- 1Department of Pharmacy, Faculty of Science and Engineering, International Islamic University Chittagong, Chittagong, Bangladesh
- 2Department of Applied Nutrition and Food Technology, Islamic University, Kushtia, Bangladesh
- 3Department of Pharmacy, University of Rajshahi, Rajshahi, Bangladesh
- 4Department of Biochemistry and Molecular Biology, University of Chittagong, Chittagong, Bangladesh
Anisomeles indica (L.) kuntze is widely used in folk medicine against various disorders including allergy, sores, inflammation, and fever. This research investigated the antinociceptive, anxiolytic and sedative effects of A. indica methanol extract. The antinociceptive activity was assessed with the acetic acid-induced writhing test and formalin-induced flicking test while sedative effects with open field and hole cross tests and anxiolytic effects with elevated plus maze (EPM) and thiopental-induced sleeping time tests were assayed. Computer aided (pass prediction, docking) analyses were undertaken to find out the best-fit phytoconstituent of total 14 isolated compounds of this plant for aforesaid effects. Acetic acid treated mice taking different concentrations of extract (50, 100, and 200 mg/kg, intraperitoneal) displayed reduced the writhing number. In the formalin-induced test, extract minimized the paw licking time of mice during the first phase and the second phase significantly. The open field and hole-cross tests were noticed with a dose-dependent reduction of locomotor activity. The EPM test demonstrated an increase of time spent percentage in open arms. Methanol extract potentiated the effect of thiopental-induced hypnosis in lesser extent comparing with Diazepam. The results may account for the use of A. indica as an alternative treatment of antinociception and neuropharmacological abnormalities with further intensive studies. The compound, 3,4-dihydroxybenzoic acid was found to be most effective in computer aided models.
Introduction
In the last few decades ethnobotanical research has reflected multifarious medicinal properties of plants including analgesic (Wirth et al., 2005), anti-inflammatory (Kumar et al., 2013), anti-rheumatic (Kaur et al., 2012), anti-cancer (Fridlender et al., 2015), and anti-depressive activities (Sarris et al., 2011). A number of herbal preparations is prescribed as analgesic and anti-depressive in the literature of alternative medicine. Search for new analgesic and anti-depressive drugs from a wider hub of medicinally important plants has been more focused since the last couple of decades. This is due to the exploration of novel therapeutic agents for suppression or relief of pain as well as depression (Anil, 2010).
The emotional responses or unusual sensory linked with potential tissue damage contributed by muscle spasm, tumor, nerve damage, inflammation, exposure to noxious chemical, thermal or mechanical stimuli refer to pain (Morrison and Morrison, 2006). As a therapeutic option, non-steroidal anti-inflammatory drugs (NSAIDs) are chosen for mild to moderate pain, while steroidal and opioids for intense acute and severe pain conditions. However, the side effects of both NSAIDs and opioids limit their frequent and free usages (Grosser et al., 2011; Yaksh and Wallace, 2011). Anxiety which has been reported as a psychological disorder is described as an unpleasant emotional state for which the cause is neither readily identified nor perceived to be uncontrollable. Anxiety impairs performances and it is associated with numbers of medically unexplained symptoms triggering insomnia. It is independently and strongly associated with chronic illnesses and low levels of life-quality (Hoffman et al., 2008). Therapeutic agents available for the treatment of anxiety include benzodiazepines, opioids, and non-steroidal anti-inflammatory drugs. These agents, however, are not without significant side effects that limit their use. Tolerance and dependence to some of these agents particularly opioids and benzodiazepines, make the search for potent alternatives very important (Kimiskidis et al., 2007). Medicinal plants have contributed enormously to the development of important therapeutic drugs for modern medical sciences (Olorunnisola et al., 2012). There is an increasing recognition that medicinal plants might provide a viable source for new drug molecules, especially in the case of failure of the more popular synthetic drugs (Prasad et al., 2005).
Anisomeles indica belongs to Lamiaceae family. It is usually familiar as gobura of annual shrub class distributed in most of the districts of Bangladesh. A. indica leaves are used for children's whooping cough and fever (Yusuf et al., 1994; Rahman et al., 2007). The roots have long been used for allergy, uterine infection, sores, and mouth abscess. Root also has anti-inflammatory, astringent, tonic properties (Yusuf et al., 1994). The extract of this plant is found to work on inflammatory mediators, bacteria, tumor cell proliferation and melanogenesis (Wang and Huang, 2005; Hsieh et al., 2008; Rao et al., 2009; Huang et al., 2012). Water extract of A. indica has primarily been found as centrally working analgesic (Dharmasiri et al., 2003). We thus comprehensively evaluated the antinociceptive, anxiolytic, and sedative effects effects of A. indica methanol extract (MEAI) in mice model elucidating the structure-activity relationship for aforesaid biological activities with the isolated phytocompounds of this plant by using in silico PASS prediction and molecular docking tools.
Methods and Materials
Plant Sample
Whole plant material of A. indica (L.) Kuntze (Lamiaceae) was collected from the Rajshahi University premises, Bangladesh in September, 2012 by the authors, and plant sample was identified by an expert taxonomist Dr. Sheikh Bokhtear Uddin who is working in the Department of Botany of University of Chittagong. A voucher specimen (accession no. 1304) has been preserved in the institutional herbarium of the aforesaid Department.
Extract Preparation
The shade-dried whole plants of A. indica were powdered (500 g) to macerate in absolute methanol (purity 99.99%, 1,500 ml). Powdered material was placed into an amber bottle for a 7-days-exhaustive extraction with occasional stirring and shaking in every 3 days. The extracts obtained were pooled and filtered using Whatman Filter paper #1. The final combined methanol specimen (850 ml) was evaporated to dryness using a vacuum rotary evaporator (RE200 Biby Sterling, UK) and weighted (16.19 g dry weight, 3.23% w/W) to determine the yield of soluble constituents. The semi-solid black-green crude extract soluble in methanol was preserved at 4°C.
Maintenance of Experimental Animals
Six-seven weeks old Swiss albino mice of both sexes (50% male and 50% female) weighing ~35–40 g were procured from the animal research division of the International Centre for Diarrheal Disease and Research, Bangladesh (ICDDR, B). Mice were housed in polycarbonated cages ensuring a standard laboratory condition of temperature 25°C and humidity 55–56% in a 12 h day light cycle. They had a free access to supplied pellet animal diet and tap water. Animal handling protocol was endorsed by the Planning and Development (P&D) committee of the Department of Pharmacy of International Islamic University Chittagong, Bangladesh.
Drugs and Reagents
Reagent grade formalin and acetic acid were purchased from MERCK, India through Taj Scientific Ltd. Diazepam and diclofenac-Na were brought from Sigma-Aldrich, USA via local supplier. Morphine sulfate kindly donated by Popular Pharmaceuticals Ltd., Bangladesh. All other reagents were of analytical grade unless otherwise specified.
Antinociceptive Activities
Acetic Acid-Induced Writhing Test
Either sex of mice (n = 6) weighing 35–40 g were divided into five groups. Normal control group received normal saline (10 ml/kg bw), reference control group received standard drug diclofenac sodium (10 mg/kg bw) while the rest of the groups were injected intraperitoneally with 50, 100, and 200 mg/kg bw of methanol extract of A. indica (MEAI). After 30 min of administration, the animals were injected (i.p.) 1% (v/v) (10 ml/kg bw) acetic acid. After 5 min of acetic acid injection, abdominal constrictions were counted for 10 min and the responses were compared with control group (Koster et al., 1959). Antinociceptive activity was calculated as the writhing percentage of inhibition. The percentage of inhibition was calculated using the following ratio:
Formalin Induced Licking Test
Formalin induced biphasic method employed in mice model was assessed as described previously (Burgos et al., 2010). Formalin solution (2.5%, 20 μl) prepared by 0.9% saline solution was injected into the sub-plantar region of the right hind paw of mice. Animals were intraperitoneally pretreated with saline solution, morphine sulfate (10 mg/kg bw), diclofenac sodium (10 mg/kg bw) and MEAI (50, 100, and 200 mg/kgbw) 1 h prior to formalin injection. Pain response was measured by the licking and biting of the injected paw. Responses measured for 5 min is considered as first phase and 15–30 min is considered as second phase after formalin injection. First phase and second phase response corresponds to the neurogenic and inflammatory pain responses, respectively. Antinociceptive activity was calculated as the percentage inhibition of licking time.
Locomotor Activity
Open Field Test
The spontaneous locomotor performances of MEAI were assessed by using the open field test as described earlier. Briefly, the mice were placed in the test room at least 1 h before each test for habituation. The open field devices were comprised of a Plexiglas square box (50 × 50 × 40 cm) with the floor divided into 25 small squares of equal dimensions (10 cm × 10 cm) marked by black lines. In this study, test animals were randomly divided into five groups. Normal control received orally 1% Tween-80 in water (10 ml/kg), positive control Diazepam (1 mg/kg) administered intraperitoneally and treatment groups received MEAI orally at the doses of 100, 200, and 400 mg/kg bw. One hour administration, each animal was placed individually at the center of the device and observed for 5 min to count the number of squares crossed by the animal with its four paws. The open field arena was thoroughly cleaned between each test so that the animal was not influenced by the odors of urine and feces of the previous one (Saleem et al., 2011). Inhibition of movements was calculated using the following formula:
Hole Cross Test
Hole cross test was accomplished with slight modification of the protocol previously described by Takagi et al. (1971). Briefly, mice were randomly divided into five groups: Control group received orally 1% Tween-80 in water (10 ml/kg bw), positive control group Diazepam (1 mg/kg bw) administered intraperitoneally and treatment group received MEAI orally at the doses of 100, 200, and 400 mg/kg bw. Afterwards the animals were placed in a compartment where a steel partition was fixed in the middle having a size of 30 × 20 × 14 cm3. A 3 cm (diameter) hole was made at a height of 7.5 cm in the center of the cage. After administration of control, positive control and different concentrations of extract the animals were allowed to cross the hole from one chamber to another and the numbers of passage through the hole from one chamber to other was counted. The total number of passage was counted for a period of 5 min on 0, 30, 60, 90, and 120 min during the study period. Percentage inhibition of movements was calculated using the same formula used in open field test.
Anxiolytic Activity
Elevated Plus-Maze Test (EPM)
The Elevated plus-maze test is the modified method of the validated assay of Lister for mice (Pellow and File, 1986). The apparatus consists of two open arms (35 × 5 cm2) and two closed arms (30 × 5 × 15 cm3) extended from a common central platform (5 × 5 cm2). The walls and floor of the closed arms are made of wood and painted black. The whole maze is elevated to a height of 50 cm above the basement. An edge (0.25 cm) was included around the perimeter of the open to facilitate exploration. Mice (35–40 g) were housed for 10 days prior to the test in the apparatus. To reduce stress, the mice were handled by the researcher on alternate days. Control group received orally 1% Tween-80 in water (10 ml/kg bw), positive control group Diazepam (1 mg/kg bw) administered intraperitoneally and treatment group received MEAI orally at the doses of 100, 200, and 400 mg/kg bw. After 30 min treatment with control, diazepam, and treatment group each mouse was set onto the center of the maze facing one of the enclosed arms. Number of entries and time spent onto the open arm were noted for a 5 min session. Throughout the test procedure a calm and smooth environment was ensured to obtain accurate results.
Sedative Activity
Thiopental Sodium Induced Sleeping Time Test
In this test animals were divided into four groups comprised of six mice each. Vehicle (1% Tween-80 in water 10 ml/kg), diazepam (1 mg/kg bw) and MEAI (100, 200, and 400 mg/kg bw) were injected intraperitoneally into control group, reference group and test groups, respectively. After 20 mins of treatment, thiopental sodium (40 mg/kg bw) was injected to each mouse for inducing sleep. The animals were observed to record the time between thiopental sodium administrations to loss of righting reflex (latent period) and duration between the loss and regaining of righting reflex (sleep duration) (File and Pellow, 1985). Percentage of effect was calculated using the following formula:
Selection of Compounds for Pass Prediction
Pedalitin, apigenin, methylgallate, 3,4-dihydroxybenzoic acid, calceolarioside, betonyoside A, campneoside II, acteoside, isoacteoside, and terniflorin were selected based on the availability as major compounds through literature survey (Rao et al., 2009). The structures of the compounds were collected from PubChem data base. Survey of quite a huge number of literatures made us to decide the above compounds as major compounds.
In Silico Experiment to Predict the Activity Spectra for Substances (PASS)
The selected phytoconstituents especially pedalitin, apigenin, methylgallate, 3,4-dihydroxybenzoic acid, calceolarioside, betonyoside A, campneoside II, acteoside, isoacteoside, terniflorin (Rao et al., 2009) were subjected for evaluating the antinociceptive activity with the aid of PASS program. This experiment predicts activity spectrum of a compound as probable activity (Pa) and probable inactivity (Pi) (Mohuya Mojumdar and Kabir, 2016) based on the structure-activity relationship analysis of the training set consisting of more than 205,000 compounds showing more than 3,750 types of biological activities. The values of Pa and Pi fluctuate between 0.000 and 1.000. A compound is considered experimentally active with Pa > Pi. Pa > 0.7, indicates the probability of pharmacological potential is high and the values following 0.5 < Pa < 0.7 reflect the considerable pharmacological effects experimentally. Pa < 0.5 shows less the pharmacological activity which may impart a chance of finding new compound (Goel et al., 2011; Khurana et al., 2011).
In Silico Molecular Docking
Preparation of Protein
Three dimensional crystal structure of Cyclooxygenase-1 (COX 1, PDB id: 2OYE), cyclooxygenase-2 (COX 2, PDB id: 3HS5), and 5-HT1B (PDB id: 4IAQ) were downloaded in PDB format from the protein data bank (Berman et al., 2002). Structure was prepared and refined using the Protein Preparation Wizard of Schrödinger-Maestro v10.1. Charges and bond orders were assigned while hydrogens were added to the heavy atoms and selenomethionines were converted to methionines followed by deleting all water molecules. Using force field OPLS_2005, minimization was carried out setting maximum heavy atom RMSD (root-mean-square-deviation) to 0.30 Å.
Ligand Preparation
Target compounds i.e., pedalitin, apigenin, methylgallate, 3,4-dihydroxybenzoic acid, calceolarioside, betonyoside A, campneoside II, acteoside, isoacteoside, and terniflorin were retrieved from Pubchem databases,. The 3D structures of the ligands were built by using Ligprep 2.5 in Schrödinger Suite 2015 with an OPLS_2005 force field. The pH 7.0 ± 2.0 was used to generate the ionization states of the compounds using Epik 2.2 in Schrödinger Suite. Up to 32 possible stereoisomers per ligand were retained.
Receptor Grid Generation
Receptor grids were calculated for prepared proteins so that various ligand poses bind within the predicted active site during docking. In Glide, grids were generated in a way to keep the default parameters of van der Waals scaling factor 1.00 and charge cutoff value 0.25 subjected to OPLS 2005 force field. A cubic box of specific dimensions centered on the centroid of the active site residues was generated for receptor. The bounding box was set to 14 × 14 × 14 × for docking experiments.
Glide Standard Precision (SP) Ligand Docking
SP flexible ligand docking was carried out in Glide of Schrödinger-Maestro v 10.1 (Friesner et al., 2004, 2006) within which penalties were applied to non-cis/trans amide bonds. For ligand atoms, Van der partial charge cutoff and scaling factor was selected to be 0.15 and 0.80, respectively. Final scoring was done on energy-minimized poses and showed as Glide score. The best docked pose with the lowest Glide score was recorded for each ligand.
ADME/T Property Analysis
Ligand Based ADME/Toxicity Prediction
The QikProp module of Schrodinger (Maestro, version 10.1) is a prompt, accurate, easy-to-use absorption, distribution, metabolism, and excretion (ADME) prediction program designed to produce certain descriptors linked to ADME. It predicts both pharmacokinetic and physicochemical significant descriptors relevant properties. ADME properties determine drug-like activity of ligand molecules based on Lipinski's rule of five. ADME/T properties of the compound (DIM) were analyzed using Qikprop 3.2 module (Natarajan et al., 2015).
Statistical Analysis
Data were presented as mean ± standard error of mean (SEM) values from triplicates. One-way analysis of variance (ANOVA) followed by Dunnet's test was used to describe the data for significant differences between the test and control groups using GraphPad Prism Data Editor for Windows, Version 6.0 (GraphPad software Inc., San Diego, CA). P-values (<0.05 and <0.01) were considered as statistically significant.
Results
Antinociceptive Activities
Acetic Acid Induced Writhing Response
The results demonstrated the significant antinociceptive activity of MEAI as shown in Figure 1. The analgesic and writhing-inhibitory effect were increased with the dose in a noteworthy manner. The MEAI was found to be moderately active with its low dose and showed inhibitory effects of 20.00 and 45.71% at the doses of 50 mg/kg and 100 mg/kg, respectively. MEAI at a dose of 200 mg/kg exhibited the maximum inhibition of acetic acid induced writhing numbers which was significantly close (p < 0.05, F = 49.74) to that of the reference standard diclofenac sodium.
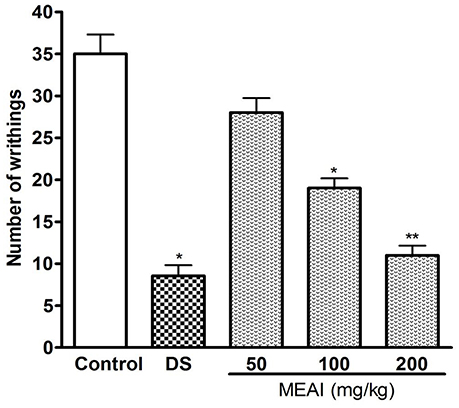
Figure 1. Effect of methanol extract of the A. indica and DS (10 mg/kg) on acetic acid induced writhing test. Values are mean ± S.E.M. *p < 0.05 and **p < 0.01, significantly different from control; ANOVA followed Dunnett's test (n = 6, per group). DS, diclofenac sodium; MEAI, methanolic extract of A. indica.
Formalin Induced Licking Test
A characteristic biphasic nociceptive response is induced in the test animals after i.p administration of formalin. In both the phases the pain behaviors were evaluated individually by observing licking duration of animal models. The MEAI showed significant (p < 0.05) reduction of nociceptive behaviors evoked by formalin compared with control group. In the early/neurogenic phase (F = 24.03), MEAI showed protection percentages of 31.94, 45.18, and 58.53% at the doses of 50, 100, and 200 mg/kg, respectively. While in the late phase, MEAI markedly reduced the licking duration, significantly (p < 0.05, F = 15.63) lower than reference drug morphine, by exerting 44.18, 59.67, and 70.53% inhibition at the same concentration, respectively (Figure 2).
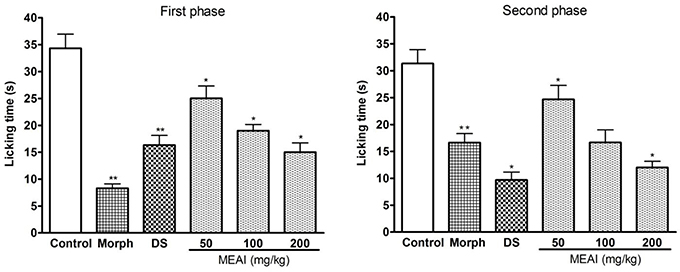
Figure 2. Effect of methanol extract of the A. indica, DS, and morphine (10 mg/kg) on formalin test (first phase and second phase). Values are mean ± S.E.M. *p < 0.05 and **p < 0.01, significantly different from control; ANOVA followed Dunnett's test (n = 6, per group). DS, diclofenac sodium; MEAI, methanolic extract of A. indica.
Locomotor Activity
Open Field Test
The CNS activity of drug is evaluated by its effect on locomotion of the test animal model. In this test, the A. indica showed a dose dependent decrease in locomotor activity at the tested dose levels and the effects were statistically significant (p < 0.05). The locomotion lowering effect was pronouncedly evident from the second observation period continued till last observation period (120 min). In this study, test animal showed dose dependent decrease of movement figured as 38.52 ± 3.40, 27.71 ± 2.87, 15.90 ± 1.74 with 100, 200, and 400 mg/kg, respectively. While the reference drug diazepam (1 mg/kg) decreased (p < 0.05, F = 0.8140) the number of movement 54.56 ± 3.76, 34.59 ± 2.76, 20.15 ± 3.50, and 16.35 ± 3.12 with the similar doses from second observation period to last observation period (Figure 3).
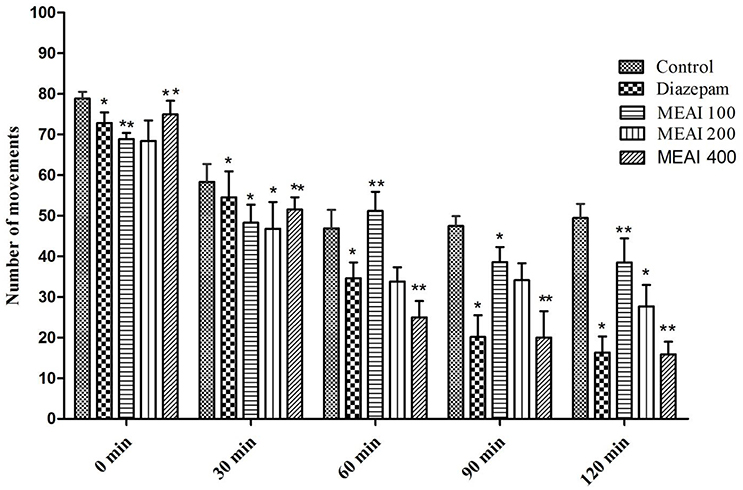
Figure 3. Effect of methanolic extract of A. indica on open field test in mice. Values are mean ± S.E.M. *p < 0.05 and **p < 0.01, significantly different from control; ANOVA followed Dunnett's test (n = 6, per group). MEAI, methanolic extract of A. indica.
Hole Cross Test
MEAI demonstrated a gradual decrease in locomotion of the test animal model starting from second observation period (30 min) as confirmed by the reduction in the number of passes of tested mice through the hole in contrast to the control group (Figure 4). Therefore, a potent central nervous system (CNS) depressant activity was exhibited by MEAI at respective doses from second (30 min) to final (120 min) observation point which was statistically significant (p < 0.05–0.01). The results displayed the number of holes crossed at dose 400 mg/kg bw (1.95 ± 1.67) was comparable (p < 0.05, F = 0.5559) with the standard drug diazepam (2.78 ± 0.51) at final observation period (120 min). The other two dosages (100, 200 mg/kg bw) also reduced the locomotor activity (4.52 ± 1.21 and 2.10 ± 0.25, respectively). The CNS was found to be depressed during the observation period 0 to 120 min.
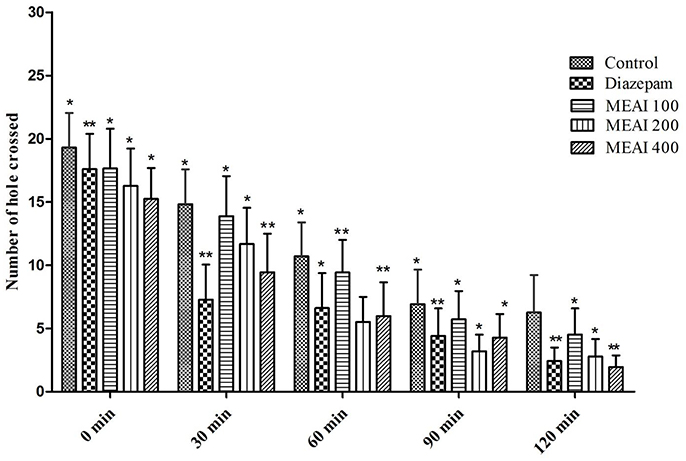
Figure 4. Effect of methanolic extract of A. indica on hole cross test in mice. Values are mean ± S.E.M. *p < 0.05 and **p < 0.01, significantly different from control; ANOVA followed Dunnett's test (n = 6, per group). MEAI, methanolic extract of A. indica.
Anxiolytic Activity
Elevated Plus-Maze Test (EPM)
As shown in Figure 5, all doses of MEAI increased the entries into the open arms. Meanwhile, the time spent into open arms was also increased to 43.04 ± 2.15, 48.25 ± 3.60, 64.75 ± 3.19 with the three doses. The values were statistically significant (p < 0.05, F = 18.81) compared to the control (35.75 ± 2.80). All the tested doses demonstrated a dose-dependent increase of time spent in open arms. Additionally, there was also a significant increase in duration of time (64.75 ± 3.19) for 400 mg/kg, into open arm, which was significant (p < 0.05, F = 17.52) in comparison to that (78.20 ± 4.12) of reference group.
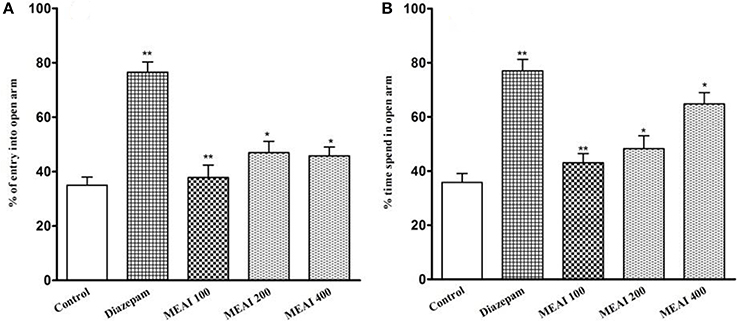
Figure 5. (A) Effect of methanolic extract of A. indica on percentage of entry into open arm in elevated plus maze test in mice. (B) Effect of methanolic extract of A. indica on percentage of time spent into open arm in elevated plus maze test in mice. Values are mean ± S.E.M. *p < 0.05 and **p < 0.01, significantly different from control; ANOVA followed Dunnett's test (n = 6, per group). MEAI: methanolic extract of A. indica.
Sedative Activity
Thiopental Sodium Induced Sleeping Time Test
It was found that MEAI significantly (p < 0.05, F = 34.45) potentiated a decrease in onset of sleep and dose dependently increased in duration of sleep in thiopental-induced sleeping time test (F = 28.51). The prolongation of duration of sleeping time at dosages 100, 200, and 400 mg/kg (65.01 ± 4.71, 104.60 ±3.20, 146.46 ± 4.89, respectively) in comparison to control group (49.50 ± 2.70). Thiopental sodium induced sleeping time test was shown in Figure 6.
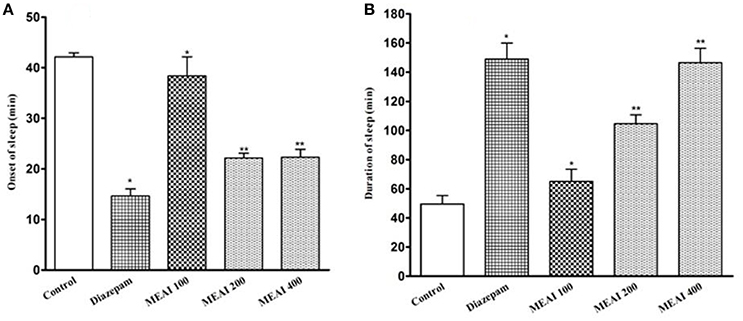
Figure 6. (A) Effect of methanolic extract of A. indica on onset of sleep in thiopental sodium induced sleeping time test in mice. (B) Effect of methanolic extract of A. indica on duration of sleep in thiopental sodium induced sleeping time test in mice. Values are mean ± S.E.M. *p < 0.05 and **p < 0.01, significantly different from control; ANOVA followed Dunnett's test (n = 6, per group). MEAI, methanolic extract of A. indica.
In Silico Pass Prediction
Ten constituents namely pedalitin, apigenin, methylgallate, 3,4-dihydroxybenzoic acid, calceolarioside, betonyoside A, campneoside II, acteoside, isoacteoside, terniflorin were analyzed by the PASS for their antinociceptive effects and results were used in a flexible manner. The chosen compounds showed higher Pa than Pi (Table 1). The compound 3,4-dihydroxybenzoic acid showed highest Pa-value for antinociceptive activity (Pa = 0.563) followed by methylgallate (Pa = 0.537).
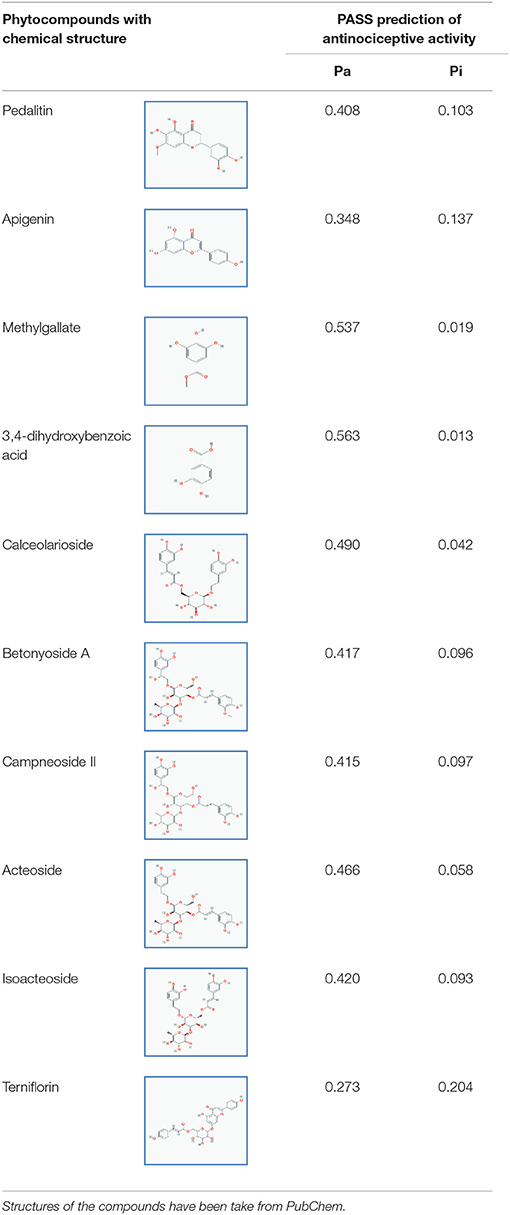
Table 1. Pass prediction of pedalitin, apigenin, methylgallate, 3,4-dihydroxybenzoic acid, calceolarioside, betonyoside A, campneoside II, acteoside, isoacteoside, terniflorin for antinociceptive activity.
In Silico Molecular Docking Analysis for Analgesic Effect
Advancement and sophistication of computational techniques have developed virtual screening to get a positive impact on the process of discovery. Virtual screening process uses the scoring and docking of the compound individually from a database while the fundamental of the technique is prediction of binding modes and affinity of the compounds by means of docking to an X-ray crystallographic structure. Grid based docking model was used to analyze the binding pattern of molecules with the amino acids present in the active pocket of the protein. To evaluate the potential analgesic molecule, we have undertaken the docking analysis of the isolated active compounds of A. indica to the active site cyclooxygenase enzymes viz. COX-1. Fourteen compounds so far isolated by different researchers from A. indicia have been listed up (Rao et al., 2009) and the prevailing 10 compounds have been chosen to find out the most potential compounds suitable for therapeutic approaches through in silico docking study. Despite the diverse chemical structures of non-steroidal anti-inflammatory drugs, their analgesic effects are mainly due to the common property of inhibiting cyclo-oxygenases (COX) involved in the formation of prostaglandins, the inflammatory mediators, which are formed by the conversion of arachidonic acid. Therefore, this study has chosen the isoforms Cox-1 and Cox-2 of Cox enzyme for in silico model (Lenardão et al., 2016). Additionally the receptor 5-HT1B was selected as the receptor as well modulates the antinociception through its agonist binding (Jeong et al., 2012). For studying the interaction of the compounds acteoside, betonyoside A, β-sitosterol, isoacteoside, stigmasterol with 2OYE,Glide docking analysis was performed by Schrodinger suite v10.1, where among of these compounds apigenin shows highest docking score shown in Table 2. The low and negative free energy values for binding indicates a strong favorable bond between 2OYE. According to Docking Score, apigenin was found to have the highest affinity to the COX-1 enzymes corresponding to the methylgallate, 3,4-dihydroxybenzoic acid and calceolarioside. The results of docking analysis were presented in Tables 2–4 and the docking figure showed in Figures 7–9.
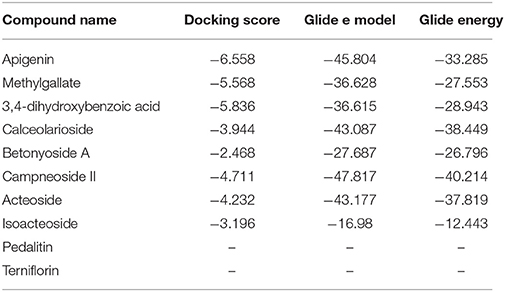
Table 2. Docking results of pedalitin, apigenin, methylgallate, 3,4-dihydroxybenzoic acid, calceolarioside, betonyoside A, campneoside II, acteoside, isoacteoside, terniflorin with COX 1 (PDB: 2OYE) for analgesic effect.
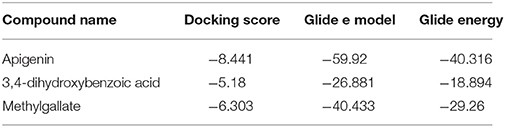
Table 3. Docking results of apigenin, methylgallate, 3,4-dihydroxybenzoic acid with COX 2 (PDB: 3HS5) for analgesic effect.
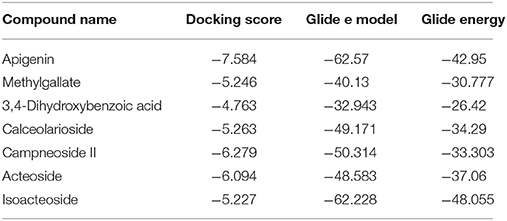
Table 4. Docking results of apigenin, methylgallate, 3,4-dihydroxybenzoic acid, calceolarioside, campneoside II, acteoside, isoacteosidewith 5-HT1B (PDB id: 4IAQ) for antidepressant effect.
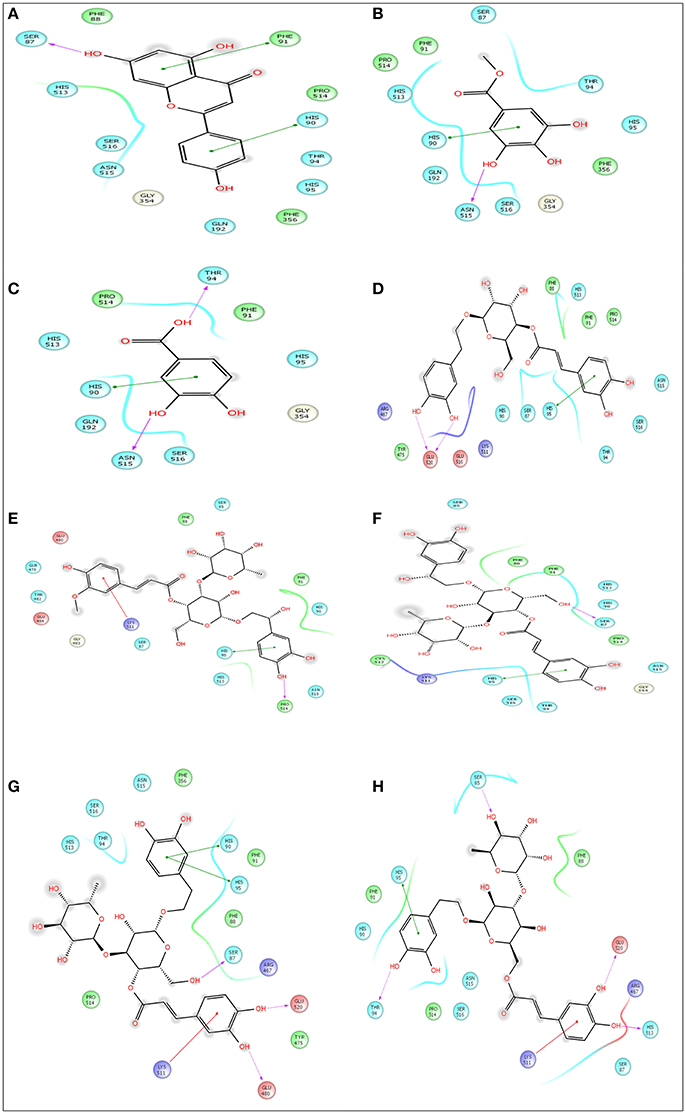
Figure 7. Docking results of apigenin (A), methylgallate (B), 3,4-dihydroxybenzoic acid (C), calceolarioside (D), betonyoside A (E), campneoside II (F), acteoside (G), isoacteoside (H) with COX 1 (PDB: 2OYE) for analgesic effect. The colors indicate the residue (or species) type: Red-acidic (Asp, Glu), Green-hydrophobic (Ala, Val, Ile, Leu, Tyr, Phe, Trp, Met, Cys, Pro), Purple-basic (Hip, Lys, Arg), Blue-polar (Ser, Thr, Gln, Asn, His, Hie, Hid), Light gray-other (Gly, water), Darker gray-metal atoms. Interactions with the protein are marked with lines between ligand atoms and protein residues: Solid pink—H-bonds to the protein backbone, Dotted pink-H-bonds to protein side chains, Green—pi-pi stacking interactions, Orange-pi-cation interactions. Ligand atoms that are exposed to solvent are marked with gray spheres. The protein “pocket” is displayed with a line around the ligand, colored with the color of the nearest protein residue. The gap in the line shows the opening of the pocket.
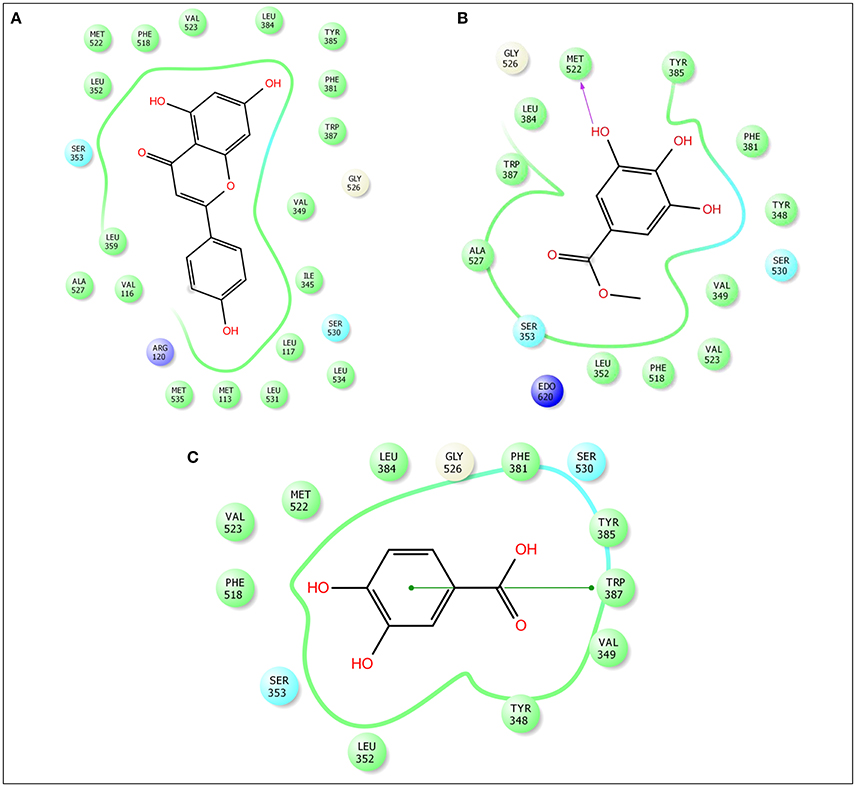
Figure 8. Docking results of apigenin (A), methylgallate (B), 3,4-dihydroxybenzoic acid (C) with COX 2 (PDB: 3HS5) for analgesic effect. The colors indicate the residue (or species) type: Red-acidic (Asp, Glu), Green-hydrophobic (Ala, Val, Ile, Leu, Tyr, Phe, Trp, Met, Cys, Pro), Purple-basic (Hip, Lys, Arg), Blue-polar (Ser, Thr, Gln, Asn, His, Hie, Hid), Light gray-other (Gly, water), Darker gray-metal atoms. Interactions with the protein are marked with lines between ligand atoms and protein residues: Dotted pink-H-bonds to protein side chains, Green—pi-pi stacking interactions, Orange-pi-cation interactions. Ligand atoms that are exposed to solvent are marked with gray spheres. The protein “pocket” is displayed with a line around the ligand, colored with the color of the nearest protein residue. The gap in the line shows the opening of the pocket.
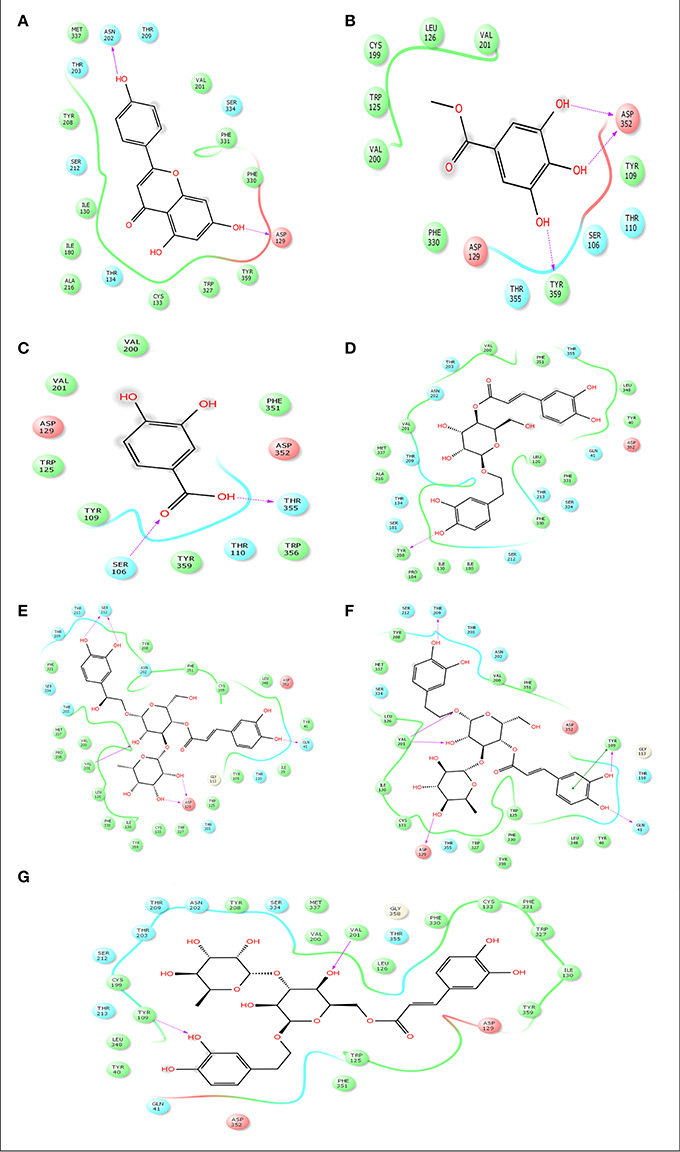
Figure 9. Docking results of apigenin (A), methylgallate (B), 3,4-dihydroxybenzoic acid (B), calceolarioside (D), campneoside II (E), acteoside (F), isoacteoside (G) with 5-HT1B (PDB id: 4IAQ) for antidepressant effect. The colors indicate the residue (or species) type: Red-acidic (Asp, Glu), Green-hydrophobic (Ala, Val, Ile, Leu, Tyr, Phe, Trp, Met, Cys, Pro), Purple-basic (Hip, Lys, Arg), Blue-polar (Ser, Thr, Gln, Asn, His, Hie, Hid), Light gray-other (Gly, water), Darker gray-metal atoms. Interactions with the protein are marked with lines between ligand atoms and protein residues: Solid pink—H-bonds to the protein backbone, Dotted pink-H-bonds to protein side chains, Green—pi-pi stacking interactions, Orange-pi-cation interactions. Ligand atoms that are exposed to solvent are marked with gray spheres. The protein “pocket” is displayed with a line around the ligand, colored with the color of the nearest protein residue. The gap in the line shows the opening of the pocket.
Toxicity and ADME Analysis Through Ligand Based Toxicity/ADME Prediction
The drug-like activity of the ligand molecule was classified using ADME properties by QikProp module of Schrodinger. The ADME properties of the pedalitin, apigenin, methylgallate, 3,4-dihydroxybenzoic acid, calceolarioside, betonyoside A, campneoside II, acteoside, isoacteoside, terniflorin were clarified with QikProp module of Schrodinger, shown in Table 5. The selected properties are known to influence cell permeation, metabolism, and bioavailability. Predicted properties of the pedalitin, apigenin, methylgallate, 3,4-dihydroxybenzoic acid were in the range to satisfy the Lipinski's rule of five to be recognized as drug like potential. All other compounds were not satisfying the all five rules.
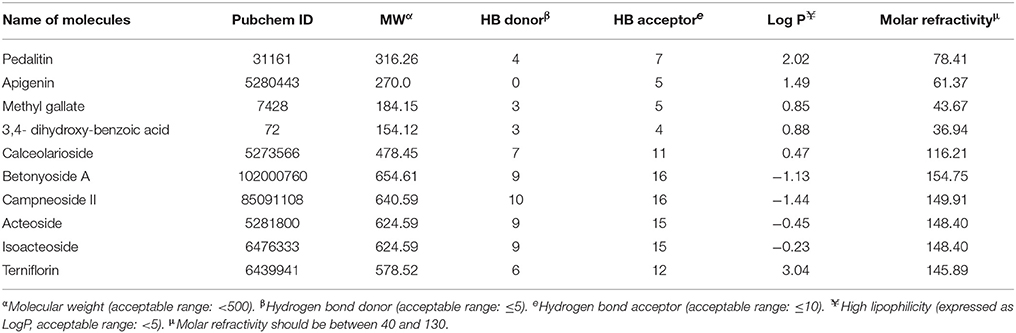
Table 5. ADME/T properties of pedalitin, apigenin, methylgallate, 3,4-dihydroxybenzoic acid, calceolarioside, betonyoside A, campneoside II, acteoside, isoacteoside, terniflorin by QikProp.
Discussion
Pain is one of the most pervasive problems in current time and it has high social and economic impacts (Julius and Basbaum, 2001). During inflammation, several mediators can activate and/or sensitize nociceptive fibers and mediators; these mediators are also involved in edema formation and leukocyte infiltration (Mazzon et al., 2008). Several analgesics are used to treat a wide range of painful and inflammatory conditions, including non-steroidal anti-inflammatory drugs (NSAIDs), glucocorticoids and opioids (Ferreira et al., 1997). Despite the high diversity of available anti-inflammatory and analgesic drugs, their side effects and the ineffectiveness of some drugs for some conditions require the continuous search for new drugs. Investigating plant–derived products is a vital way to discover effective and less toxic drugs (Fine et al., 2009).
This study therefore investigated the anti-nociceptive activity of MEAI in classical non-narcotic peripheral and central acting pain models by acetic acid induced writhing test and formalin induced licking test, respectively. Acetic acid induced writhing test evaluated the antinociceptive activity of MEAI characterizing through abdominal contractions, body movements as a whole and twisting of the abdominal muscles. This non-specific pain evaluating method is suitable to detect the effects showed by weak analgesics. Pain is generated by endogenous inflammatory mediators such as serotonin and bradykinin which stimulate peripheral nociceptive neurons (Sakiyama et al., 2008). The pain-sensation in acetic acid induced model is triggered by localized inflammatory response for the release of free arachidonic acid from tissue phospholipids via cyclooxygenase (COX-1 and COX-2), and prostaglandin specifically PGE2 and PGF2 biosynthesis, the level of lipoxygenase products may also be increased in peritoneal fluids (Adzu et al., 2003). MEAI administration significantly reduced acetic acid-induced writhing in mice. This result supports the hypothesis that the extract from A. indica may act by inhibiting prostaglandin synthesis because the nociceptive mechanism of abdominal writhing induced by acetic acid involves the release of arachidonic acid metabolites via cyclooxygenase (COX), and prostaglandin biosynthesis. Additionally, the possible involvement of neurotransmitter systems, such as opioid, serotonergic, purinergic, cholinergic, catecholaminergic, cannabinoid, GABAergic systems as well as ATP-gated potassium channels could be involved. Apart from these, flavonoid and their derivatives have been found to be antinociceptive and anti-inflammatory agents due to their ability to inhibit arachidonic acid metabolism (Middleton et al., 2000). It is possible that the presence of apigenin, terniflorin and other flavonoid molecules in the extract of A. indica be responsible for the antinociceptive effect. In silico study also complies with the binding of cyclooxygenase with the target compounds especially 3,4-dihydroxybenzoic acid and apigenin used in this study.
The formalin test is one of the widely used methods of expressing pain and analgesic mechanism in contrast to mechanical or thermal stimulus methods (Khan et al., 2010). Applying this method we can also differentiate between the peripheral and central antinociceptive pain. This is a biphasic model where the early phase represents neurogenic (1–5 min) and late phase represents inflammatory pain (15–30 min), respectively (Dallel et al., 1995). The early phase demonstrates an acute response observed immediately after the administration of formalin because of direct stimulation of nociceptive neurons. While the late phase gives a delayed response made by the release of inflammatory mediators especially prostaglandins, histamine, serotonin and bradykinin, and activation of the neurons in the dorsal horns of the spinal cord (Clavelou et al., 1995). Administration of MEAI in different doses significantly inhibited the pain response in both phases as confirmed by reduced licking behavior but the effect was more prominent in the late phase. Considering the inhibitory property of MEAI on the second phase of formalin, we might suggest an anti-inflammatory action of the plant extract. This result is in line with that obtained in formalin model and indicates that MEAI probably interacts with COX receptors occupied by the A. indica compounds showing formalin-induced nociception. Opioid analgesics exert its antinociceptive effects for both phases while the early phase is more sensitive whereas NSAIDs seem to suppress only the late phase. Therefore, reduced liking time in both phases indicates a possible interaction with neurogenic and inflammatory pain modulators.
To evaluate the drug action on CNS, observation of locomotor activity of the test animal is regarded as a noteworthy method. Because this activity is regarded as an indicator of alertness and a decreased locomotor performance has been used as an index of CNS depressant activity (Hunskaar and Hole, 1987). Various researches have confirmed that open field method is a classic model to evaluate general and spontaneous activity of animals (Sousa et al., 2004; Gahlot et al., 2013). The decrease in locomotor activity due to the treatment with MEAI in the tested mice was corresponding with the effect of antidepressants because sensory afferent neurons provide excitatory feedback to motor neurons and spinal interneurons in response to muscle contraction during active locomotion. Additionally local GABAergic interneurons modulate this pathway by inhibiting sensory afferents at the presynaptic level (Rudomin, 2009). Pharmacological manipulations showed that GABAergic neurons modulate the burst frequency of motor neurons during fictive locomotion (Schmitt et al., 2004).
The hole-cross test is similar to the open field test in the constructs that it can assess locomotor activity and detect the wide spectrum antidepressants. Accordingly, it is believed that complementary and/or converging information on potential antidepressants could be achieved by using both the tests can. From our study data, it is evident that i.p. administration of MEAI significantly suppressed the spontaneous locomotion of test mice in the hole-cross test. Substances which have sedative and CNS depressant activity either decrease the time for onset of sleep or prolong the duration of sleep or both. It is possible that MEAI act by potentiating GABAergic inhibition in the CNS via membrane hyperpolarization since GABA is the major inhibitory neurotransmitter in the CNS and it leads to a reduction in the firing rate of critical neurons in the brain. This inhibition may be due to direct activation of GABA receptor by MEAI. It may also be due to enhanced affinity for GABA or an increase in the duration of the GABA-gated channel opening (Barria et al., 1997).
The use of the elevated plus maze (EPM) test is considered to be better examine anxiety-like behavior (Katz et al., 1981). Drugs having anxiolytic activity decreases distance for open arms and induces test animal to spend much time in open arms whereas anxiogenics decrease open arm exploration by reducing both the number of entries and spent time into the open arms (Griebel et al., 2000). Administration of MEAI displayed an inclination of increasing the time spent in the open arms, an indicator of decreased anxiety implying a reduction in anxiety-like behavior. It is believed that antidepressive efficiency in the elevated plus-maze test is a bit controversial because acute antidepressants may give rise to anxiogenic effect in this paradigm (Kõks et al., 2001; Holmes and Rodgers, 2003; Drapier et al., 2007). However, anxiety behavior was not induced by MEAI.
Thiopental sodium induced sleeping test was used for assessing the sleeping behavior. Indeed, thiopental induces hypnosis by potentiating GABA mediated post synaptic inhibition caused due to the allosteric modification of GABAA receptors. Components possessing CNS depressant effects either reduce the time of onset of sleep or extend the sleep duration or both (Nyeem et al., 2006; Hasan et al., 2009). Earlier, it has been found a relationship between enhancement of hypnosis and index of CNS depressant activity (Fujimori, 1965). Phytochemical analysis revealed that leaves of A. indica contain alkaloids and tannins as major phytochemicals along with saponins, glycosides, carotenoids, polyuronoides and aromatic oil as bioactive constituents (Ulhe and Narkhede, 2013). Moreover, several behavioral researches demonstrated that isoquinoline-type alkaloid structures possess anticonvulsant and hypnotic properties (Singla et al., 2010). The presence of alkaloidal phytochemicals in A. indica may exert its sedative activity possibly through inhibition of GABAA receptors.
A. indica containing flavonoids, terpenoids, and propanoids exerted anti-inflammatory activities, in vitro experiment displayed previously (Rao et al., 2009). Further support for the significance of our results is the finding of antinociceptive, antidepressive, and anxiolytic properties of MEAI in vivo. Earlier, it has been reported that pathophysiology of major depression is associated with oxidative stress (Bilici et al., 2001). Therefore, treatment with antioxidant may reduce the oxidative stress and depressive disorder as well. Recently, we have reported that A. indica possesses anticholinesterase and antioxidative properties (Uddin et al., 2016)which may substantiate the possible contribution to its antidepressant-like effect.
PASS computer program was used to screen biological activity of selected compounds as antinociceptive. The compound 3,4-dihydroxybenzoic acid showed the highest Pa-value (Pa = 0.563) for antinociceptive activity which was preceded by methyl gallate showed the Pa-value 0.537 indicating a high probability of these compounds acting as antinociceptive agents. Previous researches showed some benzoic acid derivatives to be involved in the antinociceptive action with direct or indirect activation of opioid receptors (Déciga-Campos et al., 2007).
This research has conducted a molecular docking analysis, which allows accurate prediction of ligand and receptor interaction as well as binding energy, to have a good picture of Cyclooxygenase A. indica compounds interaction. Grid based docking study was used to analyze the binding modes of molecules with the amino acids present in the active pocket of the protein (Veeramachaneni et al., 2015). To identify a potential lead molecules for analgesic activity and antidepressant effect, docking analysis of the active compounds A. indica has been performed with the active site of cyclooxygenase enzymes-1 (COX-1), cyclooxygenase enzymes-2 (COX-2) and serotonin receptors. The interaction between compounds and the active site was assessed with docking analysis by Schrodinger suite v10.1.
Among of the compounds of A. indica, apigenin gives the lowest docking score −6.558 and −8.441 with COX-1 and COX-2 receptors, respectively, followed by methylgallate and 3,4-dihydroxybenzoic acid. It has been evident that the negative and low binding energy demonstrates a strong bonding. Additionally, apigenin is the potential compound for non-selective analgesic activity. The antinociceptive and neuroprotective effect of this flavonoid molecule is already been established (Kowalski et al., 2005).
In addition, the performance of molecular docking, determining the antidepressant activity among of the isolated compounds with the serotonin receptors, apigenin displayed the negative and low value (−7.584) of free energy of binding demonstrates making a strong favorable bond. Docking score suggests apigenin might be the responsible compound for potential antidepressant activity.
Virtual screening is important for natural product chemists to search the theoretically active principles with attractive ADME/T profiles which have been isolated previously but not assayed the activity against specified drug targets (Ntie-Kang et al., 2013). This prediction procedure can be a better option for lead search than the random screening. From the results of ADME/T test, it is clear that among all the compounds only pedalitin, apigenin, methylgallate, and 3,4-dihydroxybenzoic acid were in the range for satisfying the Lipinski's rule of five to be considered as potential drug in terms of better pharmacokinetics properties with less toxicity.
Conclusion
The methanol extract of all tested doses of A. indica possesses analgesic activity in central as well as peripheral pain models. In addition, results of the present neuropharmacological study of A. indica exhibited antidepressant and anxiolytic activity with less sedative side effects. In both cases the effect was dose dependent and statistically significant. Moreover, the computer programs PASS reflected the antinociceptive activity; molecular docking demonstrated higher binding affinity with COX-1, COX-2, serotonin and ADME/Toxicity analysis displayed the satisfactory pharmacokinetics and toxicity profiles of 3,4-dihydroxybenzoic acid and apigenin. Further research must be conducted to elucidate the antinociceptive and neuropharmacological activities of selected potential compounds in animal model with a dose-response study.
Author Contributions
MU, AA, and MA-A-M together planned and designed the research and arrange the whole facilities for the research; MN, SA, and MA conducted all the laboratory works, MK performed the in silico works using bioinformatics tools, MR imparted in study design and interpreted the results putting efforts on statistical analysis with MU, AA, and MA-A-M.
Funding
This work is managed to perform with the individual funding of all authors.
Conflict of Interest Statement
The authors declare that the research was conducted in the absence of any commercial or financial relationships that could be construed as a potential conflict of interest.
The reviewer ND and handling Editor declared their shared affiliation.
Acknowledgments
Authors wish to thank the authority of International Islamic University Chittagong for their kind support in progress of the research.
References
Adzu, B., Amos, S., Kapu, S., and Gamaniel, K. (2003). Anti-inflammatory and anti-nociceptive effects of Sphaeranthus senegalensis. J. Ethnopharmacol. 84, 169–173. doi: 10.1016/S0378-8741(02)00295-7
Barria, A., Derkach, V., and Soderling, T. (1997). Identification of the Ca2+/calmodulin-dependent protein kinase II regulatory phosphorylation site in the alpha-amino-3-hydroxyl-5-methyl-4-isoxazole-propionate-type glutamate receptor. J. Biol. Chem. 272, 32727–32730.
Berman, H. M., Battistuz, T., Bhat, T., Bluhm, W. F., Bourne, P. E., Burkhardt, K., et al. (2002). The protein data bank. Acta Crystallogr. Sec. D Biol. Crystallogr. 58, 899–907. doi: 10.1107/S0907444902003451
Bilici, M., Efe, H., Köroglu, M. A., Uydu, H. A., Bekaroglu, M., and Deger, O. (2001). Antioxidative enzyme activities and lipid peroxidation in major depression: alterations by antidepressant treatments. J. Affect. Disord. 64, 43–51. doi: 10.1016/S0165-0327(00)00199-3
Burgos, E., Pascual, D., Martín, M. I., and Goicoechea, C. (2010). Antinociceptive effect of the cannabinoid agonist, WIN 55,212-2, in the orofacial and temporomandibular formalin tests. Eur. J. Pain 14, 40–48. doi: 10.1016/j.ejpain.2009.02.003
Clavelou, P., Dallel, R., Orliaguet, T., Woda, A., and Raboisson, P. (1995). The orofacial formalin test in rats: effects of different formalin concentrations. Pain 62, 295–301. doi: 10.1016/0304-3959(94)00273-H
Dallel, R., Raboisson, P., Clavelou, P., Saade, M., and Woda, A. (1995). Evidence for a peripheral origin of the tonic nociceptive response to subcutaneous formalin. Pain 61, 11–16. doi: 10.1016/0304-3959(94)00212-W
Déciga-Campos, M., Montiel-Ruiz, R. M., Navarrete-Vázquez, G., and López-Muñoz, F. J. (2007). Palmitic acid analogues exhibiting antinociceptive activity in mice. Proc. West. Pharmacol. Soc. 50, 75–77.
Dharmasiri, M., Ratnasooriya, W., and Thabrew, M. I. (2003). Water extract of leaves and stems of preflowering but not flowering plants of Anisomeles indica possesses analgesic and antihyperalgesic activities in rats. Pharm. Biol. 41, 37–44. doi: 10.1076/phbi.41.1.37.14699
Drapier, D., Bentué-Ferrer, D., Laviolle, B., Millet, B., Allain, H., Bourin, M., et al. (2007). Effects of acute fluoxetine, paroxetine and desipramine on rats tested on the elevated plus-maze. Behav. Brain Res. 176, 202–209. doi: 10.1016/j.bbr.2006.10.002
Ferreira, S. H., Cunha, F. Q., Lorenzetti, B. B., Michelin, M. A., Perretti, M., Flower, R. J., et al. (1997). Role of lipocortin-1 in the anti-hyperalgesic actions of dexamethasone. Braz. J. Pharmacol. 121, 883–888. doi: 10.1038/sj.bjp.0701211
File, S. E., and Pellow, S. (1985). The effects of PK 11195, a ligand for benzodiazepine binding sites, in animal tests of anxiety and stress. Pharmacol. Biochem. Behav. 23, 737–741. doi: 10.1016/0091-3057(85)90064-4
Fine, P. G., Mahajan, G., and McPherson, M. L. (2009). Long-acting opioid sandshort-acting opioids: appropriate use in chronic pain management. Pain Med. 10(Suppl. 2), S79–S88. doi: 10.1111/j.1526-4637.2009.00666.x
Fridlender, M., Kapulnik, Y., and Koltai, H. (2015). Plant derived substances with anti-cancer activity: from folklore to practice. Front. Plant Sci. 6:799. doi: 10.3389/fpls.2015.00799
Friesner, R. A., Banks, J. L., Murphy, R. B., Halgren, T. A., Klicic, J. J., Mainz, D. T., et al. (2004). Glide: a new approach for rapid, accurate docking and scoring. 1. Method and assessment of docking accuracy. J. Med. Chem. 47, 1739–1749. doi: 10.1021/jm0306430
Friesner, R. A., Murphy, R. B., Repasky, M. P., Frye, L. L., Greenwood, J. R., Halgren, T. A., et al. (2006). Extra precision glide: docking and scoring incorporating a model of hydrophobic enclosure for protein– ligand complexes. J. Med. Chem. 49, 6177–6196. doi: 10.1021/jm051256o
Fujimori, H. (1965). Potentiation of barbital hypnosis as an evaluation method for central nervous system depressants. Psychopharmacologia 7, 374–378. doi: 10.1007/BF00403761
Gahlot, K., Lal, V. K., and Jha, S. (2013). Anticonvulsant potential of ethanol extracts and their solvent partitioned fractions from Flemingia strobilifera root. Pharmacognosy Res. 5:265. doi: 10.4103/0974-8490.118825
Goel, R. K., Singh, D., Lagunin, A., and Poroikov, V. (2011). PASS-assisted exploration of new therapeutic potential of natural products. Med. Chem. Res. 20, 1509–1514. doi: 10.1007/s00044-010-9398-y
Griebel, G., Belzung, C., Perrault, G., and Sanger, D. J. (2000). Differences in anxiety-related behaviours and in sensitivity to diazepam in inbred and outbred strains of mice. Psychopharmacology 148, 164–170. doi: 10.1007/s002130050038
Grosser, T., Smyth, E., and FitzGerald, G. A. (2011). “Anti-inflammatory, antipyretic, and analgesic agents; pharmacotherapy of gout,” in Goodman and Gilman's the Pharmacological basis of Therapeutics, 12th Edn., ed L. L. Brunton (New York, NY: The McGraw-Hill Companies, Inc.), 959–1004.
Hasan, S., Hossain, M., Akter, R., Jamila, M., Mazumder, M., Hoque, E., et al. (2009). Sedative and anxiolytic effects of different fractions of the Commelina benghalensis Linn. Drug Discov. Ther. 3, 221–227.
Hoffman, D. L., Dukes, E. M., and Wittchen, H. U. (2008). Human and economic burden of generalized anxiety disorder. Depress. Anxiety 25, 72–90. doi: 10.1002/da.20257
Holmes, A., and Rodgers, R. J. (2003). Prior exposure to the elevated plus-maze sensitizes mice to the acute behavioral effects of fluoxetine and phenelzine. Eur. J. Pharmacol. 459, 221–230. doi: 10.1016/S0014-2999(02)02874-1
Hsieh, S.-C., Fang, S.-H., Rao, Y. K., and Tzeng, Y.-M. (2008). Inhibition of pro-inflammatory mediators and tumor cell proliferation by Anisomeles indica extracts. J. Ethnopharmacol. 118, 65–70. doi: 10.1016/j.jep.2008.03.003
Huang, H.-C., Lien, H.-M., Ke, H.-J., Chang, L.-L., Chen, C.-C., and Chang, T.-M. (2012). Antioxidative characteristics of Anisomeles indica extract and inhibitory effect of ovatodiolide on melanogenesis. Int. J. Mol. Sci. 13, 6220–6235. doi: 10.3390/ijms13056220
Hunskaar, S., and Hole, K. (1987). The formalin test in mice: dissociation between inflammatory and non-inflammatory pain. Pain 30, 103–114. doi: 10.1016/0304-3959(87)90088-1
Jeong, H. J., Mitchell, V. A., and Vaughan, C. W. (2012). Role of 5-HT1 receptor subtypes in the modulation of pain and synaptic transmission in rat spinal superficial dorsal horn. Br. J. Pharm. 165, 1956–1965. doi: 10.1111/j.1476-5381.2011.01685.x
Julius, D., and Basbaum, A. I. (2001). Molecular mechanisms of nociception. Nature 413, 203–210. doi: 10.1038/35093019
Katz, R. J., Roth, K. A., and Carroll, B. J. (1981). Acute and chronic stress effects on open field activity in the rat: implications for a model of depression. Neurosci. Biobehav. Rev. 5, 247–251. doi: 10.1016/0149-7634(81)90005-1
Kaur, A., Nain, P., and Nain, J. (2012). Herbal plants used in treatment of rheumatoid arthritis: a review. Int. J. Pharm. Pharm. Sci. 4, 44–57.
Khan, H., Saeed, M., Khan, M. A., Dar, A., and Khan, I. (2010). The antinociceptive activity of Polygonatumverticillatum rhizomes in pain models. J. Ethnopharmacol. 127, 521–527. doi: 10.1016/j.jep.2009.10.003
Khurana, N., Ishar, M. P. S., Gajbhiye, A., and Goel, R. K. (2011). PASS assisted prediction and pharmacological evaluation of novel nicotinic analogs for nootropic activity in mice. Eur. J. Pharmacol. 662, 22–30. doi: 10.1016/j.ejphar.2011.04.048
Kimiskidis, V. K., Triantafyllou, N. I., Kararizou, E., Gatzonis, S., Fountoulakis, K. N., Siatouni, A., et al. (2007). Depression and anxiety in epilepsy: the association with demographic and seizure-related variables. Ann. Gen. Psychiatry 6:28. doi: 10.1186/1744-859X-6-28
Kõks, S., Beljajev, S., Koovit, I., Abramov, U., Bourin, M., and Vasar, E. (2001). 8-OH-DPAT, but not deramciclane, antagonizes the anxiogenic-like action of paroxetine in an elevated plus-maze. Psychopharmacology 153, 365–372. doi: 10.1007/s002130000594
Koster, R., Anderson, M., and De Beer, E. J. (1959). “Acetic acid for analgesic screening,” in Federation Proceedings, 412–417.
Kowalski, J., Samojedny, A., Paul, M., Pietsz, G., and Wilczok, T. (2005). Effect of apigenin, kaempferol and resveratrol on the expression of interleukin-1beta and tumor necrosis factor-alpha genes in J774.2 macrophages. Pharmacol. Rep. 57, 390–394.
Kumar, S., Bajwa, B., Kuldeep, S., and Kalia, A. (2013). Anti-inflammatory activity of herbal plants: a review. Int. J. Adv. Pharm. Biol. Chem. 2, 272–281.
Lenardão, E. J., Savegnago, L., Jacob, K. G., Victoria, F. N., and Martinez, D. M. (2016). Antinociceptive effect of essential oils and their constituents: an update review. J. Braz. Chem. Soc. 27, 435–474. doi: 10.5935/0103-5053.20150332
Mazzon, E., Esposito, E., Di Paola, R., Muia, C., Crisafulli, C., Genovese, T., et al. (2008). Effect of tumour necrosis fator alpha receptor 1genetic deletion on Cg-induced acute inflammation: a comparison with etanercept. Clin. Exp. Immunol. 153, 136–149. doi: 10.1111/j.1365-2249.2008.03669.x
Middleton, E., Kandaswami, C., and Theoharides, T. C. (2000). The effects of plant flavonoids on mammalian cells: implications for inflammation, heart disease and cancer. Pharmacol. Rev. 52, 673–751.
Mohuya Mojumdar, A., and Kabir, M. S. H. (2016). Molecular docking and pass prediction for analgesic activity of some isolated compounds from acalypha indica l and adme/t property analysis of the compounds. World J. Pharm. Res. 5, 1761–1770.
Morrison, L. J., and Morrison, R. S. (2006). Palliative care and pain management. Med. Clin. 90, 983–1004. doi: 10.1016/j.mcna.2006.05.016
Natarajan, A., Sugumar, S., Bitragunta, S., and Balasubramanyan, N. (2015). Molecular docking studies of (4 Z, 12 Z)-cyclopentadeca-4, 12-dienone from Grewia hirsuta with some targets related to type 2 diabetes. BMC Complement. Altern. Med. 15:73. doi: 10.1186/s12906-015-0588-5
Ntie-Kang, F., Lifongo, L. L., Mbah, J. A., Owono, L. C. O., Megnassan, E., Mbaze, L. M. A., et al. (2013). In silico drug metabolism and pharmacokinetic profiles of natural products from medicinal plants in the Congo basin. In Silico Pharmacol. 1:12. doi: 10.1186/2193-9616-1-12
Nyeem, M., Alam, M., Awal, M., Mostofa, M., Uddin, S., Islam, N., et al. (2006). CNS depressant effect of the crude ethanolic extract of the flowering tops of Rosa Damascena. Iran. J. Pharm. Ther. 5, 171–174.
Olorunnisola, O. S., Bradley, G., and Afolayan, A. J. (2012). Chemical composition, antioxidant activity and toxicity evaluation of essential oil of Tulbaghia violacea Harv. J. Med. Plants Res. 6, 2340–2347. doi: 10.5897/JMPR11.843
Pellow, S., and File, S. E. (1986). Anxiolytic and anxiogenic drug effects on exploratory activity in an elevated plus-maze: a novel test of anxiety in the rat. Pharmacol. Biochem. Behav. 24, 525–529. doi: 10.1016/0091-3057(86)90552-6
Prasad, A. K., Kumar, V., Arya, P., Kumar, S., Dabur, R., and Singh, N. (2005). Investigations toward new lead compounds from medicinally important plants. Pure Appl. Chem. 77, 25–40. doi: 10.1351/pac200577010025
Rahman, M. A., Uddin, S., and Wilcock, C. (2007). Medicinal plants Used by Chakma tribe in Hill Tracts Districts of Bangladesh. CSIR.
Rao, Y. K., Fang, S.-H., Hsieh, S.-C., Yeh, T.-H., and Tzeng, Y.-M. (2009). The constituents of Anisomeles indica and their anti-inflammatory activities. J. Ethnopharmacol. 121, 292–296. doi: 10.1016/j.jep.2008.10.032
Rudomin, P. (2009). In search of lost presynaptic inhibition. Exp. Brain Res. 196, 139–151. doi: 10.1007/s00221-009-1758-9
Sakiyama, Y., Sujaku, T., and Furuta, A. (2008). A novel automated method for measuring the effect of analgesics on formalin-evoked licking behavior in rats. J. Neurosci. Methods 167, 167–175. doi: 10.1016/j.jneumeth.2007.08.003
Saleem, A., Hidayat, M. T., Jais, A. M., Fakurazi, S., Moklas, M. M., Sulaiman, M., et al. (2011). Antidepressant-like effect of aqueous extract of Channa striatus fillet in mice models of depression. Eur. Rev. Med. Pharmacol. Sci. 15, 795–802.
Sarris, J., Panossian, A., Schweitzer, I., Stough, C., and Scholey, A. (2011). Herbal medicine for depression, anxiety and insomnia: a review of psychopharmacology and clinical evidence. Eur. Neuropsychopharmacol. 21, 841–860. doi: 10.1016/j.euroneuro.2011.04.002
Schmitt, D. E., Hill, R. H., and Grillner, S. (2004). The spinal GABAergic system is a strong modulator of burst frequency in the lamprey locomotor network. J. Neurophysiol. 92, 2357–2367. doi: 10.1152/jn.00233.2004
Singla, D., Sharma, A., Kaur, J., Panwar, B., and Raghava, G. P. (2010). BIAdb: a curated database of benzylisoquinoline alkaloids. BMC Pharmacol. 10:4. doi: 10.1186/1471-2210-10-4
Sousa, F., Melo, C., Monteiro, A., Lima, V., Gutierrez, S., Pereira, B., et al. (2004). Antianxiety and antidepressant effects of riparin III from Aniba riparia (Nees) Mez (Lauraceae) in mice. Pharmacol. Biochem. Behav. 78, 27–33. doi: 10.1016/j.pbb.2004.01.019
Takagi, K., Watanabe, M., and Saito, H. (1971). Studies of the spontaneous movement of animals by the hole cross test; effect of 2-dimethyl-aminoethanol and its acyl esters on the central nervous system. Jpn. J. Pharmacol. 21, 797–810. doi: 10.1254/jjp.21.797
Uddin, M. J., Abdullah-Al-Mamun, M., Biswas, K., Asaduzzaman, M., and Rahman, M. M. (2016). Assessment of anticholinesterase activities and antioxidant potentials of Anisomeles indica relevant to the treatment of Alzheimer's disease. Orient. Pharm. Exp. Med. 16, 113–121. doi: 10.1007/s13596-016-0224-z
Ulhe, S., and Narkhede, S. (2013). Histological and phytochemical studies on aromatic plant, Anisomeles indica (L.) of family Lamiaceae (MS) India. Int. J. Life Sci. 1, 270–272.
Veeramachaneni, G. K., Raj, K. K., Chalasani, L. M., Annamraju, S. K., JS, B., and Talluri, V. R. (2015). Shape based virtual screening and molecular docking towards designing novel pancreatic lipase inhibitors. Bioinformation 11:535. doi: 10.6026/97320630011535
Wang, Y. C., and Huang, T. L. (2005). Screening of anti-Helicobacter pylori herbs deriving from Taiwanese folk medicinal plants. Pathog. Dis. 43, 295–300. doi: 10.1016/j.femsim.2004.09.008
Wirth, J. H., Hudgins, J. C., and Paice, J. A. (2005). Use of herbal therapies to relieve pain: a review of efficacy and adverse effects. Pain Manage. Nurs. 6, 145–167. doi: 10.1016/j.pmn.2005.08.003
Yaksh, T. L., and Wallace, M. S. (2011). “Opioids, analgesia, and pain management,” in Goodman & Gilman's The Pharmacological Basis of Therapeutics, eds L. Brunton, B. Chabner, and B. Knollman (New York, NY: McGraw-Hill Medical), 481–526.
Keywords: Anisomeles indica, writhing test, central pain, peripheral pain, neuropharmacology
Citation: Uddin MJ, Ali Reza ASM, Abdullah-Al-Mamun M, Kabir MSH, Nasrin MS, Akhter S, Arman MSI and Rahman MA (2018) Antinociceptive and Anxiolytic and Sedative Effects of Methanol Extract of Anisomeles indica: An Experimental Assessment in Mice and Computer Aided Models. Front. Pharmacol. 9:246. doi: 10.3389/fphar.2018.00246
Received: 09 November 2017; Accepted: 05 March 2018;
Published: 12 April 2018.
Edited by:
Milica S. Prostran, University of Belgrade, SerbiaReviewed by:
Mariana Spetea, University of Innsbruck, AustriaNevena Divac, University of Belgrade, Serbia
Copyright © 2018 Uddin, Ali Reza, Abdullah-Al-Mamun, Kabir, Nasrin, Akhter, Arman and Rahman. This is an open-access article distributed under the terms of the Creative Commons Attribution License (CC BY). The use, distribution or reproduction in other forums is permitted, provided the original author(s) and the copyright owner are credited and that the original publication in this journal is cited, in accordance with accepted academic practice. No use, distribution or reproduction is permitted which does not comply with these terms.
*Correspondence: Md. Atiar Rahman, YXRpYXJAY3UuYWMuYmQ=