- 1Laboratory of Medical and Biological Research, Institute of General and Experimental Biology, Siberian Division, Russian Academy of Science, Ulan-Ude, Russia
- 2Department of Biochemistry and Biotechnology, North-Eastern Federal University, Yakutsk, Russia
- 3Department of Studying the Mechanisms of Adaptation, Scientific Center of Complex Medical Sciences, Yakutsk, Russia
- 4Department of Biological Sciences, Pusan National University, Busan, South Korea
- 5Regulatory and Medical Scientific Affairs, Padma AG, Hinwil, Switzerland
Artemisia genus of Asteraceae family is a source of medicinal plants known worldwide and used as ethnopharmacological remedies for the treatment of diabetes in Northern Asia (Siberia). The aim of this study was to determine the phenolic profile of 12 Siberian Artemisia species (A. anethifolia, A. commutata, A. desertorum, A. integrifolia, A. latifolia, A. leucophylla, A. macrocephala, A. messerschmidtiana, A. palustris, A. sericea, A. tanacetifolia, A. umbrosa) and to test the efficacy of plant extracts and pure compounds for antidiabetic potential. Finally, by HPLC-DAD-ESI-TQ-MS/MS technique, 112 individual phenolic compounds were detected in Artemisia extracts in a wide range of concentrations. Some species accumulated rare plant phenolics, such as coumarin-hemiterpene ethers (lacarol derivatives) from A. latifolia and A. tanacetifolia; melilotoside from A. tanacetifolia; dihydrochalcones (davidigenin analogs) from A. palustris; chrysoeriol glucosides from A. anethifolia, A. sericea, and A. umbrosa; eriodictyol glycosides from A. messerschmidtiana; and some uncommon flavones and flavonols. The predominant phenolic group from Artemisia species herb was caffeoylquinic acid (CQAs), and in all species, the major CQAs were 5-O-CQA (20.28–127.99 μg/g) and 3,5-di-O-CQA (7.35–243.61 μg/g). In a series of in vitro bioassays, all studied Artemisia extracts showed inhibitory activity against principal enzymes of carbohydrate metabolism, such as α-amylase (IC50 = 150.24–384.14 μg/mL) and α-glucosidase (IC50 = 214.42–754.12 μg/mL). Although many phenolic compounds can be inhibitors, experimental evidence suggests that the CQAs were key to the biological response of Artemisia extracts. Mono-, di- and tri-substituted CQAs were assayed and showed inhibition of α-amylase and α-glucosidase, with IC50 values of 40.57–172.47 μM and 61.08–1240.35 μM, respectively, and they were more effective than acarbose, a well-known enzyme inhibitor. The results obtained in this study reveal that Siberian Artemisia species and CQAs possess a pronounced inhibitory activity against α-amylase and α-glucosidase and could become a complement to synthetic antidiabetic drugs for controlling blood glucose level.
Introduction
Ethnopharmacology constitutes the scientific basis for the development of active therapeutics based on traditional medicines of various ethnic groups. In recent years, the preservation of local knowledge, the promotion of indigenous medical systems in primary health care and the conservation of biodiversity have become more of a concern to all scientists working at the interface of social and natural sciences. A wide range of innovations in phytochemical analysis allowed an ever-faster analysis and isolation of bioactive natural products (Kumar and Pandey, 2013; Tǎnase et al., 2018) and their identification/structure elucidation (Heinrich, 2010). Novel treatment strategies are needed for all diseases, and recently herbal medicines from such traditions have received attention in the area of prevention or treatment of chronic metabolic disorders, such as diabetes mellitus. Many plant species of various families have been noted for their antidiabetic potential. Among them were known plant extracts that inhibited carbohydrate hydrolysis enzymes, such as α-amylase (Etxeberria et al., 2012; Xiao et al., 2013b) and α-glucosidase (Xiao et al., 2013a), which play a key role in carbohydrate digestion (Yin et al., 2014). The inhibition of these enzymes is one of the possible ways to avert diabetes.
Asteraceae (Compositae) is one of the largest and widespread families of plants, with about 33,000 accepted species. The significance of the Asteraceae family for the curative aims has been described over the centuries. Due to the availability of a large variety of species in this family, it is important in ethnopharmacological medicine throughout the world. Artemisia is a large, diverse genus of plants with more than 480 species belonging to Asteraceae. Recent years have witnessed a widespread increase of interest in research of Artemisia phytocomponents with antimalarial, cytotoxic, antihepatotoxic, antibacterial and antioxidant activity. (Tan et al., 1998; Bora and Sharma, 2011; Abad et al., 2012; Ivanescu et al., 2015; Pandey and Singh, 2017). It was observed that the various Artemisia aqueous and alcoholic extracts possessed an antidiabetic effect caused by hypoglycaemic action (Dabe and Kefale, 2017). Pharmacological data provides convincing evidence that the extracts of A. absinthium (Daradka and Abas, 2014), A. afra (Afolayan and Sunmonu, 2011), A. amygdalina (Ghazanfar et al., 2014), A. dracundulus (Ribnicky et al., 2006), A. judaica (Nofal et al., 2009), A. herba-alba (Awad et al., 2012), A. ludoviciana (Anaya-Eugenio et al., 2014), and A. sphaerocephala (Xing et al., 2009) were effective in streptozotocin- and alloxan-induced hyperglycemia experimental animal models due to their significant ability to reduce blood glucose level and protect against metabolic aberrations caused by diabetes. The only reported application of Artemisia drugs in humans was conducted on type II diabetic individuals and used A. absinthium capsules for 30 days (Li et al., 2015). As a result, it was observed that blood glucose level was reduced by 32% compared with the baseline value. This suggests that other Artemisia plants may have anti-diabetic properties.
As shown previously by our team, the plants growing at extreme natural habitats, such as Northern Asia or Siberia, are able to accumulate the phenolic components with antidiabetic potential (Olennikov and Kashchenko, 2014, 2015, 2017; Olennikov et al., 2015a, 2017a,b,c; Kashchenko et al., 2017a,b). It is evident that Siberian species of Artemisia genus need to be included in our continuing search for the best antidiabetic agents of plant origin.
Analyzing ethnopharmacological data, at least 12 species of the Artemisia genus were noted as used by nomads of Northern Asia or Siberia, such as the ancient Buryat (major northern subgroup of the Mongols) or Yakuts (the largest indigenous Turkic group in Siberia). Doctors of nomads (lamas, healers) applied Artemisia plants alone or as components of complex remedies for the prophylaxis and treatment of disorders related to diabetes. These plants were known as mkhan pa (A. commutata, A. integrifolia, A. leucophylla, A. sericea), phur nag or phur mong (A. anethifolia), tshar bong (A. desertorum, A. latifolia, A. macrocephala, A. messerschmidtiana, A. tanacetifolia, A. umbrosa), and yog ma (A. palustris) (Aseeva et al., 2008; Prajnya, 2008; Batorova et al., 2013; Puntsog, 2017; Figure 1).
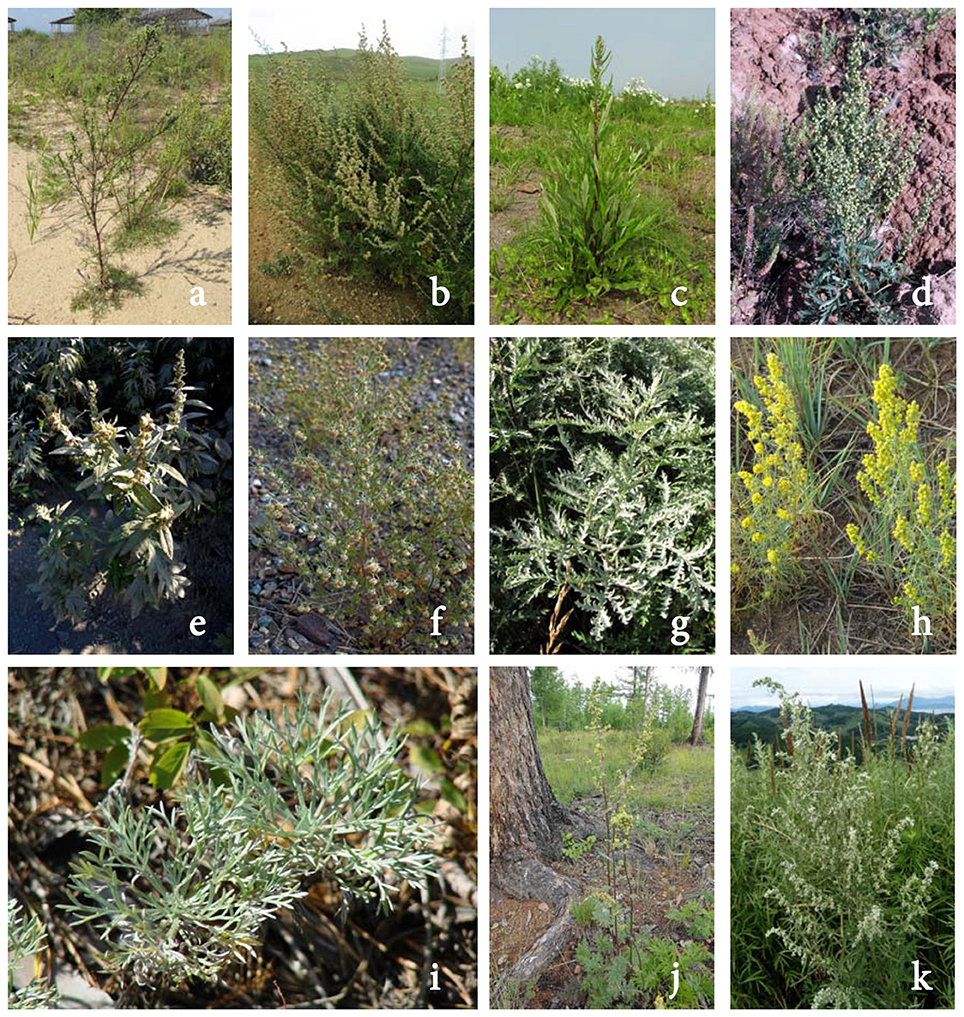
Figure 1. Artemisia species mentioned in the present research: A. commutata (a) A. desertorum (b) A. integrifolia (c) A. latifolia (d) A. leucophylla (e) A. macrocephala (f) A. messerschmidtiana (g) A. palustris (h) A. sericea (i) A. tanacetifolia (j) A. umbrosa (k).
Even so, none of the mentioned species have been previously examined for their antidiabetic potential. Lastly, with regard to chemical profile of Siberian artemisias, the known data had been very limited. Some terpenoids were detected in A. anethifolia (Evstratova and Sinyukhin, 1971; Bakhshyeva et al., 2011); essential oil (Zhu et al., 2013) and flavonoid aglycones (Wang J.-L. et al., 2016) in A. integrifolia; sesquiterpenes in A. latifolia (Adekenov et al., 1984); essential oil (Shoaib et al., 2015) and sesquiterpenes (Shoaib et al., 2017) in A. macrocephala; flavonoids in A. palustris (Chemesova et al., 1978); coumarins in A. tanacetifolia (Szabó et al., 1985); and sesquiterpenes (Tashenov et al., 2013) and essential oil (Suleimen et al., 2014) in A. umbrosa. There is no chemical information about A. commutata, A. desertorum, A. leucophylla, A. messerschmidtiana, and A. sericea. Overall, the phenolic components of the named species remain a largely unexplored group of Artemisia constituents.
As a part of our ongoing research of antidiabetic plant constituents, the present work aimed to conduct chromato-mass-spectrometric profiling of 12 Siberian Artemisia species, traditionally used in Siberia as antidiabetic herbs, with high performance liquid chromatography with diode array and electrospray triple quadrupole mass-spectrometric detection (HPLC-DAD-ESI-TQ-MS/MS), as well as analysis of plant extracts and pure components for their inhibitory activity against digestive enzymes (α-amylase, α-glucosidase). This multidisciplinary approach is an essential basis for the advancement of bioactive compounds of the Artemisia genus for use in tomorrow's medicines.
Materials and Methods
Chemicals
The following chemicals were purchased from Carbosynth Limited (Berkshire, UK): 3-O-coumaroylquinic acid (Cat. No. FC71593, ≥95%); 6-O-feruloylglucose (Cat. No. MF11960, ≥95%); isorhamnetin-3-O-galactoside (Cat. No. MI106502, ≥95%); luteolin-6,8-di-C-glucoside (lucenin-2; Cat. No. FL157201, ≥95%); nepetin (Cat. No. FE146185, ≥95%); scutellarein-7-O-glucoside (Cat. No. FS65327, ≥95%); ChemFaces (Wuhan, China): apigenin-6-C-glucoside-4″-O-glucoside (isosaponarin, Cat. No. CFN90133, ≥98%), apigenin-6-C-glucoside-7-O-glucoside (saponarin, Cat. No. CFN90134; ≥98%); apigenin-6-C-arabinoside-8-C-glucoside (isoschaftoside; Cat. No. CFN92029; ≥98%); apigenin-6,8-di-C-glucoside (vicenin-2; Cat. No. CFN92031, ≥98%); apigenin-6-C-glucoside-8-C-arabinoside (schaftoside; Cat. No. CFN90197, ≥98%); chrysoeriol-7-O-glucoside (Cat. No. CFN93021, ≥98%); genkwanin (Cat. No. CFN98670, ≥98%); chrysosplenetin (Cat. No. CFN97026, ≥98%); isorhamnetin-3-O-glucoside (Cat. No. CFN99757, ≥98%); luteolin-7-O-glucuronide (Cat. No. CFN98512, ≥98%); hispidulin (Cat. No. CFN99491, ≥98%); hispidulin-7-O-glucoside homoplantaginin; Cat. No. CFN90344, ≥98%); jaceosidin (Cat. No. CFN90386, ≥98%); kaempferol (Cat. No. CFN98838, ≥98%); nepetin-7-O-glucoside (nepetrin; Cat. No. CFN90438, ≥98%); quercetin-3-O-rutinoside (rutin; Cat. No. CFN99642, ≥98%); scopoletin-7-O-glucoside (scopolin; Cat. No. 531-44-2, ≥98%); 2,4,4′-trihydroxydihydrochalcone (Cat. No. CFN89071, ≥98%), quercetin-3-O-galactoside (hyperoside; Cat. No. CFN98754, ≥98%); velutin (Cat. No. CFN98290, ≥98%); sinensetin (Cat. No. CFN99599, ≥98%); Extrasynthese (Lyon, France): apigenin (Cat. No. 1102 S, ≥99%); chrysin (Cat. No. 1362 S, ≥99%); chrysoeriol (Cat. No. 1104 S, ≥ 99%); isorhamnetin-3-O-rutinoside (narcissin; Cat. No. 1333 S, ≥99%); luteolin (Cat. No. 1125 S, ≥99%); luteolin-7-O-glucoside (cynaroside; Cat. No. 1126 S, ≥98%); scutellarein (Cat. No. 1334 S, ≥99%); Phytopanaceya (Moscow, Russia): chrysoeriol-6,8-di-C-glucoside (stellarin-2; Cat. No. 1862, ≥94%), chrysoeriol-7-O-glucuronide (Cat. No. 0641, ≥95%); Sigma-Aldrich (St. Louis, MO, USA): acarbose (Cat. No. A8980, ≥95%); acetonitrile for HPLC (Cat. No. 34851, ≥99.9%); aluminum chloride (Cat. No. 206911, ≥98%); 4-aminoantipyrine (Cat. No. A4382); α-amylase from Aspergillus oryzae (Cat. No. 10065); apigenin-6-C-glucoside (isovitexin; Cat. No. 17804, ≥98%); apigenin-7-O-glucoside (cosmosiin; Cat. No. 44692, ≥97%); bovine serum albumin (Cat. No. 05470); 4-O-caffeoylquinic acid (Cat. No. 65969, ≥98%); 5-O-caffeoylquinic acid (neochlorogenic acid; Cat. No. 94419, ≥98%); 1,3-di-O-caffeoylquinic acid (Cat. No. D8196, ≥98%); 3,4-di-O-caffeoylquinic acid (Cat. No. SMB00224, ≥90%); 3,5-di-O-caffeoylquinic acid (Cat. No. SMB00131, ≥95%); 1,5-di-O-caffeoylquinic acid (Cat. No. 16917, ≥98%); 4,5-di-O-caffeoylquinic acid (Cat. No. SMB00221, ≥85%); cirsiliol (Cat. No. SML0953, ≥95%); dibasic potassium phosphate (Cat. No. 1551128); sodium phosphate dibasic (Cat. No. 795410); eupatilin (Cat. No. SML1689, ≥98%); dimethyl sulfoxide (Cat. No. D1435, ≥99.5%); eupatorin (Cat. No. E4660, ≥97%); eriodictyol (Cat. No. 74565, ≥95%); esculetin (Cat. No. 68923, ≥97.5%); α-glucosidase from Saccharomyces cerevisiae (Cat. No. G5003); glucose oxidase from Aspergillus niger (Cat. No. G7141); kaempferol-3-O-rutinoside (nicotiflorin; Cat. No. 90242, ≥98%); isorhamnetin (Cat. No. 17794, ≥95%); kaempferol-3-O-glucoside (astragalin; Cat. No. 68437, ≥90%); luteolin-6-C-glucoside (isoorientin; Cat. No. 02187, ≥98%); melilotoside (Cat. No. SMB00121, ≥95%); methanol (Cat. No. 34860, ≥99%); monobasic potassium phosphate (Cat. No. 1551139); 4-nitrophenyl α-D-glucopyranoside (Cat. No. N1377, ≥99%); peroxidase from horseradish (Cat. No. 77332); phenol (Cat. No. P5566, ≥99.5%); quercetin (Cat. No. Q4951, ≥95%); sodium carbonate anhydrous (Cat. No. 791768, ≥99.5%); umbelliferone-7-O-glucoside (skimmin; Cat. No. SMB00511, ≥90%); quercetin-3-O-glucoside (isoquercitrin; Cat. No. 16654, ≥98%); sakuranetin (Cat. No. 73422, ≥98%); starch (Cat. No. 03967); water deionized (Cat. No. 38796). Quercetin-3-O-(2″-rhamnosyl-)glucoside (calendoflavobioside, ≥95%), quercetin-3-O-(4″-rhamnosyl-)glucoside (≥95%), and scopoletin-7-O-neohesperidoside were isolated previously from Calendula officinalis (Olennikov and Kashchenko, 2013; Olennikov et al., 2017c); 3′-hydroxygenkwanin (≥92%), 5,3′-hydroxy-7,4′-dimetoxyflavone (≥90%), 5-desmethylnobiletin (≥95%), 5-desmethylsinensetin (≥94%) form Ziziphora pamiroalaica (Olennikov and Akobirshoeva, 2016); luteolin-6-C-glucoside-7-O-glucoside (≥94%), luteolin-6-C-glucoside-8-C-xyloside (lucenin-3, ≥92%), luteolin-6-C-xyloside-8-C-glucoside (lucenin-1, ≥90%) from Gastrolychnis tristis (Olennikov, 2018), 6-hydroxyluteolin (≥94%) from Scutellaria scordiifolia (Olennikov and Chirikova, 2013).
Plant Material
The samples of Artemisia species were collected in the appropriate flowering period in the Siberian Regions (Russian Federation—Buryatia Republic (BR), Sakha (Yakutia) Republic (SR): Artemisia anethifolia Weber ex Stechm.—Yakutsk (Yakutskii region, SR, 23.VII.2017, 62°2′9.6568″ N, 129°41′4.1522″ E, voucher specimen No. AAs/ae-07/14-27/0717); A. commutata Besser – Kyren (Tunkinskii district, BR, 25.VII.2017, 51°41′15.4501″ N, 102°8′21.8127″ E, voucher specimen No. AAs/ae-07/16-28/0717); A. desertorum Spreng. – Verkhnevilyuysk (Verkhnevilyuysky district, SR, 01.VIII.2017, 63°26′41.3387″ N, 120°19′26.7261″ E, voucher specimen No. AAs/ae-07/11-27/0817); A. integrifolia Richards. – Berdigestyakh (Gorny district, SR, 24.VII.2018, 62°5′50.2118″ N, 126°41′3.3254″ E, voucher specimen No. AAs/ae-07/18-27/0717); A. latifolia Ledeb. – Kyakhta (Kyakhtinsky district, BR, 02.VIII.17, 50°20′58.8231″ N, 106°28′56.9459″ E, voucher specimen No. AAs/ae-07/13-28/0817); A. leucophylla (Turcz. ex Besser) Turcz. ex C.B.Clarke – Ust-Barguzin (Barguzinsky district, BR, 03.VIII.2017, 53°24′58.4813″ N, 109°1′45.0859″ E, voucher specimen No. AAs/ae-07/21-28/0817); A. macrocephala Jacquem. ex Besser – Mirny (Mirninsky district, SR, 02.VIII.17, 62°32′23.1008″ N, 113°57′16.7372″ E, voucher specimen No. AAs/ae-07/25-27/0817); A. messerschmidtiana Besser – Khandyga (Tomponsky district, SR, 30.07.2017, 62°39′36.7192″ N, 135°33′38.1657″ E, voucher specimen No. AAs/ae-07/12-27/0717); A. palustris L. – Babushkin (Kabansky district, BR, 21.VII.2018, 51°42′57.7529″ N, 105°52′39.3969″ E, voucher specimen No. AAs/ae-07/09-28/0717); A. sericea Weber ex Stechm. – Churapcha (Churapchinsky district, SR, 20.VII.2017, 62°0′17.6204″ N, 132°25′30.2125″ E, voucher specimen AAs/ae-07/31-27/0717); A. tanacetifolia L. – Kurumkan (Kurumkansky district, BR, 02.VIII.17, 54°19′17.4945″ N, 110°20′20.2181″ E, voucher specimen No. AAs/ae-07/22-2800817); A. umbrosa (Besser) Turcz. – Zhigansk (Zhigansky district, SR, 18.VII.2017, 66°45′11.5770″ N, 123°23′52.7074″ E, voucher specimen No. AAs/ae-07/32-27/0717). Prof. T.A. Aseeva (IGEB SB RAS, Ulan-Ude) determined the species. The samples of Artemisia species were dried in a convective drying oven UT-4610 (Ulab, Sankt-Petersburg, Russia) at 40°C (20–24 hs) up to the humidity level 9–12% and stored at 4 °C in the Institute of General and Experimental Biology Plant Repository. The samples were grounded in an analytical mill A11 basic (IKA®-WerkeGmbH & Co.KG, Staufen, Germany) and then sieved using sieving machine ERL-M1 (Zernotekhnika, Moscow, Russia) up to an average particle diameter of 0.5 mm.
Total Extract Preparation
For preparation of the total extract accurately-weighed dried and grounded sample of Artemisia herb (100 g) was transferred to a conical glass flask (2 L). After that, 1.5 L of 60% ethanol solution was added with stirring and put in an ultrasonic bath. The extraction conditions were 90 min at 45°C, ultrasound power of 100 W, the frequency 35 kHz. The extraction was repeated three times. The obtained extracts were filtered through a cellulose filter and combined. The filtrates were evaporated in vacuo at 50°C until dryness with the use of a rotary evaporator. The total extracts were stored at 4°C until further chemical composition analysis and bioactivity assays.
SPE Fractionation of Artemisia Extracts
The sample of milled Artemisia extract (10 g) was dissolved in 20 mL of 70% ethanol, and then added to 100 mL of distilled water and the mixture was filtered under reduced pressure. Polyamide column (15 g) was prepared: primed with 400 mL methanol followed by 800 mL tridistilled water (td-water). An aliquot (100 mL) of Artemisia extract was loaded on polyamide column. Sequential elution was done with 300 mL of td-water (dephenolized fraction) and 500 mL of 70% ethanol (flavonoid-enriched fraction). Fractions were concentrated to dryness under reduced pressure and stored at 4°C until further chemical composition analysis and bioactivity assays.
Chemical Composition Assays
The total flavonoid content was estimated as rutin equivalents after spectrophotometric procedure after 5% AlCl3 addition (Chirikova et al., 2010). The total caffeoylquinic acid content was determined by the colorimetric Arnow method using 3-O-caffeoylquinic acid as the standard (Olennikov et al., 2011).
Enzyme Inhibition Assays
The α-glucosidase inhibition assay was performed using spectrophotometric method (Olennikov et al., 2017b). α-Glucosidase from Saccharomyces cerevisiae was dissolved in phosphate buffer (pH 6.8) containing bovine serum albumin (2 mg/mL) up to 0.5 U/mL concentration. Solution (10 μL) of sample in DMSO–phosphate buffer, pH 6.8 (1:9) at varying concentrations (10, 100, 250, 500, and 1,000 μg/mL) was premixed with 490 μL of phosphate buffer (pH 6.8) and 250 μL 5 mM p-nitrophenyl α-d-glucopyranoside. After preincubating at for 5 min, 250 μL α-glucosidase (0.5 U/mL) was added and incubated at for 15 min. The reaction was terminated by the addition of 2,000 μL Na2CO3 (200 mM). Absorbance was measured at 400 nm. A solution of acarbose (20 mg/mL) was used as a positive control (PC), and water was used as a negative control (NC). The ability to inhibit α-glucosidase was calculated using the following equation:
where A400 NC is the absorbance of the negative control (water) at 400 nm, A400 PC is the absorbance of the positive control (acarbose) at 400 nm and A400 Sample is the absorbance of the sample solution at 400 nm. The IC50 value is the effective concentration at which α-glucosidase activity was inhibited by 50%. Values are expressed as mean obtained from five independent experiments.
α-Amylase inhibitory activity was assayed according to a previously published spectrophotometric protocol (Olennikov and Kashchenko, 2014). Sample solution in DMSO (10 μL) at varying concentrations (10, 100, 250, 500, and 1,000 μg/mL), 30 μL of phosphate buffer (pH 5.0) and 10 μL of α-amylase from Aspergillus oryzae (3 U/mL) were incubated for 20 min at . Then 10 μL of 2% starch solution, 40 μL of phosphate buffer (pH 5.0) and 100 μL of the reagent were added and incubated for 30 min at . The reagent was a solution of K2HPO4 (0.8 mM), KH2PO4 (0.4 mM), phenol (220 mM), 4-aminoantipyrine (1.5 μM), glucose oxidase from Aspergillus oryzae (3 U/mL), and peroxidase from horseradish (0.3 U/mL) in deionized water. Absorbance was measured at 510 nm. A solution of acarbose (20 mg/mL) was used as a positive control (PC), and water was used as a negative control (NC). The ability to inhibit α-amylase was calculated using the following equation:
where A510 NC is the absorbance of the negative control (water) at 510 nm, A510 PC is the absorbance of the positive control (acarbose) at 510 nm and A510 Sample is the absorbance of the sample solution at 510 nm. The IC50 value is the effective concentration at which amylase activity was inhibited by 50%. Values are expressed as mean obtained from five independent experiments.
HPLC-DAD-ESI-TQ-MS/MS Profiling and Compounds Identification Condition
Reversed-phase high-performance liquid chromatography with diode array detection and electrospray ionization mass spectrometry (RP-HPLC-DAD-ESI-TQ-MS/MS) procedure was used for the phenolic compounds profiling. Experiments were performed on an LCMS 8050 liquid chromatograph coupled with diode-array-detector and triple-quadrupole electrospray ionization detector (Shimadzu, Columbia, MD, USA), using a ProntoSIL-120-5-C18 AQ column (1 × 50 mm, Ø 1 μm; Metrohm AG; Herisau, Switzerland), column temperature was . Eluent A was water and eluent B was acetonitrile. The injection volume was 1 μL, and elution flow was 100 μL/min. Gradient program: 0.0–1.0 min 5–21% B, 1.0–2.0 min 21–38% B, 2.0–2.7 min 38–55% B, 2.7–3.5 min 55–61% B, 3.5–5.0 min 61–94% B. The DAD acquisitions were performed in the range of 200–600 nm and chromatograms were integrated at 280 nm. For ESI-MS, the parameters were set as follows: temperature levels of ESI interface, desolvation line and heat block were 300, 250, and 400°C, respectively; the flow levels of nebulizing gas (N2), heating gas (air) and collision-induced dissociation gas (Ar) were 3, 10, and 0.3 mL/min, respectively. The capillary voltage was kept at +3 kV (coumarins) in positive mode and at −4.5 kV (phenylpropanoids, dihydrochalcones and flavonoids) in negative mode. ESI-MS spectra were recorded by scanning in the range of m/z 100–1,900. The identification of compounds was done by analysis of their retention time, ultraviolet and mass-spectrometric data comparing the same parameters with the reference samples and / or literature data. The product ion spectra of the selected precursor ions (MS/MS) were used to improve the reliability of identification of Artemisia phenolic compounds.
HPLC-DAD Quantification Condition
Quantification of the phenolic compounds was performed in HPLC-DAD experiments using chromatographic conditions mentioned above. To prepare the stock solutions of reference compounds, 15 mg of 4-O-caffeoylquinic acid, 5-O-caffeoylquinic acid, 1,3-di-O-caffeoylquinic acid, 3,4-di-O-caffeoylquinic acid, 3,5-di-O-caffeoylquinic acid, 4,5-di-O-caffeoylquinic acid, apigenin-6-C-glucoside-4″-O-glucoside (isosaponarin), quercetin-3-O-(2″-rhamnosyl-)glucoside (calendoflavobioside), quercetin-3-O-(4″-rhamnosyl-)glucoside, quercetin-3-O-(6″-rhamnosyl-)glucoside (rutin), quercetin-3-O-glucoside (isoquercitrin), quercetin-3-O-galactoside (hyperoside), kaempferol-3-O-(6″-rhamnosyl-)glucoside (nicotiflorin), isorhamnetin-3-O-glucoside, and quercetin were accurately weighed and individually dissolved in DMSO/methanol mixture (1:4) in volumetric flasks (1 mL). The external standard calibration curve was generated using 10 data points, covering the concentration ranges 1–1,000 μg/mL. The calibration curves were created by plotting the peak area vs. the concentration levels. Scopoletin-7-O-neohesperidoside (tR 0.92 min) was used as the internal standards and was dissolved separately in DMSO/methanol mixture (1:4) at concentration 1,000 μg/mL. All the analyses were carried out in triplicate and the data were expressed as mean value ± standard deviation (SD). For preparation of extract solution, an accurately weighed extract of Artemisia plant (10 mg) was placed in an Eppendorf tube, 1 mL of 60% ethanol was added, and the mixture was weighted. Then the sample was extracted in an ultrasonic bath for 10 min at 40°C. After cooling, the tube weight was reduced to initial sign, and the resultant extract was filtered through a 0.22-μm PTFE syringe filter before injection into the HPLC system for analysis.
Method Validation
For validation of the analytical method, the guidelines established by the International Conference on the Harmonization of Technical Requirements for the Registration of Pharmaceuticals for Human Use (ICH) were employed (2005). The linearity of the method was studied by injecting five known concentrations of the standard compounds in the defined range. Results from each analysis were averaged and subjected to regression analysis. Limits of detection (LOD) and quantification (LOQ) were determined using the following equations:
where SYX is a standard deviation of the response (Y intercept) and a is a slope of calibration curve. The precision of the analytical method was evaluated by intra-day, inter-day, and repeatability test. Intra-day assay was determined by assaying the mixture solution containing 15 standards (50 μg/mL) during the same day (five injections), and inter-day assay was analyzed using the same concentration for intra-day precision on four different days (interval of 1 day) in the same laboratory. The repeatability test of the sample was performed on 7-fold experiments of the mixture solution containing 15 standards (100 μg/mL). The stability test was performed with one sample solution, which was stored at room temperature and analyzed at 0, 2, 4, 8, 12, 24, and 48 h. For analysis of recovery data, the appropriate amounts of the powdered sample of 15 standards were weighted and spiked with a known amount of each reference compound and then analyzed. Each sample was analyzed in five times.
Statistical and Multivariative Analysis
Statistical analyses were performed using a one-way analysis of variance (ANOVA), and the significance of the mean difference was determined by Duncan's multiple range test. Differences at p < 0.05 were considered statistically significant. The results are presented as mean values ± SD (standard deviations) of the three replicates. Advanced Grapher 2.2 (Alentum Software Inc., Ramat-Gan, Israel) was used to perform linear regression analysis and to generate graphs. Principal component analysis (PCA) based on a data matrix (15 markers × 12 samples) was performed using Graphs 2.0 utility for Microsoft Excel (Komi NTc URO RAN, Syktyvkar, Russia) to generate an overview for groups clustering.
Results
Phenolic Group Content and Inhibitory Activity Against α-Amylase and α-Glucosidase of 12 Artemisia Species
At the preliminary stage of the investigation, the extracts of Artemisia species were obtained and the yields of the extracts were determined (Table 1). The average value of extraction yield of Artemisia species was 29.26 %. Further, the characterization of the phenolic group content was performed. There were significant variations in flavonoid and caffeoylquinic acids (CQAs) contents of Artemisia species. The maximum flavonoid content was observed in A. palustris extract (202.67 mg/g), in turn, minimal value was evident for A. desertorum extract (2.46 mg/g). The content of CQAs in A. commutata extract (514.65 mg/g) considerably exceeded the values of CQAs for other Artemisia species. Minimal CQAs value was detected in A. sericea extract (26.46 mg/g).
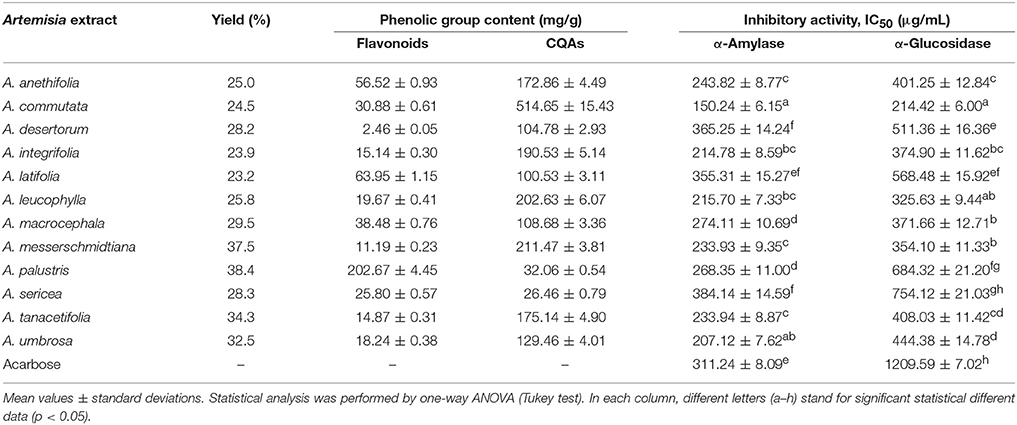
Table 1. Yield of extraction (% of dry plant weight), total flavonoid and caffeoylquinic acids (CQAs) content (mg/g) and inhibitory activity of Artemisia extracts against α-amylase and α-glucosidase (IC50, μg/mL).
After determination of the phenolic contents, the investigation of α-amylase and α-glucosidase inhibitory activity of the extracts examined was conducted. Enzymatic analysis showed that A. commutata extract demonstrated an ability to inhibit α-amylase activity with the highest value (IC50 = 150.24 μg/mL) and the lowest value for A. sericea extract (IC50 = 384.14 μg/L). A similar trend was seen in the α-glucosidase inhibition experiments. A. commutata extract was the most active (IC50 = 150.24 μg/mL) and A. sericea extract displayed considerably worse inhibitor activity of α-glucosidase (IC50 = 754.12 μg/mL). The remaining Artemisia species showed inhibitory activity when present in concentrations higher than 207.12 μg/mL for α-amylase, and 325.63 μg/mL in the case of α-glucosidase. Acarbose inhibited α-amylase and α-glucosidase with IC50 values of 311.24 and 1209.59 μg/mL, respectively.
To understand the links among all the studied chemical parameters and biological potential, linear regression analysis was used (Figure 2). The highest correlation was observed between total CQAs content and enzyme inhibiting activity (r2 = 0.5315–0.6611) opposite total flavonoid content demonstrating weak relationships (r2 = 0.0056–0.2330).
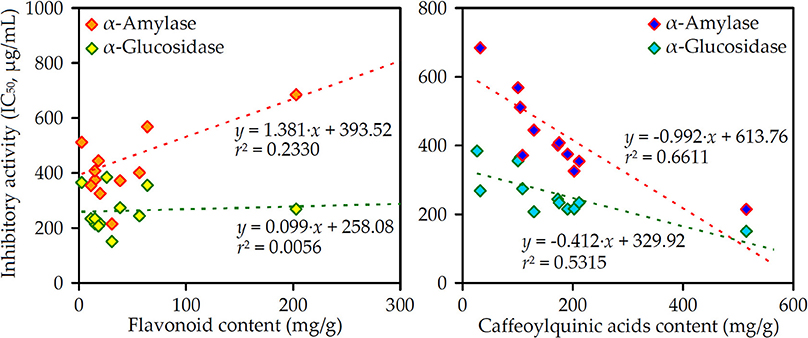
Figure 2. Correlation graphs between flavonoid/caffeoylquinic acids content (mg/g) in the Artemisia extracts and their α-amylase/α-glucosidase inhibitory activity (IC50, μg/mL).
Importantly, the elimination of the phenolic compounds from Artemisia extracts after SPE procedure caused a drastic reduction of α-amylase and α-glucosidase inhibiting activity of the resultant dephenolized extracts (Supplementary Table S1).
Phenolic Profile of 12 Artemisia Species by HPLC-DAD-ESI-TQ-MS/MS
Phenolome profiling of the Artemisia species was implemented after high performance liquid chromatography (HPLC) separation of total methanolic extracts (Figure 3) and flavonoid-enriched SPE fractions (Figure 4) using diode array detection (DAD) and triple quadrupole electrospray ionization detection (TQ-ESI-MS), with both positive and negative mode.
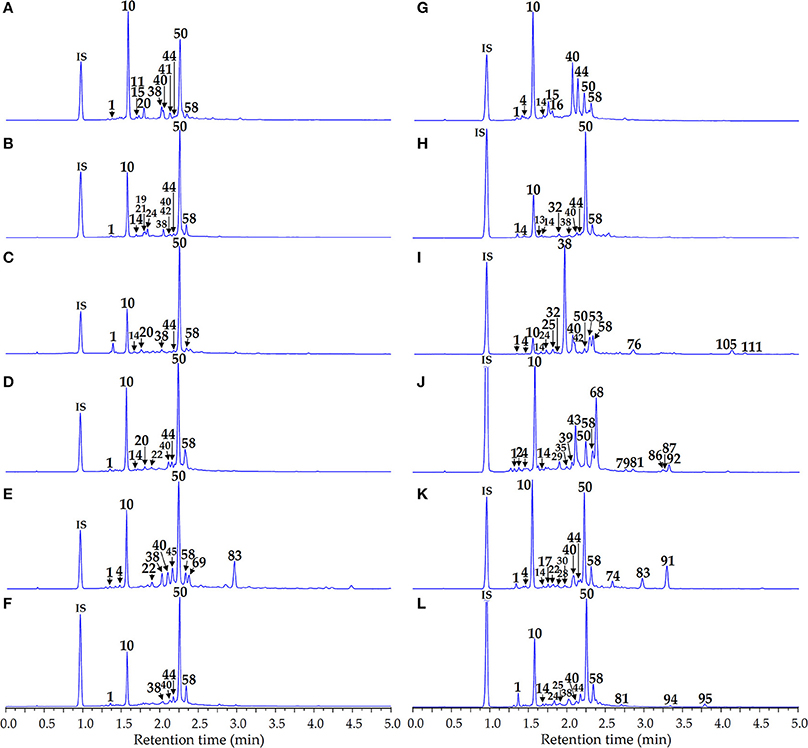
Figure 3. HPLC-DAD chromatograms of the total extracts of Artemisia species at 280 nm. (A) A. anethifolia; (B) A. commutata; (C) A. desertorum; (D) A. integrifolia; (E) A. latifolia; (F) A. leucophylla; (G) A. macrocephala; (H) A. messerschmidtiana; (I) A. palustris; (J) A. sericea; (K) A. tanacetifolia; (L) A. umbrosa. Compounds are numbered as listed in Table 2. IS, internal standard (scopoletin-7-O-neohesperidoside).
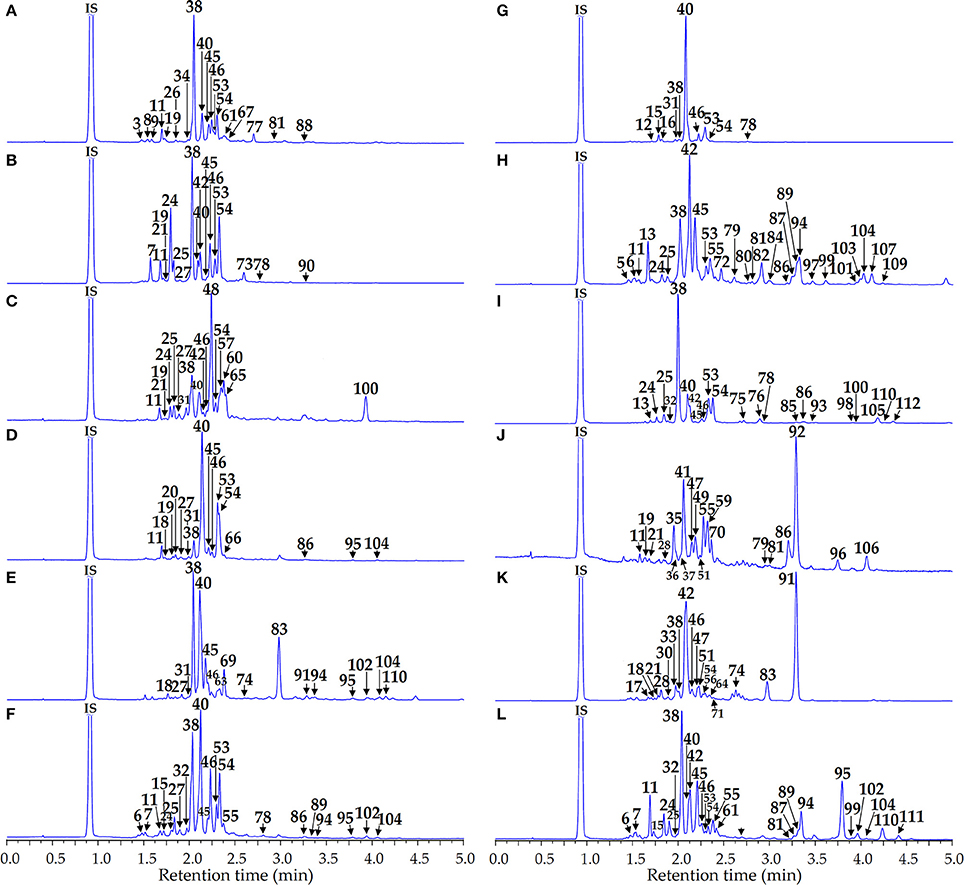
Figure 4. HPLC-DAD chromatograms of the flavonoid-enriched SPE fractions of Artemisia extracts at 280 nm. (A) A. anethifolia; (B) A. commutata; (C) A. desertorum; (D) A. integrifolia; (E) A. latifolia; (F) A. leucophylla; (G) A. macrocephala; (H) A. messerschmidtiana; (I) A. palustris; (J) A. sericea; (K) A. tanacetifolia; (L) A. umbrosa. Compounds are numbered as listed in Table 2. IS, internal standard (scopoletin-7-O-neohesperidoside).
The identification data summarized in Table 2 allowed us to detect a total of 112 phenolic compounds in 12 Artemisia extracts, including phenylpropanoid quinates and glycosides, simple phenolic acid, coumarins, dihydrochalcones and flavonoids (flavones, flavonols, flavanones) both in glycosidic and aglycone form.
Fourteen phenylpropanoids were found in Artemisia extracts in the present study. Compounds 1 and 10 were confirmed as mono-O-caffeoylquinic acids (CQA), according to their UV-spectra and [M–H]− ion with m/z 353, and differentiated as 4-O-CQA and 5-O-CQA, respectively, after comparing with standards. Similar UV-patterns were found for 14, 44, 50, 52, and 58 resulting in [M–H]− ion with m/z 515 and daughter ions with m/z 353 and 191.
Chromatographic behavior was identical to known di-O-caffeoylquinic acids as 1,3-di-O-CQA, 3,4-di-O-CQA, 3,5-di-O-CQA, 1,5-di-O-CQA and 4,5-di-O-CQA, respectively. Compound 22 was characterized by the different UV-maxima (307 nm) and [M–H]− ion with m/z 337 specific for the standard of 5-O-coumaroylquinic acid. Compounds 1, 10, 14, 44, 50, and 58 were found in all Artemisia extracts, in contrast to 22, detected in A. latifolia and A. tanacetifolia, and 52, observed in A. tanacetifolia only. Phenylpropanoid glycosides were presented by 6-O-feruloylglucose (8) in A. anethifolia, two isomers of melilotoside (17, 30) in A. tanacetifolia, dihydroferulic acid-O-glycoside (3) in A. anethifolia and caffeic acid-O-glycoside (5) in A. messerschmidtiana. Compounds 8, 17, and 30 were identified by comparing their chromatographic and spectral data with standards. 3 and 5 were putatively identified using literature data (Vallverdú-Queralt et al., 2010).
The only simple phenolic acid O-glycoside from A. sericea had a UV-maxima at 255, 293 nm and MS-ions (m/z 315 → 153), and was identified as protocatechuic acid-O-glycoside (Chen et al., 2012).
A total of 7 coumarins, of simple structure and hemiterpene-coupled substances, were identified using MS detection with positive ionization mode. Component 14, discovered in five Artemisia extracts, had a pseudomolecular ion with m/z 325 [M+H]+, and an adduct ion with m/z 347 [M+Na]+ as well as a deglucosidated fragment with m/z 163. Similarity to the standard UV-pattern and tR value, allowed us to identify 14 as umbelliferone-7-O-glucoside or skimmin. The values of m/z for the pseudomolecular and adduct ions and deglucosidated fragment of 18, were 30 Da more than 14, indicating a close similarity to 14 structures, with additional methoxy-function. After comparing with the reference compound, it was summarized as scopoletin-7-O-glucoside or scopolin, and was detected in A. anethifolia, A. integrifolia, A. latifolia and A. tanacetifolia. Chromatographic behavior and spectral data of 13, from A. messerschmidtiana, was identical to esculetin.
Compounds 74, 83, and 91, found in A. latifolia and A. tanacetifolia, were putatively identified as rare coumarin-hemiterpene ethers. Specific MS/MS patterns of 74 showed an [M+H]+ ion with m/z 295, two adduct ions with m/z 317 [M+Na]+ and 339 [M+HCOO]+ and fragments initiate by the elimination of water {m/z 277 [(M–H2O)+H]+} and 4′-hydroxy-3′-methylbythoxy-group {m/z 209 [(M–C5H10O)+H]+; 195 [(M–C5H10O–CH2)+H]+}. Thus, it was characterized as 5-hydroxy-7-methoxy-8-(4′-hydroxy-3′-methylbythoxy)coumarin or lacarol, previously isolated from A. laciniata (Hofer et al., 1986) and A. armeniaca (Mojarrab et al., 2011). Compound 83 is similar to 74 MS-fragmentation, with an m/z difference of 16 Da less for all general fragments, indicating a compound structure as the desoxy-analog of lacarol or 7-methoxy-8-(4′-hydroxy-3′-methylbythoxy)coumarin (desoxylacarol). The nature of compound 91 was determined to be methyllacarol, or 5,7-dimethoxy-8-(4′-hydroxy-3′-methylbythoxy)coumarin, due to the difference of m/z values of 14 Da more for the principal MS-fragments, pointing to additional methyl-function (Hofer et al., 1986).
The extract of A. palustris contained four components (85, 98, 105, 112), with a particular UV pattern, distinctive for dihydrochalcones, described early for A. dracunculus (Logendra et al., 2006). Compound 85, which gave a pseudomolecular ion [M–H]− with m/z 257 in the negative ionization mode, and adsorption bands in UV spectrum at 275, 312, and 364 nm, was identified as 4,2′,4′-trihydroxydihydrochalcone, or davidigenin, after comparison with standard (Jensen et al., 1977; Logendra et al., 2006). Three related components (98, 105, 112), with close spectral data, were clearly identified as O-methyl esters of davidigenin. Their MS fragments included [M–H]− ions (m/z 271, 285, 299) and fragments caused by sequential elimination of methyl-functions, like m/z 271 → 256 for 98; m/z 285 → 270 → 255 for 105; and m/z 299 → 284 → 269 → 254 for 112. Thus, they were identified as mono- (98), di- (105) and tri-O-methyl ester of davidigenin (112).
Flavone derivatives of Artemisia extracts were the most representative group of phenolics, which totaled 60 compounds, including 36 glycosides and 24 aglycones. Glycosidic components were derivatives of apigenin (14 compounds), luteolin (12), chrysoeriol (4), 6-hydroxyluteolin (2) and some minor flavones as O-, C- and C,O-glucosides with arabinose, glucose, glucuronic acid and xylose residues.
Apigenin glycosides were detected in all species, except A. palustris, and unambiguous identification was done after comparing spectral data with standards for 19 as apigenin-6-C-glucoside-4″-O-glucoside (isosaponarin), 21 as apigenin-6-C-glucoside-7-O-glucoside (saponarin), 27 as apigenin-6-C-arabinoside-8-C-glucoside (isoschaftoside), 28 as apigenin-6,8-di-C-glucoside (vicenin-2), 31 as apigenin-6-C-glucoside-8-C-arabinoside (schaftoside), 49 as apigenin-6-C-glucoside (isovitexin), and 55 as apigenin-7-O-glucoside (cosmosiin). Two compounds, 6 and 7, with similar UV- and MS-parameters, gave a pseudomolecular ion [M–H]− with m/z 755 and two ions initiated by elimination of two O-bonded hexose moieties (m/z 755 → 593 → 431). Detection of an ion with m/z 341 [(M−2 × Hex−90)–H]−, 311 [(M−2 × Hex−120)–H]−, 313 [(M−2 × Hex−90–CO)–H]−, and 283 [(M−2 × Hex−120–CO)–H]− was characteristic for C-glycosides (Han et al., 2008). Thus, both compounds were isomeric and their structures were determined to be apigenin-C-hexoside-O-hexosyl-hexoside (or apigenin-C-hexoside-di-O-hexoside). Compounds 11, 15, and 20 gave [M–H]− ion with m/z 593 and a series of ions with m/z 431 [(M–Hex)–H]−, 341 [(M-Hex−90)–H]−, 311 [(M–Hex−120)–H]−, 313 [(M–Hex−90–CO)–H]−, and 283 [(M–Hex−120–CO)–H]−, suggesting their structures were apigenin-C-hexoside-O-hexoside. Compound 23, detected in A. desertorum and A. integrifolia, yielded an ion [M–H]− with m/z 607, and the ion of the de-hexuronidated fragment was m/z 431 [(M–HexA)–H]−, as well as C-hexose-specific ions with m/z 341 [(M–HexA−90)–H]−, 311 [(M–HexA−120)–H]−, 313 [(M–HexA−90–CO)–H]−, and 283 [(M–HexA−120–CO)–H]−, characterized the structure as apigenin-C-hexoside-O-hexuronide. Lipophilic O-hexoside 70 from A. tanacetifolia, with a UV spectral maxima at 266 and 334 nm, and [M–H]− ion with m/z 461 and de-hexosidated fragment with m/z 299 was interpreted as apigenin monomethyl ester-O-hexoside.
Twelve luteolin derivatives were discovered only in five Artemisia species, with maximal occurrence in A. sericea (7 compounds). Among them, seven glycosides were identified, with standards, as luteolin-6-C-glucoside-7-O-glucoside (9), luteolin-6,8-di-C-glucoside or lucenin-2 (26), luteolin-6-C-glucoside-8-C-xyloside or lucenin-3 (35), luteolin-6-C-xyloside-8-C-glucoside or lucenin-1 (36), luteolin-6-C-glucoside or isoorientin (41), luteolin-7-O-glucuronide (43) and luteolin-7-O-glucoside or cymaroside (47). Compound 37 gave an [M–H]− ion with m/z 609, and the fragments belonged to flavone-di-C-hexosides like 519 [(M−90)–H]−, 489 [(M−120)–H]−, 429 [(M−2 × 90)–H]−, 399 [(M−120–90)–H]−, 369 [(M−2 × 120)–H]−, 401 [(M−2 × 90–CO)–H]−, 373 [(M−2 × 90–2 × CO)–H]−, 371 [(M−120–90–CO)–H]−, 343 [(M−120–90-2 × CO)–H]−, 341 [(M−2 × 120–CO)–H]−, and 313 [(M−2 × 120–2 × CO)–H]− (Han et al., 2008). The most likely structure of 37 was luteolin-di-C-hexoside, or lucenin-2, that was previously detected in A. monosperma and A. herba-alba (Saleh et al., 1985). Two luteolin-O-hexosides, 51 and 56 (m/z 447 → 285), were discovered in A. sericea and A. tanacetifolia. Luteolin-trimethyl ester-O-hexoside (59; m/z 505 → 343) and luteolin-methyl ester-O-hexoside (77; m/z 461 → 299) were the components of A. sericea and A. anethifolia, respectively.
Three chrysoeriol glycosides, from A. anethifolia, A. sericea and A. umbrosa, were determined to be chrysoeriol-6,8-di-C-glycoside (34), chrysoeriol-7-O-glucoside (61) and chrysoeriol-7-O-glucuronide (62) after comparison with standards. Compound 67, with UV maxima at 283 and 327 nm and [M–H]− ion with m/z 461 and fragment with m/z 299 [(M–Hex)–H]−, was identified as chrysoeriol-O-hexoside. Only two 6-hydroxyluteolin glycosides (29, 39) were detected and both were in A. sericea. Compound 29 gave an [M–H]− ion with m/z 639 and two principal fragments caused by elimination of the residues of hexuronic acid (m/z 463) and hexose (m/z 301), demonstrating its possible structure as 6-hydroxyluteolin-O-hexoside-O-hexuronide (or 6-hydroxyluteolin-O-hexuronyl-hexoside) (Es-Safi et al., 2005). Compound 39 exhibited an [M–H]− ion with m/z 477 and a de-hexuronidated fragment with m/z 301 and was identified as 6-hydroxyluteolin-O-hexuronide. Scutellarein-7-O-glucoside (63) and hispidulin-7-O-glucoside, or homoplantaginin (69), from A. latifolia and nepetin-7-O-glucoside or nepetrin (72) from A. messerschmidtiana were identified with standards. Compound 68 from A. sericea, with UV maxima at 251, 267 and 346 nm and MS fragments with m/z 505 [M-H]− and 329 [(M–GlcA)–H]−, was determined as 5,7,3′-trihydroxy-6,4′-dimetoxyflavone-7-O-glucuronide that has already been found in that species (Wang Q. et al., 2016).
The aglycones with a flavone structure, the largest group of flavonoids, included 24 compounds detected in 8 Artemisia species, except A. commutata, A. desertorum, A. macrocephala and A. tanacetifolia. The vast majority of them have been identified comparing chromatographic and spectral data with standards, identifying scutellarein (76), 6-hydroxyluteolin (79), luteolin (81), nepetin (82), apigenin (86), chrysoeriol (87), hispidulin (89), chrysin (93), jaceosidin (94), eupatilin (95), cirsiliol (97), eupatorin (99), 3′-hydroxygenkwanin (103), genkwanin (104), 5,3′-hydroxy-7,4′-dimetoxyflavone (106), velutin (107), 5-desmethylnobiletin (109), 5-desmethylsinensetin (110) and sinensetin (111). Compound 84 from A. messerschmidtiana gave an [M–H]− ion with m/z 345, and fragments with m/z 330 and 315 indicating the presence of two methoxy-groups and was tentatively identified as 6,8-dihydroxyluteolin-dimethyl ester. Compound 92, from A. sericea with UV maxima at 272 and 343 nm, gave as MS fragment with m/z 329 [M–H]− and two demethylated ions with m/z 314 and 299, indicating the existence of two CH3 groups. It was identified with 5,7,3′-trihydroxy-6,4′-dimetoxyflavone, which was reported as A. frigida previously (Wang Q. et al., 2016). Flavone aglycone 96 gave an [M–H]− ion with m/z 343 and fragments with m/z 328, 313 and 298, caused by elimination of three methyl-groups, and was determined to be 5,4′-dihydroxy-6,7,3′-trimetoxyflavone, also found in A. frigida (Wang Q. et al., 2016). Compounds 101 from A. messerschmidtiana and 108 from A. palustris were provisionally identified as 6-hydroxyluteolin-dimethyl ester and 6-hydroxyluteolin-trimethyl ester, due to their UV-patterns and MS fragmentation (m/z 329 → 314 → 299 for 101; m/z 357 → 342 → 327 → 312 for 108).
Flavonol glycosides were derivatives of kaempferol (5 compounds), quercetin (7), isorhamnetin (4) and quercetagetin (2), with only an O-type of glycosidic bond. Kaempferol glycosides were identified using the standards of nicotiflorin (kaempferol-3-O-rutinoside; 45) and astragalin (kaempferol-3-O-glycoside; 53). Three components (57, 66, 73), with close MS fragmentation (m/z 461, 299) and UV spectra, were assigned the structure of kaempferol-methyl ester-O-hexoside isomers.
The majority of quercetin glycosides were adequately identified using reference compounds with quercetin-3-O-neohesperidoside or calendoflavobioside (24), quercetin-3-O-(4″-rhamnosyl)glycoside (25), quercetin-3-O-rutinoside or rutin (38), quercetin-3-O-glucoside or isoquercitrin (40) and quercetin-3-O-galactoside or hyperoside (42). Compound 32, detected in A. leucophylla, A. palustris and A. umbrosa, gave an [M–H]− ion with m/z 609, and two fragments, induced by loss of desoxyhexose (m/z 463) and hexose moieties (m/z 301). The structure of 32 was assigned as quercetin-O-desoxyhexoside-O-hexoside. Compound 65 was found only in A. desertorum and produced an [M–H]− ion with m/z 491, dehexosylated fragment [(M–Hex)–H]− with m/z 329 and demethylated ions with m/z 314 and 299, demonstrating its structure as quercetin-dimethyl ester-O-hexoside.
Isorhamnetin-3-O-rutinoside or narcissin (46), isorhamnetin-3-O-glucoside (54) and isorhamnetin-3-O-galactoside (71) were found by comparing their properties with standards. Compound 64 was identified on the basis of UV spectrum (255, 354 nm) and MS pattern (m/z 477 → 315) as isorhamnetin-O-hexoside.
Two components of A. desertorum (48, 60) have similar UV spectra but different MS patterns. Compound 48 gave a deprotonated ion with m/z 653 and two fragments with m/z 507 and 345, caused by elimination of desoxyhexose and hexose, but 60 has two principal fragments with m/z 507 [M–H]− and 345 [(M–Hex)–H]−. The presence of the ions with m/z 330 and 315 indicated the loss of two methyl-groups in an aglycone moiety, assigned as quercetagetin-dimethyl ester. Thus, the predicted structures of the compounds were quercetagetin-dimethyl ester-O-desoxyhexosyl-hexoside for 48 and quercetagetin-dimethyl ester-O-hexoside for 60.
Only five flavonol aglycones were detected in seven Artemisia species, among them were quercetin (78), kaempferol (88), isorhamnetin (90) and chrysosplenetin (102), easily identified with standards. Compound 100, from A. desertorum and A. Palustris, gave an [M–H]− ion with m/z 373 and four fragments, after sequential loss of methyl-groups, with m/z 358, 343, 328, and 313. The component was classified as a tetramethoxy-substituted quercetagetin derivative (Valant-Vetschera and Wollenweber, 1995; Valant-Vetschera et al., 2003).
Two flavanone glycosides were found in A. Macrocephala, identified as pyracanthoside (eriodictyol-7-O-glycoside; 16) by comparing with standard, and eriodictyol-O-hexoside (12), based on its spectral data. Aglycones of flavanone were sakuranetin (75) in A. palustris and eriodictyol (80) in A. messerschmidtiana.
Quantitative Analysis of 15 Phenolic Compounds by Microcolumn HPLC-DAD
We identified 15 phenolic compounds, including six caffeoylquinic acids (1, 10, 14, 44, 50, 58) and nine flavonoids (19, 24, 25, 38, 40, 42, 45, 54, 78) that were chosen for their simultaneous quantitative estimation in Artemisia extracts by microcolumn HPLC-DAD assay. Total analysis time was 5 min and scopoletin-7-O-neohesperidoside was used as the internal standard to enhance the quality of method validation criteria. Chromatogram samples of the reference substances mixture and Artemisia extracts are illustrated in Figures 5, 3, respectively. Analyses of calibration curve validation data of 15 compounds, demonstrated their satisfactory linearity with correlation coefficient (r2) values 0.9998–0.9999 and standard deviation (SYX) values 1.14·10−3−9.26·10−3 (Table 3).
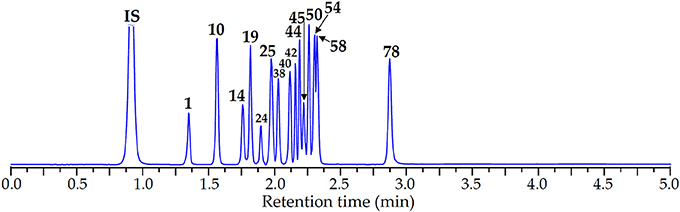
Figure 5. Microcolumn RP-HPLC-DAD chromatogram of the reference mixture of 15 compounds at 280 nm. Compounds are numbered as listed in Table 2. IS, internal standard (scopoletin-7-O-neohesperidoside).
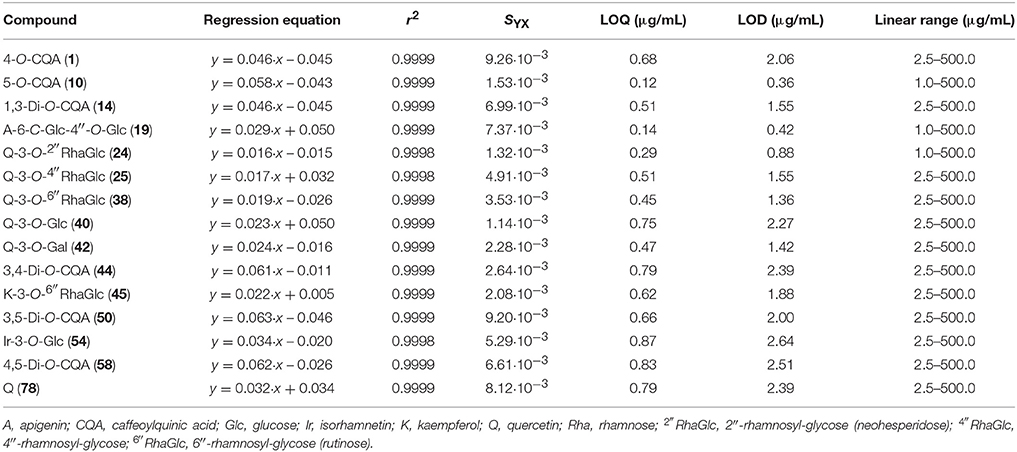
Table 3. Regression equations, correlation coefficients (r2), standard deviation (SYX), limits of detection (LOD), limits of quantitative (LOQ), and linear ranges for 15 compounds.
Concentration linear ranges from 1 to 500 μg/mL were appropriate for quantification and LOQ and LOD parameters and were 0.12–0.87 and 0.36–2.64 μg/mL, respectively. The values of intra- and inter-day precision, repeatability and stability were determined for all compounds analyzed, and their means of RSD did not exceed 3% (Table 4). The range of variation of the recovery parameter was 98.06–102.11%, and is acceptable for quantitative HPLC assay according the guidelines established by the International Conference on the Harmonization of Technical Requirements for the Registration of Pharmaceuticals for Human Use (2005).
Chemical Comparison of 12 Artemisia Species Based on Content of 15 Phenolic Compounds and Principal Component Analysis (PCA)
The quantification of 15 phenolic principal compounds in 12 Artemisia extracts is presented in Table 5. The content of 4-O-caffeoylquinic acid (1) in the extracts analyzed ranged from trace amounts (A. anethifolia) to 5.64 mg/g (A. umbrosa). Another monosubstituted phenylpropanoid, 5-O-caffeoylquinic acid (10), dominated in the extracts of A. anethifolia (78.88 mg/g), A. sericea (20.28 mg/g) and A. tanacetifolia (73.71 mg/g). The highest content of 10 was noticed for A. commutata (127.99 mg/g) extract and the lowest value was found in A. desertorum extract (21.24 mg/g).
Disubstituted phenylpropanoids were represented by the minor compound 1,3-di-O-caffeoylquinic acid (14) with the maximum content revealed in A. macrocephala extract (6.74 mg/g) and traces for A. anethifolia extract; 3,4-di-O-caffeoylquinic acid (44) also had a maximal value for A. macrocephala extract (19.83 mg/g) and traces for A. anethifolia, A. latifolia and A. sericea extracts. Disubstituted 3,5-di-O-caffeoylquinic acid (50) was the predominant compound for a number of Artemisia extracts: A. commutata (243.61 mg/g), A. desertorum (60.51 mg/g), A. integrifolia (87.02 mg/g), A. latifolia (58.55 mg/g), A. leucophylla (108.18 mg/g), A. messerschmidtiana (125.76 mg/g), A. tanacetifolia (65.17 mg/g) and A. umbrosa (57.74 mg/g). The highest content of 4,5-di-O-caffeoylquinic acid (58) was noticed for the A. integrifolia extract (22.71 mg/g), while the traces were found for A. commutata and A. sericea. The maximum total content of phenylpropanoids was detected for A. commutata extract (382.77 mg/g) that significantly exceeded other total contents. The lowest total content was noticed for A. sericea extract (33.16 mg/g).
Principal flavonoids were presented mostly by flavonol glycosides (24, 25, 38, 40, 42, 45, 54), aglycone (78) and flavone glycoside (19). Isosaponarin (19), the derivative of apigenin, was quite rare compound that was quantified only in the extracts of A. anethifolia (1.96 mg/g), A. commutata (8.40 mg/g) and A. integrifolia (0.71 mg/g). The majority of flavonol glycosides were quercetin derivatives (24, 25, 38, 40, 42). Calendoflavobioside (24) also was analyzed in some Artemisia extracts studied: in the extracts of A. commutata (8.48 mg/g), A. palustris (5.90 mg/g) and A. umbrosa (4.08 mg/g). The highest content of quercetin-3-O-(4″-O-rhamnosyl)glycoside (25) was noticed for A. palustris extract (16.14 mg/g), while the remaining species were characterized by minor amounts or traces of 25. The presence of rutin (38) was revealed in all extracts analyzed. Isoquercitrin (40) was the predominant compound for A. palustris extract (82.31 mg/g) and was absent only in A. sericea extract. The presence of hyperoside was observed (42) in the extracts of A. commutata (2.49 mg/g) and A. tanacetifolia (2.00 mg/g). Flavonol glycoside, nicotiflorin (45), the derivative of kaempferol, was discovered with a maximum value in A. palustris extract (17.97 mg/g) and was absent in the extracts of A. desertorum, A. macrocephala, A. sericea, and A. tanacetifolia.
Isorhamnetin derivative, isorhamnetin-3-O-glucoside (54), also was noted with the highest content in A. palustris extract (12.82 mg/g). Flavonol aglycone, quercetin (78), was found only in A. macrocephala extract (1.76 mg/g) in a quantity exceeding a trace. The maximum total content of flavonoids was detected for A. palustris extract (135.14 mg/g) that significantly exceeded other total contents (Figure 6A). It is interesting to note that A. sericea extract either did not contain flavonoids, in general, or contained them in trace amounts.
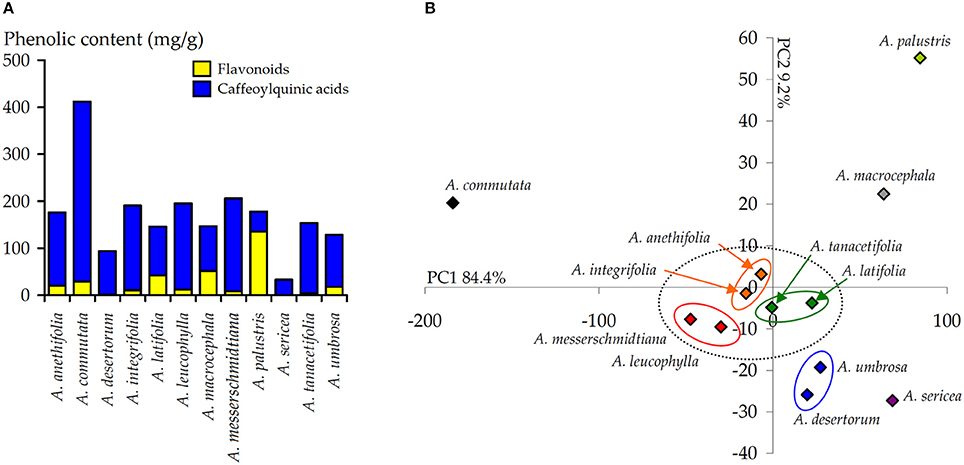
Figure 6. (A) Total content of flavonoids and CQA in the extracts of 12 Artemisia species. (B) Results of principal component analysis (PCA) used the content of 15 phenolic compounds in the extracts of 12 Artemisia species.
The obtained data on the content of 15 phenolic compounds in the extracts of 12 Artemisia species were subjected to principal component analysis (PCA) (Figure 6B). The final scores plot of PCA, as a two-component model, cumulatively consider 93.6% of total variables (PC1, 84.4%; PC2, 9.2%). In the space of the main axes of the ordination, four groups were formed, while the largest number of the samples was located at the center of the diagram. The main part of this group consisted of three pairs elements as A. anethifolia-A. integrifolia, A. tanacetifolia-A. latifolia and A. messerschmidtiana-A. leucophylla. Also, near the centrally located group, the elements of another pair were located A. umbrosa-A. desertorum. In contrast to the main group of elements, four species (A. commutata, A. palustris, A. sericea, A. macrocephala) were not associated into groups.
Inhibitory Activity of Pure Caffeoylquinic Acids Against α-Amylase and α-Glucosidase
Because a high correlation was obtained between the caffeoylquinic acid (CQA) content in Artemisia extracts and extract's enzyme inhibitory activity, some pure compounds were assayed for their inhibitory potential against α-amylase and α-glucosidase. Ten compounds with various degrees of substitution of the quinic acid skeleton were divided into three groups. There were four mono-substituted CQAs (1-O-CQA, 3-O-CQA; 4-O-CQA; 5-O-CQA), five di-substituted CQAs (1,3-di-O-CQA; 1,5-di-O-CQA; 3,4-di-O-CQA; 3,5-di-O-CQA; 4,5-di-O-CQA) and one tri-substituted CQA (3,4,5-tri-O-CQA). The inhibitory effect of the tested CQAs is summarized in Table 6.
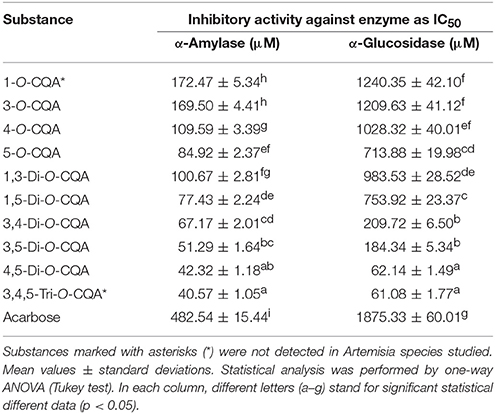
Table 6. Inhibitory activity of pure caffeoylquinic acids (CQA) against α-amylase and α-glucosidase (IC50, μM).
The trisubstituted caffeoylated analog of quinic acid, 3,4,5-tri-O-CQA, as well as 4,5-di-O-CQA, exhibited stronger activity as α-amylase inhibitors with IC50 values of 40.57 and 42.32 μM, respectively, than acarbose (IC50 = 482.54 μM). Two disubstituted CQAs, 3,5-di-O-CQA and 3,4-di-O-CQA, demonstrated similar activities, with IC50 = 51.29 and 67.17 μM, respectively. The other di-CQAs and all mono-substituted CQAs showed moderate inhibition of α-amylase in the range of IC50 from 77.43 (1,5-di-O-CQA) to 172.47 μM (1-O-CQA).
For the α-glucosidase inhibition, the general trend of activity was similar. Two compounds, 3,4,5-tri-O-CQA and 4,5-di-O-CQA, were the most active (IC50 = 61.08 and 62.14 μM, respectively) greatly exceeding the activity of the reference inhibitor acarbose (IC50 = 1875.33 μM). The additional di-CQAs showed average activities with IC50 range 184.34–983.53 μM. The potency of mono-substituted CQAs against α-glucosidase was close to acarbose, because their IC50 values were from 713.88 (5-O-CQA) to 1240.35 μM (1-O-CQA).
Discussion
Some studies have shown that plant species of the Artemisia genus, used in the ethnomedical systems of Asia, have antidiabetic properties (Dabe and Kefale, 2017). The people living in the territory of present-day Northern Asia or Siberia, widely used Artemisia extracts for the treatment of diabetes-like conditions. To our knowledge, this is the first report that validates the carbohydrate digestive enzyme inhibiting activity of Artemisia species, popular in Siberia, using ethnopharmacological information. Additionally, we identified the compounds responsible for the biological activity. Twelve Artemisia species selected for the investigation were characterized as concentrators and superconcentrators of flavonoids with quantitative content ranging from 2.46 (A. desertorum) to 202.67 mg/g (A. palustris). A similar conclusion was reached by quantification of caffeoylquinic acids (CQAs) found ranging from 26.46 (A. sericea) to 514.65 mg/g (A. commutata). All studied Artemisia extracts were effective inhibitors of α-amylase (IC50 = 150.24–384.14 μg/mL; acarbose IC50 = 311.24 μg/mL) and α-glucosidase (IC50 = 214.42–754.12 μg/mL; acarbose IC50 = 1209.59 μg/mL), two digestive enzymes involved in the process of increasing postprandial blood glucose level (Yin et al., 2014). Correlation analysis revealed a direct relationship between the content of CQAs in Artemisia extracts and their enzyme inhibitory activity, in contrast to flavonoids that showed weak correlation links. Removal of the phenolic compounds from Artemisia extracts resulted in a complete loss of inhibitory properties (IC50 > 2,000 μg/mL) and indicated the leading role of these natural compounds in the manifestation of the hypoglycemic effect. Thus, we can confirm the appropriateness of the application of Siberian Artemisia species as α-amylase and α-glucosidase inhibitors, while identifying the phenolic compounds, and especially CQAs, as the main active compounds.
To establish the metabolomic profile of the phenolic compounds, a detailed chromatographic investigation of all Artemisia extracts was performed. At the preliminary stage of the investigation of HPLC profile of the total extracts of Artemisia, it was found that caffeoylquinic acids (CQA), the predominant compounds, interfered with the chromatographic separation process, disguising minor flavonoid glycosides and other compounds. To address this deficiency, methanol extracts were preliminarily subjected to solid phase extraction (SPE) on polyamide, thereby removing of the major proportion of CQA to obtain the flavonoid-enriched SPE fraction. The effectiveness of that prechromatographic treatment of plant extracts to analyze minor compounds was earlier shown (Svehliková et al., 2004; Olennikov et al., 2015a,b; Kashchenko et al., 2017b). The application of two types of extracts to analyze Artemisia species allowed us to achieve greater specificity and sensitivity of metabolomic profiling.
As far as we are concerned, the present study is the first such large-scale scientific project on the investigation of Siberian Artemisia species, resulting in the presence of over a 100 compounds revealed with the capacities of the HPLC-DAD-ESI-TQ-MS/MS method in 12 Artemisia species. Previously, the information of any phenolic compounds was known only for A. integrifolia (flavonoids; Wang J.-L. et al., 2016), A. palustris (flavonoids; Chemesova et al., 1978) and A. tanacetifolia (coumarins; Szabó et al., 1985). The data on the phenolic compounds of A. anethifolia, A. latifolia, A. macrocephala, and A. umbrosa were collected for the first time, and our research is the first to have studied the following five species: A. commutata, A. desertorum, A. leucophylla, A. messerschmidtiana and A. sericea.
Phenylpropanoid quinates were the most common group of the phenolic compounds found in all types of Artemisia, including mono- and dicaffeoylquinic acids with different types of substitutions, as well as 5-O-coumaroylquinic acid, (22) found only in A. latifolia and A. tanacetifolia. Previously it has been repeatedly shown that monotypic and mixed quinates of cinnamic, caffeic and ferulic acids are obligatory components of Artemisia species, including A. absinthium (Fiamegos et al., 2011), A. annua (Han et al., 2008), A. gmelinii (Könczöl et al., 2012), A. iwayomogi (Lee et al., 2017), A. princeps (Cui et al., 2009), A. vulgaris (Fraisse et al., 2011), and others. In contrast to phenylpropanoid quinates, there were only four species of Artemisia in which the representatives of a rare group of phenylpropanoid glycosides were detected, including glycosides of caffeic (5; A. messerschmidtiana), ferulic (8) and dehydroferulic acids (3; A. anethifolia), which, together with glycoside of protocatechuic acid (2; A. sericea), had not been described previously for the genus Artemisia. This applied equally to the isomers of melilotoside (17, 30) from A. tanacetifolia, previously mentioned only for A. splendens (Afshar et al., 2017).
Coumarins are considered ordinary common group of compounds for the genus Artemisia (Al-Hazimi and Basha, 1991). These compounds were discovered in seven studied species in the present research, including 7-O-glycosides of umbelliferone (4) and scopoletin (18). Only in two species, A. latifolia and A. tanacetifolia, coumarin-hemiterpene esters, such as lacarol (74), desoxylacarol (83) and methyllacarol (91) were identified. Both species are related to the Laciniatae subsection of the Abrotanum section, that contains A. laciniata and A. armeniaca, which 74, 83, and 91 were identified earlier (Hofer et al., 1986; Mojarrab et al., 2011). Such a limited rate of detectability of these compounds, only within the subsection Laciniatae, indicates their considerable chemosistematic significance.
Davididenin (85) and its methyl esters (98, 105, 112) were detected only in A. palustris, which is related to the small Auratae subsection of the of the Abrotanum section, together with the unexplored Asian species A. aurata. Despite the lack of scientific information, in view of their uniqueness, these compounds are promising systematic markers for the species of this subsection.
The flavonoid group of flavones is widely distributed in Siberian Artemisia species and has been identified in all studied species. Specific accumulation of C,O-glycosides of apigenin has been established for species of the section Artemisia (A. integrifolia, A. leucophylla, A. umbrosa), as was also shown earlier for the taxonomically similar species A. vulgaris (Lee et al., 1998). A similar pattern of accumulation was found for two representatives of the subgenus Dracunculus – A. commutata (subsection Commutatae, section Campestris) and A. desertorum (subsection Japonica, section Dracunculus), that can concentrate the flavonoids of this group (Kaneta et al., 1978). A. sericea was the only species capable of concentrating flavone glycosides of various types, such as the glycosides of apigenin, luteolin, 6-hydroxyluteoline and others detected. This species relates to the subsection Frigidae (section Absinthium) together with A. frigida, known for its ability to biosynthesize flavones (Wang Q. et al., 2016). A. anethifolia, in particular, was distinguished from the other studied Artemisia species, which selectively accumulated chrysoeriol glycosides (34, 61, 67). Probably, this chemical feature is characteristic for the subsection Anethifoliae (section Absinthium), to which this species belongs. Flavone glycosides were not found in A. palustris, which contains only aglycons, indicating the uniqueness of this species and its distant taxonomic position from other species. It was clearly shown that individual flavones (and even their groups) were distributed extremely unevenly in the species studied. A completely different pattern of occurrence was found for flavonoid glycosides of the flavonol group, where a high frequency for at least eight compounds was observed. These compounds included nicotiflorin (45), astragalin (53), calendoflavobioside (24), quercetin-3-O-(4″-rhamnosyl)glucoside (25), rutin (38), isoquercitrin (40), narcissin (46) and isoramnetin-3-O-glucoside (54). There was an earlier reference to the diversity of flavonols of the genus Artemisia and their almost obligate presence in all species of the genus (Al-Hazimi and Basha, 1991). However, despite their low taxonomic significance, the species A. sericea (subsection Frigidae) should be noted to contain only traces 24 and 25, which can also be considered as a sign of species differentiation at the level of the Absinthium section. Eriodictyol glycosides (12, 16) were found only in A. macrocephala and can be considered a chemical feature of this species, or even the subsection Absinthium (section Absinthium), to which it is related.
In general, it is necessary to note the unusual chemodiversity of the types of the phenolic compounds we found in the Siberian species of Artemisia by the HPLC-DAD-ESI-TQ-MS/MS method. The aforementioned chemical features of accumulation of the individual compounds or their groups can be used further as a convenient taxonomic tool for analysis of the genus Artemisia as a whole. The uniqueness of the individual compound structures can be the reason for the high biological activity of the investigated plant extracts.
The investigation of the accumulation features of individual compounds in 12 Artemisia species showed a significant variability in their quantitative profiles, which is probably due to the taxonomic position of the species within the genus. Caffeoylquinic acids (CQA) were not only obligate components, but also the dominant group of the phenolic compounds for all studied Artemisia species (95.61–382.77 mg/g), except A. palustris where flavonoids prevailed. 5-O-caffeoylquinic acid (10) and 3,5-di-O-caffeoylquinic acid (50) were two major phenylpropanoid quinates and required special attention, owing to widely varying content within the range of 20.28–127.99 and 7.35–243.61 mg/g, respectively. Both compounds are the most frequently detectable and quantifiable pair of dominant phenylpropanoids in various types of Artemisia. For example, it is known that, the concentration of 50 in the ethyl acetate extract of A. selengensis is 106.52 mg/g with a total CQAs content of 241.46 mg/g (Li et al., 2018), the presence of 10 (4.1 mg/g) and 50 (11.99 mg/g) were identified in the methanol extract of A. princeps (Lee et al., 2011). For the A. iwayomogi herb, the presence of 10 and 50 were discovered at 1.66–33.61 and 14.33–68.87 mg/g, respectively (Lee et al., 2017). Diverse CQAs have also been detected in other Asian species of Artemisia, such as A. annua (Han et al., 2008), A. argyi (Zhang et al., 2012), A. capillaris (Seo et al., 2003), A. gmelinii (Könczöl et al., 2012), and A. montana (Jung et al., 2011). The high content of CQAs in the Siberian species of Artemisia indicates their promise as plant sources of phenylpropanoids. There is a high probability that CQAs are obligatory components of the Artemisia genus phenolome, in contrast to flavonoids in which total concentration was significantly lower in the studied species (<0.01–135.14 mg/g). According to literature, the content of flavonoids in previously studied Artemisia extracts was 2.35 mg/g in A. splendens, 2.60 mg/g in A. spicigera (Afshar et al., 2012), 17.21–29.68 mg/g in A. campestris (Khettaf et al., 2016), 18.85–87.04 mg/g in A. absinthium (Lee et al., 2016), 67.98 mg/g in A. vulgaris, 71.41 mg/g in A. arborescens, 93.86 mg/g in A. campestris, 109.02 mg/g in A. santonicum and 121.96 mg/g in A. scoparia (Baykan Erel et al., 2012). Thus, the Siberian species of Artemisia were close to other Eurasian representatives of the same genus, due to the ability to accumulate flavonoids.
In our early studies of natural enzyme inhibitors, high efficiency against α-amylase and α-glucosidase of the phenolic compounds of various structural types was shown, including flavonoids (Kashchenko et al., 2017a; Olennikov and Kashchenko, 2017), coumarins (Olennikov et al., 2017c), phenylpropanoids (Olennikov and Kashchenko, 2014), arylheptanoids (Olennikov and Kashchenko, 2015) and ellagitannins (Kashchenko et al., 2017b). Caffeic acid, which had the greatest biological effect in the series of homologous compounds, was of interest. The investigated Artemisia species were characterized by high content of caffeoylquinic acids (CQAs) derived from caffeic acid, and thereby high inhibitory activity against digestive enzymes was expected. As was shown earlier, the correlation analysis indicated an unambiguous relationship between the concentration of CQAs in the plant extract and its inhibitory activity. The research of CQAs of different structural types confirmed this assumption. Di-CQAs were the most effective inhibitors of α-amylase and α-glucosidase, which exceeded the activity of mono-CQAs, indicating the importance of the number of aromatic substituents in the structure of the compound. The location of the caffeoyl substituent influenced the activity of the compound, while the greatest inhibitory effect was established for 5-O and 4-O-substituted derivatives, in contrast to 1-O- and 3-O-derivatives. This effect was retained for di-substituted CQAs and even tri-substituted CQAs (3,4,5-tri-O-CQAs), although the latter compounds were not identified in the species studied. The most likely reason of this effect could be the ability of CQAs to interact with the functional groups of α-amylase and α-glucosidase that, ultimately, led to a decrease in their effective activity and ability to decompose carbohydrates. Previously, for individual CQAs, it was shown that hydroxyl groups of CQAs can bind with polar groups of enzymes (-OH, -SH, -NH), affecting their hydrophobic properties (Moorthy et al., 2012a,b). Besides, this effect can involve the secondary structure of enzymes, as was shown for 5-O-CQA and 3,5-di-O-CQA (Xu et al., 2015). Similar activity was detected for individual CQAs detected in Tussilafo farfara (Gao et al., 2008), Lonicera fulvotomentosa (Tang et al., 2008), Coffea arabica (Narita and Inouye, 2011), and Ilex kudingcha (Xu et al., 2015), but for the first time, it was revealed for compounds characteristic for Siberian Artemisia species.
Conclusion
The present paper demonstrated the results of the first comprehensive investigation of the Siberian Artemisia species as a source of bioactive phenolics with special attention paid to their inhibitory potential against of α-glucosidase and α-amylase. Bioassay guided screening gave indisputable evidence of the pronounced activity of the extracts from the 12 ethnopharmacologically selected Artemisia species caused by the phenolic compounds. A total 112 phenolics of various groups were unambiguously, or tentatively, identified by HPLC-DAD-ESI-TQ-MS/MS. It was suggested that the flavonoid or coumarin patterns of Artemisia species are as suitable chemotaxonomic tools as other groups of plant phenolics and should be also applied. Quantification data for 15 principal phenolics helped to conclude the caffeoylquinic acids (CQAs) as major constituents, and their concentration levels were in good correlation with enzyme-inhibitory activity of plant extracts. Additional study of pure compounds showed the highest inhibitory potential among di-O-substituted CQAs, indicating that the caffeoyl-substitution at C-5 and C-4 of the quinic acid moiety appears to be a key factor in the α-glucosidase and α-amylase inhibition. This activity can also be a candidate for bioactivity targeting and further in vivo investigations are required to estimate glucose-lowering effect of Artemisia extracts.
Author Contributions
The first author DO and CV mainly responsible for the operation of the whole experiment and the writing of the paper. NC, NK, VN, and S-WK mainly responsible for assisting the first author in the experiment. All authors responsible for providing technical guidance and experimental materials.
Funding
This work was supported by the Federal Agency of Scientific Organizations FASO (0337-2016-0006, AAAA-AA17-117011810037-0) and the Ministry of Education and Science of Russia (20.7216.2017/6.7).
Conflict of Interest Statement
The authors declare that the research was conducted in the absence of any commercial or financial relationships that could be construed as a potential conflict of interest.
Acknowledgments
The authors acknowledge the Chromatographic Center of IGEB for contributing to this work. The authors are very thankful to friends and colleagues kindly providing the photos of Artemisia species as Alexander V. Fateryga (T. I. Vyazemsky Karadag Scientific Station, Nature Reserve of RAS, Feodosiya; Artemisia commutata), Lidia V. Onishchenko (Syktyvkar; Artemisia desertorum, A. messerschmidtiana), Marina Voroshilova (Magadan; Artemisia integrifolia), Mikhail Knyazev (Botanic Garden, UD RAS, Ekaterinburg; Artemisia latifolia), Boris V. Bolshakov (Petropavlovsk Kamtchatskii; Artemisia leucophylla), Petr A. Kosachev (Altai State University, Barnaul; Artemisia macrocephala), Ludmila Palamarchuk (Irkutsk; Artemisia palustris, A. sericea), Elena V. Bayandina (Irkutsk; Artemisia tanacetifolia) and Natal'ya V. Suvorovtseva (Nakhodka, Primorskii Krai; Artemisia umbrosa). Also, we thank the reviewers for carefully reviewing our manuscript and making valuable suggestions.
Supplementary Material
The Supplementary Material for this article can be found online at: https://www.frontiersin.org/articles/10.3389/fphar.2018.00756/full#supplementary-material
References
Abad, M. J., Bedoya, L. M., Apaza, L., and Bermejo, P. (2012). The Artemisia L. genus: a review of bioactive essential oils. Molecules 17, 2542–2566. doi: 10.3390/molecules17032542
Adekenov, S. M., Kadirberlina, G. M., Kagarlitskii, A. D., Mukhametzhanov, M. N., Fomichev, A. A., and Golovtsov, N. I. (1984). Arlatin–a new sesquiterpene lactone from Artemisia latifolia. Chem. Nat. Comp. 20, 755–757. doi: 10.1007/BF00580049
Afolayan, A. J., and Sunmonu, T. O. (2011). Artemisia afra Jacq. ameliorates oxidative stress in the pancreas of streptozotocin-induced diabetic Wistar rats. Biosci. Biotechnol. Biochem. 75, 2083–2086. doi: 10.1271/bbb.100792
Afshar, F. H., Delazar, A., Nazemiyeh, H., Esnaashari, S., and Moghadam, S. B. (2012). Comparison of the total phenol, flavonoid contents and antioxidant activity of methanolic extracts of Artemisia spicigera and A. splendens growing in Iran. Pharm. Sci. 18, 165–170.
Afshar, F. H., Delazar, A., Nazemiyeh, H., Nahar, L., Moghaddam, S. B., Mbaebie, B. O., et al. (2017). Melilotoside derivatives from Artemisia splendens (Asteraceae). Rec. Nat. Prod. 11, 43–50.
Al-Hazimi, H. M. G., and Basha, R. M. Y. (1991). Phenolic compounds from various Artemisia species. J. Chem. Soc. Pak. 13, 277–289.
Anaya-Eugenio, G. D., Rivero-Cruz, I., Rivera-Chávez, J., and Mata, R. (2014). Hypoglycemic properties of some preparations and compounds from Artemisia ludoviciana Nutt. J. Ethnopharmacol. 155, 416–425. doi: 10.1016/j.jep.2014.05.051
Aseeva, T. A., Dashiev, D. B., Dashiev, A. D., Nikolaev, S. M., Surkova, N. A., Chekhirova, G. V., et al. (2008). Tibetan Medicine of Buryats. SO RAN: Novosibirsk, 217–263.
Awad, N. E., Seida, A. A., Shaffie, Z. E., El-Aziz, A. M., and Awad, N. E. (2012). Hypoglycemic activity of Artemisia herba-alba (Asso) used in Egyptian traditional medicine as hypoglycemic remedy. J. Appl. Pharm. Sci. 2, 30–39. doi: 10.7324/JAPS.2012.2306
Bakhshyeva, N. C., Aleskerova, A. N., and Serkerov, S. V. (2011). Terpenoids from Artemisia anethifolia. Chem. Nat. Comp. 47, 648–649. doi: 10.1007/s10600-011-0021-3
Batorova, S. M., Yakovlev, G. P., and Aseeva, T. A. (2013). Reference-Book of Traditional Tibetan Medicine Herbs. Nauka: St. Petersburg. 172–175.
Baykan Erel, S., Reznicek, G., Senol, S. G., Karabay Yavaşogulu, N. Ü., Konyalioglu, S., and Zeybek, A. U. (2012). Antimicrobial and antioxidant properties of Artemisia L. species from western Anatolia. Turk. J. Biol. 36, 75–84. doi: 10.3906/biy-0912-27
Bora, K. S., and Sharma, A. (2011). The genus Artemisia: a comprehensive review. Pharm. Biol. 49, 101–109. doi: 10.3109/13880209.2010.497815
Chemesova, I. I., Belenovskaya, L. M., and Markova, L. P. (1978). Phenolic compounds of Artemisia palustris. Chem. Nat. Comp. 14, 338–338. doi: 10.1007/BF00713338
Chen, H. J., Inbaraj, B. S., and Chen, B. (2012). Determination of phenolic acids and flavonoids in Taraxacum formosanum kitam by liquid chromatography-tandem mass spectrometry coupled with a post-column derivatization technique. Int. J. Mol. Sci. 13, 260–285. doi: 10.3390/ijms13010260
Chirikova, N. K., Olennikov, D. N., and Tankhaeva, L. M. (2010). Quantitative determination of flavonoid content in the aerial parts of Baikal skullcap (Scutellaria baicalensis Georgi). Russ. J. Bioorg. Chem. 36, 915–922. doi: 10.1134/S1068162010070204
Cui, C.-B., Jeong, S. K., Lee, Y. S., Lee, S. O., Kang, I.-J., and Lim, S. S. (2009). Inhibitory activity of caffeoylquinic acids from the aerial parts of Artemisia princeps on rat lens aldose reductase and on the formation of advanced glycation end products. J. Korean Soc. Appl. Biol. Chem. 52, 655–662. doi: 10.3839/jksabc.2009.109
Dabe, N. E., and Kefale, A. T. (2017). Antidiabetic effects of Artemisia species: a systematic review. Anc. Sci. Life 36, 175–181. doi: 10.4103/asl.ASL_87_17
Daradka, H. M., and Abas, M. M. (2014). Antidiabetic effect of Artemisia absinthium extracts on alloxan-induced diabetic rats. Comp. Clin. Pathol. 23, 1733–1742. doi: 10.1007/s00580-014-1963-1
Es-Safi, N. E., Kerhoas, L., and Ducrot, P. H. (2005). Application of positive and negative electrospray ionization, collision-induced dissociation and tandem mass spectrometry to a study of the fragmentation of 6-hydroxyluteolin 7-O-glucoside and 7-O-glucosyl-(1-3)-glucoside. Rapid Commun. Mass Spectrom. 19, 2734–2742. doi: 10.1002/rcm.2122
Etxeberria, U., De La Garza, A. L., Campin, J., Martnez, J. A., and Milagro, F. I. (2012). Antidiabetic effects of natural plant extracts via inhibition of carbohydrate hydrolysis enzymes with emphasis on pancreatic alpha amylase. Expert Opin. Ther. Targets 16, 269–297. doi: 10.1517/14728222.2012.664134
Evstratova, R. I., and Sinyukhin, A. M. (1971). Ketopelenolide b from Artemisia anethifolia. Chem. Nat. Comp. 7, 719–719. doi: 10.1007/BF00567959
Fiamegos, Y. C., Kastritis, P. L., Exarchou, V., Han, H., Bonvin, A. M., Vervoort, J., et al. (2011). Antimicrobial and efflux pump inhibitory activity of caffeoylquinic acids from Artemisia absinthium against gram-positive pathogenic bacteria. PLoS ONE 6:e18127. doi: 10.1371/journal.pone.0018127
Fraisse, D., Felgines, C., Texier, O., and Lamaison, J.-L. (2011). Caffeoyl derivatives: major antioxidant compounds of some wild herbs of the Asteraceae family. Food Nutr. Sci. 2, 181–192. doi: 10.4236/fns.2011.23025
Gao, H., Huang, Y.-N., Gao, B., Xu, P.-Y., Inagaki, C., and Kawabata, J. (2008). α-Glucosidase inhibitory effect by the flower buds of Tussilago farfara L. Food Chem. 106, 1195–1201. doi: 10.1016/j.foodchem.2007.07.064
Ghazanfar, K., Ganai, B. A., Akbar, S., Mubashir, K., Dar, S. A., Dar, M. Y., et al. (2014). Antidiabetic activity of Artemisia amygdalina decne in streptozotocin induced diabetic rats. Biomed. Res. Int. 2014:185676. doi: 10.1155/2014/185676
Han, J., Ye, M., Qiao, X., Xu, M., Wang, B., and Guo, D. (2008). Characterization of phenolic compounds in the Chinese herbal drug Artemisia annua by liquid chromatography coupled to electrospray ionization mass spectrometry. J. Pharm. Biomed. Anal. 47, 516–525. doi: 10.1016/j.jpba.2008.02.013
Heinrich, M. (2010). Ethnopharmacology in the 21st century–grand challenges. Front. Pharmacol. 1:8. doi: 10.3389/fphar.2010.00008
Hofer, O., Szabó, G., Greger, H., and Nikiforov, A. (1986). Leaf coumarins from the Artemisia laciniata group. Eur. J. Org. Chem. 12, 2142–2149. doi: 10.1002/jlac.198619861208
International Conference on the Harmonization of Technical Requirements for the Registration of Pharmaceuticals for Human Use (2005). In Q2B(R1): Guideline on Validation of Analytical Procedure—Methodology. Brussels: ICH Steering Committee.
Ivanescu, B., Miron, A., and Corciova, A. (2015). Sesquiterpene lactones from Artemisia genus: biological activities and methods of analysis. J. Anal. Methods Chem. 2015:247685. doi: 10.1155/2015/247685
Jensen, S. R., Nielsen, B. J., and Norn, V. (1977). Dihydrochalcones from Viburnum davidii and V. lantanoides. Phytochemistry 16, 2036–2038. doi: 10.1016/0031-9422(77)80128-3
Jung, H. A., Islam, M. D., Kwon, Y. S., Jin, S. E., Son, Y. K., Park, J. J., et al. (2011). Extraction and identification of three major aldose reductase inhibitors from Artemisia montana. Food Chem. Toxicol. 49, 376–384. doi: 10.1016/j.fct.2010.11.012
Kaneta, M., Hikichi, H., Endo, S., and Sugiyama, N. (1978). Identification of flavones in sixteen Compositae species. Agric. Biol. Chem. 42, 475–477. doi: 10.1080/00021369.1978.10863001
Kashchenko, N. I., Chirikova, N. K., and Olennikov, D. N. (2017a). Acylated flavonoids of Spiraea genus as α-amylase inhibitors. Khimiya Rastitel'nogo Syr'ya No 4, 81–90. doi: 10.14258/jcprm.2017042144
Kashchenko, N. I., Chirikova, N. K., and Olennikov, D. N. (2017b). Agrimoniin, an active ellagitannin from Comarum palustre herb with anti-α-glucosidase and antidiabetic potential in streptozotocin-induced diabetic rats. Molecules 22:73. doi: 10.3390/molecules22010073
Khettaf, A., Belloula, N., and Dridi, S. (2016). Antioxidant activity, phenolic and flavonoid contents of some wild medicinal plants in southeastern Algeria. Afr. J. Biotechnol. 15, 524–530. doi: 10.5897/AJB2015.14459
Könczöl, A., Béni, Z., Sipos, M. M., Rill, A., Háda, V., Hohmann, J., et al. (2012). Antioxidant activity-guided phytochemical investigation of Artemisia gmelinii Webb. ex Stechm.: isolation and spectroscopic challenges of 3,5-O-dicaffeoyl (epi?) quinic acid and its ethyl ester. J. Pharm. Biomed. Anal. 59, 83–89. doi: 10.1016/j.jpba.2011.10.012
Kumar, S., and Pandey, A. K. (2013). Chemistry and biological activities of flavonoids: an overview. Sci. World J. 2013:162750. doi: 10.1155/2013/162750
Lee, S. G., Lee, H., Nam, T. G., Eom, S. H., Heo, H. J., Lee, C. Y., et al. (2011). Neuroprotective effect of caffeoylquinic acids from Artemisia princeps Pampanini against oxidative stress-induced toxicity in PC-12 cells. J. Food Sci. 76, C250–C256. doi: 10.1111/j.1750-3841.2010.02010.x
Lee, S.-J., Chung, H.-Y., Maier, C. G.-A., Wood, A. R., Dixon, R. A., and Mabry, T. J. (1998). Estrogenic flavonoids from Artemisia vulgaris L. J. Agric. Food Chem. 46, 3325–3329. doi: 10.1021/jf9801264
Lee, Y.-J., Thiruvengadam, M., Chung, I.-M., and Nagella, P. (2016). Polyphenol composition and antioxidant activity from the vegetable plant Artemisia absinthium L. Ausr. J. Crop Sci. 7, 1921–1926.
Lee, Y. K., Hong, E. Y., and Whang, W. K. (2017). Inhibitory effect of chemical constituents isolated from Artemisia iwayomogi on polyol pathway and simultaneous quantification of major bioactive compounds. Bio. Med. Res. Int. 2017:7375615. doi: 10.1155/2017/7375615
Li, Y., Wan, Y., Li, R., Xu, L., Xie, M., and Fu, G. (2018). Solvent extraction of caffeoylquinic acids from Artemisia selengensis Turcz leaves and their in vitro inhibitory activities on xanthine oxidase. Ind. Crops Prod. 118, 296–301. doi: 10.1016/j.indcrop.2018.03.055
Li, Y., Zheng, M., Zhai, X., Huang, Y., Khalid, A., Malik, A., et al. (2015). Effect of Gymnema sylvestre, Citrullus colocynthis and Artemisia absinthium on blood glucose and lipid profile in diabetic human. Acta Pol. Pharm. 72, 981–985.
Logendra, S., Ribnicky, D. M., Yang, H., Poulev, A., Ma, J., Kennelly, E. J., et al. (2006). Bioassay-guided isolation of aldose reductase inhibitors from Artemisia dracunculus. Phytochemistry 67, 1539–1546. doi: 10.1016/j.phytochem.2006.05.015
Mojarrab, M., Delazar, A., Moghadam, S. B., Nazemiyeh, H., Nahar, L., Kumarasamy, Y., et al. (2011). Armenin and isoarmenin–Two prenylated coumarins from the aerial parts of Artemisia armeniaca. Chem. Biodiv. 8, 2097–2103. doi: 10.1002/cbdv.201000284
Moorthy, N. S. H. N., Ramos, M. J., and Fernandes, P. A. (2012a). Comparative structural analysis of α-glucosidase inhibitors on difference species: a computational study. Arch. Pharm. 345, 265–274. doi: 10.1002/ardp.201100047
Moorthy, N. S. H. N., Ramos, M. J., and Fernandes, P. A. (2012b). Studies on α-glucosidase inhibitors development: magic molecules for the treatment of carbohydrate mediated diseases. Mini Rev. Med. Chem. 12, 713–720. doi: 10.2174/138955712801264837
Narita, Y., and Inouye, K. (2011). Inhibitory effects of chlorogenic acids from green coffee beans and cinnamate derivatives on the activity of porcine pancreas α-amylase isozyme. Food Chem. 127, 1532–1539. doi: 10.1016/j.foodchem.2011.02.013
Nofal, S. M., Mahmoud, S. S., Ramadan, A., Soliman, G. A., and Fawzy, R. (2009). Anti-diabetic effect of Artemisia judaica extracts. Res. J. Med. Med. Sci. 4, 42–48.
Olennikov, D. N. (2018). Phytoecdysteroids and flavonoids from Gastrolychnis tristis. Chem. Nat. Comp. 54, 204–206. doi: 10.1007/s10600-018-2300-8
Olennikov, D. N., and Akobirshoeva, A. A. (2016). Flavonoids and phenypropanoids from Nepeta glutinosa? Ziziphora pamiroalaica. Chem. Nat. Comp. 52, 775–777. doi: 10.1007/s10600-016-1812-3
Olennikov, D. N., and Chirikova, N. K. (2013). Phenolic compounds and cinnamamide from Scutellaria scordiifolia. Chem. Nat. Comp. 49, 124–126. doi: 10.1007/s10600-013-0528-x
Olennikov, D. N., Gornostai, T. G., Penzina, T. A., and Borovskii, G. B. (2017a). Lupane triterpenoids and sterols from Inonotus rheades mycelium and their anti-glucosidase activity. Chem. Nat. Comp. 53, 988–990. doi: 10.1007/s10600-017-2180-3
Olennikov, D. N., and Kashchenko, N. I. (2013). New isorhamnetin glucosides and other phenolic compounds from Calendula officinalis. Chem. Nat. Comp. 49, 833–840. doi: 10.1007/s10600-013-0759-x
Olennikov, D. N., and Kashchenko, N. I. (2014). Componential profile and amylase inhibiting activity of phenolic compounds from Calendula officinalis L. leaves. Sci. World J. 2014:654193. doi: 10.1155/2014/654193
Olennikov, D. N., and Kashchenko, N. I. (2015). 1-Dehydro-[14]-gingerdione, a new component from Zingiber officinale. Chem. Nat. Comp. 51, 753–758. doi: 10.1007/s10600-015-1438-x
Olennikov, D. N., and Kashchenko, N. I. (2017). Spireasalicin, a new acylated glycoside of quercetin from Spiraea salicicfolia. Chem. Nat. Comp. 53, 1038–1044. doi: 10.1007/s10600-017-2197-7
Olennikov, D. N., Kashchenko, N. I., and Chirikova, N. K. (2015a). Bitter gentian teas: nutritional and phytochemical profiles, polysaccharide characterisation and bioactivity. Molecules 20, 20014–20030. doi: 10.3390/molecules201119674
Olennikov, D. N., Kashchenko, N. I., and Chirikova, N. K. (2017b). Meadowsweet teas as new functional beverages: comparative analysis of nutrients, phytochemicals and biological effects of four Filipendula species. Molecules 22, A64. doi: 10.3390/molecules22010016
Olennikov, D. N., Kashchenko, N. I., Chirikova, N. K., and Tankhaeva, L. M. (2015b). Iridoids and flavonoids of four Siberian gentians: chemical profile and gastric stimulatory effect. Molecules 20, 19172–19188. doi: 10.3390/molecules201019172
Olennikov, D. N., Kashchenko, N. I., and Vennos, C. (2017c). A new esculetin glycoside from Calendula officinalis (Asteraceae) and its bioactivity. Farmacia 65, 698–702.
Olennikov, D. N., Stolbikova, A. V., Tankhaeva, L. M., and Petrov, E. V. (2011). Phenylpropanoids and polysaccharides of Plantago depressa and P. media. Chem. Nat. Comp. 47, 165–169. doi: 10.1007/s10600-011-9872-x
Pandey, A. K., and Singh, P. (2017). The genus Artemisia: a 2012–2017 literature review on chemical composition, antimicrobial, insecticidal and antioxidant activities of essential oils. Medicines 4:68. doi: 10.3390/medicines4030068
Prajnya, S. (2008). Kun Phan Bdud Rtsi: Big Receipt Book of Aginskii Datsan. Moscow: Vostochnaya Literatura, 179–195.
Ribnicky, D. M., Poulev, A., Watford, M., Cefalu, W. T., and Raskin, I. (2006). Antihyperglycemic activity of Tarralin, an ethanolic extract of Artemisia dracunculus L. Phytomedicine 13, 550–557. doi: 10.1016/j.phymed.2005.09.007
Saleh, N. A. M., El-Negoumy, S. I., Abd-Alla, M. F., Abou-Zaid, M. M., Dellamonica, G., and Chopin, J. (1985). Flavonoid glycosides of Artemisia monosperma and A. herba-alba. Phytochemistry 24, 201–203. doi: 10.1016/S0031-9422(00)80845-6
Seo, H.-C., Suzuki, M., Ohnishi-Kameyama, M., Oh, M.-J., Kim, H.-R., Kim, J.-H., et al. (2003). Extraction and identification of antioxidant components from Artemisia capillaris herba. Plant Foods Hum. Nutr. 58, 1–12. doi: 10.1023/B:QUAL.000
Shoaib, M., Shah, I., Ali, N., Adhikari, A., Tahir, M. N., Shah, S. W., et al. (2017). Sesquiterpene lactone! a promising antioxidant, anticancer and moderate antinociceptive agent from Artemisia macrocephala Jacquem. BMC Complement. Altern. Med. 17:27. doi: 10.1186/s12906-016-1517-y
Shoaib, M., Shah, I., Ali, N., and Shah, S. W. A. (2015). In vitro acetylcholinesterase and butyrylcholinesterase inhibitory potentials of essential oil of Artemisia macrocephala. Bangladesh J. Pharmacol. 10, 87–91. doi: 10.3329/bjp.v10i1.21171
Suleimen, E. M., Dudkin, R. V., Gorovoi, P. G., Wang, M., Khan, I., and Ross, S. A. (2014). Composition and bioactivity of Artemisia umbrosa essential oil. Chem. Nat. Comp. 50, 545–546. doi: 10.1007/s10600-014-1011-z
Svehliková, V., Bennett, R. N., Mellon, F. A., Needs, P. W., Piacente, S., Kroon, P. A., et al. (2004). Isolation, identification and stability of acylated derivatives of apigenin 7-O-glucoside from chamomile (Chamomilla recutita [L.] Rauschert). Phytochemistry 65, 2323–2332. doi: 10.1016/j.phytochem.2004.07.011
Szabó, G., Greger, H., and Hofer, O. (1985). Coumarin-hemiterpene ethers from Artemisia species. Phytochemistry 24, 537–541. doi: 10.1016/S0031-9422(00)80763-3
Tǎnase, C., Coşarcă, S., Toma, F., Mare, A., Man, A., Miklos, A., et al. (2018). Antibacterial activities of beech bark (Fagus sylvatica L.) polyphenolic extract. Environ. Eng. Manag. J. 17, 877–884.
Tan, R. X., Zheng, W. F., and Tang, H. Q. (1998). Biologically active substances from the genus Artemisia. Planta Med. 64, 295–302. doi: 10.1055/s-2006-957438
Tang, D., Li, H. J., Li, P., Wen, X. D., and Qian, Z. M. (2008). Interaction of bioactive components caffeoylquinic acid derivatives in Chinese medicines with bovine serum albumin. Chem. Pharm. Bull. 56, 360–365. doi: 10.1248/cpb.56.360
Tashenov, E. O., Dzhalmakhanbetova, R. I., Smagulova, F. M., Dudkin, R. V., Gorovoi, P. G., Suleiman, E. M., et al. (2013). Cirsilineol and cubreuva lactone from Artemisia umbrosa and their biological activity. Chem. Nat. Comp. 49, 97–98. doi: 10.1007/s10600-013-0517-0
Valant-Vetschera, K. M., Fischer, R., and Wollenweber, E. (2003). Exudate flavonoids in species of Artemisia (Asteraceae-Anthemideae): new results and chemosystematic interpretation. Biochem. Syst. Ecol. 31, 487–498. doi: 10.1016/S0305-1978(02)00178-3
Valant-Vetschera, K. M., and Wollenweber, E. (1995). Flavonoid aglycones from the leaf surfaces of some Artemisia spp. (Compositae-Anthemideae). Z. Naturforsch. 50c, 353–357. doi: 10.1515/znc-1995-5-604
Vallverdú-Queralt, A., Jáuregui, O., Medina-Remón, A., Andrés-Lacueva, C., and Lamuela-Raventós, R. M. (2010). Improved characterization of tomato polyphenols using liquid chromatography/electrospray ionization linear ion trap quadrupole Orbitrap mass spectrometry and liquid chromatography/electrospray ionization tandem mass spectrometry. Rapid Commun. Mass Spectrom. 24, 2986–2992. doi: 10.1002/rcm.4731
Wang, Q., Jin, J., Dai, N., Han, N., Han, J., and Bao, B. (2016). Anti-inflammatory effects, nuclear magnetic resonance identification, and high-performance liquid chromatography isolation of the total flavonoids from Artemisia frigida. J. Food Drug Anal. 24, 385–391. doi: 10.1016/j.jfda.2015.11.004
Wang, J.-L., Yi, Z.-C., Ma, Y.-L., Zhao, M., Li, J., and Zhang, S.-J. (2016). Study on chemical constituents of Artemisia integrifolia. Chin. Tradit. Herbal Drugs 47, 2241–2245. doi: 10.7501/j.issn.0253-2670.2016.13.007
Xiao, J., Kai, G., Yamamoto, K., and Chen, X. (2013a). Advance in dietary polyphenols as α-glucosidases inhibitors: a review on structure-activity relationship aspect. Crit. Rev. Food Sci. Nutr. 53, 818–836. doi: 10.1080/10408398.2011.561379
Xiao, J., Ni, X., Kai, G., and Chen, X. (2013b). A review on structure-activity relationship of dietary polyphenols inhibiting α-amylase. Crit. Rev. Food Sci. Nutr. 53, 497–506. doi: 10.1080/10408398.2010.548108
Xing, X. H., Zhang, Z. M., Hu, X. Z., Wu, R. Q., and Xu, C. (2009). Antidiabetic effects of Artemisia sphaerocephala Krasch. Gum, a novel food additive in China, on streptozotocin-induced type 2 diabetic rats. J. Ethnopharmacol. 125, 410–416. doi: 10.1016/j.jep.2009.07.021
Xu, D., Wang, Q., Zhang, W., Hu, B., Zhou, L., Zeng, X., et al. (2015). Inhibitory activities of caffeoylquinic acid derivatives from Ilex kudingcha C.J. Tseng on α-glucosidase from Saccharomyces cerevisiae. J. Agric. Food Chem. 63, 3694–3703. doi: 10.1021/acs.jafc.5b00420
Yin, Z., Zhang, W., Feng, F., Zhang, Y., and Kang, W. (2014). α-Glucosidase inhibitors isolated from medicinal plants. Food Sci. Hum. Well. 3, 136–174. doi: 10.1016/j.fshw.2014.11.003
Zhang, Y. H., Xue, M. Q., Bai, Y. C., Yuan, H. H., Zhao, H. L., and Lan, M. B. (2012). 3,5-Dicaffeoylquinic acid isolated from Artemisia argyi and its ester derivatives exert anti-leucyl-tRNA synthetase of Giardia lamblia (GlLeuRS) and potential anti-giardial effects. Fitoterapia 83, 1281–1285. doi: 10.1016/j.fitote.2012.05.016
Keywords: Artemisia, Asteraceae, caffeoylquinic acids, flavonoids, high performance liquid chromatography, mass spectrometry, α-amylase inhibition, α-glucosidase inhibition
Citation: Olennikov DN, Chirikova NK, Kashchenko NI, Nikolaev VM, Kim S-W and Vennos C (2018) Bioactive Phenolics of the Genus Artemisia (Asteraceae): HPLC-DAD-ESI-TQ-MS/MS Profile of the Siberian Species and Their Inhibitory Potential Against α-Amylase and α-Glucosidase. Front. Pharmacol. 9:756. doi: 10.3389/fphar.2018.00756
Received: 27 April 2018; Accepted: 21 June 2018;
Published: 12 July 2018.
Edited by:
Sujogya Kumar Panda, North Orissa University, IndiaReviewed by:
Susan Semple, University of South Australia, AustraliaPinarosa Avato, Università degli studi di Bari Aldo Moro, Italy
Andrei Mocan, Iuliu Haţieganu University of Medicine and Pharmacy, Romania
Luísa Custódio, Centro de Ciências do Mar (CCMAR), Portugal
Copyright © 2018 Olennikov, Chirikova, Kashchenko, Nikolaev, Kim and Vennos. This is an open-access article distributed under the terms of the Creative Commons Attribution License (CC BY). The use, distribution or reproduction in other forums is permitted, provided the original author(s) and the copyright owner(s) are credited and that the original publication in this journal is cited, in accordance with accepted academic practice. No use, distribution or reproduction is permitted which does not comply with these terms.
*Correspondence: Daniil N. Olennikov, olennikovdn@mail.ru