- 1Zoology Department, College of Science, King Saud University, Riyadh, Saudi Arabia
- 2Al-Jeraisy Chair for DNA Research, Zoology Department, College of Science, King Saud University, Riyadh, Saudi Arabia
- 3National Center for Natural Products Research, School of Pharmacy, The University of Mississippi, Oxford, MS, United States
- 4Department of BioMolecular Sciences, School of Pharmacy, The University of Mississippi, Oxford, MS, United States
Dioscorea villosa, commonly known as “Wild Yam” and native to North America, is well documented for its pharmacological properties due to the presence of steroidal glycosides. However, the hepatoprotective potential of these compounds has not been studied so far. The present investigation was aimed to study the hepatoprotective effect of the steroidal glycosides from D. villosa against H2O2, a known hepatotoxin, in human liver cell line (HepG2). Cytotoxicity assessment was carried out in cells exposed to various concentrations (10–50 μM) of compounds for 24 h using MTT assay and morphological changes. All tested compounds were known and among them, spirostans (zingiberensis saponin I, dioscin, deltonin and progenin III) were found to be cytotoxic whereas, furostans (huangjiangsu A, pseudoprotodioscin, methyl protobioside, protodioscin, and protodeltonin) were non-cytotoxic. Further, HepG2 cells were pretreated with biologically safe concentrations (10, 30, and 50 μM) of non-cytotoxic compounds and then cytotoxic (0.25 mM) concentration of H2O2. After 24 h, cell viability was assessed by MTT and NRU assays, while morphological changes were observed under the microscope. The results showed that treatment of HepG2 cells with compounds prior to H2O2 exposure effectively increased cell viability in a concentration-dependent manner. Furthermore, huangjiangsu A, pseudoprotodioscin, methyl protobioside, protodioscin, and protodeltonin at 50 μM increased GSH level and decreased intracellular ROS generation against H2O2-induced damages. The results from this study revealed that compounds isolated from D. villosa have hepatoprotective potential against H2O2-induced cytotoxicity and ROS generation and could be promising as potential therapeutic agents for liver diseases.
Introduction
The liver is the largest glandular organ, and plays a key role in the regulation of various physiological processes in the human body (Bechmann et al., 2012). It is the most important site of intermediary metabolism responsible for the detoxification and excretion of various substances like xenobiotics by altering and expelling toxins and wastes (Chaudhari and Mahajan, 2016). Owing to continuous exposure to the toxicant via the portal blood circulation, the liver is highly susceptible to damage by xenobiotics, drugs, and hazardous substances (Sharma et al., 2011). Due to handling the metabolism of various proteins, lipids, carbohydrates, secretion of bile, and storage of vitamins, it is considered as one of the most vital organs (Bechmann et al., 2012). The liver diseases are a global health problem therefore, the maintenance of healthy liver is imperative for human health (Haidry and Malik, 2014). A variety of chemicals, such as tert-butyl hydroperoxide (t-BHP), D-galactosamine/lipopolysaccharide, carbon tetrachloride (CCl4), acetaminophen, alcohol, and hydrogen peroxide can cause potential damage to the liver cells leading to progressive dysfunction (Simeonova et al., 2014). Oxidative stress is a redox imbalance between pro-oxidant, i.e., the production of reactive oxygen species (ROS) and antioxidant defense counteracting the reactive intermediates and initiating the cellular damage (Videla, 2009). Oxidative stress is also known to be involved in the mechanism of hepatotoxicity and pathogenesis (Li et al., 2015). Overproduction of ROS results in oxidative stress, a deleterious process that can be an important mediator of damage to cell structures, lipids, membranes, proteins, and DNA (Ray et al., 2012). Hydrogen peroxide (H2O2) is well known to increase the levels of intracellular ROS generation and causes cellular oxidative damage (Cheong et al., 2016). Several evidences also showed that H2O2-induced cytotoxicity in human liver cells (Al-Sheddi et al., 2016; Gao et al., 2016) and has been linked with various alterations including anti- and pro-apoptosis proteins and caspases (Cheong et al., 2016). Therefore, H2O2 was chosen in this study as an inducer of oxidative stress and cytotoxicity in the human liver cell line (HepG2). Despite the recent therapeutic advances and significant development of modern medicine, hepatic diseases remain a health problem worldwide (Hong et al., 2015). Thus, the search for new therapeutic agents to treat liver diseases is still in demand. Many synthetic drugs have been demonstrated to be strong radical scavengers, but they are also carcinogenic and cause liver damage (Cheong et al., 2016). Due to the fact that the available drugs can cause damage to healthy hepatic cells, the new researches have been focused to protect hepatic diseases and reduce adverse side effects by using natural compounds isolated from the plants. A literature survey revealed that many plant extracts and their constituents have constantly shown hepatoprotective effects (Jiménez-Arellanes et al., 2016). It has also been reported that pure compounds and extracts from plants possess good antioxidant activity against chemically induced liver damage (Pereira et al., 2016).
Dioscorea villosa L. (Family: Dioscoreaceae), a tuber vegetable commonly known as “Wild Yam,” is native to North America and has been recommended for menopausal symptoms and menstrual complaints (Komesaroff et al., 2001). Dioscorea plants are rich in steroidal saponins and sapogenins. Over 50 steroid saponins of furostane-, spirostane-, and pregnane-type skeletons have been isolated and characterized from various Dioscorea species, which have been reported to be the major physiologically active constituents in yams (Hayes et al., 2007; Ali et al., 2013a,b). Among the isolated constituents of D. villosa, the cytotoxic activities of diosgenin and dioscin have been studied against a number of cancer cell lines (Kim et al., 2014; Aumsuwan et al., 2016). Hypocholesterolemic and hypoglycemic effects of dioscin have also been reported (McAnuff et al., 2005) and no subchronic toxicity of dioscin was observed in vivo with a high dose for 3 months (Xu et al., 2012). A number of preclinical and mechanistic studies have been reported supporting the antitumor potential of dioscin (Aumsuwan et al., 2016). The epigenetic mechanism of the anticancer effect of wild yam root extract (Aumsuwan et al., 2015) and a detailed study on anticancer potential of dioscin on cell viability, invasion, migration, and wound healing in an invasive breast cancer cell line has been reported (Aumsuwan et al., 2016). These studies demonstrated that constituents of D. villosa possess pharmacological potential especially antitumor effects. To the best of our knowledge, there are no reports available on their hepatoprotective effect. Therefore, this study was aimed to investigate the hepatoprotective effect of the constituents of D. villosa against H2O2-induced cytotoxicity and oxidative stress in HepG2 cell line. HepG2 cell line is well reported to be involved in the metabolism (Kim et al., 2011) and have been proven to be a good model system for cytotoxicity assessment and cyto-protective potential of various compounds against the toxic substances (González et al., 2017).
Materials and Methods
Chemicals and Consumables
Dulbecco’s modified Eagle medium (DMEM) culture medium, trypsin, fetal bovine serum (FBS), and antibiotics/antimycotic solution were procured from Invitrogen, Life Technologies, Grand Island, NY, United States. Plastic wares and other consumables used in the study were purchased from Nunc, Roskilde, Denmark. H2O2 and all specified chemicals and reagents were purchased from Sigma Chemical Company Pvt., Ltd., St. Louis, MO, United States.
Plant Material
Root powder of Dioscorea villosa L. was purchased from Starwest Botanicals, United States. A sample specimen of the purchased material (No. 9412) was deposited at the National Center for Natural Products Research, The University of Mississippi.
Extraction and Isolation
Root powder (0.9 kg) was extracted with MeOH (3.0 L × 4 × 24 h) at room temperature. Following the removal of the solvent, a gummy residue (MeOH extract, 75 g) was obtained. An aliquot (55 g) was subjected to size exclusion chromatography using Sephadex LH-20 (17 × 10 cm) and eluted with MeOH to furnish 2 parts, A (7.0 L, 45.2 g) and B (5.0 L, 9.4 g). Part A (30 g) was separated by normal phase column chromatography (NPCC) using silica gel (30 × 10 cm) into 12 fractions (A1–A12) with lower layer of CHCl3-MeOH-H2O (13:7:2) [A1 (0.5 L, 782 mg), A2 (1.0 L, 730 mg), A3 (0.5 L, 304 mg), A4 (0.5 L, 767 mg), A5 (0.5 L, 490 mg), A6 (0.5 L, 1.8 g), A7 (1.0 L, 657 mg), A8 (1.0 L, 2.8 g), A9 (1.0 L, 1.9 g), A10 (1.5 L, 4.4 g), A11 (1.5 L, 4.5 g), and A12 (1.5 L, 5.9 g)]. Fractions A3 and A4 were individually subjected to NPCC [silica gel (87 × 1.3 cm), EtOAc-CHCl3-MeOH-H2O (10:6:4:1), 1.0 L] to yield progenin III (375 mg) and dioscin (126 mg). Deltonin (785 mg) and zingiberensis saponin I (90 mg) were obtained from fractions A6 and A8, respectively, as MeOH insoluble materials. Methyl protobioside (325 mg) was purified from the MeOH soluble part of fraction A8 by NPCC [silica gel (87 × 2.5 cm), CHCl3-MeOH-H2O (32:8:1), 4.5 L] and RPC [RP-18 silica gel (37 × 2.5 cm), acetone-H2O (1:1), 0.5 L]. Fraction A9 was resolved by NPCC [silica gel (37 × 2.5 cm), lower layer of CHCl3-MeOH-H2O (13:7:2), 2.7 L] into three subfractions (A9a–A9c). Pseudoprotodioscin (45 mg) and protodioscin (212 mg) were purified from subfraction A9c (665 mg) by RPC [RP-18 silica gel (45 × 2.5 cm), acetone-H2O (2:3), 0.7 L]. Huangjiangsu A (646 mg) and protodeltonin (1.3 g) were purified from fraction A11 by RPC [RP-18 silica gel (50 × 3.7 cm), acetone-H2O (2:3), 2.5 L].
Cell Culture
HepG2 cell line was purchased from ATCC and was cultured in DMEM, supplemented with 10% FBS, 0.2% sodium bicarbonate and antibiotic/antimycotic solution (1 mL/100 mL of medium). Cells were grown in a CO2 incubator at 37°C. Prior to the experiments, viability of cells was assessed using the previously described protocol (Siddiqui et al., 2008). Batches showing more than 98% cell viability and passage number of cells between 22 and 24 were used in this study.
Experimental Design
For cytotoxicity assessments, HepG2 cells were exposed to various concentrations (10–50 μM) of the D. villosa compounds and H2O2 (0.025–2 mM) for 24 h. To study the cytoprotective potential, HepG2 cells were pretreated with biologically safe concentrations (10, 30, and 50 μM) of non-cytotoxic D. villosa compounds (huangjiangsu A, pseudoprotodioscin, methyl protobioside, protodioscin, and protodeltonin) and then cytotoxic concentrations of H2O2 (0.25 mM) were added in the medium containing compounds for 24 h. Further, for protective potential of compounds on GSH level and intracellular ROS generation against H2O2, cells were pre-exposed to 50 μM of the D. villosa compounds for 24 h then, H2O2 (0.25 mM) for 24 h.
Preparation of Stock Solutions of Compounds
The stock solutions of all the compounds (25 mM) were prepared in dimethylsulfoxide (DMSO) and diluted in culture medium to reach the desired concentrations. The final DMSO concentration used for the experiments was not more than 0.2% and this was used as control.
Cytotoxicity Assessment by MTT Assay
The MTT assay was performed for cytotoxicity assessments following the method described (Siddiqui et al., 2008). In brief, HepG2 cells were seeded in 96-well plates at a density of 1 × 104 cells per well and allowed to grow overnight. Then, cells were exposed to various concentrations of compounds of D. villosa for 24 h. After the exposure, 50 μg MTT was added in each well and plates were incubated further for 4 h. Then, the reaction mixture was carefully taken out and 200 μL of DMSO was added in each well and mixed gently by pipetting up and down. The absorbance of plates was measured at 550 nm.
Cytotoxicity Assessment by Neutral Red Uptake (NRU) Assay
Neutral red uptake (NRU) assay was performed according to the method described (Siddiqui et al., 2008). In brief, cells were exposed to various concentrations of compounds of D. villosa for 24 h. After the exposure, the test solution was aspirated and cells were washed twice with PBS and incubated further in medium containing 50 μg/mL neutral red for 3 h. Then, the medium was washed with a solution of CaCl2 (1%) and formaldehyde (0.5%). Following 20 min incubation at 37°C in a mixture of acetic acid (1%) and ethanol (50%), the plates were read at 550 nm.
Morphological Changes by Phase Contrast Microscope
The alterations in the morphology was observed under the inverted microscope to determine the changes induced by compounds of D. villosa in HepG2 cells exposed to 10–50 μM for 24 h. The cell images were grabbed using phase contrast inverted microscope (Olympus) at 20× magnification.
Glutathione (GSH) Level
The level of glutathione in HepG2 cells was measured following the method (Chandra et al., 2002). In brief, HepG2 cells were exposed to 50 μM of the compounds of D. villosa for 24 h and then H2O2 (0.25 mM) for 24 h. After respective exposure, cells were centrifuged and cellular protein was precipitated with 1 mL TCA (10%) on ice for 1 h. Supernatant was taken by centrifugation at 956 × g for 10 min. Then, 2 mL of 0.4 M Tris buffer (pH 8.9) containing 0.02 M EDTA and 0.01 M 5,5′-dithionitrobenzoic acid (DTNB) were added in the supernatant. The absorbance of the developed yellow color was read at 412 nm after incubating for 10 min at 37°C.
Reactive Oxygen Species (ROS) Generation
The intracellular ROS generation was measured using 2,7-dichlorodihydrofluorescein diacetate (DCFH-DA) dye (Siddiqui et al., 2013). HepG2 cells were exposed to 50 μM of the compounds for 24 h and then H2O2 (0.25 mM) was added in the medium containing compounds for 24 h. After the respective exposure, HepG2 cells were washed with PBS and incubated with DCFH-DA (5 μM) at 37°C for 1 h in dark. The fluorescence of cells was analyzed using a fluorescence microscope (Olympus).
Statistical Analysis
Data were statistically analyzed by ANOVA and results are presented as mean ± standard deviation (SD) of three separate experiments (N = 6). The treated and control groups were compared using the post hoc Dunnett’s test using statistical analysis software GraphPad Prism and considered a significant level of p < 0.05.
Results and Discussion
Compounds
The molecular formula, C51H82O21, of pseudoprotodioscin was determined from an [M+Na]+ ion peak in the HRESIMS at m/z 1053.5347 and 51 carbon resonances in its 13C NMR spectrum attributable to furostan steroid and four sugar units. Its structure was elucidated by analyzing 1D and 2D NMR spectral data which were comparable with those of published in the literature (Yoshikawa et al., 2007). An [M+H]+ ion peak at m/z 1047.5482 in the HRESIMS and 51 carbon resonances (ascribable to furostan steroid and four sugar units) in the 13C NMR spectrum of huangjiangsu A, established its molecular formula as C51H82O22. Its structure was elucidated by analyzing the NMR data, which were analogous to those of published in the literature (Ali et al., 2013c). Methyl protobioside (Silva et al., 1998; Shen et al., 2002), protodioscin (Shao et al., 1997), and protodeltonin (Munafo et al., 2010) were also identified as furostan steroid glycosides by comparing their NMR data with those of reported. Methyl protobioside, protodioscin, and protodeltonin showed [M+Na]+ ion peaks in their HRESI mass spectra at m/z 939.5056 (corresponded to molecular formula of C46H76O18), m/z 1071.5453 (corresponded to molecular formula of C51H84O22), and m/z 1087.5383 (corresponded to molecular formula of C51H84O23), respectively. Zingiberensis saponin I, progenin III, dioscin, and deltonin were identified as spirostan steroidal glycosides by analyzing their 1D and 2D NMR spectroscopic and HRESIMS spectrometric data as well as by comparing their NMR data with those of published in the literature (Hayes et al., 2007). The HRESI mass spectra of zingiberensis saponin I, progenin III, dioscin, and deltonin displayed [M+Na]+ ion peaks at m/z 1069.5209 (C51H82O22+Na), m/z 745.4219 (C39H62O12+Na), m/z 891.4803 (C45H72O16+Na), and m/z 907.4587 (C45H72O17+Na), respectively. The structures of the isolated compounds are given in Figure 1 and their purities were found to be over 95%, except for protodioscin (90% pure).
Cytotoxicity Assessment of Compounds and H2O2
The cytotoxic effects of compounds have been assessed by MTT assay and morphological changes. The results (Figures 2, 3) showed that huangjiangsu A, pseudoprotodioscin, methyl protobioside, protodioscin, and protodeltonin (furostane-type steroidal glycosides) did not cause any effects on cell viability of HepG2 cells (Figure 2A) at 10–50 μM concentrations. However, some compounds, i.e., zingiberensis saponin I (furostane-type steroidal glycoside), dioscin, deltonin, and progenin III (spirostane-type steroidal glycosides) were found to be cytotoxic (Figure 2B). Moreover, the cytotoxic compounds induced cell damage was complemented by changes in cell morphology as observed in the loss of the characteristics in round form; however, no change was observed in non-cytotoxic compounds at highest concentration, i.e., 50 μM (Figure 3). Further, the non-cytotoxic compounds were selected to study the hepatoprotective effect against H2O2-induced cytotoxicity in HepG2 cells. As shown in Figure 4, H2O2 ranging from 0.05 to 2 mM induced cell death in a concentration-dependent manner. Based on the cytotoxicity data, the 0.25 mM concentration of H2O2-induced cytotoxicity in a moderate manner, therefore, this concentration (0.25 mM) of H2O2 was used for all further experiments. The results of this study are consistent with the previous reports of concentration-dependent cytotoxicity of H2O2 in HepG2 (Al-Sheddi et al., 2016) and other cells (Sattayasai et al., 2013). These studies also demonstrated that H2O2 could be used as a substance to induce oxidative stress mediated cytotoxicity in cell system.
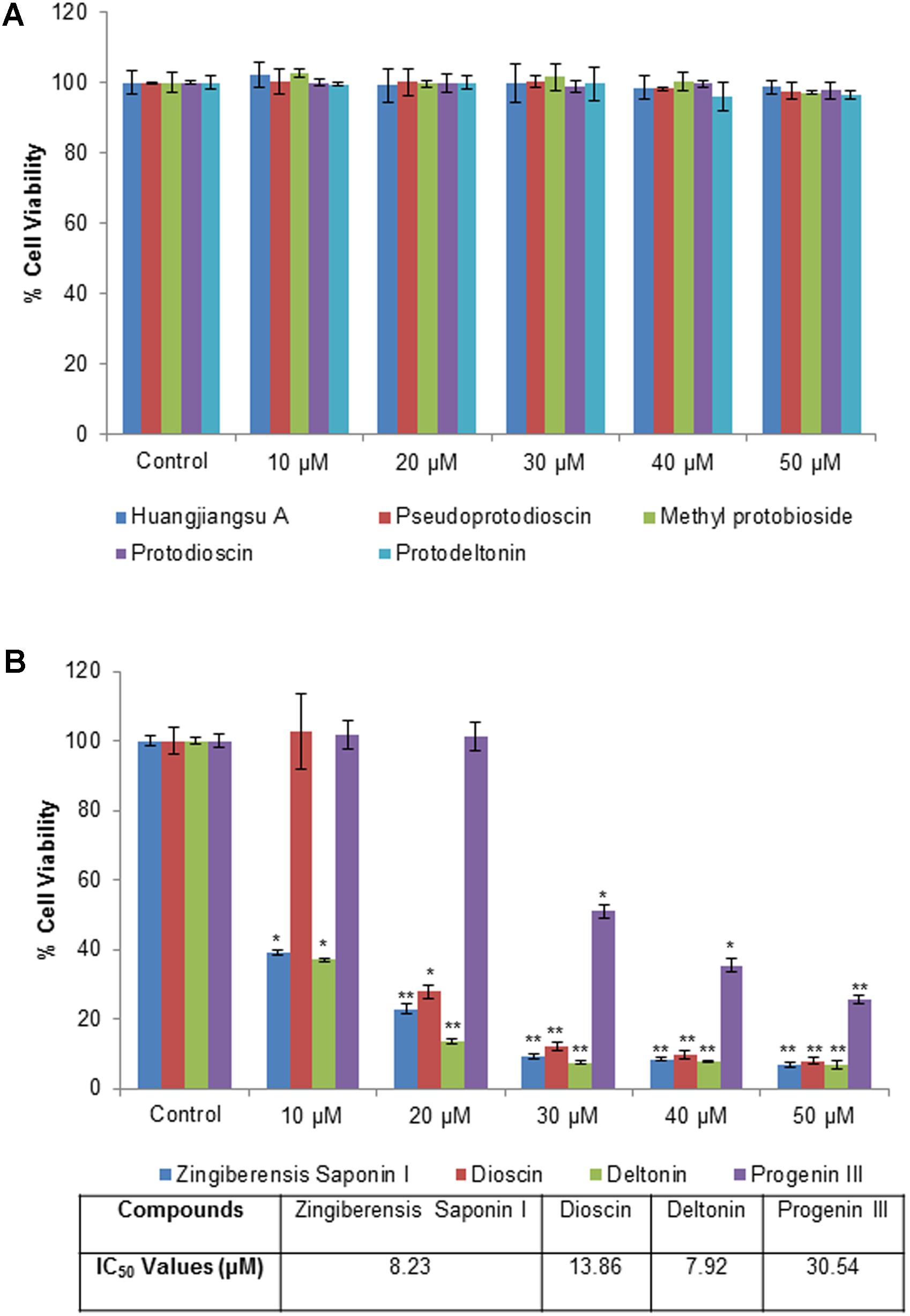
FIGURE 2. Cytotoxicity assessment by MTT assay in HepG2 cells following the exposure of different concentrations of compounds isolated from Dioscorea villosa. All values are given as mean ± SD. (A) Non-cytotoxic compounds and (B) Cytotoxic compounds. ∗p < 0.05, ∗∗p < 0.01 versus control.
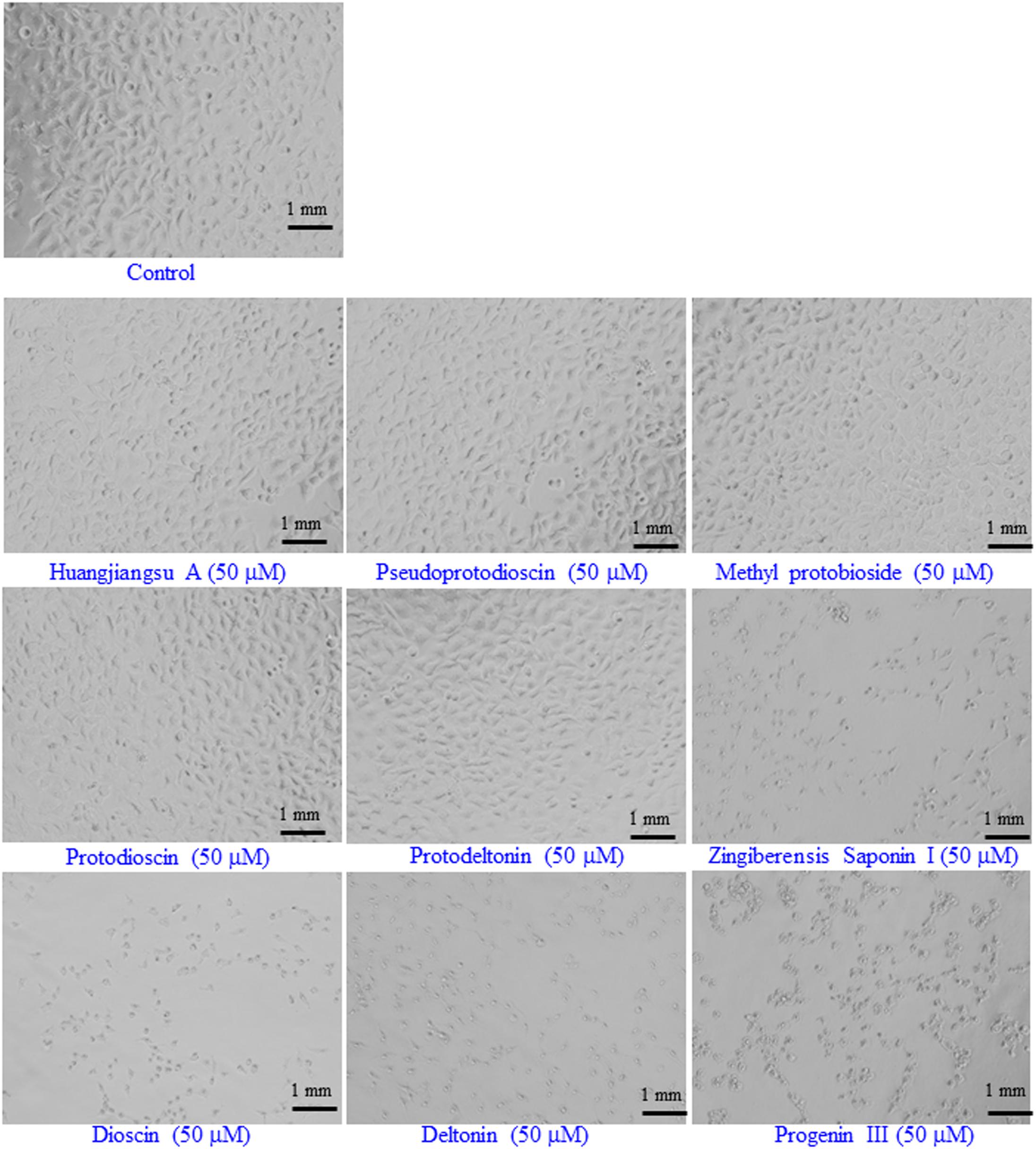
FIGURE 3. Representative images of the morphological changes in HepG2 cells after the exposure of the compounds isolated from Dioscorea villosa. All the images were acquired using a phase contrast inverted microscope at 20× magnification. Each scale bar = 1 mm.
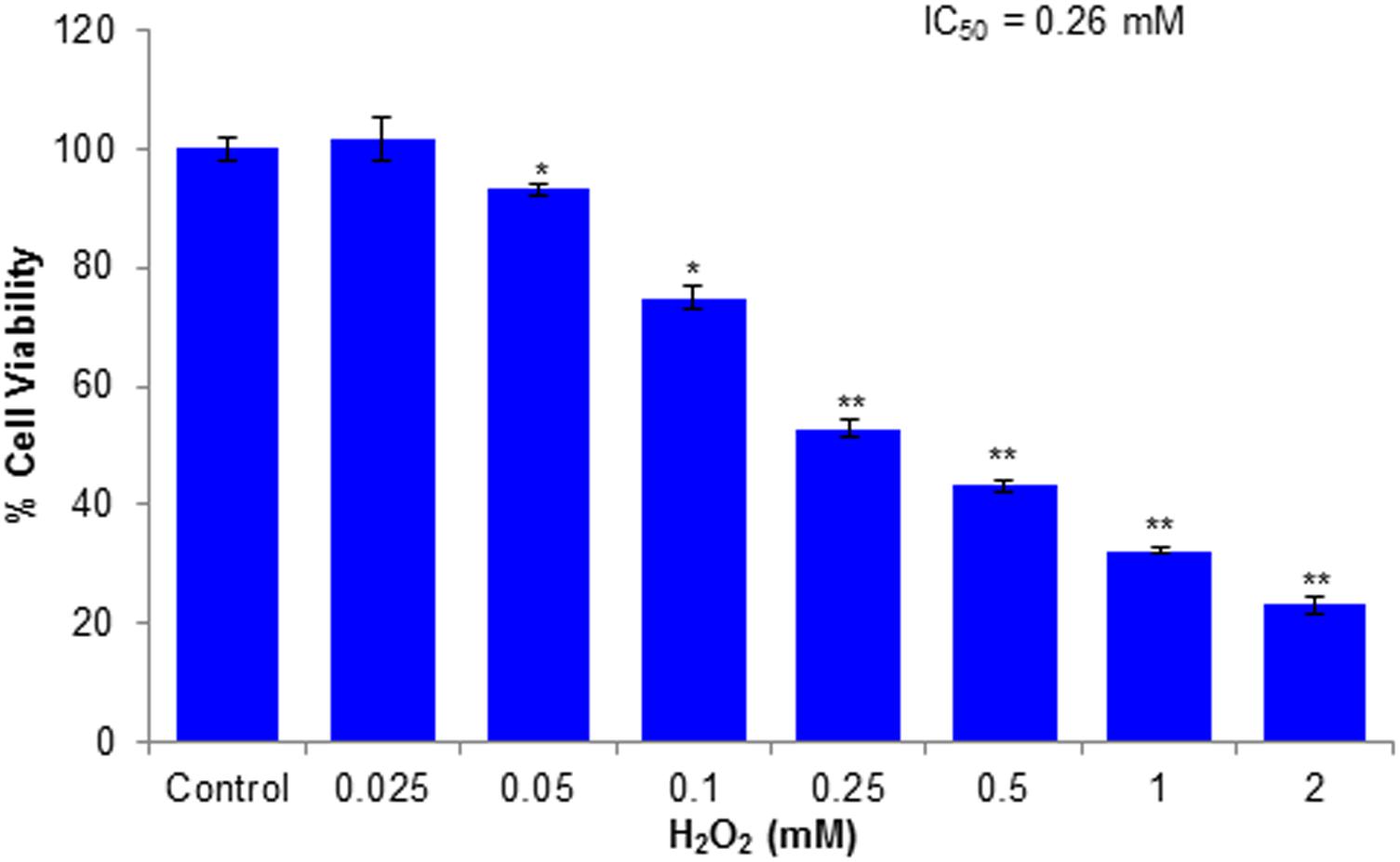
FIGURE 4. Cytotoxicity assessments by MTT assay in HepG2 cells following the exposure of various concentrations of H2O2 for 24 h. All values are given as mean ± SD. ∗p < 0.05, ∗∗p < 0.01 versus control.
Cytoprotective Potential of Compounds Against H2O2-Induced Cytotoxicity
To illustrate cytoprotective effects of the non-cytotoxic compounds (huangjiangsu A, pseudoprotodioscin, methyl protobioside, protodioscin, and protodeltonin) on cell viability of HepG2 cells against cytotoxicity induced by the H2O2, the HepG2 cells were incubated with 10, 30, and 50 μM concentrations of test compounds for 24 h and then with cytotoxic concentration (0.25 mM) of H2O2. The H2O2-induced cell death and hepatoprotective effect were determined by MTT and NRU assays and morphological changes. The protective potential of each compound as observed in HepG2 cells is presented in Figure 5. A significant (p < 0.01) reduction in cell viability was observed in HepG2 cells following the exposure with H2O2 (0.25 mM) for 24 h by MTT assay (Figure 5A), and NRU assay (Figure 5B). However, a concentration-dependent increase in the cell viability was observed in HepG2 cells pre-exposed to huangjiangsu A, pseudoprotodioscin, methyl protobioside, protodioscin, and protodeltonin compounds at 10, 30, and 50 μM for 24 h. The increase in cell viability was observed to be 13, 24, and 29% by huangjiangsu A; 15, 24, and 32% by pseudoprotodioscin; 10, 19, and 26% by methyl protobioside; 16, 25, and 34% by protodioscin; and 20, 30, and 37% by protodeltonin at 10, 30, and 50 μM, respectively, in pre-exposed HepG2 cells (Figure 5A). Similar to MTT assay, a concentration-dependent protective effect was also observed in NRU assay. The increase in cell viability by NRU assay was observed to be 13, 23, and 28% by huangjiangsu A; 15, 30, and 36% by pseudoprotodioscin; 14, 25, and 28% by methyl protobioside; 17, 26, and 33% by protodioscin; and 21, 31, and 37% by protodeltonin at 10, 30, and 50 μM concentrations, respectively, in pre-exposed HepG2 cells (Figure 5B). The results of this study showed that pre-exposure of cells to the test compounds significantly increased the viability of HepG2 cells against H2O2-induced cell death. Our results support the previous studies that compounds isolated from natural products exhibited significant hepatoprotective effects against H2O2 (Xia et al., 2017) and against other toxicants such as acetaminophen and CCl4 (González et al., 2017). Several studies have also demonstrated that various compounds isolated from plants exhibited prominent cytoprotective potential against H2O2-induced cell death in different cell system (Yang et al., 2015b; Wang et al., 2015).
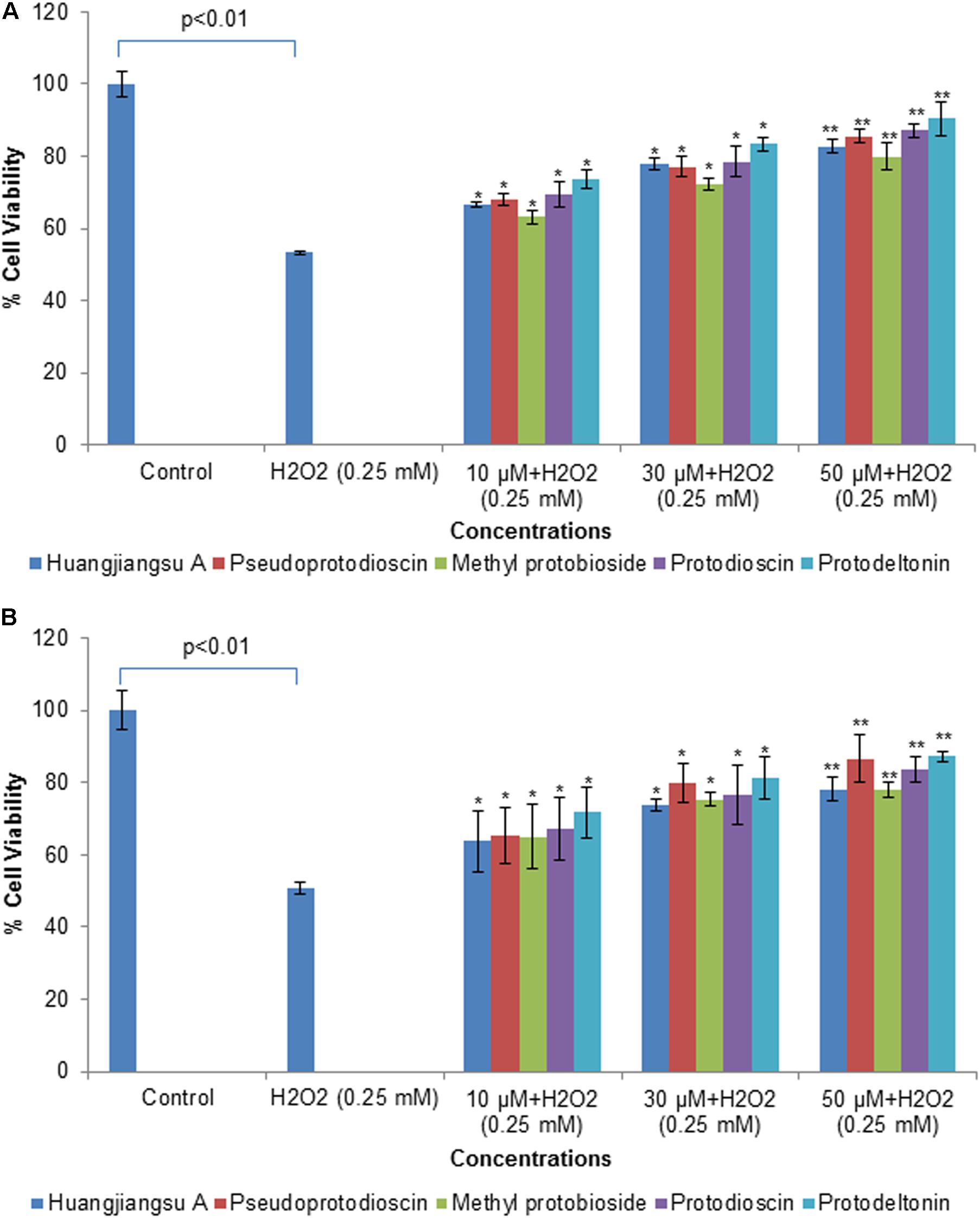
FIGURE 5. Assessment of protective potential of compounds on cell viability of HepG2 cells by (A) MTT assay and (B) NRU assay. Cells were exposed to 10, 30, and 50 μM of non-cytotoxic compounds for 24 h. Then the cells were exposed to H2O2 at 0.25 mM for 24 h. All values are given as mean ± SD. ∗p < 0.05, ∗∗p < 0.01 versus H2O2.
Morphological Changes
The morphological changes observed in HepG2 cells pre-exposed to compounds from D. villosa and then H2O2 for 24 h are summarized in Figure 6. As shown in the figure, H2O2 at 0.25 mM concentration induced characteristic morphological changes, such as cell shrinkage, appearance as rounded bodies and loss of adhesion capacity as compared to the control. However, HepG2 cells pre-exposed to 10, 30, and 50 μM concentrations of huangjiangsu A, pseudoprotodioscin, methyl protobioside, protodioscin, and protodeltonin restored their original morphology similar to the control in a concentration-dependent manner. The preservation of the normal morphology of HepG2 cells observed by phase contrast microscope indicated the preventive role of the compounds against H2O2-induced morphological damage.
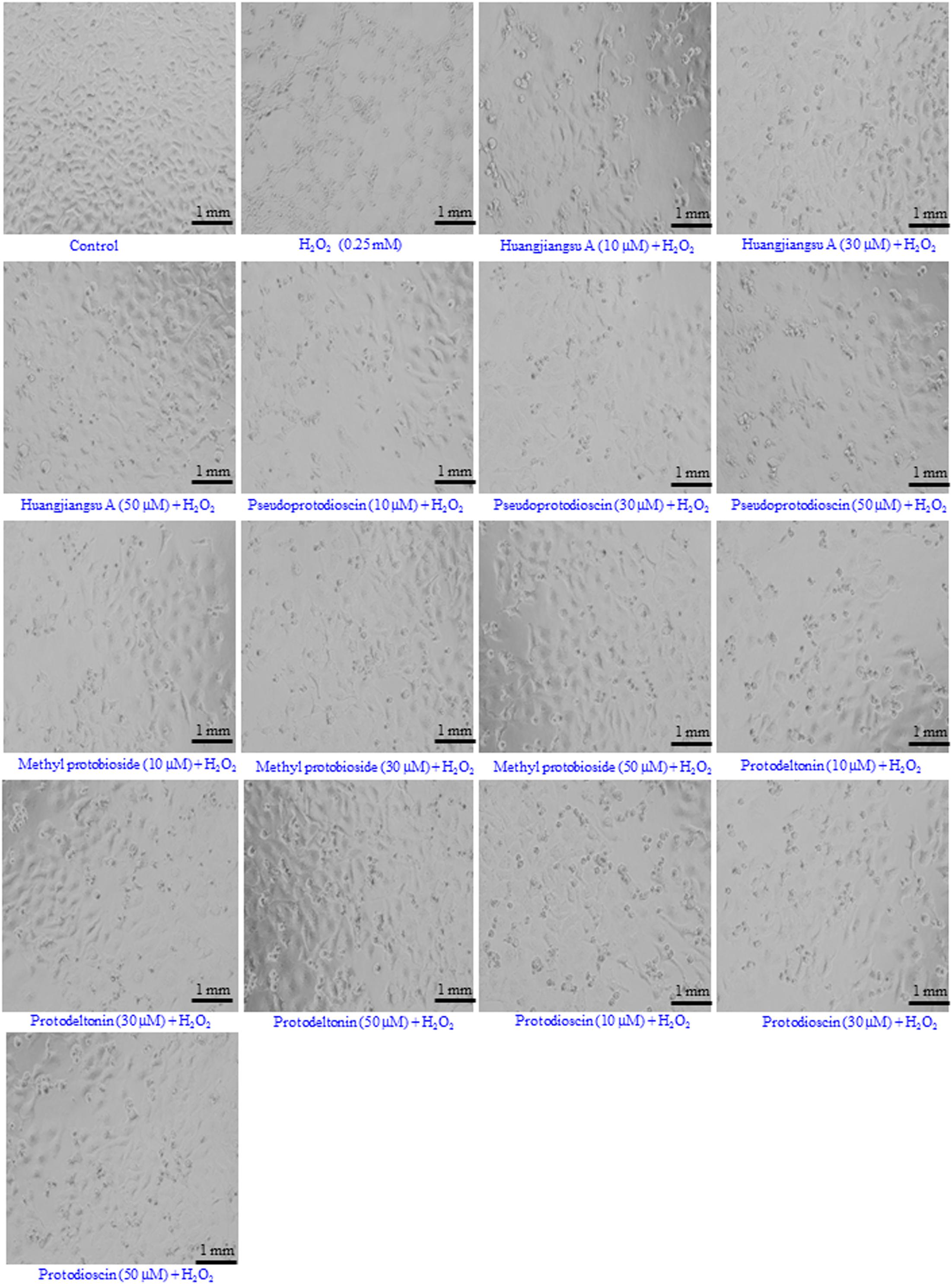
FIGURE 6. Morphological changes observed in HepG2 cells. Cells were pre-exposed with non-cytotoxic compounds for 24 h and then H2O2 (0.25 mM) for 24 h. Images were acquired using a phase contrast inverted microscope at 20× magnification. Each scale bar = 1 mm.
Protective Potential of Compounds Against H2O2 Reduced GSH Level
In order to evaluate the effects of compounds of D. villosa on antioxidant defense systems, the glutathione (GSH) level was measured in H2O2 exposed HepG2 cells. The results of this study showed that GSH level in H2O2 exposed HepG2 cells was significantly decreased by 43% (p < 0.01) as compared to the control (Figure 7). However, as shown in the figure, pre-exposure of cells to huangjiangsu A, pseudoprotodioscin, methyl protobioside, protodioscin, and protodeltonin at 50 μM concentration significantly increased the GSH level in H2O2 exposed HepG2 cells. The increase in GSH level was 15% by huangjiangsu A, 22% by pseudoprotodioscin, 16% by methyl protobioside, 27% by protodioscin, and 34% by protodeltonin at 50 μM (Figure 7). Our results showed that pre-exposure of cells to the test compounds effectively restored the GSH level. It is well known that exogenous exposure of H2O2 can increase the intracellular ROS generation and induced cellular oxidative damage in hepatic cells and it can be eliminated by hepatocyte antioxidant defense mechanisms (Xia et al., 2017). GSH is a main non-enzymatic antioxidant, which plays an important role in the cellular defense system against oxidative stress (Scharf et al., 2003). It has been reported that glutathione peroxidase catalyses GSH oxidation to GSSG at the expense of H2O2 and glutathione reductase recycles oxidized GSH back to reduced GSH (Al-Sheddi et al., 2016). Thus, it could be concluded that the pre-exposure of HepG2 cells to these compounds quenches the intracellular destructive peroxide and increases the level of glutathione thus enhancing the antioxidant status and protecting the cells against the H2O2-induced damage.
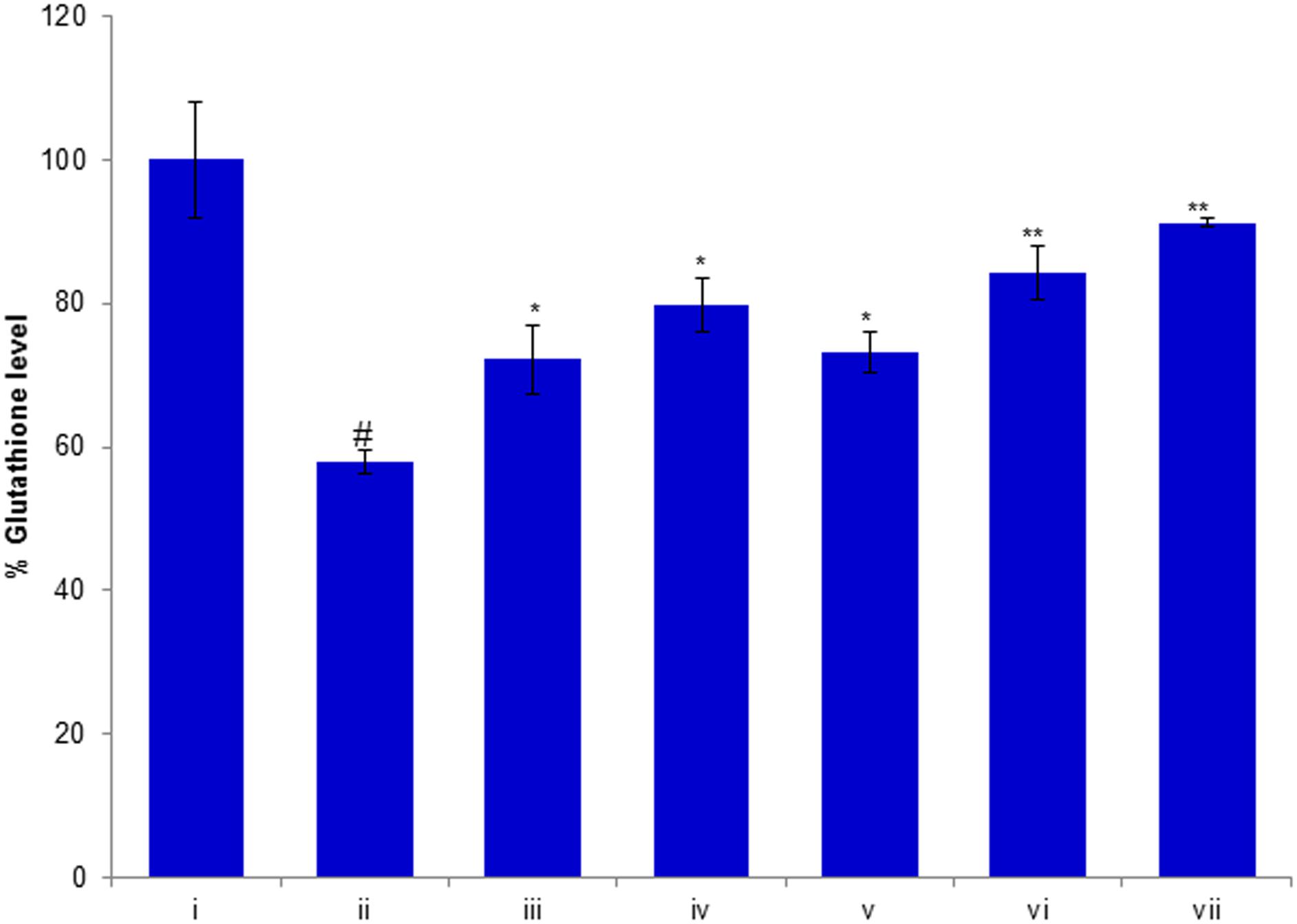
FIGURE 7. Protective potential of compounds isolated from Dioscorea villosa on depletion in glutathione level. HepG2 cells were exposed to 50 μM of non-cytotoxic compounds for 24 h prior to the addition of H2O2 for 24 h. All values are represented as mean ± SD. (i) Control; (ii) H2O2 (0.25 mM); (iii) Huangjiangsu A (50 μM) + H2O2 (0.25 mM); (iv) Pseudoprotodioscin (50 μM) + H2O2 (0.25 mM); (v) Methyl protobioside (50 μM) + H2O2 (0.25 mM); (vi) Protodioscin (50 μM) + H2O2 (0.25 mM); and (vii) Protodeltonin (50 μM) + H2O2 (0.25 mM). #p < 0.01 versus control, ∗p < 0.05 and ∗∗p < 0.01 versus H2O2 exposure.
Protective Potential of Compounds Against H2O2-Induced ROS Generation
The level of intracellular ROS generation in HepG2 cells was evaluated using a DCFH-DA fluorescent probe. The HepG2 cells were pre-exposed to 50 μM of compounds (huangjiangsu A, pseudoprotodioscin, methyl protobioside, protodioscin, and protodeltonin) for 24 h and then H2O2 for 24 h. The results showed that the DCF fluorescence intensity in H2O2-treated HepG2 cells was significantly increased to 104% as compared to the control group (Figure 8). However, the fluorescence intensity was notably reduced up to 42, 67, 79, 87, and 80% in HepG2 cells pre-exposed to 50 μM of huangjiangsu A, pseudoprotodioscin, methyl protobioside, protodeltonin, and protodioscin compounds, respectively (Figure 8). As shown in figure, compound huangjiangsu A showed the highest scavenging activity of intracellular ROS generation. It is reported that exogenous exposure of H2O2 resulted in accumulation of intracellular ROS generation, which plays an important role in cell differentiation, cell proliferation, and cell death (Park, 2013). ROS generation is well known to be associated with the principle of oxidative stress (Schieber and Chandel, 2014). Oxidative stress is the net result of an imbalance in the amount of ROS generation and destruction, which is involved in causation and consequences of many diseases (Aruoma, 1998; Rodriguez and Redman, 2005). It is also been reported that induction in ROS generation plays an important role in the hepatocellular damage (Jiang et al., 2014). Since H2O2-induced oxidative stress and ROS generation have been previously reported, we used H2O2 to induce ROS generation in HepG2 cells. In this study, an increase in the ROS generation indicated that H2O2 could cause oxidative stress in HepG2 cells. The decrease in the intracellular ROS generation in HepG2 pre-exposed to 50 μM showed the protective potential of various compounds against H2O2-induced ROS generation. Our results are also in accordance with the previous reports showing that the administration of compounds isolated from plant suppresses the level of ROS generation (Yang et al., 2015a).
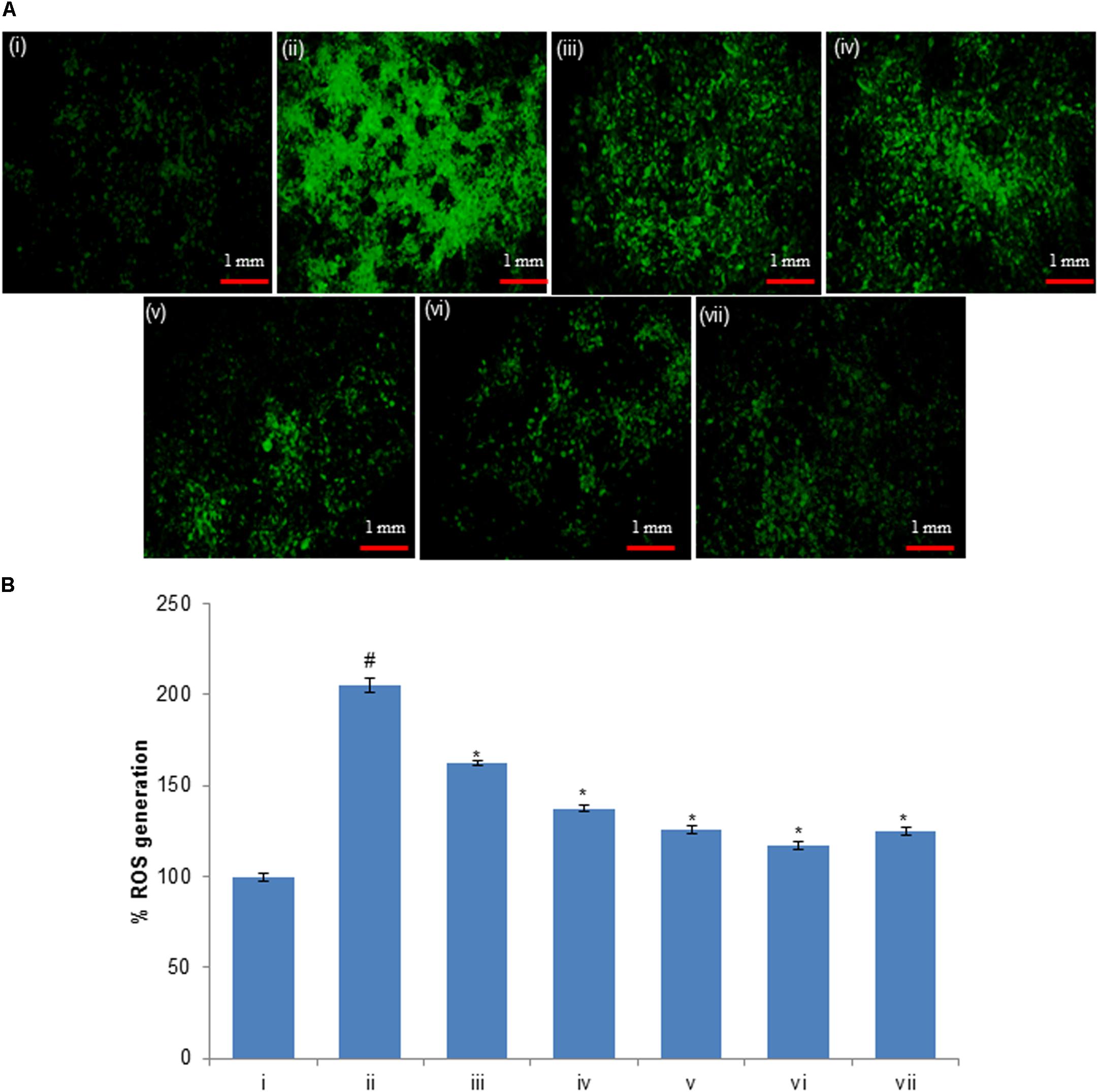
FIGURE 8. (A) H2O2-induced ROS generation and ameliorative effect of pre-treatment of compounds in HepG2 cells and (B) percent change in ROS generation. ROS generation was evaluated using dichlorodihydrofluorescein diacetate (DCFH-DA) dye. (i) Untreated control; (ii) H2O2 (0.25 mM); (iii) Huangjiangsu A (50 μM) + H2O2 (0.25 mM); (iv) Pseudoprotodioscin (50 μM) + H2O2 (0.25 mM); (v) Methyl protobioside (50 μM) + H2O2 (0.25 mM); (vi) Protodioscin (50 μM) + H2O2 (0.25 mM); and (vii) Protodeltonin (50 μM) + H2O2 (0.25 mM). #p < 0.01 versus control, ∗p < 0.05 versus H2O2 exposure. Each scale bar = 1 mm.
Conclusion
The present study demonstrated the cytotoxic and cyto-protective potential of selected steroidal glycosides, i.e., zingiberensis saponin I, dioscin, deltonin, progenin III, huangjiangsu A, pseudoprotodioscin, methyl protobioside, protodioscin, and protodeltonin, isolated from D. villosa. Furostane-type steroidal glycosides (huangjiangsu A, pseudoprotodioscin, methyl protobioside, protodioscin, and protodeltonin) were found to attenuate H2O2-induced cytotoxicity in HepG2 cells. Their protective effect seems to be mediated by inhibiting intracellular ROS generation and restoring the GSH level. Together, results from the present study suggest that the constituents of D. villosa can protect HepG2 cells against H2O2-induced oxidative damage through antioxidant activity.
Author Contributions
MS and IK designed the experiments. ZA, AC, and MS performed the experiments. ZA and MS analyzed the data and drafted the manuscript. IK and AC supervised the study. All the authors read and approved for the publication.
Conflict of Interest Statement
The authors declare that the research was conducted in the absence of any commercial or financial relationships that could be construed as a potential conflict of interest.
Acknowledgments
The authors would like to thank Twasol Research Excellence Program (TRE Program), King Saud University, Riyadh, Saudi Arabia for support.
References
Ali, Z., Smillie, T. J., and Khan, I. A. (2013a). 7-Oxodioscin, a new spirostan steroid glycoside from the rhizomes of Dioscorea nipponica. Nat. Prod. Commun. 8, 319–321.
Ali, Z., Smillie, T. J., and Khan, I. A. (2013b). Cholestane steroid glycosides from the rhizomes of Dioscorea villosa (wild yam). Carbohydr. Res. 370, 86–91. doi: 10.1016/j.carres.2012.12.022
Ali, Z., Smillie, T. J., and Khan, I. A. (2013c). Two spirostan steroid glycoside fatty esters from Dioscorea cayenensis. Nat. Prod. Commun. 8, 323–326.
Al-Sheddi, E. S., Farshori, N. N., Al-Oqail, M. M., Musarrat, J., Al-Khedhairy, A. A., and Siddiqui, M. A. (2016). Protective effect of Lepidium sativum seed extract against hydrogen peroxide-induced cytotoxicity and oxidative stress in human liver cells (HepG2). Pharm. Biol. 54, 314–321. doi: 10.3109/13880209.2015.1035795
Aruoma, O. I. (1998). Free radicals, oxidative stress, and antioxidants in human health and disease. J. Am. Oil Chem. Soc. 75, 199–212. doi: 10.1007/s11746-998-0032-9
Aumsuwan, P., Khan, S. I., Khan, I. A., Ali, Z., Avula, B., Walker, L. A., et al. (2016). The anticancer potential of steroidal saponin, dioscin, isolated from wild yam (Dioscorea villosa) root extract in invasive human breast cancer cell line MDA-MB-231 in vitro. Arch. Biochem. Biophys. 591, 98–110. doi: 10.1016/j.abb.2015.12.001
Aumsuwan, P., Khan, S. I., Khan, I. A., Avula, B., Walker, L. A., Helferich, W. G., et al. (2015). Evaluation of wild yam (Dioscorea villosa) root extract as a potential epigenetic agent in breast cancer cells. In Vitro Cell. Dev. Biol. Anim. 51, 59–71. doi: 10.1007/s11626-014-9807-5
Bechmann, L. P., Hannivoort, R. A., Gerken, G., Hotamisligil, G. S., Trauner, M., and Canbay, A. (2012). The interaction of hepatic lipid and glucose metabolism in liver diseases. J. Hepatol. 56, 952–964. doi: 10.1016/j.jhep.2011.08.025
Chandra, D., Ramana, K. V., Wang, L., Christensen, B. N., Bhatnagar, A., and Srivastava, S. K. (2002). Inhibition of fiber cell globulization and hyperglycemia-induced lens opacification by aminopeptidase inhibitor bestatin. Invest. Ophthalmol. Vis. Sci. 43, 2285–2292.
Chaudhari, G. M., and Mahajan, R. T. (2016). In vitro hepatoprotective activity of Terminalia arjuna stem bark and its flavonoids against CCl4 induced hepatotoxicity in goat liver slice culture. Asian J. Plant Sci. Res. 6, 10–17.
Cheong, C. U., Yeh, C. S., Hsieh, Y. W., Lee, Y. R., Lin, M. Y., Chen, C. Y., et al. (2016). Protective effects of costunolide against hydrogen peroxide-induced injury in PC12 cells. Molecules 21:E898. doi: 10.3390/molecules21070898
Gao, X., Li, C., Tang, Y. L., Zhang, H., and Chan, S. W. (2016). Effect of Hedyotis diffusa water extract on protecting human hepatocyte cells (LO2) from H2O2-induced cytotoxicity. Pharm. Biol. 54, 1148–1155. doi: 10.3109/13880209.2015.1056310
González, L. T., Minsky, N. W., Espinosa, L. E. M., Aranda, R. S., Meseguer, J. P., and Pérez, P. C. (2017). In vitro assessment of hepatoprotective agents against damage induced by acetaminophen and CCl4. BMC Complement. Altern. Med. 17:39. doi: 10.1186/s12906-016-1506-1
Haidry, M., and Malik, A. T. (2014). Hepatoprotective and antioxidative effects of Terminalia arjuna against cadmium provoked toxicity in albino rats (Rattus norvegicus). Biochem. Pharmacol. 3, 1–4. doi: 10.4172/2167-0501.1000130
Hayes, P. Y., Lambert, L. K., Lehmann, R., Penman, K., Kitchin, W., and De Voss, J. J. (2007). Complete 1H and 13C assignments of the four major saponins from Dioscorea villosa (wild yam). Magn. Reson. Chem. 45, 1001–1005. doi: 10.1002/mrc.2071
Hong, M., Li, S., Tan, H. Y., Wang, N., Tsao, S. W., and Feng, Y. (2015). Current status of herbal medicines in chronic liver disease therapy: the biological effects, molecular targets and future prospects. Int. J. Mol. Sci. 16, 28705–28745. doi: 10.3390/ijms161226126
Jiang, J., Yu, S., Jiang, Z., Liang, C., Yu, W., Li, J., et al. (2014). N-acetyl-serotonin protects HepG2 cells from oxidative stress injury induced by hydrogen peroxide. Oxid. Med. Cell. Longev. 2014:310504. doi: 10.1155/2014/310504
Jiménez-Arellanes, M. A., Gutiérrez-Rebolledo, G. A., Meckes-Fischer, M., and León-Díaz, R. (2016). Medical plant extracts and natural compounds with a hepatoprotective effect against damage caused by antitubercular drugs: a review. Asian Pac. J. Trop. Med. 9, 1141–1149. doi: 10.1016/j.apjtm.2016.10.010
Kim, E. A., Jang, J. H., Lee, Y. H., Sung, E. G., Song, I. H., Kim, J. Y., et al. (2014). Dioscin induces caspase-independent apoptosis through activation of apoptosis-inducing factor in breast cancer cells. Apoptosis 19, 1165–1175. doi: 10.1007/s10495-014-0994-z
Kim, J. H., Kim, D., Kim, J., and Hwang, J. K. (2011). Euchresta horsfieldii Benn. activates peroxisome proliferatoractivated receptor a and regulates expression of genes involved in fatty acid metabolism in human HepG2 cells. J. Ethnopharmacol. 133, 244–247. doi: 10.1016/j.jep.2010.09.029
Komesaroff, P. A., Black, C. V. S., Cable, V., and Sudhir, K. (2001). Effects of wild yam extract on menopausal symptoms, lipids and sex hormones in healthy menopausal women. Climacteric 4, 144–150. doi: 10.1080/cmt.4.2.144.150
Li, S., Tan, H. Y., Wang, N., Zhang, Z. J., Lao, L., Wong, C. W., et al. (2015). The role of oxidative stress and antioxidants in liver diseases. Int. J. Mol. Sci. 16, 26087–26124. doi: 10.3390/ijms161125942
McAnuff, M. A., Harding, W. W., Omoruyi, F. O., Jacobs, H., Morrison, F. Y., and Asemota, H. N. (2005). Hypoglycemic effects of steroidal sapogenins isolated from Jamaican bitter yam, Dioscorea polygonoides. Food Chem. Toxicol. 43, 1667–1672. doi: 10.1016/j.fct.2005.05.008
Munafo, J. P., Ramanathan, A., Jimenez, L. S., and Gianfagna, T. J. (2010). Isolation and structural determination of steroidal glycosides from the bulbs of Easter lily (Lilium longiflorum Thunb.). J. Agric. Food Chem. 58, 8806–8813. doi: 10.1021/jf101410d
Park, W. H. (2013). The effects of exogenous H2O2 on cell death, reactive oxygen species and glutathione levels in calf pulmonary artery and human umbilical vein endothelial cells. Int. J. Mol. Med. 31, 471–476. doi: 10.3892/ijmm.2012.1215
Pereira, C., Barros, L., and Ferreira, I. C. (2016). Extraction, identification, fractionation and isolation of phenolic compounds in plants with hepatoprotective effects. J. Sci. Food Agric. 96, 1068–1084. doi: 10.1002/jsfa.7446
Ray, P. D., Huang, B. W., and Tsuji, Y. (2012). Reactive oxygen species (ROS) homeostasis and redox regulation in cellular signaling. Cell. Signal. 24, 981–990. doi: 10.1016/j.cellsig.2012.01.008
Rodriguez, R., and Redman, R. (2005). Balancing the generation and elimination of reactive oxygen species. Proc. Natl. Acad. Sci. U.S.A. 102, 3175–3176. doi: 10.1073/pnas.0500367102
Sattayasai, J., Chaonapan, P. T., Arkaravichie, R., Soi-ampornkul, S., Junnu, P., Charoensilp, J., et al. (2013). Protective effects of Mangosteen extract on H2O2-induced cytotoxicity in SK-NSH cells and scopolamine-induced memory impairment in mice. PLoS One 8:e85053. doi: 10.1371/journal.pone.0085053
Scharf, G., Prustomersky, S., Knasmuller, S., Schulte-Hermann, R., and Huber, W. W. (2003). Enhancement of glutathione and glutamylcysteine synthetase, the rate limiting enzyme of glutathione synthesis, by chemoprotective plant-derived food and beverage components in the human hepatoma cell line HepG2. Nutr. Cancer 45, 74–83. doi: 10.1207/S15327914NC4501_9
Schieber, M., and Chandel, N. S. (2014). ROS function in redox signaling and oxidative stress. Curr. Biol. 24, 453–462. doi: 10.1016/j.cub.2014.03.034
Shao, Y. U., Poobrasert, O., Kennelly, E. J., Chin, C. K., Ho, C. T., Huang, M. T., et al. (1997). Steroidal saponins from Asparagus officinalis and their cytotoxic activity. Planta Med. 63, 258–262. doi: 10.1055/s-2006-957667
Sharma, S. K., Arogya, S. M., Bhaskarmurthy, D. H., Agarwal, A., and Velusami, C. C. (2011). Hepatoprotective activity of the Phyllanthus species on tert-butyl hydroperoxide (t-BH)-induced cytotoxicity in HepG2 cells. Pharmacogn. Mag. 7, 229–233. doi: 10.4103/0973-1296.84237
Shen, P., Wang, S. L., Liu, X. K., Yang, C. R., Cai, B., and Yao, X. S. (2002). A new steroidal saponin from Dioscorea deltoidea wall var. orbiculata. Chin. Chem. Lett. 13, 851–854.
Siddiqui, M. A., Ahmad, J., Farshori, N. N., Saquib, Q., Jahan, S., Kashyap, M. P., et al. (2013). Rotenone-induced oxidative stress and apoptosis in human liver HepG2 cells. Mol. Cell Biochem. 384, 59–69. doi: 10.1007/s11010-013-1781-9
Siddiqui, M. A., Singh, G., Kashyap, M. P., Khanna, V. K., Yadav, S., Chandra, D., et al. (2008). Influence of cytotoxic doses of 4-hydroxynonenal on selected neurotransmitter receptors in PC-12 cells. Toxicol. In Vitro 22, 1681–1688. doi: 10.1016/j.tiv.2008.07.001
Silva, B. P., Bernardo, R. R., and Parente, J. P. (1998). New furostanol glycosides from Costus spicatus. Phytochemistry 69, 528–532.
Simeonova, R., Kondeva-Burdina, M., Vitcheva, V., and Mitcheva, M. (2014). Some in vitro/in vivo chemically-induced experimental models of liver oxidative stress in rats. BioMed Res. Int. 2014:706302. doi: 10.1155/2014/706302
Videla, L. A. (2009). Oxidative stress signaling underlying liver disease and hepatoprotective mechanisms. World J. Hepatol. 1, 72–78. doi: 10.4254/wjh.v1.i1.72
Wang, J., Zhao, Y. M., Zhang, B., and Guo, C. Y. (2015). Protective effect of total phenolic compounds from Inula helenium on hydrogen peroxide-induced oxidative stress in SH-SY5Y cells. Indian J. Pharm. Sci. 77, 163–169. doi: 10.4103/0250-474X.156553
Xia, T., Yao, J., Zhang, J., Zheng, Y., Song, J., and Wang, M. (2017). Protective effects of Shanxi aged vinegar against hydrogen peroxide-induced oxidative damage in LO2 cells through Nrf2-mediated antioxidant responses. RSC Adv. 7, 17377–17386. doi: 10.1039/C6RA27789F
Xu, T., Zhang, S., Zheng, L., Yin, L., Xu, L., and Peng, P. A. (2012). 90-day subchronic toxicological assessment of dioscin, a natural steroid saponin, in Sprague-Dawley rats. Food Chem. Toxicol. 50, 1279–1287. doi: 10.1016/j.fct.2012.02.027
Yang, J., Kwon, Y. S., and Kim, M. J. (2015a). Isolation and characterization of bioactive compounds from Lepisorus thunbergianus (Kaulf.). Arab. J. Chem. 8, 407–413. doi: 10.1016/j.arabjc.2014.11.056
Yang, D., Liu, X., Liu, M., Chi, H., Liu, J., and Han, H. (2015b). Protective effects of quercetin and taraxasterol against H2O2-induced human umbilical vein endothelial cell injury in vitro. Exp. Ther. Med. 10, 1253–1260. doi: 10.3892/etm.2015.2713
Keywords: Dioscorea villosa, Dioscoreaceae, steroidal glycosides, H2O2, cytotoxicity, ROS generation
Citation: Siddiqui MA, Ali Z, Chittiboyina AG and Khan IA (2018) Hepatoprotective Effect of Steroidal Glycosides From Dioscorea villosa on Hydrogen Peroxide-Induced Hepatotoxicity in HepG2 Cells. Front. Pharmacol. 9:797. doi: 10.3389/fphar.2018.00797
Received: 04 April 2018; Accepted: 02 July 2018;
Published: 23 July 2018.
Edited by:
Thomas Efferth, Johannes Gutenberg-Universität Mainz, GermanyReviewed by:
Luísa Custódio, Centro de Ciências do Mar (CCMAR), PortugalFunda Nuray Yalcin, Hacettepe University, Turkey
Copyright © 2018 Siddiqui, Ali, Chittiboyina and Khan. This is an open-access article distributed under the terms of the Creative Commons Attribution License (CC BY). The use, distribution or reproduction in other forums is permitted, provided the original author(s) and the copyright owner(s) are credited and that the original publication in this journal is cited, in accordance with accepted academic practice. No use, distribution or reproduction is permitted which does not comply with these terms.
*Correspondence: Maqsood A. Siddiqui, maqsoodahmads@gmail.com