- 1Heilongjiang Key Laboratory for Animal Disease Control and Pharmaceutical Development, Faculty of Basic Veterinary Science, College of Veterinary Medicine, Northeast Agricultural University, Harbin, China
- 2Laboratory of Veterinary Pathology, Faculty of Basic Veterinary Science, College of Animal Science and Veterinary Medicine, Shenyang Agricultural University, Shenyang, China
- 3Changchun Dirui Medical Company Ltd., Changchun, China
- 4College of Life Science Engineering, Shenyang Institute of Technology, Fushun, China
- 5College of Food Science, Northeast Agricultural University, Harbin, China
It is well understood that liver cytochrome p450 enzymes are responsible for AFB1 bioactivation, while phase-II enzymes regulated by the transcription factor nuclear factor-erythroid-2-related factor 2 (Nrf2) are involved in detoxification of AFB1. In this study, we explored the potential of curcumin to prevent AFB1-induced liver injury by modulating liver phase-I and phase-II enzymes along with Nrf2 involved in AFB1 bioactivation and detoxification. Arbor Acres broiler were divided into four groups including control group (G1; fed only basal feed), curcumin alone-treated group (G2; 450 mg/kg feed), AFB1-fed group (G3; 5 mg/kg feed), and curcumin plus AFB1 group (G4; 5 mg AFB1+450 mg curcumin/kg feed). After 28 days, liver and blood samples were collected for different analyses. Histological and phenotypic results revealed that AFB1-induced liver injury was partially ameliorated by curcumin supplementation. Compared to AFB1 alone-treated group, serum biochemical parameters and liver antioxidant status showed that curcumin supplementation significantly prevented AFB1-induced liver injury. RT-PCR and western blot results revealed that curcumin inhibited CYP enzymes-mediated bioactivation of AFB1 at mRNA and protein level. Transcription factor Nrf2, its downstream genes such as GSTA3, and GSTM2 mRNA, and protein expression level significantly upregulated via dietary curcumin. In addition, GSTs enzyme activity was enhanced with dietary curcumin which plays a crucial role in AFB1-detoxification. Conclusively, the study provided a scientific basis for the use of curcumin in broiler’s diet and contributed to explore the multi-target preventive actions of curcumin against AFB1-induced liver injury through the modulation of phase-I and phase-II enzymes, and its potent anti-oxidative effects.
Introduction
Recently, several reports demonstrated that aflatoxin B1 (AFB1) is probably the most common researched mycotoxin in the world (Liu and Wu, 2010; Abrar et al., 2013). Many Aspergillus species such as Aspergillus flavus and Aspergillus parasiticus produce AFB1 (Nakai et al., 2008). It is considered to be one of the most harmful and potent aflatoxins among all other known mycotoxins (Ferenčík and Ebringer, 2003). AFB1 causes many adverse effects such as mutagenicity, carcinogenicity, hepatotoxicity, and immunotoxicity in humans, poultry and in many other animal species (Stettler and Sengstag, 2001; Kalpana et al., 2012). AFB1 has the ability to affect more than one system at a time and cause serious alterations in the normal homeostasis of the affected organism (Ferenčík and Ebringer, 2003; Herzallah, 2013). It has been placed in Group-1 human carcinogen by the International Agency for Research on Cancer (IARC) (Zuckerman, 2006). AFB1 leads to carcinogenicity in humans by p53 gene mutation at codon 249 and formation of DNA adducts (Aguilar et al., 1993, 1994). Previous reports showed that the liver is the major target organ of AFB1, as the liver plays a crucial role in drug or toxin metabolism and detoxification in the body (Grant et al., 2001; Rawal et al., 2010). AFB1 causes liver toxicity including infiltration of mononuclear and heterophilic cells and proliferation of bile duct along with changes in liver morphology, such as increase in liver weight, enlargement, congestion, necrosis, and pallor discoloration (Newberne and Butler, 1969; Hussain and Anwar, 2008), but the susceptibility of a species to AFB1 depends on various factors such as type of breed, immunity and nutritional status, age, sex, dose, length of exposure to AFB1, and managemental conditions (Richard, 2007).
The use of naturally occurring phytochemicals to combat human diseases and prevent toxicity is of growing scientific and public interest due to their potential therapeutic uses (Sharma et al., 2005). Curcumin is a polyphenol commonly composed of three types of curcuminoids, bisdemethoxycurcumin, demethoxycurcumin, and diferuloylmethane, derived from the rhizome of Curcuma longa (Common name: turmeric) famous for its pharmacological and therapeutic properties (Anand et al., 2008). Several reports have documented the anti-inflammatory, anti-oxidative, anti-cancerous, and potent chemotherapeutic properties of curcumin (Aggarwal et al., 2003; Joe et al., 2004; Liew and Mohd-Redzwan, 2018; Limaye et al., 2018; Rauf et al., 2018). It has been proved earlier that curcumin is effective against AFB1-induced oxidative stress (Gowda et al., 2009; El-Bahr, 2015). Moreover, previous studies demonstrated that curcumin modulated phase-I enzymes activity and has the potential to control AFB1-induced carcinogenesis and toxic effects (Firozi et al., 1996; Appiah-Opong et al., 2007; Nayak and Sashidhar, 2010). Cytochrome p450 (phase-I) enzymes are responsible for the biotransformation of AFB1 into its harmful metabolites (Yunus et al., 2011). Earlier reports showed that several CYP enzymes (CYP1A1, CYP1A2, CYP1A5, CYP2A6, CYP3A37, and CYP3A4) were involved in bioactivation of AFB1 into its harmful electrophilic metabolite aflatoxin-8,9-epoxide (AFBO) and to AFM1, AFP1, AFQ1, etc. (Gregorio et al., 2015). AFBO, the most potent metabolite may quickly react with DNA to form DNA adducts or cause mutations (Aguilar et al., 1993), or conjugated with phase-II enzymes such as glutathione S-transferases (GST) (Johnson et al., 2014). Importantly, the sensitivity of a species to AFB1 is not only related to AFB1 bioactivation via CYP enzymes but also related to detoxification enzymes (phase-II enzymes) (Klein et al., 2000). It is well known that the transcription factor nuclear factor-erythroid-2-related factor 2 (Nrf2) plays a crucial role in regulating several pathways, including drug metabolism, antioxidant, and detoxification such as phase-II enzymes like superoxide dismutase, catalase, NAD(P)H:quinine oxidoreductase-1, uridine 5-diphospho (UDP) glucuronosyltransferase, heme oxygenase-1 (HO-1), glutathione S-transferase, glutathione peroxidase (GPx), thioredoxin, and uridine 5-diphospho (UDP)-glucuronosyltransferase (Li et al., 2008). Besides, Slocum and Kensler’s (2011) studies demonstrated that up-regulation of Nrf2-pathway and its downstream genes (GSTs) prevented chemical carcinogenesis . Moreover, Nrf2 plays an important cytoprotective role in disease prevention, especially at the initiation stage of cancer development (Krajka-Kuźniak et al., 2017). Thus, inhibition of phase-I enzymes (involved in AFB1-bioactivation) and activation of Nrf2-pathway and its downstream genes (related to AFB1-detoxification) could be a novel pharmacological approach for the prevention of AFB1-induced liver injury and oxidative stress in broilers. The present study was designed to shed light on the multi-target effects of curcumin through inhibition of phase-I enzymes, and activation of Nrf2 as well as phase-II enzymes against AFB1-induced liver injury and ameliorating oxidative stress in Arbor Acres broiler liver. The study contributed to provide a biochemical basis for the supplementation of curcumin in broiler’s diet along with its chemopreventive action against AFB1-induced liver injury.
Materials and Methods
Ethics Statement
All the experiments were performed according to animal ethics guidelines and approved protocols of the Harbin Veterinary Research Institute of the Chinese Academy of Agricultural Sciences [SYXK (Hei) 2012-2067].
Chemicals and Reagents
Methanol, ethanol (99.0%), isopropanol, chloroform, and glycine were bought from Tian Li Company Ltd. (Tianjin, China). AFB1 (purity ≥ 99.0%) was obtained from Sigma-Aldrich (St. Louis, United States). Curcumin (2.5%) was bought from Weisheng Long Chemical Company Ltd. (China). Hematoxylin and eosin were bought from Nanjing chemical reagent factory (China).
Experimental Chickens, Diets, and Sample Collection
One-day-old healthy Arbor Acres broiler chickens were bought from Yi Nong commercial hatchery (Registration number; 230108799294096, Heilongjiang, China). The broilers were divided in four groups (15 chicks/group) and assigned to different cages. Chickens were provided with 12 h light and 12 h dark cycle and fed ad libitum for 28 days. Four dietary groups were: (G1) control group; fed only basal diet, (G2) curcumin control group: basal diet incorporated with 450 mg curcumin/kg feed; (G3) AFB1 group: basal diet contaminated with 5.0 mg AFB1/kg feed; and (G4) curcumin+AFB1 group: basal diet incorporated with 5.0 mg AFB1 and 450 mg curcumin/kg feed. After 28 days, chickens were first weighed, euthanized with sodium pentobarbital, and sacrificed in an antiseptic environment. Liver was collected, weighed and stored at -80°C for further analysis.
Serum Biochemical Analysis
Serum biochemical markers were measured to evaluate liver toxicity. 5 ml blood sample was collected from the vein wing before slaughtering of chickens. Serum was separated after centrifugation at 3000 g for 1.5 h and biochemical analysis was carried out at the same time. Serum samples were analyzed for alanine aminotransferase (ALT), aspartate aminotransferase (AST), alkaline phosphatase (ALP), and gamma-glutamyl transferase (GGT) activities in an automatic biochemical analyzer (Model 7080, Japan Hitachi Limited).
Histological Observation
Liver morphology was assessed by histopathological examination. Fresh liver tissue pieces were stained with hematoxylin and eosin (HE) as previously described (Wang X.H. et al., 2016). In brief, liver sections (1 cm × 1 cm) were fixed in 10% formalin for 24 h. The fixed liver tissues were embedded in paraffin after processed through a series of graded ethanol and dimethyl benzene. 4-μm tissue sections were mounted on glass slides, and finally stained with HE dye for microscopic examination (Light microscope; Nikon E100, 40× magnification).
Measurement of Antioxidant Enzyme Activities
A piece of fresh liver tissue (0.4–0.5 g) was immediately taken out after slaughtering and chilled in 0.85% NaCl at 0°C. It was then dried and homogenized as previously described (Soetikno et al., 2013). The homogenized solution was then centrifuged (12,000 ×g for 15 min) at 4°C and the supernatants were analyzed for antioxidant activity. All the kits were bought from Nanjing Jiancheng Bioengineering Factory (China). GSH-Px (Glutathione peroxidase, kit; A005), SOD (Superoxide dismutase, kit; A001-1) and MDA (Malonaldehyde, kit; A003-1) activities were measured in the supernatant according to the instructions of the kit.
RNA Extraction and Real-Time Quantitative Polymerase Chain Reaction (qPCR)
TRIzol (Invitrogen Inc., Carlsbad, CA) reagent was used to isolate total RNA (Cheng et al., 2017). RNA quality was verified by measuring the 260/280 ratio (Wang J. et al., 2016). Toyobo first strand cDNA synthesis kit (Osaka, Japan) was used for the synthesis of cDNA. The target genes and β-actin loading control gene specific primers are given in Table 1. The kit bought from Toyobo Company Ltd. (Japan) was utilized for RT-PCR reaction in a Roche LightCycle instrument (Shanghai, China). The data were analyzed according to 2-ΔΔCt method (Livak and Schmittgen, 2001).
Western Blot Analysis
Western blotting was performed as described previously (Lu et al., 2017). In brief, fresh liver tissues were homogenized in RIPA and PMSF. 5 × SDS-PAGE loading buffer was added, followed by protein degeneration via boiling for 5 min. The loaded protein were separated by 10 or 12% SDS-PAGE, and transferred to nitrocellulose membrane (NC). The membranes were incubated for 1 h in non-fat dry milk in TBST. CYP1A1 (1:500 diluted, Sangon Biotech. Shanghai, China), CYP1A2 (1:400 diluted, Sangon Biotech, Shanghai, China), CYP3A4 (1:200 diluted, Sangon biotech. Shanghai, China), CYP2A6 (1:200 diluted, ImmunoWay Biotechnology Company, Suzhou, China), Nrf2 (1:500 diluted, Wanleibio Co., Ltd., Harbin, China), GSTA3, (1:200 diluted, Life Span Biosciences, Inc., Shanghai, China), GSTM2 (1:800 diluted, BBI Life Sciences, Canada), and β-actin (1:1000 diluted, Applied Biological Materials Inc., Canada) or GAPDH (1:100 diluted, Applied Biological Materials Inc., Canada) protein was detected using specific primary antibodies incubated overnight at 4°C. Secondary anti-mouse or anti-rabbit horseradish peroxidase conjugated IgG was used for 1 h, and immune-complexes were detected using enhanced chemiluminescence (ECL) detection. Signals were visualized on a computer using Image J software (National Institute of Health, United States).
Statistical Analysis
The data significance were analyzed by one way ANOVA followed by LSD test using the statistical package for social science (SPSS, version 21.0), and p < 0.05 was considered statistically significant. The experiments were performed at least three times unless otherwise mentioned. The data are represented as means ± standard errors. GraphPad Prism (Version 6.01) was used to draw all the graphs with standard error bars.
Results
Curcumin Prevented AFB1-Induced Liver Injury
Liver sections from control group (Figure 1; G1) and curcumin control group (Figure 1; G2) showed normal morphology. Dietary AFB1 induced severe liver injury (Figure 1; G3) compared to control and curcumin control group. Severe to moderate lesions such as hepatic granular degeneration, lymphocyte infiltration, and hepatic steatosis were observed in AFB1-fed group. The supplementation of curcumin in the diet partially ameliorated AFB1-induced hepatic lesions (Figure 1; G4). These results confirmed the preventive effects of curcumin against AFB1-induced liver injury.
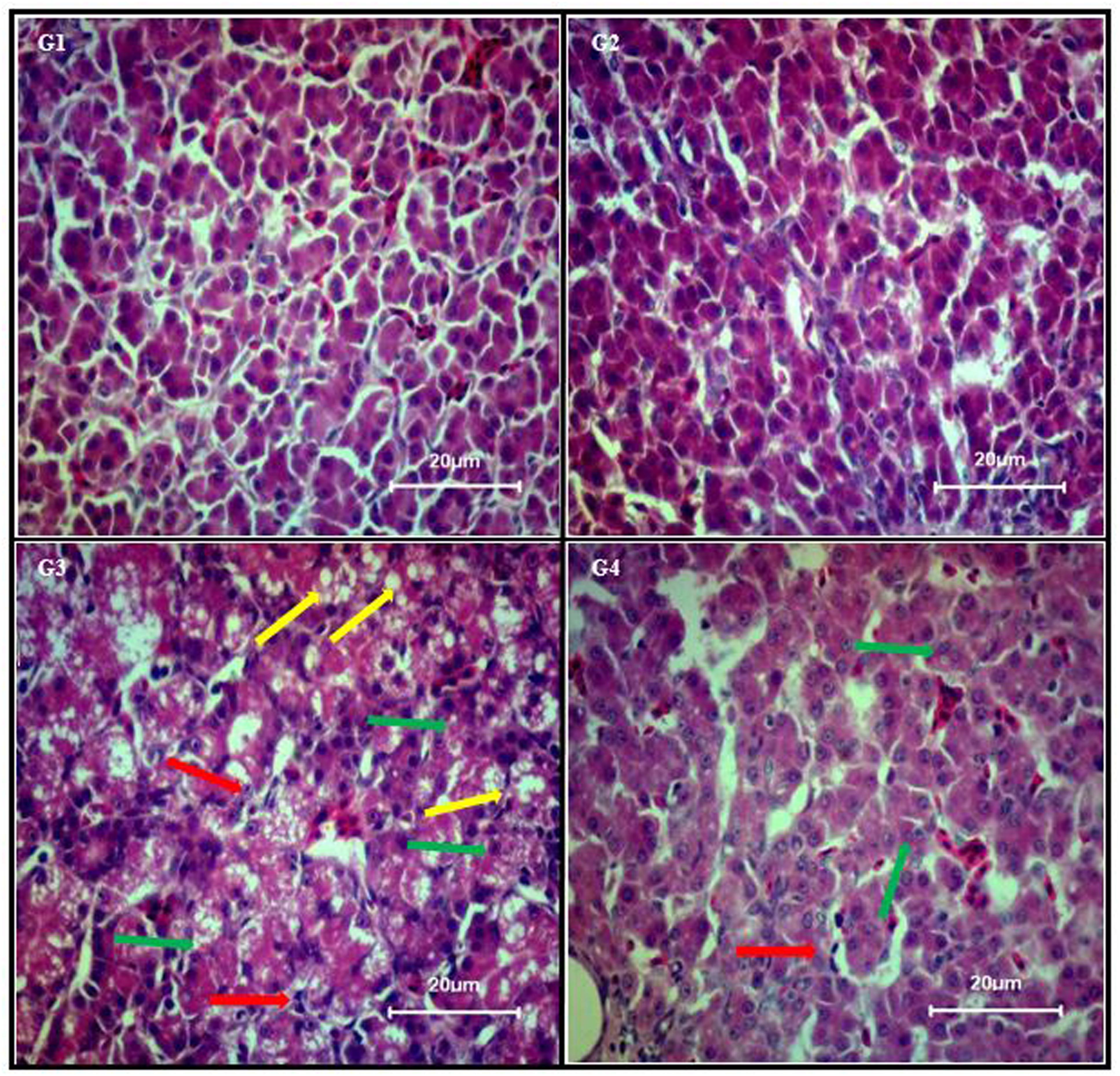
FIGURE 1. Chicken liver photomicrographs of hematoxylin and eosin-stained liver sections observed under light microscope (40X magnification), where n = 3 (H&E, scale bar = 20 μm). Treatments represented as (G1) control group; fed only basal diet, (G2) curcumin control group; basal diet + 450 mg curcumin/kg feed, (G3) AFB1 group; basal diet + 5.0 mg AFB1/kg feed, and (G4) curcumin+AFB1 group; basal diet + 5.0 mg AFB1 + 450 mg curcumin/kg feed. Yellow arrow, hepatic steatosis; red arrow, lymphocyte infiltration; and green arrow, hepatic granular degeneration.
Effect of Curcumin and AFB1 on Serum Biochemical Parameters and Body and Liver Weight
Serum biochemical parameters (ALP, ALT, AST, and GGT level) are represented in Table 2. Compared to control group (Table 2; G1), AFB1 supplemented diet (Table 2; G3) significantly (p < 0.05) affected serum ALP, ALT, AST, and GGT level, While compared to curcumin control group (Table 2; G2), AFB1-induce increase in ALT, ALP, and AST level is not statistically significant (p > 0.05) except GGT level (p < 0.05) (Table 2; G3). However, curcumin supplementation in the diet prevented AFB1-induced changes in serum biochemical parameters (Table 2; G4). The changes in serum biochemical parameters were inconsistent with the liver histological analysis (Figure 1). In addition, body weight and liver weight were recorded to study the changes in phenotypic effects (Table 2). Notably, compared to control (Table 2; G1) and curcumin control group (Table 2; G2), body weight significantly (p < 0.05) decreased in AFB1-fed group (Table 2; G3). Moreover, liver weight significantly (p < 0.05) decreased in AFB1-fed group. However, the supplementation of dietary curcumin (Table 2; G4) reversed AFB1-induced changes in body and liver weight significantly (p < 0.05).
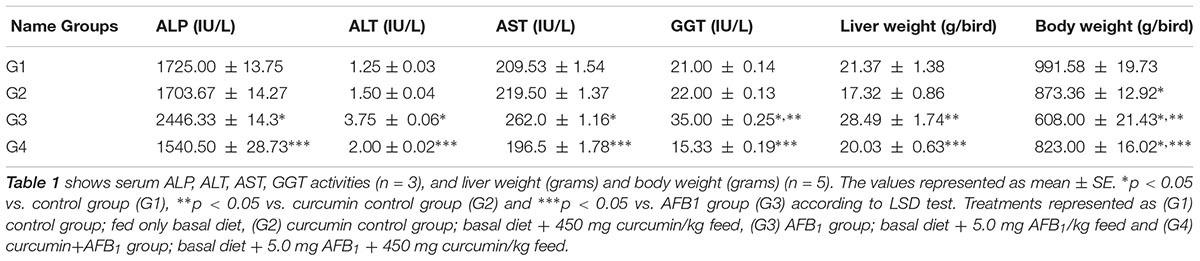
TABLE 2. Effect of curcumin and AFB1 on serum biochemical parameters, liver weight, and body weight of chickens.
Curcumin Attenuated AFB1-Induced Oxidative Stress
After 28 days, the hepatic antioxidant parameters were significantly (p < 0.05) altered by dietary AFB1 (Figure 2). Compared to control group (Figure 2; G1), GSH-Px and SOD activity were significantly (p < 0.05) decreased while MDA activity was significantly (p < 0.05) enhanced in AFB1-fed group (Figure 2; G3). The addition of curcumin alone (Figure 2; G2) increased GSH-Px activity significantly (p < 0.05) but not significantly affected SOD and MDA activity. Importantly, dietary curcumin (Figure 2; G4) significantly (p < 0.05) reversed AFB1-induced changes in antioxidant parameters. It is obvious from the results that curcumin effectively ameliorated AFB1-induced stress in broiler liver.
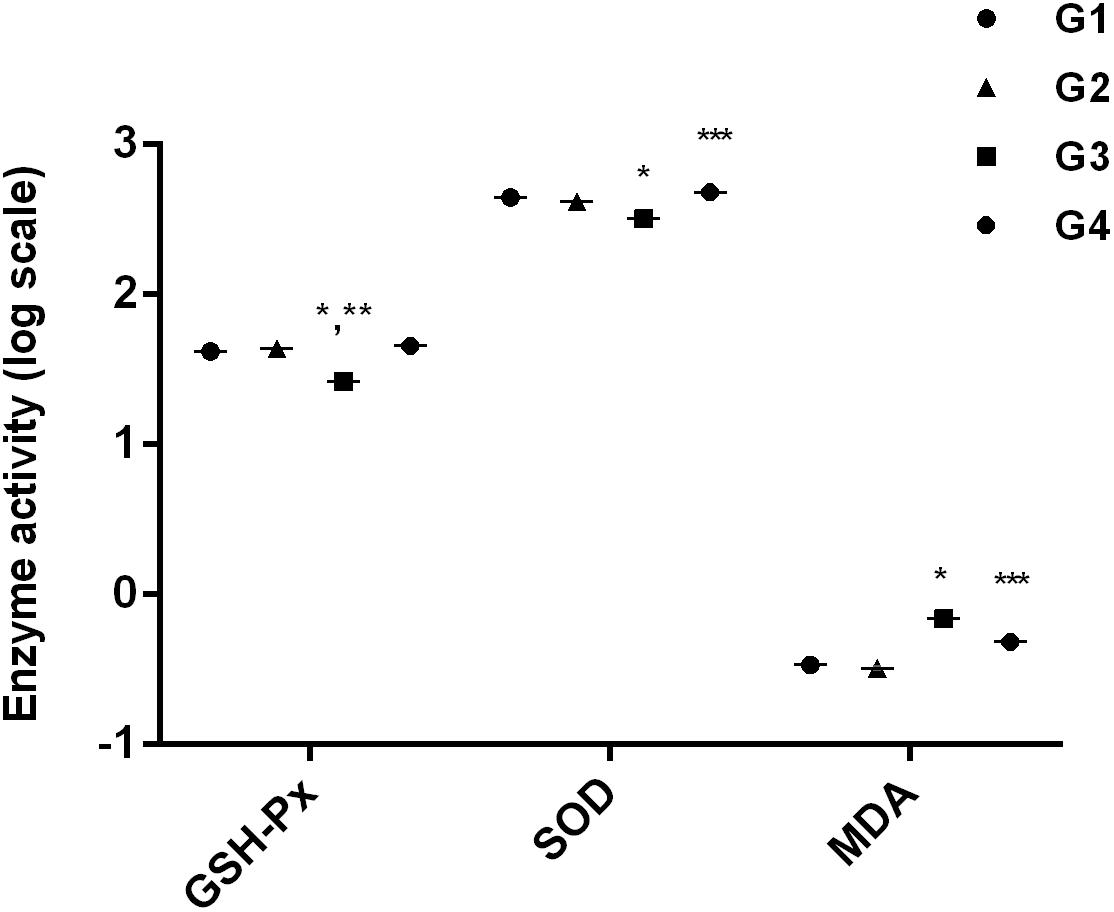
FIGURE 2. It represents anti-oxidant activities of GSH-Px activity, SOD activity, and MDA activity. The values are represented as mean ± SE (n = 5). ∗p < 0.05 vs. control group (G1), ∗∗p < 0.05 vs. curcumin control group (G2), and ∗∗∗p < 0.05 vs. AFB1 group (G3). Treatments represented as (G1) control group: fed only basal diet, (G2) curcumin control group: basal diet + 450 mg curcumin/kg feed, (G3) AFB1 group: basal diet + 5.0 mg AFB1/kg feed, and (G4) curcumin+AFB1 group: basal diet + 5.0 mg AFB1 + 450 mg curcumin/kg feed.
Inhibition of Phase-I Enzymes
The effect of curcumin, AFB1, and curcumin+AFB1 diet was investigated at the mRNA and protein level. Figure 3 shows four major CYP enzymes (CYP1A1, CYP1A2, CYP3A4, and CYP2A6) mRNA expression levels. Compared to control (Figure 3; G1) and curcumin control group (Figure 3; G2), AFB1 (Figure 3; G3) significantly (p < 0.05) upregulated the mRNA expression level of the four CYP enzymes. However, curcumin supplementation (Figure 3; G4) in diet significantly (p < 0.05) down-regulated AFB1-induced increase in CYP enzymes. Protein expression levels (Figure 4) showed the same trends as mRNA expression levels. Compared to control (Figure 4; G1) and curcumin control group (Figure 4; G2), a significant (p < 0.05) increase has been noted in all CYP enzyme protein levels in AFB1-fed group (Figure 4; G3). While curcumin supplementation (Figure 4; G4) significantly (p < 0.05) decreased the protein expression levels of CYP1A1, CYP1A2 and CYP3A4, the decrease is not statistically significant for CYP2A6 (p > 0.05) protein expression. The data clearly showed that CYP1A1, CYP1A2, CYP3A4 and CYP2A6 actively involved in AFB1 bioactivation. However, curcumin supplementation significantly down-regulated the expression level of these enzymes at mRNA and protein level, and hence inhibited CYP enzymes-mediated bioactivation of AFB1.
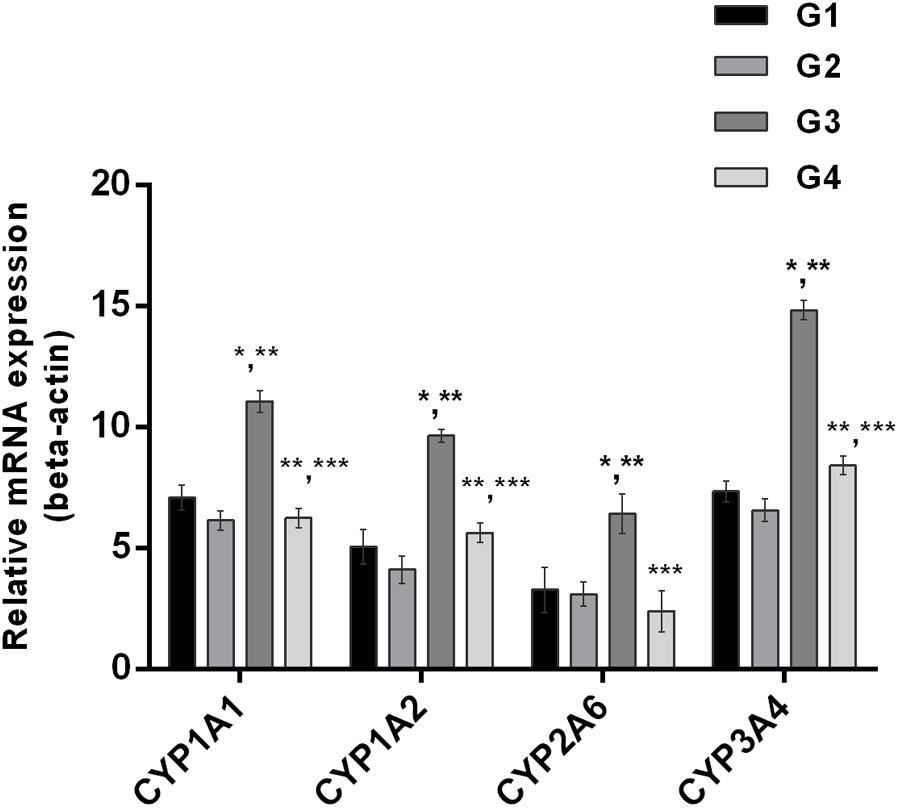
FIGURE 3. Figure shows the liver mRNA expression of CYP1A1, CYP1A2, CYP3A4, and CYP2A6 enzyme of the four treated groups. The values are represented as mean ± SE (n = 3). Treatments represented as (G1) control group: fed only basal diet, (G2) curcumin control group: basal diet + 450 mg curcumin/kg feed, (G3) AFB1 group: basal diet + 5.0 mg AFB1/kg feed, and (G4) curcumin+AFB1 group: basal diet + 5.0 mg AFB1 + 450 mg curcumin/kg feed. The value of P < 0.05 was considered statistically significant. ∗p < 0.05 vs. control group (G1), ∗∗p < 0.05 vs. curcumin control group (G2) and ∗∗∗p < 0.05 vs. AFB1 group (G3).
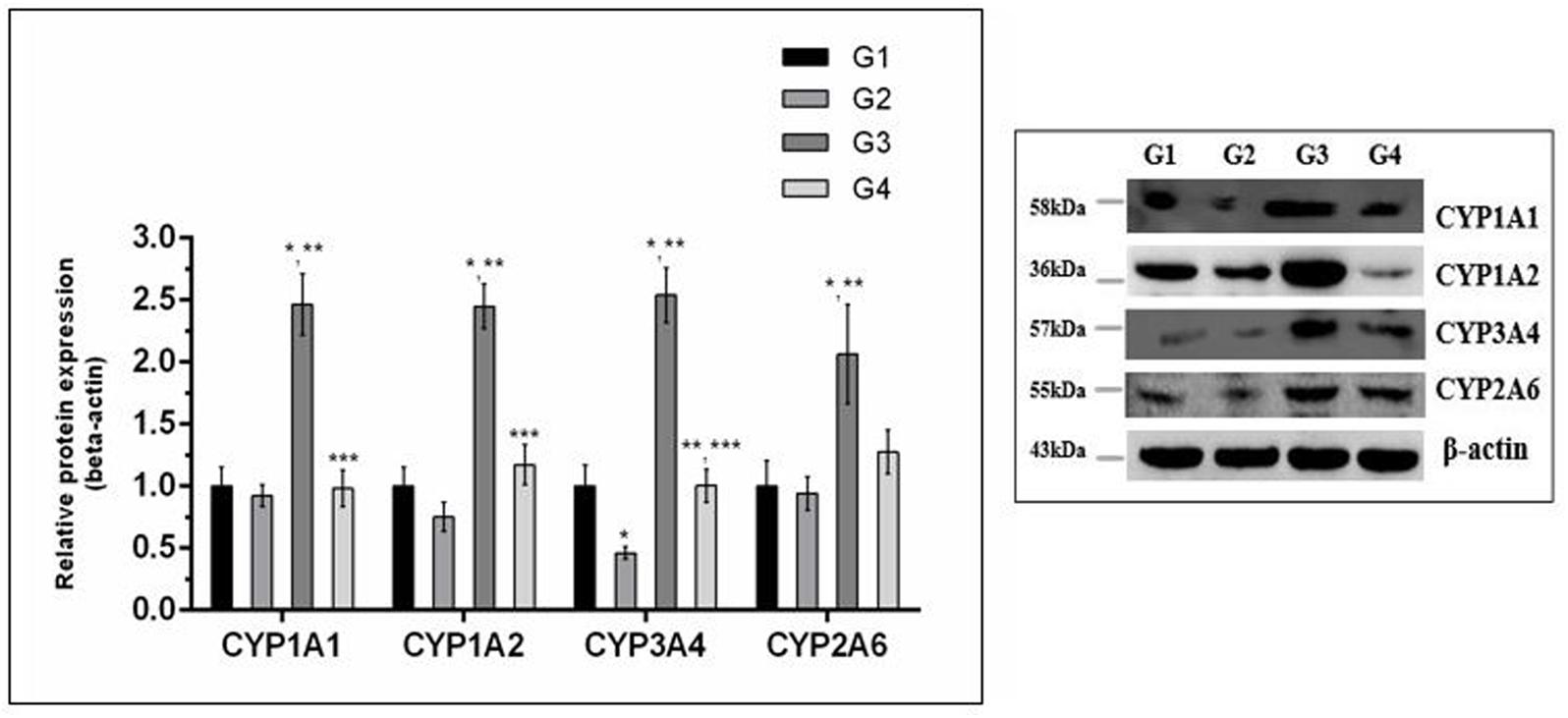
FIGURE 4. Western blot analysis of CYP enzymes involved in AFB1-bioactivation. CYP1A1, CYP1A2, CYP3A4, and CYP2A6 protein expression level of the four treated groups shown in the figure. The intensity of the protein bands was quantified by densitometry analysis. The levels of beta-actin were used as internal standard control. The values represented as mean ± SE (n = 3). Treatments represented as (G1) control group: fed only basal diet, (G2) curcumin control group: basal diet + 450 mg curcumin/kg feed, (G3) AFB1 group: basal diet + 5.0 mg AFB1/kg feed and (G4) curcumin+AFB1 group: basal diet + 5.0 mg AFB1 + 450 mg curcumin/kg feed. The value of P < 0.05 was considered statistically significant. ∗p < 0.05 vs. control group (G1), ∗∗p < 0.05 vs. curcumin control group (G2) and ∗∗∗p < 0.05 vs. AFB1 group (G3).
Curcumin Induced the Transcriptional Activation of Nrf2 and Phase-II Enzymes
It is well understood that Nrf2 plays a crucial role in the defense system of a body and is an important regulator of several cellular pathways including phase-II enzymes involved in the detoxification of AFB1. Here, we examined the changes induced by diets supplemented with AFB1 and curcumin alone or in combination on Nrf2, GSTA3, and GSTM2 at mRNA and protein level. The mRNA expression level of Nrf2, GSTA3, and GSTM2 are displayed in Figure 5. Compared to control (Figure 5; G1) and curcumin control group (Figure 5; G2), AFB1 (Figure 5; G3) significantly (p < 0.05) down-regulated Nrf2, GSTA3, and GSTM2 mRNA expression level. Interestingly, curcumin significantly (p < 0.05) enhanced AFB1-induced decrease in Nrf2, GSTA3, and GSTM2 mRNA expression level (Figure 5; G4). Similar trends were observed in Nrf2, GSTA3, and GSTM2 protein expression level (Figure 6A). Notably, Nrf2, GSTA3, and GSTM2 protein expression level has been significantly (p < 0.05) reduced in AFB1-fed group (Figure 6A; G3) relative to control (Figure 6A; G1) and curcumin control group (Figure 6A; G2). On the other hand, curcumin supplemented diet (Figure 6A; G4) significantly (p < 0.05) restored AFB1-induced decrease in Nrf2 and GSTA3, protein level. However, the increase in GSTM2 (Figure 6A; G4) is not statistically significant relative to AFB1-fed group (Figure 6A; G3). The data showed that curcumin significantly upregulated the transcription factor Nrf2 and subsequently its downstream genes such as phase-II enzymes (GSTA3, and GSTM2), and thus improves the defense system against AFB1-induced toxicity.
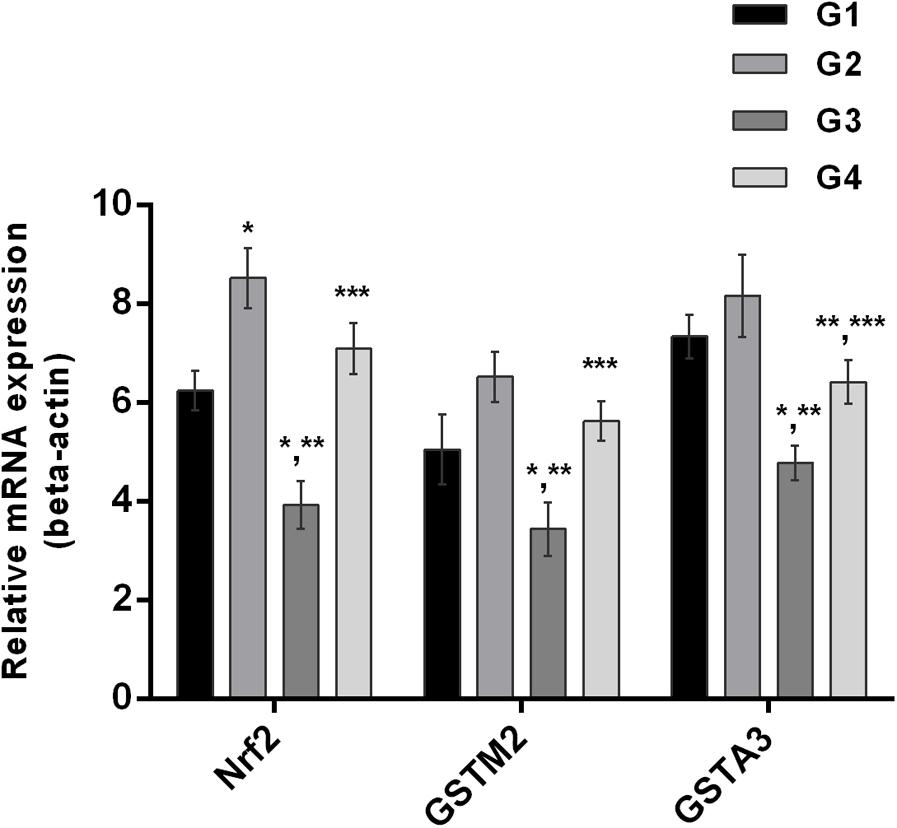
FIGURE 5. Nrf2, GSTA3, and GSTM2 mRNA expression level of the four treated groups shown in the figure. Treatments represented as (G1) control group: fed only basal diet, (G2) curcumin control group: basal diet + 450 mg curcumin/kg feed, (G3) AFB1 group: basal diet + 5.0 mg AFB1/kg feed and (G4) curcumin+AFB1 group: basal diet + 5.0 mg AFB1 + 450 mg curcumin/kg feed. The values represented as mean ± SE (n = 3). The value of P < 0.05 was considered statistically significant. ∗p < 0.05 vs. control group (G1), ∗∗p < 0.05 vs. curcumin control group (G2) and ∗∗∗p < 0.05 vs. AFB1 group (G3).
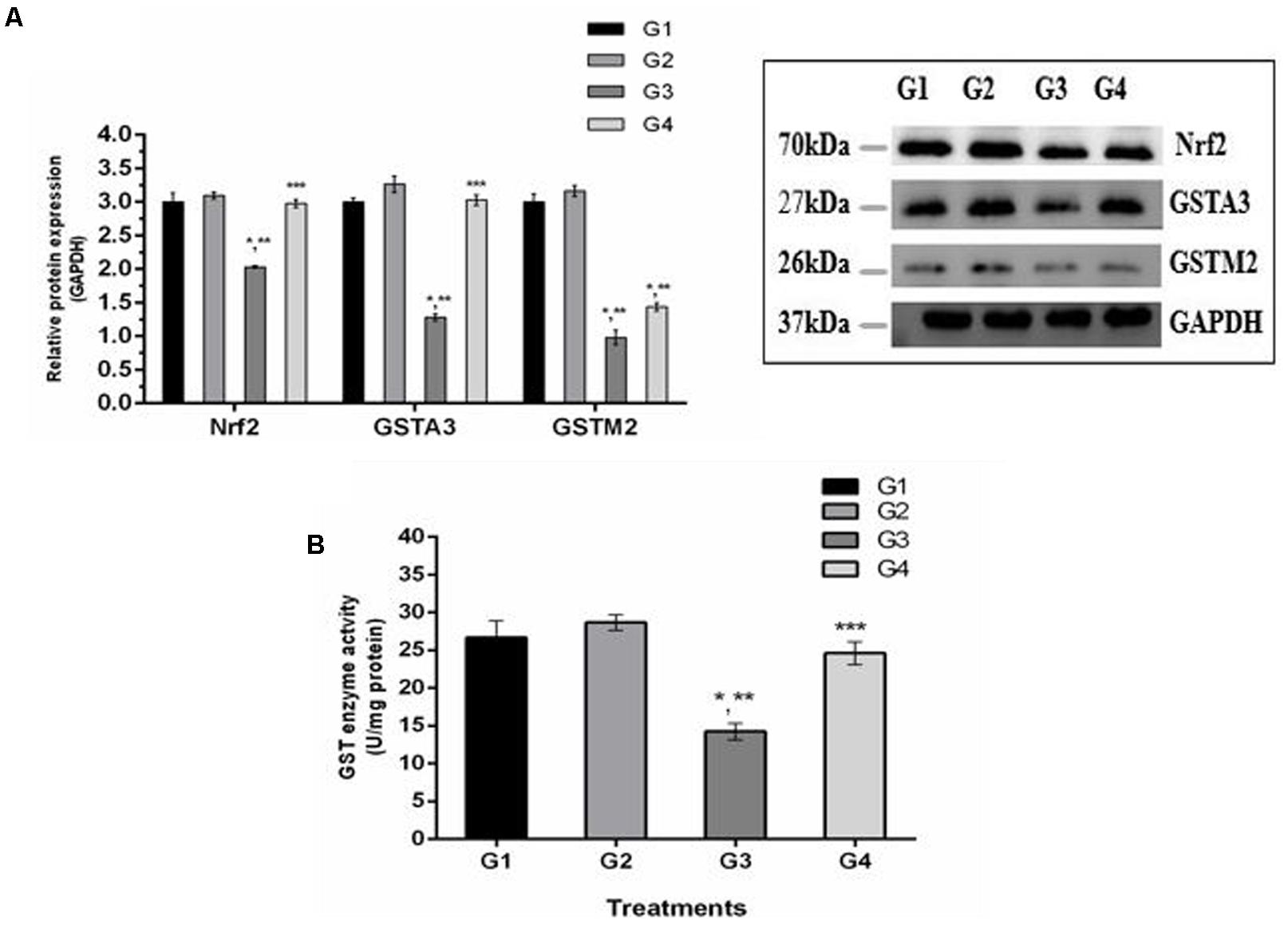
FIGURE 6. Western blot analysis of Nrf2, GSTA3, and GSTM2 involved in AFB1-detoxification. (A) Nrf2, GSTA3, and GSTM2 protein expression level of the four treated groups shown. The intensity of the protein bands was quantified by densitometry analysis. The levels of GAPDH gene were used as internal standard control. The values represented as mean ± SE (n = 3). (B) Cytosolic GST enzyme activity (U/mg protein) of the four treated groups presented in the figure. Treatments represented as (G1) control group: fed only basal diet, (G2) curcumin control group: basal diet + 450 mg curcumin/kg feed, (G3) AFB1 group: basal diet + 5.0 mg AFB1/kg feed and (G4) curcumin+AFB1 group: basal diet + 5.0 mg AFB1 + 450 mg curcumin/kg feed. The value of P < 0.05 was considered statistically significant. ∗p < 0.05 vs. control group (G1), ∗∗p < 0.05 vs. curcumin control group (G2) and ∗∗∗p < 0.05 vs. AFB1 group (G3).
Hepatic GST Enzyme Activity
GST enzymes play an important role in the detoxification (conjugation) of AFB1 metabolites. Figure 6B displays GST enzyme activity detected in liver cytosol. It has been noted that AFB1-supplemented diet (G3) significantly (p < 0.05) reduced GST enzyme activity compared to control group (Figure 6B; G1) and curcumin control group (Figure 6B; G2). However, GST activity was markedly (p < 0.05) elevated in curcumin+AFB1-fed group (Figure 6B; G4) relative to AFB1-fed group (Figure 6B; G3). These findings showed that curcumin actively increased GST enzyme activity to catalyze the harmful metabolites of AFB1.
Discussion
Numerous studies reported that AFB1 is a well-known hepatotoxic and hepatocarcinogen and affects many systems at a time (Rawal et al., 2010). The current study showed that AFB1 significantly modulated serum biochemical parameters. Liver histological observations showed abnormal morphological signs in AFB1-treated group, while oxidative stress parameters clearly revealed that AFB1 induced oxidative stress in hepatocytes. Liver weight markedly increased and body weight was significantly suppressed in the current study in AFB1-fed group. These abnormal findings related to AFB1 toxicity were in general agreement with previous studies (Kumar and Balachandran, 2009; Ibrahim, 2013; El-Bahr, 2015; Muhammad et al., 2017). Notably, our data showed that dietary supplementation of curcumin ameliorated AFB1-induced oxidative stress and reversed serum biochemical changes. AFB1-induced abnormal morphological signs in the liver partially disappear with curcumin treatment. The above findings were in agreement with some earlier reports that curcumin significantly mitigated AFB1-induced toxicity in broiler liver (Gowda et al., 2008, 2009). It has been reported earlier that CYP1A1, CYP1A2, CYP2A6, and CYP3A4 are responsible for the bioactivation of AFB1 in poultry species (ducks, quails, turkeys and chickens) (Diaz et al., 2010a,b). In this study, liver CYP1A1, CYP1A2, CYP3A4, and CYP2A6 were significantly upregulated in AFB1-fed group showing its involvement in AFB1 biotransformation into harmful metabolites. Interestingly, the inhibition of these enzymes may be a promising treatment to prevent AFB1-induced liver toxicity. Importantly, the use of curcumin markedly reduced the mRNA and protein expression levels of the selected CYP enzymes in broiler liver in vivo. Our previous study demonstrated that curcumin inhibited CYP2A6 enzyme activity (in a dose dependent manner) involved in AFB1-bioactivation (Muhammad et al., 2017). Zhang et al. (2016) also reported that curcumin significantly inhibited CYP enzymes-mediated bioactivation of AFB1 into AFBO. Similarly, it has been noted that AFB1-induced increase expression of chicken CYP2H1 and CYP1A1 was alleviated with dietary curcumin (Yarru et al., 2009). Moreover, curcumin attenuated Benzo[a]Pyrene-induced oxidative stress and reduced DNA adducts in mice via inhibiting CYP1A1/1A2 activities (in vivo) (Garg et al., 2008), decreased CYP3A activity in rats (Kim et al., 2015), and modulated CYP1A2, CYP3A4, CYP2B6, CYP2C9, and CYP2D6 activity in humans (Appiah-Opong et al., 2007). Thus, curcumin could possibly prevent AFB1-induced hepatotoxicity though the synergistic effects of increased antioxidant capacities and inhibition of the cytochrome enzymes mediated activation to AFBO. However, the mechanism behind cytochrome P450 enzymes inhibition is still unknown and further molecular studies are needed to know the exact molecular mechanism of curcumin-mediated CYP450 inhibition.
Transcription factor Nrf2 is an attractive target in toxin chemoprevention due to its cytoprotective response and combat oxidative stress leading to prevention of DNA damage, carcinogenesis, and removal of toxic metabolites from the body (Slocum and Kensler, 2011). Previous reports demonstrated that curcumin is a potent inducer of Nrf2-pathway and its downstream genes involved in many cytoprotective mechanisms (Sahin et al., 2012; Soetikno et al., 2013). Nrf2 plays a crucial role in chemoprevention and reduce liver injury via up-regulating GST enzymes responsible for the detoxification of AFB1 harmful metabolites such as AFBO (Poapolathep et al., 2015), and meanwhile upregulate antioxidant defense system (Soetikno et al., 2013). The nuclear translocation of AFB1 is associated with many cellular proteins carrying signals for its translocation and subsequent binding to DNA, which results in DNA damage or causes deterioration of cellular proteins (Ricordy et al., 2002). Intriguingly, AFB1-induced impairment of specific protein functions or DNA damage decreased cell survival in cultured cells (Besaratinia et al., 2009). Previous studies demonstrated that protein biosynthesis is weakened due to the formation of AFB1-DNA adducts. In addition, AFB1 could change the activity of enzymes such as cyclic nucleotide phosphodiesterase, protein kinase, and Ca2+-ATPase, alter hepatic protein phosphorylation (Mistry et al., 1996; Ricordy et al., 2002), and causes p53 mutation which may develop carcinogenesis in experimental animals (Aguilar et al., 1994). Similarly, our data showed that Nrf2 mRNA and protein expression level significantly down-regulated in AFB1-fed group. Consequently, the downstream genes of Nrf2 such as GSTA3, and GSTM2 mRNA and protein expression levels markedly decreased in AFB1-fed group. Hence, it is speculated that the reduction in protein level could be due to AFB1-induced impairment in protein synthesis in broiler hepatocytes. Meanwhile, curcumin supplementation reversed AFB1-induced decrease in Nrf2, GSTA3, and GSTM2 mRNA and protein expression level. Furthermore, curcumin markedly increased GST enzyme activity involved in detoxification (conjugation) of AFB1. Similarly, previous studies reported that GST enzymes play a major role in prevention of AFB1-induced toxic effects and carcinogenesis (Larsson et al., 1994), and could prevent AFB1-induced hepatic carcinoma in fisher F344 rats via Nrf2-pathway (Eaton and Schaupp, 2014). We found that curcumin could inhibit cytochrome P450 enzymes-mediated bioactivation of AFB1 along with activation of Nrf2-pathway. In addition, our data showed that curcumin alleviated oxidative stress and caused alteration in AFB1-induced changes in serum biochemical parameters. These evidences suggested that curcumin could effectively inhibit AFB1-induced toxicity in broilers liver. Recently, many studies reported the chemopreventive effects of curcumin against AFB1-induced liver injury in several experimental animals (Larsson et al., 1994; Gowda et al., 2008; Yarru et al., 2009; Poapolathep et al., 2015; Zhang et al., 2016). However, these studies only investigated the preventive effects of curcumin through phase-I enzymes or phase-II enzymes against AFB1-induced liver injury. In contrast, in this study, we scrutinized the multi-target effects of curcumin regulating both phase-I enzymes and phase-II enzymes via Nrf2-pathway involved in AFB1 bioactivation and detoxification. It has been noted from our previous study that 450 mg/kg curcumin provided maximum amelioration and significantly inhibited CYP2A6-mediated AFB1 bioactivation (Muhammad et al., 2017). Therefore, in this study, we selected the dose of curcumin (450 mg/kg feed) to explore its preventive effects against dietary AFB1 at a dose of 5 mg/kg feed. However, previous studies reported that curcumin is regarded a safe compound at a dose of 12 g/day (Basak et al., 2015), acts as anti-inflammatory, anti-tumor, and anti-oxidant, etc. (Abdel-Wahhab, 2015), regulates pharmacological activities in a direct or indirect way and modulates various signaling molecules including cell survival proteins, apoptosis, drug resistance protein, etc. (Basak et al., 2015; Abdel-Wahhab et al., 2016), and possess the ability to scavenge free radicals (Trujillo et al., 2013). Our data also showed that curcumin prevented liver dysfunction due to its anti-oxidant activity as well as modulating drug metabolizing enzymes including liver phase-I enzymes, Nrf2, and phase-II enzymes. In addition, higher studies are needed to investigate the preventive effects of curcumin and AFB1-induced undesirable changes in other cellular pathways such as cell cycle arrest, autophagy, apoptosis and cell death.
Conclusion
In conclusion, we showed that dietary AFB1 negatively altered serum biochemical parameters, reduced activities of liver anti-oxidative enzymes, suppressed Nrf2 and phase-II enzymes involved in AFB1 detoxification, and markedly increased the expression level of CYP enzymes involved in AFB1 biotransformation, while dietary curcumin significantly reversed AFB1-induced alterations in serum biochemical parameters and enhanced liver antioxidant enzyme activities. Moreover, curcumin inhibited CYP enzymes-mediated bioactivation of AFB1 and upregulated Nrf2 defense system including GST enzyme activity. Therefore, the above novel findings suggested that dietary curcumin is recommended as a prophylactic measure to prevent AFB1-induced hepatocellular toxicity and oxidative stress.
Author Contributions
XZ supervised all the experiments. IM contributed to paper writing. HW, XS, and MH performed the practical work and completed the experiments. XW, ZL, PC, and MAH provided help during experiments.
Funding
The National Natural Science Foundation of China (registration number: 31172369) and the Natural Science Foundation of Heilongjiang Province (registration number: ZD201405) provided funding for this work.
Conflict of Interest Statement
MH was employed by Changchun Dirui Medical Company Ltd., No. 3333 liju road, Changchun, China.
The other authors declare that the research was conducted in the absence of any commercial or financial relationships that could be construed as a potential conflict of interest.
References
Abdel-Wahhab, M. A. (2015). Ameliorative effects of curcumin nanoparticles on hepatotoxicity induced by zearalenone mycotoxin. Glob. J. Pharmacol. 9, 234–245.
Abdel-Wahhab, M. A., Salman, A. S., Ibrahim, M. I., El-Kady, A. A., Abdel-Aziem, S. H., Hassan, N. S., et al. (2016). Curcumin nanoparticles loaded hydrogels protects against aflatoxin B1-induced genotoxicity in rat liver. Food Chem. Toxicol. 94, 159–171. doi: 10.1016/j.fct.2016.06.005
Abrar, M., Anjum, F. M., Butt, M. S., Pasha, I., Randhawa, M. A., Saeed, F., et al. (2013). aflatoxins: biosynthesis, occurrence, toxicity, and remedies. Crit. Rev. Food Sci. Nutr. 53, 862–874. doi: 10.1080/10408398.2011.563154
Aggarwal, B. B., Kumar, A., and Bharti, A. C. (2003). Anticancer potential of curcumin: preclinical and clinical studies. Anticancer Res. 2003, 363–398.
Aguilar, F., Harris, C., Sun, T., Hollstein, M., and Cerutti, P. (1994). Geographic variation of p53 mutational profle in nonmalignant human liver. Science 264, 1317–1319. doi: 10.1126/science.8191284
Aguilar, F., Hussain, S. P., and Cerutti, P. (1993). Aflatoxin B1 induces the transversion of G– > T in codon 249 of the p53 tumor suppressor gene in human hepatocytes. Proc. Natl. Acad. Sci. U.S.A. 90, 8586–8590. doi: 10.1073/pnas.90.18.8586
Anand, P., Sundaram, C., Jhurani, S., Kunnumakkara, A. B., and Aggarwal, B. B. (2008). Curcumin and cancer: an “old-age” disease with an “age-old” solution. Cancer Lett. 267, 133–164. doi: 10.1016/j.canlet.2008.03.025
Appiah-Opong, R., Commandeur, J. N., van Vugt-Lussenburg, B., and Vermeulen, N. P. (2007). Inhibition of human recombinant cytochrome P450s by curcumin and curcumin decomposition products. Toxicology 235, 83–91. doi: 10.1016/j.tox.2007.03.007
Basak, S. K., Zinabadi, A., Wu, A. W., Venkatesan, N., Duarte, V. M., Kang, J. J., et al. (2015). Liposome encapsulated curcumin-difluorinated (CDF) inhibits the growth of cisplatin resistant head and neck cancer stem cells. Oncotarget 6, 18504–18517. doi: 10.18632/oncotarget.4181
Besaratinia, A., Kim, S. I., Hainaut, P., and Pfeifer, G. P. (2009). In vitro recapitulating of TP53 mutagenesis in hepatocellular carcinoma associated with dietary aflatoxin B1 exposure. Gastroenterology 137, 1127–1137. doi: 10.1053/j.gastro.2009.06.002
Cheng, P., Wang, T., Li, W., Muhammad, I., Wang, H., Sun, X., et al. (2017). Baicalin alleviates lipopolysaccharide-induced liver inflammation in chicken by suppressing TLR4-mediated NF-κB pathway. Front. Pharmacol. 8:547. doi: 10.3389/fphar.2017.00547
Diaz, G. J., Murcia, H. W., and Cepeda, S. M. (2010a). Bioactivation of aflatoxin B1 by turkey liver microsomes: responsible cytochrome P450 enzymes. Br. Poult. Sci. 51, 828–837. doi: 10.1080/00071668.2010.528752
Diaz, G. J., Murcia, H. W., and Cepeda, S. M. (2010b). Cytochrome P450 enzymes involved in the metabolism of aflatoxin B-1 in chickens and quail. Poult. Sci. 89, 2461–2469. doi: 10.3382/ps.2010-00864
Eaton, D. L., and Schaupp, C. M. (2014). Of mice, rats, and men: could Nrf2 activation protect against aflatoxin heptocarcinogenesis in humans? Cancer Prev. Res. 7, 653–657. doi: 10.1158/1940-6207.CAPR-14-0119
El-Bahr, S. M. (2015). Effect of curcumin on hepatic antioxidant enzymes activities and gene expressions in rats intoxicated with aflatoxin B1. Phytother. Res. 2015, 134–140. doi: 10.1002/ptr.5239
Ferenčík, M., and Ebringer, L. (2003). Modulatory effects of selenium and zinc on the immune system. Folia Microbiol. 48, 417–426. doi: 10.1007/BF02931378
Firozi, P. F., Aboobaker, V. S., and Bhattacharya, R. K. (1996). Action of curcumin on the cytochrome P450-system catalyzing the activation of aflatoxin B1. Chem. Biol. Interact. 100, 41–51. doi: 10.1016/0009-2797(95)03684-9
Garg, R., Gupta, S., and Maru, G. B. (2008). Dietary curcumin modulates transcriptional regulators of phase I and phase II enzymes in benzo[a]pyrene-treated mice: mechanism of its anti-initiating action. Carcinogenesis 29, 1022–1032. doi: 10.1093/carcin/bgn064
Gowda, N. K., Ledoux, D. R., Rottinghaus, G. E., Bermudez, A. J., and Chen, Y. C. (2008). Efficacy of turmeric (Curcuma longa), containing a known level of curcumin, and a hydrated sodium calcium aluminosilicate to ameliorate the adverse effects of aflatoxin in broiler chicks. Poult. Sci. 87, 1125–1130. doi: 10.3382/ps.2007-00313
Gowda, N. K., Ledoux, D. R., Rottinghaus, G. E., Bermudez, A. J., and Chen, Y. C. (2009). Antioxidant efficacy of curcuminoids from turmeric (Curcuma longa L.) powder in broiler chickens fed diets containing aflatoxin B1. Br. J. Nutr. 102, 1629–1634. doi: 10.1017/S0007114509990869
Grant, D., Mendicino, M., and Levy, G. (2001). Xenotransplantation: Just around the corner? Surgery 129, 243–247. doi: 10.1067/msy.2001.118380
Gregorio, M. C. D., Bordin, K., de Castro Souto, P. C. M., Corassin, C. H., and Fernandes Oliveira, C. A. (2015). Comparative biotransformation of aflatoxin B1 in swine, domestic fowls, and humans. Toxin Rev. 34, 142–150. doi: 10.3109/15569543.2015.1091979
Herzallah, S. M. (2013). Aflatoxin B1 residues in eggs and flesh of laying hens fed aflatoxin B1 contaminated diet. Am. J. Agric. Biol. Sci. 8, 156–161. doi: 10.3844/ajabssp.2013.156.161
Hussain, I., and Anwar, J. (2008). A study on contamination of aflatoxin M1 in raw milk in the Punjab province of Pakistan. Food Control 19, 393–395. doi: 10.1016/j.foodcont.2007.04.019
Ibrahim, Q. (2013). Histopathological study of Quails liver experimentally induced by aflatoxin. Braz. J. Vet. Res. 12, 116–127.
Joe, B., Vijaykumar, M., and Lokesh, B. R. (2004). Biological properties of curcumin – cellular and molecular mechanisms of action. Crit. Rev. Food Sci. Nutr. 44, 97–111. doi: 10.1080/10408690490424702
Johnson, N. M., Egner, P. A., Baxter, V. K., Sporn, M. B., Wible, R. S., Sutter, T. R., et al. (2014). Complete protection against aflatoxin B(1)-induced liver cancer with a triterpenoid: DNA adduct dosimetry, molecular signature, and genotoxicity threshold. Cancer Prev. Res. 7, 658–665. doi: 10.1158/1940-6207.CAPR-13-0430
Kalpana, S., Aggarwal, M., Rao, G. S., and Malik, J. K. (2012). Effects of aflatoxin B1 on tissue residues of enrofloxacin and its metabolite ciprofloxacin in broiler chickens. Environ. Toxicol. Pharmacol. 33, 121–126. doi: 10.1016/j.etap.2011.11.005
Kim, S., Cho, S., Cho, H., and Yoon, I. (2015). Modulation of hepatic cytochrome P450 enzymes by curcumin and its pharmacokinetic consequences in sprague-dawley rats. Pharmacogn. Mag. 114, S580–S584. doi: 10.4103/0973-1296.172965
Klein, P. J., Buckner, R., Kelly, J., and Coulombe, R. A. Jr. (2000). Biochemical basis for the extreme sensitivity of turkeys to aflatoxin B(1). Toxicol. Appl. Pharmacol. 165, 45–52. doi: 10.1006/taap.2000.8926
Krajka-Kuźniak, V., Paluszczak, J., and Baer-Dubowska, W. (2017). The Nrf2-ARE signaling pathway: an update on its regulation and possible role in cancer prevention and treatment. Pharmacol. Rep. 69, 393–402. doi: 10.1016/j.pharep.2016.12.011
Kumar, R., and Balachandran, C. (2009). Histopathological changes in broiler chickens fed aflatoxin and cyclopiazonic acid. Vet. Arhiv 79, 31–40.
Larsson, P., Busk, L., and Tjalve, H. (1994). Hepatic and extrahepatic bioactivation and GSH conjugation of aflatoxin B1 in sheep. Carcinogenesis 15, 947–955. doi: 10.1093/carcin/15.5.947
Li, W., Khor, T. O., Xu, C., Shen, G., Jeong, W. S., Yu, S., et al. (2008). Activation of Nrf2-antioxidant signaling attenuates NFkappaB-inflammatory response and elicits apoptosis. Biochem. Pharmacol. 76, 1485–1489. doi: 10.1016/j.bcp.2008.07.017
Liew, W.-P., and Mohd-Redzwan, S. (2018). Mycotoxin: its impact on gut health and microbiota. Front. Cell. Infect. Microbiol. 8:60. doi: 10.3389/fcimb.2018.00060
Limaye, A., Yu, R. C., Chou, C. C., Liu, J. R., and Cheng, K. C. (2018). Protective and detoxifying effects conferred by dietary selenium and curcumin against AFB1-mediated toxicity in livestock: a review. Toxins 10:25. doi: 10.3390/toxins10010025
Liu, Y., and Wu, F. (2010). Global burden of aflatoxin-induced hepatocellular carcinoma: a risk assessment. Environ. Health Perspect. 118, 818–824. doi: 10.1289/ehp.0901388
Livak, K. J., and Schmittgen, T. D. (2001). Analysis of relative gene expression data using real time quantitative PCR and the 2 (-Delta Delta C (T)) method. Methods 25, 402–408. doi: 10.1006/meth.2001.1262
Lu, Z., Miao, Y., Muhammad, I., Tian, E., Hu, W., Wang, J., et al. (2017). JNK mediated colistin-induced autophagy and apoptosis via JNK-Bcl2-Bax signaling pathway and JNK-p53-ROS positive feedback loop in PC 12 cells. Chem. Biol. Interact. 277, 62–73. doi: 10.1016/j.cbi.2017.08.011
Mistry, K. J., Krishna, M., Pasupathy, K., Murthy, V., and Bhattacharya, R. K. (1996). Signal transduction mechanism in response to aflatoxin B1 exposure: protein kinase C activity. Chem. Biol. Interact. 100, 177–185. doi: 10.1016/0009-2797(96)03698-8
Muhammad, I., Sun, X., Wang, H., Li, W., Wang, X., Cheng, P., et al. (2017). Curcumin successfully inhibited the computationally identified CYP2A6 enzyme-mediated bioactivation of aflatoxin B1 in arbor acres broiler. Front. Pharmacol. 8:143. doi: 10.3389/fphar.2017.00143
Nakai, V. K., Rocha, L. D. O., Gonçalez, E., Fonseca, H., Ortega, E. M. M., and Correa, B. (2008). Distribution of fungi and aflatoxins in a stored peanut variety. Food Chem. 106, 285–290. doi: 10.1016/j.foodchem.2007.05.087
Nayak, S., and Sashidhar, R. B. (2010). Metabolic intervention of aflatoxin B1 toxicity by curcumin. J. Ethnopharmacol. 17 127, 641–644. doi: 10.1016/j.jep.2009.12.010
Newberne, P. M., and Butler, W. H. (1969). Acute and chronic effects of aflatoxin on the liver of domestic and laboratory animals: a review. Cancer Res. 29, 236–250.
Poapolathep, S., Imsilp, K., Machii, K., Kumagai, S., and Poapolathep, A. (2015). The effects of curcumin on aflatoxin B1- induced toxicity in rats. Biocontrol Sci. 20, 171–177. doi: 10.4265/bio.20.171
Rauf, A., Imran, M., Orhanc, I. E., and Bawazeer, B. (2018). Health perspectives of a bioactive compound curcumin: a review. Trends Food Sci. Technol. 74, 33–45. doi: 10.1016/j.tifs.2018.01.016
Rawal, S., Ji, E. K., and Coulombe, R. (2010). Aflatoxin B1 in poultry: toxicology, metabolism and prevention. Res. Vet. Sci. 89, 325–331. doi: 10.1016/j.rvsc.2010.04.011
Richard, J. L. (2007). Some major mycotoxins and their mycotoxicoses—An overview. Int. J. Food Microbiol. 119, 3–10. doi: 10.1016/j.ijfoodmicro.2007.07.019
Ricordy, R., Gensabella, G., Cacci, E., and Augusti-Tocco, G. (2002). Impairment of cell cycle progression by aflatoxin B1 in human cell lines. Mutagenesis 17, 241–249. doi: 10.1093/mutage/17.3.241
Sahin, K., Orhan, C., Tuzcu, Z., Tuzcu, M., and Sahin, N. (2012). Curcumin ameloriates heat stress via inhibition of oxidative stress and modulation of Nrf2/HO-1 pathway in quail. Food Chem. Toxicol. 50, 4035–4041. doi: 10.1016/j.fct.2012.08.029
Sharma, R. A., Gescher, A. J., and Steward, W. P. (2005). Curcumin: The story so far. Eur. J. Cancer 41, 1955–1968. doi: 10.1016/j.ejca.2005.05.009
Slocum, S. L., and Kensler, T. W. (2011). Nrf2: control of sensitivity to carcinogens. Arch. Toxicol. 85, 273–284. doi: 10.1007/s00204-011-0675-4
Soetikno, V., Sari, F. R., Lakshmanan, A. P., Arumugam, S., Harima, M., Suzuki, K., et al. (2013). Curcumin alleviates oxidative stress, inflammation, and renal fibrosis in remnant kidney through the Nrf2-keap1 pathway. Mol. Nutr. Food Res. 57, 1649–1659. doi: 10.1002/mnfr.201200540
Stettler, P., and Sengstag, C. (2001). Liver carcinogen aflatoxin B1 as an inducer of mitotic recombination in a human cell line. Mol. Carcinog. 31, 125–138. doi: 10.1002/mc.1047
Trujillo, J., Chirino, Y. I., Molina-Jijon, E., Anderica-Romero, A. C., Tapia, E., and Pedraza-Chaverri, J. (2013). Renoprotective effect of the antioxidant curcumin: recent findings. Redox Biol. 1, 448–456. doi: 10.1016/j.redox.2013.09.003
Wang, J., Yi, M., Chen, X., Muhammad, I., Liu, F., Li, R., et al. (2016). Effects of colistin on amino acid neurotransmitters and blood-brain barrier in the mouse brain. Neurotoxicol. Teratol. 55, 32–37. doi: 10.1016/j.ntt.2016.03.004
Wang, X. H., Li, C. Y., Muhammad, I., and Zhang, X. Y. (2016). Fatty acid composition in serum correlates with that in the liver and nonalcoholic fatty liver disease activity scores in mice fed a high-fat diet. Environ. Toxicol. Pharmacol. 44, 140–150. doi: 10.1016/j.etap.2016.04.009
Yarru, L. P., Settivari, R. S., Gowda, N. K. S., Antoniou, E., Ledoux, D. R., and Rottinghaus, G. E. (2009). Effects of turmeric (Curcuma longa) on the expression of hepatic genes associated with biotransformation, antioxidant, and immune systems in broiler chicks fed aflatoxin. Poult. Sci. 88, 2620–2627. doi: 10.3382/ps.2009-00204
Yunus, A. W., Razzazi-Fazeli, E., and Bohm, J. (2011). Aflatoxin B1 in affecting broiler’s performance, immunity, and gastrointestinal tract: a review of history and contemporary issues. Toxins 3, 566–590. doi: 10.3390/toxins3060566
Zhang, N., Qi, M., Zhao, L., Zhu, M., Guo, J., Liu, J., et al. (2016). Curcumin prevents aflatoxin B1 hepatoxicity by inhibition of cytochrome P450 isozymes in chick liver. Toxins 8:327. doi: 10.3390/toxins8110327
Keywords: CYP enzymes, Nrf2, AFB1, curcumin, liver
Citation: Muhammad I, Wang H, Sun X, Wang X, Han M, Lu Z, Cheng P, Hussain MA and Zhang X (2018) Dual Role of Dietary Curcumin Through Attenuating AFB1-Induced Oxidative Stress and Liver Injury via Modulating Liver Phase-I and Phase-II Enzymes Involved in AFB1 Bioactivation and Detoxification. Front. Pharmacol. 9:554. doi: 10.3389/fphar.2018.00554
Received: 09 February 2018; Accepted: 08 May 2018;
Published: 25 May 2018.
Edited by:
Yiqun Deng, South China Agricultural University, ChinaReviewed by:
Albert P. Li, In Vitro ADMET Laboratories, United StatesPreetida J. Bhetariya, University of Utah, United States
Copyright © 2018 Muhammad, Wang, Sun, Wang, Han, Lu, Cheng, Hussain and Zhang. This is an open-access article distributed under the terms of the Creative Commons Attribution License (CC BY). The use, distribution or reproduction in other forums is permitted, provided the original author(s) and the copyright owner are credited and that the original publication in this journal is cited, in accordance with accepted academic practice. No use, distribution or reproduction is permitted which does not comply with these terms.
*Correspondence: Xiuying Zhang, zhangxiuying@neau.edu.cn