocyanins in cornelian cherry (Cornus mas L.) cultivars. J. Food Comp. Anal. 40, 95–102. doi: 10.1016/j.jfca.2014.12.016
Kyriakopoulos, A. M., and Dinda, B. (2015). Cornus mas (Linnaeus) novel devised medicinal preparations: bactericidal effect against Staphylococcus aureus and Pseudomonas aeruginosa. Molecules 20, 11202–11218. doi: 10.3390/molecules200611202
Lee, A. Y., Kim, H. S., Jo, J. E., Kang, B. K., Moon, B. C., Chun, J. M., et al. (2012). Optimization of extraction condition for major iridoid components in fruit of corni (Cornus officinalis) by UPLC-PDA using response surface methodology. Food Sci. Biotechnol. 21, 1023–1029. doi: 10.1007/s10068-012-0133-y
Lee, J., Jang, D. S., Kim, N. H., Lee, Y. M., Kim, J., and Kim, J. S. (2011). Galloyl glucoses from the seeds of Cornus officinalis with inhibitory activity against protein glycation, aldose reductase, and cataractogenesis ex vivo. Biol. Pharm. Bull. 34, 443–446. doi: 10.1248/bpb.34.443
Lee, N. H., Seo, C. S., Lee, H. Y., Jung, D. Y., Lee, J. K., Lee, J. A., et al. (2012). Hepatoprotective and antioxidative activities of Cornus officinalis against acetaminophen-induced hepatotoxicity in mice. Evid. Based Complement. Alternat. Med. 2012:804924. doi: 10.1155/2012/804924
Lee, S. H., Tanaka, T., Nonaka, G.-I., and Nishioka, I. (1989). Sedoheptulose digallate from Cornus officinalis. Phytochemistry 28, 3469–3472. doi: 10.1016/0031-9422(89)80366-8
Lee, S. O., Kim, S. Y., Han, S. M., Kim, H. M., Ham, S. S., and Kang, I. J. (2006). Corni fructuc scavenges hydroxy radicals and decreases oxidative stress in endothelial cells. J. Med. Food 9, 594–598. doi: 10.1089/jmf.2006.9.594
Li, P., Yan, Y. B., Yu, L. Z., and Ma, R. Y. (2012). Characterization and anti-oxidant activities of polysascharides from Fructus Corni. Adv. Mat. Res. 560, 231–236. doi: 10.4028/www.scientific.net/AMR.560-561.231
Lin, M. H., Liu, H. K., Huang, W. J., Huang, C. C., Wu, T. H., and Hsu, F. L. (2011). Evaluation of the potential hypoglycemic and Beta-cell protective constituents isolated from Corni fructus to tackle insulin-dependent diabetes mellitus. J. Agric. Food. Chem. 59, 7743–7751. doi: 10.1021/jf201189r
Liu, H., Xu, H., Shen, C., and Wu, C. (2012). Effect of the best compatibility of components in Corni Fructus on WT1 expression in glomerular podocytes of type 2 diabetic rats with early nephropathy. Am. J. Chin. Med. 40, 537–549. doi: 10.1142/S0192415X12500413
Liu, J. P., Feng, L., Zhang, M. H., Ma, D. Y., Wang, S. Y., Gu, J., et al. (2013). Neuroprotective effect of Liuwei Dihuang decoction on cognition deficits of diabetic encephalopathy in streptozotocin-induced diabetic rat. J. Ethnopharmacol. 150, 371–381. doi: 10.1016/j.jep.2013.09.003
Liu, X., Han, Z. P., Wang, Y. L., Gao, Y., and Zhang, Z. Q. (2011). Analysis of the interactions of multicomponents in Cornus officinalis Sieb. et Zucc. with human serum albumin using on-line dialysis coupled with HPLC. J. Chromatogr. B Analyt. Technol. Biomed. Life Sci. 879, 599–604. doi: 10.1016/j.jchromb.2011.01.022
Liu, Z., Zhu, Z. Y., Zhang, H., Tan, G. G., Chen, X. F., and Chai, Y. F. (2011). Qualitative and quantitative analysis of Fructus Corni using ultrasound assisted microwave extraction and high performance liquid chromatography coupled with diode array UV detection and time-of-flight mass spectrometry. J. Pharm. Biomed. Anal. 55, 557–562. doi: 10.1016/j.jpba.2011.02.007
Lofti, A., Shahryar, A., and Rasoolian, H. (2014). Effects of cornelian cherry (Cornus mas L.) fruit on plasma lipids, cortisol, T3 and T4 levels in hamsters. J. Anim. Plant Sci. 24, 459–462.
Lv, G., Lv, X., Tao, Y., and Xu, H. (2016). Effect of morroniside on glomerular mesangial cells through AGE-RAGE pathway. Hum. Cell 29, 148–154. doi: 10.1007/s13577-015-0128-0
Ma, Q., Liu, X., Franks, R. G., and Xiang, Q.-Y. (2017). Alterations of CorTFL1 and CorAP1 expression correlate with major evolutionary shifts of inflorescence architecture in Cornus (Cornaceae) - a proposed model for variation of closed inflorescence forms. New Phytol. 216, 519–535. doi: 10.1111/nph.14197
Ma, W., Wang, K. J., Cheng, C. S., Yan, G. Q., Lu, W. L., Ge, J. F., et al. (2014). Bioactive compounds from Cornus officinalis fruits and their effects on diabetic nephropathy. J. Ethnopharmacol. 153, 840–845. doi: 10.1016/j.jep.2014.03.051
Mau, J., Chen, C., and Hsieh, P. (2001). Antimicrobial effect of extracts from Chinese chive, cinnamon, and corni fructus. J. Agric. Food Chem. 49, 183–188. doi: 10.1021/jf000263c
Milenković-Andjelković, A. S., Andjelković, M. Z., Radovanović, A. N., Radovanović, B. C., and Nikolić, V. (2015). Phenol composition, DPPH radical scavenging and antimicrobial activity of Cornelian cherry (Cornus mas) fruit and leaf extracts. Hem. Ind. 69, 331–337. doi: 10.2298/HEMIND140216046M
Mirbadalzadeh, R., and Shirdel, Z. (2012). Antihyperglycemic and antihyperlipidemic effects of Cornus mas extract in diabetic rats compared with glibenclamide. Elixir Hormo. Signal. 47, 8969–8972.
Miyazawa, M., Anzai, J., Fujioka, J., and Isikawa, Y. (2003). Insecticidal compounds against Drosophila melanogaster from Cornus officinalis Sieb. et Zucc. Nat. Prod. Res. 17, 337–339. doi: 10.1080/1057563031000072587
Moldovan, B., Filip, A., Clichici, S., Suharoschi, R., Bolfa, P., and David, L. (2016). Antioxidant activity of Cornelian cherry (Cornus mas L.) fruits extract and the in vivo evaluation of its anti-inflammatory effects. J. Funct. Foods 26, 77–87. doi: 10.1016/j.jff.2016.07.004
Narimani-Rad, M., Zendehdel, M., Abbasi, M., Abdollahi, B., and Lotfi, A. (2013). Cornelian cherry (Cornus mas L.) extract affects glycemic status in Wistar rats. Bull. Env. Pharmacol. Life Sci. 2, 48–50.
Nawirska-Olszanska, A., Kucharska, A., and Sokół-Łetowska, A. (2010). Dietary fibre fractions in cornelian cherry fruit (Cornus mas L.). Żywność: nauka-technologia-jakość 2, 95–103.
Nouska, C., Kazakos, S., Mantzourani, I., Alexopoulos, A., Bezirtzoglou, E., and Plessas, S. (2016). Fermentation of Cornus mas L. juice for functional low alcoholic beverage production. Curr. Res. Nutr. Food Sci. 4, 119–124. doi: 10.12944/CRNFSJ.4.Special-Issue-October.16
Okuda, T., Hatano, T., and Ogawa, N. (1984). Cornusiin A, a dimeric ellagitannin forming four tautomers, and accompanying new tannins in Cornus officinalis. Chem. Pharm. Bull. 32, 4662–4665. doi: 10.1248/cpb.32.4662
Okuda, T., Hatano, T., and Yasui, T. (1981). Revised structure of isoterchebin, isolated from Cornus officinalis. Heterocycles 16, 1321–1324. doi: 10.3987/R-1981-08-1321
Pantelidis, G. E., Vasilakakis, M., Manganaris, G. A., and Diamantidis, G. (2007). Antioxidant capacity, phenol, anthcyanin and ascorbic acid contents in raspberries, blackberries, red currants, gooseberries and Cornelian cherries. Food Chem. 102, 777–783. doi: 10.1016/j.foodchem.2006.06.021
Park, C., Tanaka, T., and Yokozawa, T. (2013). Anti-diabetic action of 7-O-galloyl-D-sedoheptulose, a polyphenol from Corni Fructus, through ameliorating inflammation and inflammation-related oxidative stress in the pancreas of type 2 diabetics. Biol. Pharm. Bull. 36, 723–732. doi: 10.1248/bpb.b12-00543
Park, C. H., Cho, E. J., and Yokozawa, T. (2009). Protection against hypercholesterolemia by Corni fructus extract and its related protective mechanism. J. Med. Food 12, 973–981. doi: 10.1089/jmf.2009.0037
Park, C. H., Noh, J. S., Tanaka, T., Roh, S. S., Lee, J. C., and Yokozawa, T. (2015). Polyphenol isolated from Corni Fructus, 7-O-galloyl-D-sedoheptulose, modulates advanced glycation endproduct-related pathway in type 2 diabetic db/db mice. Arch. Pharm. Res. 38, 1270–1280. doi: 10.1007/s12272-014-0457-7
Park, C. H., Noh, J. S., Tanaka, T., Uebaba, K., Cho, E. J., and Yokozawa, T. (2011). The effects of corni fructus extract and its fractions against alpha-glucosidase inhibitory activities in vitro and sucrose tolerance in normal rats. Am. J. Chin. Med. 39, 367–380. doi: 10.1142/S0192415X11008889
Park, C. H., Noh, J. S., Tanaka, T., and Yokozawa, T. (2010). Effects of morroniside isolated from Corni Fructus on renal lipids and inflammation in type 2 diabetic mice. J. Pharm. Pharmacol. 62, 374–380. doi: 10.1211/jpp.62.03.0013
Park, C. H., Noh, J. S., Tanaka, T., and Yokozawa, T. (2012a). 7-O-galloyl-D-sedoheptulose ameliorates renal damage triggered by reactive oxygen species-sensitive pathway of inflammation and apoptosis. J. Pharm. Pharmacol. 64, 1730–1740. doi: 10.1111/j.2042-7158.2012.01559.x
Park, C. H., Tanaka, T., Kim, H. Y., Park, J. C., and Yokozawa, T. (2012b). Protective effects of Corni Fructus against advanced glycation endproducts and radical scavenging. Evid. Based Complement. Alternat. Med. 2012:418953. doi: 10.1155/2012/418953
Park, J. Y., Han, A. R., Kil, Y. S., Kang, U., Kim, S. H., Nam, S. J., et al. (2016). A new secoiridoid glycoside from the fruits of Cornus officinalis (Cornaceae). Nat. Prod. Res. 30, 1504–1510. doi: 10.1080/14786419.2015.1115996
Pawlowska, A. M., Camangi, F., and Braca, A. (2010). Quali-quantitative analysis of flavonoids of Cornus mas L. (Cornaceae) fruits. Food Chem. 119, 1257–1261. doi: 10.1016/j.foodchem.2009.07.063
Perova, I. B., Zhogova, A. A., Poliakova, A. V., Éller, K. I., Ramenskaia, G. V., and Samylina, I.A. (2014). Biologically active substances of cornelian cherry fruits (Cornus mas L.). Vopr. Pitan. 83, 86–94.
Popović, B. M., Štajner, D., Slavko, K., and Sandra, B. (2012). Antioxidant capacity of cornelian cherry (Cornus mas L.)–comparison between permanganate reducing antioxidant capacity and other antioxidant methods. Food Chem. 134, 734–741. doi: 10.1016/j.foodchem.2012.02.170
Popović, Z., Matić, R., Bajić-Ljubičić, J., Tešević, V., and Bojović, S. (2017). Geographical variability of selected phenolic compounds in fresh berries of two Cornus species. Trees. 32, 203–214. doi: 10.1007/s00468-017-1624-5
Pyrkosz-Biardzka, K., Kucharska, A., Sokol-Łetowska, A., Strugała, P., and Gabrielska, J. (2014). A comprehensive study on antioxidant properties of crude extracts from fruits of Berberis vulgaris L., Cornus mas L. and Mahonia aquifolium Nutt. Pol. J. Food Nutr. Sci. 64, 91–99. doi: 10.2478/v10222-012-0097-x
Qu, Z., Zheng, N., Zhang, Y., Zhang, L., Liu, J., Wang, Q., et al. (2016). Preventing the BDNF and NGF loss involved in the effects of cornel iridoid glycoside on attenuation of experimental autoimmune encephalomyelitis in mice. Neurol. Res. 38, 831–837. doi: 10.1080/01616412.2016.1200766
Rad, Z. S., Pakbin, B., Rahmani, K., Allahyari, S., Karami, K., Mahmoudi, R., et al. (2016). Physicochemical properties of cornelian cherry fruit (Cornus mas L.) grown in Qazvin province. Iran. J. Biol. Todays World 5, 209–212. doi: 10.15412/J.JBTW.01051201
Radovanović, B. C., Milenković-Andelković, A. S., Radovanović, A. B., and Andelković, M. Z. (2013). Antioxidant and antimicrobial activity of polyphenol extracts from wild berry fruits grown in Southeast Serbia. Trop. J. Pharmaceut. Res. 12, 813–819. doi: 10.4314/tjpr.v12i5.23
Radulović, N. S., Blagojević, P. D., Stojanović-Radić, Z. Z., and Stojanović, N. M. (2013). Antimicrobial plant metabolites: structural diversity and mechanism of action. Curr. Med. Chem. 20, 932–952. doi: 10.2174/092986713805219136
Rafieian-Kopaei, M., Asgary, S., Adelnia, A., Setorki, M., Khazaei, M., Kazemi, S., and Shamsi, F. (2011). The effects of cornelian cherry on atherosclerosis and atherogenic factors in hypercholesterolemic rabbits. J. Med. Plants Res. 5, 2670–2676.
Rahman, I., Biswas, S. K., and Kirkham, P. A. (2006). Regulation of inflammation and redox signaling by dietary polyphenols. Biochem. Pharmacol. 72, 1439–1452. doi: 10.1016/j.bcp.2006.07.004
Roh, C., and Jung, U. (2012). Screening of crude plant extracts with abti-obesity activity. Int. J. Mol. Sci. 13, 1710–1719. doi: 10.3390/ijms13021710
Rop, O., Mlcek, J., Kramarova, D., and Jurikova, T. (2010). Selected cultivars of cornelian cherry (Cornus mas L.) as a new food source for human nutrition. Afr. J. Biotechnol. 9, 1205–1210. doi: 10.5897/AJB09.1722
Rosu, C. M., Olteanu, Z., Truta, E., Ciornea, E., Manzu, C., and Zamfirache, M. M. (2011). Nutritional value of Rosa spp. L. and Cornus mas L. fruits, as affected by storage conditions. Anal. Alexandru Ioan Cuza Univ. Sect. II Genet. Mol. Biol. 12, 147–155.
Rudrapaul, P., Kyriakopoulos, A. M., De, U. C., Zoumpourlis, V., and Dinda, B. (2015). New flavonoids from the fruits of Cornus mas, Cornaceae. Phytochem. Lett. 11, 292–295. doi: 10.1016/j.phytol.2015.01.011
Saei, H., Hatami, H., Azarmi, M., and Dehghan, G. (2016). Hepatoprotective effect of Cornus mas fruits extract on serum biomarkers in methotrexate induced liver injury in male rats. Pharmacol. Online 1, 91–98.
Sak, K. (2014). Cytotoxicity of dietary flavonoids on different human cancer types. Pharmacogn. Rev. 8, 122–146. doi: 10.4103/0973-7847.134247
Seeram, N. P., Schutzki, R., Chandra, A., and Nair, M. G. (2002). Characterization, quantification, and bioactivities of anthocyanins in Cornus species. J. Agric. Food Chem. 50, 2519–2523. doi: 10.1021/jf0115903
Shao, Y. (2011). Extraction of soluble dietary fiber and hemicellulose from Cornus officinalis residue and preparation of fiber drinking water. Front. Agric. China 5, 375–381. doi: 10.1007/s11703-011-1078-2
Shishehbor, F., Azemi, M. E., Zameni, D., and Saki, A. (2016). Inhibitory effects of hydroalcoholic extracts of barberry, sour cherry and Cornelian cherry on α-amylase and α-glucosidase activities. Int. J. Pharm. Res. Allied Sci. 5, 423–428.
Sochor, J., Jurikova, T., Ercisli, S., Mlcek, J., Baron, M., Balla, S., et al. (2014). Charcterization of cornelian cherry (Cornus mas L.) genotypes–genetic resources for food production in Czech Republic. Genetika 46, 915–924. doi: 10.2298/GENSR1403915S
Soltani, R., Gorji, A., Asgary, S., Sarrafzadegan, N., and Siavash, M. (2015). Evaluation of the effects of Cornus mas L. fruit extract on glycemic control and insulin level in type 2 diabetic adult patients: a randomized double-blind placebo-controlled clinical trial. Evid. Based Complement. Alternat. Med. 2015:740954. doi: 10.1155/2015/740954
Sozanski, T., Kucharska, A. Z., Rapak, A., Szumny, D., Trocha, M., Merwid-Lad, A., et al. (2016). Iridoid-loganic acid versus anthocyanins from the Cornus mas fruits (cornelian cherry): common and different effects on diet-induced atherosclerosis, PPARs expression and inflammation. Atherosclerosis 254, 151–160. doi: 10.1016/j.atherosclerosis.2016.10.001
Sozanski, T., Kucharska, A. Z., Szumny, A., Magdalan, J., Bielska, K., Merwid-Lad, A., et al. (2014). The protective effect of the Cornus mas fruits (cornelian cherry) on hypertriglyceridemia and atherosclerosis through PPARalpha activation in hypercholesterolemic rabbits. Phytomedicine 21, 1774–1784. doi: 10.1016/j.phymed.2014.09.005
Stankovic, M. S., Zia-Ul-Haq, M., Bojovic, B. M., and Topuzovic, M. D. (2014). Total phenolics, flavonoid content and antioxidant power of leaf, flower and fruits from cornelian cherry (Cornus mas L.). Bulg. J. Agric. Sci. 20, 358–363.
Sun, F. L., Wang, W., Cheng, H., Wang, Y., Li, L., Xue, J. L., et al. (2014a). Morroniside improves microvascular functional integrity of the neurovascular unit after cerebral ischemia. PLoS ONE 9:e101194. doi: 10.1371/journal.pone.0101194
Sun, F. L., Wang, W., Zuo, W., Xue, J. L., Xu, J. D., Ai, H. X., et al. (2014b). Promoting neurogenesis via Wnt/beta-catenin signaling pathway accounts for the neurorestorative effects of morroniside against cerebral ischemia injury. Eur. J. Pharmacol. 738, 214–221. doi: 10.1016/j.ejphar.2014.05.019
Sung, Y., Chang, H., Kim, S., Ym, K., Seo, J., Shin, M., et al. (2009). Anti-inflammatory and analgesci effects of the aqueous extract of Corni fructus in murine RAW 264.7 macrophage cells. J. Med. Food 12, 788–795. doi: 10.1089/jmf.2008.1011
Szumny, D., Sozanski, T., Kucharska, A. Z., Dziewiszek, W., Piorecki, N., Magdalan, J., et al. (2015). Application of cornelian cherry iridoid-polyphenolic fraction and loganic acid to reduce intraocular pressure. Evid. Based Complement. Alternat. Med. 2015:939402. doi: 10.1155/2015/939402
Tarko, T., Duda-Chodak, A., Satora, P., Sroka, P., Pogon, P., and Machalica, J. (2014). Chaenomeles japonica, Cornus mas, Morus nigra fruits characteristics and their processing potential. J. Food Sci. Technol. 51, 3934–3941. doi: 10.1007/s13197-013-0963-5
Tian, G., Zhang, T., Yang, F., and Ito, Y. (2000). Separation of gallic acid from Cornus officinalis Sieb. et Zucc by high-speed counter-current chromatography. J. Chromatogr. A 886, 309–312. doi: 10.1016/S0021-9673(00)00480-5
Tiwari, R., Latheef, S. K., Ahmed, I., Iqbal, H. M. N., Bule, M. H., Dhama, K., et al. (2018). Herbal immunomodulators, a remedial panacea for the designing and developing effective drugs and medicines: Current scenario and future prospects. Curr. Drug Metab. 19, 264–301. doi: 10.2174/1389200219666180129125436
Tural, S., and Koca, I. (2008). Physicochemical and antioxidant properties of cornelian cherry fruits (Cornus mas L.) grown in Turkey. Sci. Hort. 116, 362–366. doi: 10.1016/j.scienta.2008.02.003
Turker, A., Yildirim, A., and Karakas, F. (2012). Antibacterial and antitumor activities of some wild fruits grown in Turkey. Biotechnol. Biotechnol. Eq. 26, 2765–2772. doi: 10.5504/BBEQ.2011.0156
Vareed, S. K., Reddy, M. K., Schutzki, R. E., and Nair, M. G. (2006). Anthocyanins in Cornus alternifolia, Cornus controversa, Cornus kousa and Cornus florida fruits with health benefits. Life Sci. 78, 777–784. doi: 10.1016/j.lfs.2005.05.094
Wang, H., Wang, Z., and Guo, W. (2008). Comparative determination of ursolic acid and oleanolic acid of Macrocarpium officinalis (Sieb. et Zucc.) Nakai by RP-HPLC. Ind. Crops Prod. 28, 328–332. doi: 10.1016/j.indcrop.2008.03.004
Wang, W., Huang, W., Li, L., Ai, H., Sun, F., Liu, C., et al. (2008). Morroniside prevents peroxide-induced apoptosis by induction of endogenous glutathione in human neuroblastoma cells. Cell. Mol. Neurobiol. 28, 293–305. doi: 10.1007/s10571-007-9168-7
Wang, W., Sun, F., An, Y., Ai, H., Zhang, L., Huang, W., et al. (2009). Morroniside protects human neuroblastoma SH-SY5Y cells against hydrogen peroxide-induced cytotoxicity. Eur. J. Pharmacol. 613, 19–23. doi: 10.1016/j.ejphar.2009.04.013
Wang, W., Xu, J., Li, L., Wang, P., Ji, X., Ai, H., et al. (2010). Neuroprotective effect of morroniside on focal cerebral ischemia in rats. Brain Res. Bull. 83, 196–201. doi: 10.1016/j.brainresbull.2010.07.003
Wang, X., Liu, J., Jin, N. A., Xu, D., Wang, J., Han, Y., et al. (2015). Fructus Corni extract-induced neuritogenesis in PC12 cells is associated with the suppression of stromal interaction molecule 1 expression and inhibition of Ca(2+) influx. Exp. Ther. Med. 9, 1773–1779. doi: 10.3892/etm.2015.2316
Wang, X., Zhang, A., Zou, D., Wang, Y., and Sun, H. (2017). “UPLC-MS determining the active ingredients of herbal Fructus Corni in vivo,” in Serum Pharmacochemistry of Traditional Chinese Medicine. Technologies, Strategies and Applications, ed X. Wang (London: Academic Press; Elsevier), 269–286.
West, B. J., Deng, S., Jensen, C. J., Palu, A. K., and Berrio, L. F. (2012a). Antioxidant, toxicity, and iridoids tests of processed Cornelian cherry fruits. Int. J. Food Sci. Technol. 47, 1392–1397. doi: 10.1111/j.1365-2621.2012.02985.x
West, B. J., Ma, D.-L., Deng, S., Jensen, C. J., and Su, C. X. (2012b). Bioactivities and iridoid determination of a beverage containing noni, cornelian cherries and olive leaf extracts. Adv. J. Food Sci. Technol. 4, 91–96.
Wu, Y., Wang, X., Shen, B., Kang, L., and Fan, E. (2013). Extraction, structure and bioactivities of the polysaccharides from Fructus corni. Recent Pat. Food Nutr. Agric. 5, 57–61. doi: 10.2174/2212798411305010009
Xia, B., Xu, B., Sun, Y., Xiao, L., Pan, J., Jin, H., et al. (2014). The effects of Liuwei Dihuang on canonical Wnt/beta-catenin signaling pathway in osteoporosis. J. Ethnopharmacol. 153, 133–141. doi: 10.1016/j.jep.2014.01.040
Xiang, Q.-Y., Brunsfeld, S., Soltis, D., and Soltis, P. (1996). Phylogenetic relationships in Cornus based on chloroplast DNA restriction sites; implications for biogeography and character evolution. Sys. Bot. 21, 515–534. doi: 10.2307/2419612
Xiang, Q.-Y., Manchester, S., Thomas, D., Zhang, W., and Fan, C. (2005). Phylogeny, biogeography, and molecular dating of cornelian cherries (Cornus, Cornaceae): tracking tertiary plant migration. Evolution 59, 1685–1700. doi: 10.1111/j.0014-3820.2005.tb01818.x
Xiang, Q.-Y., Moody, M., Soltis, D., Fan, C., and Soltis, P. (2002). Relationships within Cornales and circumscription of Cornaceae–matK and rbcL sequence data and effects of outgroups and long branches. Mol. Phylogenet. Evol. 24, 35–57. doi: 10.1016/S1055-7903(02)00267-1
Xiang, Q.-Y., Soltis, D., and Soltis, P. (1998). Phylogenetic relationships of Cornaceae and close relatives inferred from matK and rbcL sequences. Am. J. Bot. 85:285. doi: 10.2307/2446317
Xiang, Q.-Y., Thomas, D., Zhang, W., Manchester, S., and Murrell, Z. (2006). Species level phylogeny of the genus Cornus (Cornaceae) based on molecular and morphological evidence–implications for taxonomy and Tertiary intercontinental migration. Taxon 55, 9–30. doi: 10.2307/25065525
Xiang, Q.-Y., Thorne, J., Seo, T., Zhang, W., Thomas, D., and Ricklefs, R. (2008). Rates of nucleotide substitution in Cornaceae (Cornales)–pattern of variation and underlying causal factors. Mol. Phylogenet. Evol. 49, 327–342. doi: 10.1016/j.ympev.2008.07.010
Xie, X.-Y., Wang, R., and Shi, Y.-P. (2012). Chemical constituents from the fruits of Cornus officinalis. Biochem. Syst. Ecol. 45, 120–123. doi: 10.1016/j.bse.2012.07.025
Xu, H., Shen, J., Liu, H., Shi, Y., Li, L., and Wie, M. (2006). Morroniside and loganin extracted from Cornus officinalis have protective effects on rat mesangial cell proliferation exposed to advanced glycation end products by preventing oxidative stress. Can. J. Physiol. Pharmacol. 84, 1267–1273. doi: 10.1139/y06-075
Yalcinkaya, E., Erbil, Y., and Bas, M. (2009). Pomological properties of some cornelian cherry (Cornus mas L.) genotypes selected in southern Marmata region. Acta Hort. 818, 149–154. doi: 10.17660/ActaHortic.2009.818.21
Yamabe, N., Kang, K. S., Park, C. H., Tanaka, T., and Yokozawa, T. (2009). 7-O-galloyl-D-sedoheptulose is a novel therapeutic agent against oxidative stress and advanced glycation endproducts in the diabetic kidney. Biol. Pharm. Bull. 32, 657–664. doi: 10.1248/bpb.32.657
Yang, C. C., Kuai, X. X., Gao, W. B., Yu, J. C., Wang, Q., Li, L., et al. (2016). Morroniside-induced PP2A activation antagonizes tau hyperphosphorylation in a cellular model of neurodegeneration. J. Alzheimers Dis. 51, 33–44. doi: 10.3233/JAD-150728
Yang, L., Wang, Z., and Huang, L. (2010). Isolation and structural characterization of a polysaccharide FCAP1 from the fruit of Cornus officinalis. Carbohydr. Res. 345, 1909–1913. doi: 10.1016/j.carres.2010.06.009
Yao, R. Q., Zhang, L., Wang, W., and Li, L. (2009). Cornel iridoid glycoside promotes neurogenesis and angiogenesis and improves neurological function after focal cerebral ischemia in rats. Brain Res. Bull. 79, 69–76. doi: 10.1016/j.brainresbull.2008.12.010
Ye, X. S., He, J., Cheng, Y. C., Zhang, L., Qiao, H. Y., Pan, X. G., et al. (2017). Cornusides A-O, bioactive iridoid glucoside dimers from the fruit of Cornus officinalis. J. Nat. Prod. 80, 3103–3111. doi: 10.1021/acs.jnatprod.6b01127
Yilmaz, K. U., Ercisli, S., Zengin, Y., Sengul, M., and Kafkas, E. Y. (2009). Preliminary characterisation of cornelina cherry (Cornus mas L.) genotypes for their physico-chemical properties. Food Chem. 114, 408–412. doi: 10.1016/j.foodchem.2008.09.055
Yin, L., Chen, Y., Qu, Z., Zhang, L., Wang, Q., Zhang, Q., et al. (2014). Involvement of JAK/STAT signaling in the effect of cornel iridoid glycoside on experimental autoimmune encephalomyelitis amelioration in rats. J. Neuroimmunol. 274, 28–37. doi: 10.1016/j.jneuroim.2014.06.022
Yin, X., You, Q., Jiang, Z., and Zhou, X. (2016). Optimization for ultrasonic-microwave synergistic extraction of polysaccharides from Cornus officinalis and characterization of polysaccharides. Int. J. Biol. Macromol. 83, 226–232. doi: 10.1016/j.ijbiomac.2015.11.059
Yokozawa, T., Kang, K. S., Park, C. H., Noh, J. S., Yamabe, N., Shibahara, N., et al. (2010). Bioactive constituents of Corni fructus: the therapeutic use of morroniside, loganin, and 7-O-galloyl-D-sedoheptulose as renoprotective agents in type 2 diabetes. Drug Disc. Therapeut. 4, 223–234.
Yokozawa, T., Park, C. H., Noh, J. S., Tanaka, T., and Cho, E. J. (2009). Novel action of 7-O-galloyl-D-sedoheptulose isolated from Corni Fructus as a hypertriglyceridaemic agent. J. Pharm. Pharmacol. 61, 653–661. doi: 10.1211/jpp/61.05.0015
Yokozawa, T., Yamabe, N., Kim, H. Y., Kang, K. S., Hur, J. M., Park, C. H., et al. (2008). Protective effects of morroniside isolated from Corni Fructus against renal damage in streptozotocin-induced diabetic rats. Biol. Pharm. Bull. 31, 1422–1428. doi: 10.1248/bpb.31.1422
You, Q., Yin, X., and Zhao, Y. (2013). Enzyme assisted extraction of polysaccharides from the fruit of Cornus officinalis. Carbohydr. Polym. 98, 607–610. doi: 10.1016/j.carbpol.2013.06.036
Youn, K., and Jun, M. (2012). Inhibitory effects of key compounds isolated from Corni fructus on BACE1 Activity. Phytother. Res. 26, 1714–1718. doi: 10.1002/ptr.4638
Yousefi, B., Abasi, M., Abbasi, M., and Jahanban-Esfahlan, R. (2015). Anti-proliferative properties of Cornus mass fruit in different human cancer cells. Asian Pac. J. Cancer Prev. 16, 5727–5731. doi: 10.7314/APJCP.2015.16.14.5727
Yu, H.-H., Hur, J.-M., Seo, S.-J., Moon, H.-D., Kim, H.-J., Park, R.-K., et al. (2009). Protective effect of ursolic acid from Cornus officinalis on the hydrogen peroxide-induced damage of HEI-OC1 auditory cells. Am. J. Chin. Med. 37, 735–746. doi: 10.1142/S0192415X0900720X
Zhang, Q.-A., Fan, X.-H., Zhao, W.-Q., Wang, X.-Y., and Liu, H.-Z. (2013). Evolution of some physicochemical properties in Cornus officinalis wine during fermentation and storage. Eur. Food Res. Technol. 237, 711–719. doi: 10.1007/s00217-013-2045-3
Zhang, Q. C., Zhao, Y., and Bian, H. M. (2013). Antiplatelet activity of a novel formula composed of malic acid, succinic acid and citric acid from Cornus officinalis fruit. Phytother. Res. 27, 1894–1896. doi: 10.1002/ptr.4934
Keywords: Cornus mas, Cornus officinalis, cornelian cherry, cornel dogwood, traditional Chinese medicine
Citation: Czerwińska ME and Melzig MF (2018) Cornus mas and Cornus Officinalis—Analogies and Differences of Two Medicinal Plants Traditionally Used. Front. Pharmacol. 9:894. doi: 10.3389/fphar.2018.00894
Received: 02 May 2018; Accepted: 23 July 2018;
Published: 28 August 2018.
Edited by:
Rudolf Bauer, University of Graz, AustriaReviewed by:
Dezső Csupor, University of Szeged, HungaryChristian Zidorn, Christian-Albrechts-Universität zu Kiel, Germany
Copyright © 2018 Czerwińska and Melzig. This is an open-access article distributed under the terms of the Creative Commons Attribution License (CC BY). The use, distribution or reproduction in other forums is permitted, provided the original author(s) and the copyright owner(s) are credited and that the original publication in this journal is cited, in accordance with accepted academic practice. No use, distribution or reproduction is permitted which does not comply with these terms.
*Correspondence: Monika E. Czerwińska, mczerwinska@wum.edu.pl
Matthias F. Melzig, matthias.melzig@fu-berlin.de