- 1Laboratory of Cancer Biotechnology, Biotechnology Graduate Program, Technology Development Center, Federal University of Pelotas, Pelotas, Rio Grande do Sul, Brazil
- 2Pharmaceutical Sciences, Federal University of Rio Grande do Sul, Porto Alegre, RS, Brazil; Institute of Chemistry, Federal University of Rio Grande do Sul, Porto Alegre, Rio Grande do Sul, Brazil
- 3Pharmaceutical Sciences, Federal University of Rio Grande do Sul, Porto Alegre, Rio Grande do Sul, Brazil
Mycobacterium bovis bacillus Calmette–Guerin (BCG) remains at the forefront of immunotherapy for treating bladder cancer patients. However, the incidence of recurrence and progression to invasive cancer is commonly observed. There are no established effective intravesical therapies available for patients, whose tumors recur following BCG treatment, representing an important unmet clinical need. In addition, there are very limited options for patients who do not respond to or tolerate chemotherapy due to toxicities, resulting in poor overall treatment outcomes. Within this context, nanotechnology is an emergent and promising tool for: (1) controlling drug release for extended time frames, (2) combination therapies due to the ability to encapsulate multiple drugs simultaneously, (3) reducing systemic side effects, (4) increasing bioavailability, (5) and increasing the viability of various routes of administration. Moreover, bladder cancer is often characterized by high mutation rates and over expression of tumor antigens on the tumor cell surface. Therapeutic targeting of these biomolecules may be improved by nanotechnology strategies. In this mini-review, we discuss how nanotechnology can help overcome current obstacles in bladder cancer treatment, and how nanotechnology can facilitate combination chemotherapeutic and BCG immunotherapies for the treatment of non-muscle invasive urothelial bladder cancer.
Introduction
Bladder cancer (BC) is the second most common malignancy of the urinary tract, the fourth most common cancer in men with a yearly incidence rate of 330, 380 cases, and the 11th most common among women with a yearly incidence rate of 99,413. Worldwide, carcinomas of the bladder represent the ninth most common cause of cancer, with 430,000 patients diagnosed with BC annually (Jemal et al., 1999; Ferlay et al., 2013). The incidence of BC also increases with advancing age, as 90% of new diagnoses are made in people over the age of 55 (average age of 73 years at diagnosis) (American Cancer Society, 2017).
Most (75%) BC cases are non-muscle-invasive bladder cancers (NMIBC) at diagnosis with the other 25% representing muscle invasive bladder cancers (MIBC) or metastatic cancers (Moch et al., 2016). Urothelial carcinomas can be categorized as low grade or high grade according to their architectural and cytological atypia and include papillary urothelial neoplasm or low malignant potential (Cheng et al., 2012). Pathological assessment is the gold standing for tumor classification. Ta (non-invasive papillary) and Tis [carcinoma in situ (CIS)] are tumors that are restricted to the mucosa, while T1 and T2 are tumors that invade the lamina propria and the muscularis propria, respectively (Sobin and Gospodarowicz, 2009).
Initial BC treatments involve transurethral resection (TURBT) to facilitate removal of the visible tumor (Hall et al., 2007; Sylvester, 2008; Babjuk et al., 2013). Further therapy is dependent on pathologic stage and grade of the tumor and often mediated through intravesical instillation. Although the response rate to therapy in patients with NMIBC is high (~80%), 50–90% of NMIBC patients suffer from recurrence within 5 years, with muscle invasion found in up to 20% of recurrent patients (Rübben et al., 1988; Lamm and Allaway, 2000; Hussain et al., 2009). This review focuses on currently available BC therapies and describes nanotechnology tools to enhance therapeutic effects and overcome side effects, emphasizing its use to improve BCG immunotherapy.
Bladder Cancer Treatments
Following TURBT, a single intravesical chemotherapy treatment is recommended for patients with low to intermediate risk NMIBC, with mitomycin, epirubicin, and gemcitabine representing common drugs of choice (Kamat et al., 2016). It has been shown that the relative risk for tumor recurrence is reduced by 50% if the chemotherapy instillation is given with 24 h after TURBT (Kaasinen et al., 2002).
Intravesical immunotherapy with Mycobacterium bovis Bacillus Calmette-Guérin (BCG) is the treatment of choice for patients with high-risk NMIBC. BCG immunotherapy is the gold standard treatment for NMIBC due to its ability to reduce recurrence and progression to MIBC (Ahn et al., 2014). A meta-analysis with individual patient data comparing BCG immunotherapy with intravesical mitomycin chemotherapy has shown BCG to be superior in terms of reducing recurrence and delaying disease progression; however, no significant differences in progression or overall survival were observed (Malmström et al., 2009). Conventional BCG treatment consists of a percutaneous BCG vaccine administered 2–6 weeks after TURBT followed by 6 weekly courses of intravesical BCG administration (Morales et al., 1976; Kresowik, 2009; Kamat et al., 2015). However, specific BCG substrain preferences, schedules, and dosages differ across geographic regions due the wide range of BCG substrains licensed for human tuberculosis vaccination and BCG immunotherapy (Gan et al., 2013). Based on multiple meta-analyses, it is recommended to continue BCG therapy for 1 to 3 years if tolerated by the patient to decrease recurrence and progression of NMIBC (Shelley et al., 2001; Sylvester et al., 2002; Böhle and Bock, 2004; Hall et al., 2007; Gontero et al., 2010; Babjuk et al., 2013).
Despite these guidelines, it is estimated that 20% of patients with high-risk NMIBC treated with BCG will progress to muscle invasion or suffer from NMIBC recurrence within 5 years (Rübben et al., 1988). MIBC is a major clinical issue due to its aggressiveness and high 5 year mortality rate. To maximize survival rates, radical cystectomy (RC) represents the best treatment option. RC consists of removal of the bladder, prostate, seminal vesicles, proximal vas deferens, and proximal urethra in men, and bladder, uterus, ovaries, fallopian tubes, urethra, and part of vagina in women (Arcangeli et al., 2015). Many patients cannot tolerate the morbidity of RC and instead opt for continued local therapy as an effort to spare their bladder (Ahn et al., 2014). Some studies have shown progress with the use of Mytomicin C (van der Heijden et al., 2004; Halachmi et al., 2011), Gemcitabine (Skinner et al., 2013), Valrubicin (Steinberg et al., 2000), Docetaxel (Barlow et al., 2013), Nab-Paclitaxel (McKiernan et al., 2011), mycobacterial cell wall extract (Morales et al., 2009), EGFR (Rebouissou et al., 2014) and a combination therapy of Gemcitabine and Mytomicin C for the treatment of BC (Lightfoot et al., 2011). More recently, trimodal treatment with simultaneous delivery of chemotherapy and radiotherapy has emerged as an effective bladder sparing treatment with similar survival rates compared to RC (Arcangeli et al., 2015). However, none of these local therapies have shown to be more effective for BC control than early RC (Ahn et al., 2014).
Chemotherapy Optimization Using Nanotechnology for Bladder Cancer Therapy
Nanotechnology consists in the study and application of materials on the nanometer scale (Ebbesen and Jensen, 2006) and application of nanotechnology in the medical field is referred to as nanomedicine (Sweeney, 2015). Nanotechnology have proven to be a powerful tool for the development of new chemotherapies or immunotherapies for BC. The development of new drug delivery systems has been growing and is expected to continue to increase over the next few years (Brito et al., 2017).
In this context, several studies have utilized nanoparticles (NPs) to increase the therapeutic effectiveness and reduce adverse effects of chemotherapy by targeting chemotherapeutic agents to a specific tissue and increasing its bioavailability (Yurgel et al., 2014; Kang et al., 2017; Yao et al., 2017; Zhu et al., 2017). Polysaccharide-based NPs loaded with Mitomycin C and surrounded by the bioadhesive polymer chitosan mixed with polylactic acid or with poly(ε-caprolactone) have been utilized in an attempt to optimize BC treatments. This NP promoted favorable drug loading and release profiles along with improved anticancer efficacy and cellular interactions (Bilensoy et al., 2009). Erdogar et al. (2012) has also demonstrated that bioadhesive and cationic NPs loaded with Mitomycin C are able to increased exposure of the bladder to the drug resulting in a drug reservoir at the action site, which might improve local treatment (Erdogar et al., 2012). In addition, cationic core-shell nanoparticles loaded with Mitomycin C have also improved antitumor efficacy in tumor-induced rat models (Erdogar et al., 2014).
Magnetic NPs (MNPs) also show promise for delivery of quimiotherapic agents to the target tissue (Stapf et al., 2017). To limit doxorubicin's (Dox) cytotoxic effects on healthy cells, MNPs (iron oxide) were conjugated with Dox to ensure efficient delivery to cancer sites, resulting in increased BC sensitivity compared to Dox alone (Nowicka et al., 2013). In addition, monoclonal antibodies (mABs) bound to MNPs can increase the ability of MNPs to target BC cells and enable thermotherapy to cope with BC recurrence (Rezaei et al., 2017).
NPs loaded with molecules with high urothelium mucoadhesivity is another approach used to optimize the delivery of molecules to the target tissue. To increase Dox specificity for BC cells, thiol-functionalized NPs loaded with Dox were synthesized and induced cytotoxicity against UMUC3 cancer cells (Zhang et al., 2014). Besides the possibility of conjugation with different molecules, studies have also reported the importance of developing methods to modify the surface of mesoporous silica NPs to enhance the antineoplasic effects of Dox on BC (Wei et al., 2017). An enhanced therapeutic effect against UMUC3 cells was also demonstrated using Dox and peptide-modified cisplatin synergistically loaded onto positively charged mucoadhesive chitosan–polymethacrylic acid nanocapsules (Lu et al., 2016).
In addition, platinum agents can be loaded onto a variety of polymeric, lipid, and inorganic nanocarriers, including liposomes, NPs, and nanotubes to increase their antitumoral effects (Browning et al., 2017). The use of cisplatin nanocarriers is associated with reduced toxicity and adverse events (Sudha et al., 2017); however, novel strategies are required to increase drug uptake and release at the target site. In this regard, cisplatin NPs were evaluated in a preclinical study against NMIBC and cisplatin-loaded biocompatible poly(L-aspartic acid sodium salt) (PAA) NPs demonstrated potential for improved intravesical treatment of NMIBC while reducing local and systemic side effect (Kates et al., 2017).
Nanotechnology tools have also been used in clinical trials. Albumin-bound-Paclitaxel NPs showed minimal toxicity and systemic absorption when used to treat NMIBC during the first human intravesical phase I trial (McKiernan et al., 2011). In addition, phase II trials have demonstrated minimal toxicity of intravesical nab-paclitaxel in NMIBC patients with a response rate of 35.7% (Mckiernan et al., 2014). The formulation of albumin-bound-paclitaxel NPs has also been used for the first time to treat unresectable metastatic urethral cancer. Follwoing therapy, a 70% reduction in the size of the tumor was observed in addition to 19 months of progression free survival (Abaza and Alemany, 2014).
RNAi Optimization of Nanotechnology Bladder Cancer Therapy
The use of interference RNA (RNAi) combined with nanotechnology is another promising approach for BC treatment. RNAi technology can be used to inhibit tumor growth through messenger RNA inhibition of several activated oncogenes (Xin et al., 2017). In human BC, some upregulated genes associated to the development of resistance to chemotherapy have been inhibited using RNAi technology through knockdown of the target gene (Pan et al., 2016; Wang et al., 2017). Within this context, RNAi technology is a highly effective approach to combat chemoresistance and improve advanced BC outcomes.
Although RNAi technology could be used to overcome multidrug resistance and restore cells sensitivity, there are several challenges associated with RNAi delivery to diseased sites for gene therapy (Melamed et al., 2017). NPs appear to be a promising tool to help overcome existing biological barriers to RNAi delivery. Through this approach, studies have reported upregulation of BC specific genes, which can be effective targets of NP-siRNA therapeutic approaches (Seth et al., 2011; Müller et al., 2016).
Small dsRNAs, known as small activating RNA (saRNA), produce the opposite effect of RNAi by inducing gene expression (Li et al., 2006; Chen et al., 2008). The therapeutic potential of dsRNA (P21-322) coupled with nanotechnology has been demonstrated using a 20-fluoro-modified derivative loaded into lipid NPs in an orthotopic model of BC. Antitumoral activity and induction of p21 expression was confirmed in vitro and in vivo (Kang et al., 2012), suggesting induction of specific genes can provide an alternative route to BC treatment.
Functionalization of Nanoparticles to Optimize Bladder Cancer Therapy
The functionalization of nanoparticles with monoclonal antibodies is a promising strategy for targeted delivery to and uptake by cells overexpressing the antigens specific for these antibodies (Eloy et al., 2017). Development of molecules that exhibit affinity for targets expressed on tumor cells represents an emerging therapeutic approach (Diesendruck and Benhar, 2017). For example, the receptor tyrosine kinase EGFR exhibits altered expression in several types of solid tumors and its overexpression in UC is directly correlated with advanced tumor stages (Kassouf et al., 2008). The incidence of EGFR positivity in epithelial tumors varies by tumor type. BC is often characterized by a high mutation rate and high EGFR expression in approximately 50% of cases (Colquhoun and Mellon, 2002). Thanks to the expression of EGRF on BC cells, an anti-EGFR monoclonal antibody conjugated to gold nanorods was able to effectively bind EGFR-expressing BC cells and reduce the systemic exposure and clearance of nanoparticles from the body (Yang et al., 2015). These results indicate the use of monoclonal antibodies represents another possible approach for delivery of molecules to specific tissues.
Nano-BCG: Optimizing BCG Immunotherapy Using Nanotechnology to Treat Bladder Cancer
As previously mentioned, BCG is considered the standard treatment for NMIBC. However, BCG immunotherapy is associated with frequent induction of adverse effects in patients (Poletajew et al., 2017) leading researchers to investigate novel alternatives to increase their effectiveness (Begnini et al., 2013). Delivery systems and nanotechnological approaches are interesting tools to improve currently available BCG therapies and prolong exposure of the bladder tissue. The main advances of nanotechnology tools for improvement of BCG immunotherapies against BC are listed in Table 1.
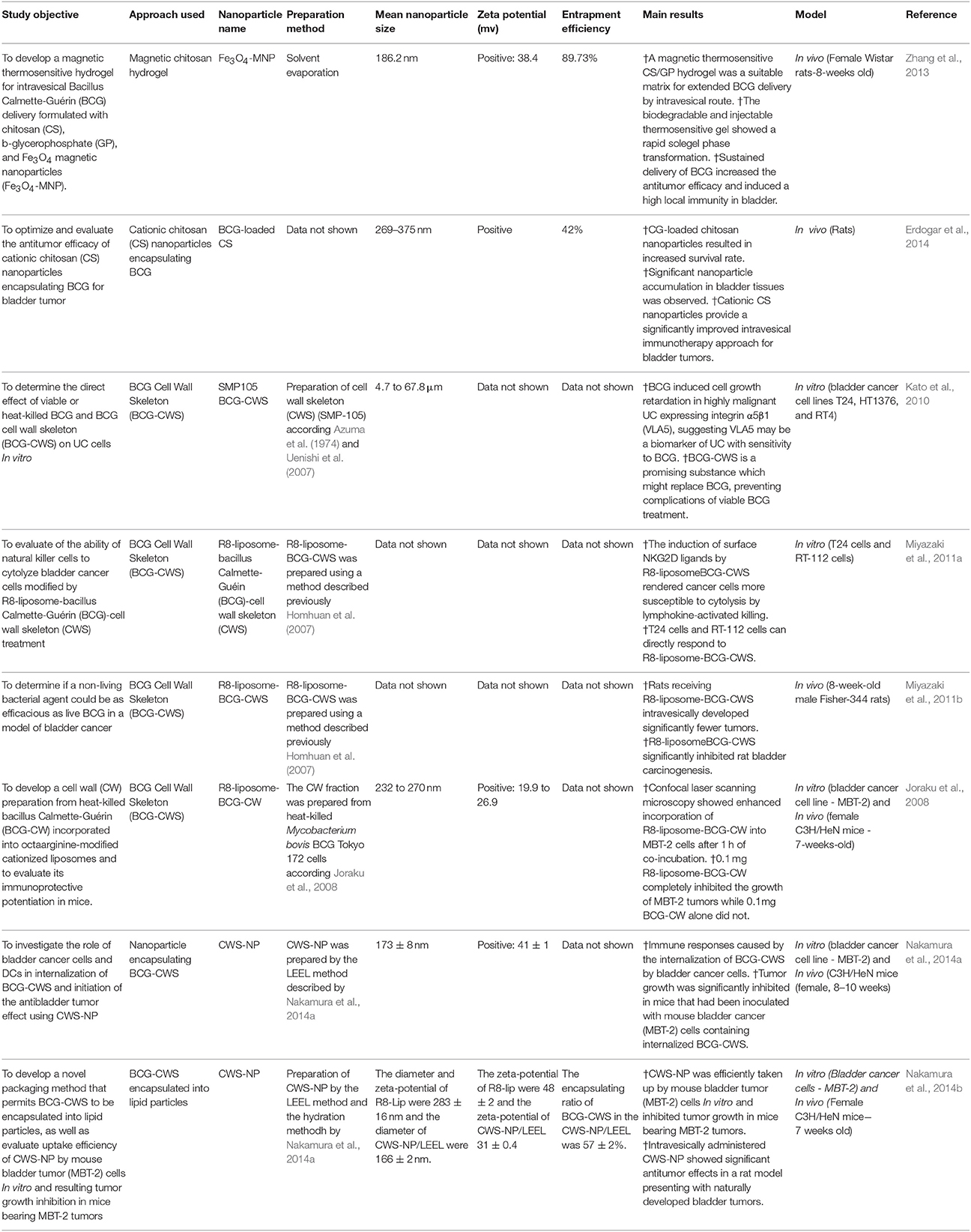
Table 1. Major studies involving nanotechnology tools applied to BCG immunotherapy against bladder cancer.
Cationic Chitosan (CS) NPs encapsulating BCG developed with the purpose of increasing the antitumor efficacy of BCG following intravesical administration have demonstrated significant advantages for the treatment of BC (Erdogar et al., 2014). Application of magnetic thermosensitive hydrogel developed with chitosan has been shown to be effective in increasing exposure of the bladder to BCG in addition to potentializing its immunological response (Zhang et al., 2013).
With the goal of overcoming side effects resulting from the administration of viable BCG, BCG cell wall skeleton (BCG-CWS) used as an immunomodulator in cancer patients (Uenishi et al., 2007, 2009; Hayashi et al., 2009) was shown to effectively control cell proliferation in UC, representing an efficient and safe alternative to BC immunotherapy (Kato et al., 2010). Nakamura et al. (2014a) was the first to develop a nanoencapsulated BCG-CWS (CWS-NP) using a liposome evaporated emulsified lipid (LEEL) method, resulting in a strong antitumor effect against MBT-2 BC cells as well as in vivo induced tumors (Nakamura et al., 2014a). CWS-NP was also shown to produce significant antitumor effects through internalization of BCG-CWS in BC cells, which contributes to the initiation of antitumor immunological activity (Nakamura et al., 2014b).
Another nanotechnological approach applied to BCG therapy consists of the use of modified nanoparticles incorporating BCG cell wall (BCG-CW) or skeleton. Utilization of octaarginine-modified liposomes incorporating BCG-CW (R8-liposome-BCG-CW) results in increased immunotherapeutic potential of BCG-CW for NMIBC through cellular internalization resulting in growth inhibition in vivo (Joraku et al., 2008). R8-liposome-BCG-CWS has also be used to investigate the suppressive effects of liposomes using a rat N-butyl-N-(4-hydroxybutyl) nitrosamine (BBN) induced BC model. This approach demonstrated that R8-liposome-BCG-CWS displays inhibitory effects against CD in vivo (Miyazaki et al., 2011b). Using this same approach, other studies have demonstrated that R8-liposome-BCG-CWS treatment results in induction of surface specific ligands (NKG2D) in BC cells, making them more susceptible to lymphokine-activated killing (LAK), indicating these cells are affected by R8-liposome BCG-CWS administration (Miyazaki et al., 2011a). These results demonstrate the efficiency of nanotechnology applications for optimization and development of novel BCG immunotherapy approaches for BC.
Perspective
Considering the promising results demonstrated by utilizing nanotechnology to develop new BC therapies, including success application of those approaches to BCG therapy, we believe that nanotechnology will provide significant advances for improving BC treatment. In this review, we described the main advances and applications of nanotechnology tools for development of novel treatments against BC, providing evidence that nanotechnology has contributed greatly to this effort by controlling drug release for longer periods, enabling the encapsulation of multiple drugs simultaneously, decreasing side effects, and increasing bioavailability. Nevertheless, we believe that combination therapies show more promise for effective treatment of this complex disease than individual approaches (Figure 1).
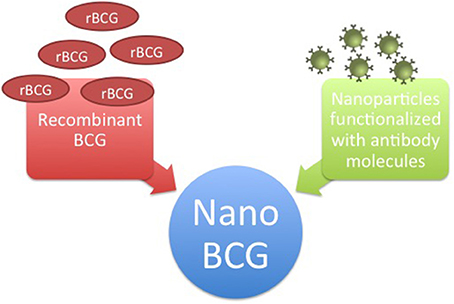
Figure 1. Combination of therapeutic approaches for bladder cancer treatment. Nano BCG has gain of effect by combination with recombinant BCG or by functionalization of nanoparticles with antibodies molecules.
Although nanotechnology has resulted in significant progress for BCG immunotherapy, including increasing immunotherapeutic effects, prolonging exposure of the bladder tissue, and reducing adverse side effects, we believe this approach can be further enhanced through intravesical BCG delivery using NPs functionalized with antibody molecules against highly expressed receptors on the surface of BC cells such as EGFR to further target drug delivery and avoid systemic exposure and clearance of NPs from the body. Therefore, considering the emerging and motivating results using these approaches to treat BC, we believe that BCG delivery using NPs functionalized with monoclonal antibodies, in particular anti-EGFR, will provide a significantly improved delivery system for treatment of human BC.
Author Contributions
JB drafted the manuscript. CB wrote the introduction section, KB wrote the bladder cancer treatments section, and all authors, mainly AP, SG, TC, FS, and KB were responsible for conception, manuscript review, and critical intellectual input.
Conflict of Interest Statement
The authors declare that the research was conducted in the absence of any commercial or financial relationships that could be construed as a potential conflict of interest.
References
Abaza, Y. M., and Alemany, C. (2014). Nanoparticle albumin-bound-paclitaxel in the treatment of metastatic urethral adenocarcinoma: the significance of molecular profiling and targeted therapy. Case Rep. Urol. 2014, 1–3. doi: 10.1155/2014/489686
Ahn, J. J., Ghandour, R. A., and McKiernan, J. M. (2014). New agents for bacillus Calmette-Guérin-refractory nonmuscle invasive bladder cancer. Curr. Opin. Urol. 24, 540–545. doi: 10.1097/MOU.0000000000000088
Arcangeli, G., Strigari, L., and Arcangeli, S. (2015). Radical cystectomy versus organ-sparing trimodality treatment in muscle-invasive bladder cancer: a systematic review of clinical trials. Crit. Rev. Oncol. Hematol. 95, 387–396. doi: 10.1016/j.critrevonc.2015.04.006
Azuma, I., Ribi, E. E., Meyer, T. J., and Zbar, B. (1974). Biologically active components from mycobacterial cell walls. I. Isolation and composition of cell wall skeleton and component P3. J. Natl. Cancer Inst. 52, 95–101. doi: 10.1093/jnci/52.1.95
Babjuk, M., Burger, M., Zigeuner, R., Shariat, S. F., van Rhijn, B. W. G., Compérat, E., et al. (2013). EAU guidelines on non-muscle-invasive Urothelial carcinoma of the bladder: update 2013. Eur. Urol. 64, 639–653. doi: 10.1016/j.eururo.2013.06.003
Barlow, L. J., McKiernan, J. M., and Benson, M. C. (2013). Long-term survival outcomes with intravesical docetaxel for recurrent nonmuscle invasive bladder cancer after previous bacillus Calmette-Guérin therapy. J. Urol. 189, 834–839. doi: 10.1016/j.juro.2012.10.068
Begnini, K. R., Rizzi, C., Campos, V. F., Borsuk, S., Schultze, E., Yurgel, V. C., et al. (2013). Auxotrophic recombinant Mycobacterium bovis BCG overexpressing Ag85B enhances cytotoxicity on superficial bladder cancer cells in vitro. Appl. Microbiol. Biotechnol. 97, 1543–1552. doi: 10.1007/s00253-012-4416-2
Bilensoy, E., Sarisozen, C., Esendagli, G., Dogan, A. L., Aktaş, Y., Sen, M., et al. (2009). Intravesical cationic nanoparticles of chitosan and polycaprolactone for the delivery of Mitomycin C to bladder tumors. Int. J. Pharm. 371, 170–176. doi: 10.1016/j.ijpharm.2008.12.015
Böhle, A., and Bock, P. R. (2004). Intravesical bacille Calmette-Guérin versus mitomycin C in superficial bladder cancer: formal meta-analysis of comparative studies on tumor progression. Urology 63, 682–686; discussion 686–687. doi: 10.1016/j.urology.2003.11.049
Brito, O. M., Mônica, V. N., and Luciano, B. M. (2017). A review of recent developments on micro/nanostructured pharmaceutical systems for intravesical therapy of the bladder cancer. Pharm. Dev. Technol. 12, 1–15. doi: 10.1080/10837450.2017.1312441
Browning, R. J., Reardon, P. J. T., Parhizkar, M., Pedley, R. B., Edirisinghe, M., Knowles, J. C., et al. (2017). Drug delivery strategies for platinum based chemotherapy. ACS Nano 11, 8560–8578. doi: 10.1021/acsnano.7b04092
Chen, Z., Place, R. F., Jia, Z.-J., Pookot, D., Dahiya, R., and Li, L.-C. (2008). Antitumor effect of dsRNA-induced p21WAF1/CIP1 gene activation in human bladder cancer cells. Mol. Cancer Ther. 7, 698–703. doi: 10.1158/1535-7163.MCT-07-2312
Cheng, L., MacLennan, G. T., and Lopez-Beltran, A. (2012). Histologic grading of urothelial carcinoma: A reappraisal. Hum. Pathol. 43, 2097–2108. doi: 10.1016/j.humpath.2012.01.008
Colquhoun, A. J., and Mellon, J. K. (2002). Epidermal growth factor receptor and bladder cancer: a review. Urol. Int. 48, 365–371. doi: 10.1136/pmj.78.924.584
Diesendruck, Y., and Benhar, I. (2017). Novel immune check point inhibiting antibodies in cancer therapy-opportunities and challenges. Drug Resist. Updat. 30, 39–47. doi: 10.1016/j.drup.2017.02.001
Ebbesen, M., and Jensen, T. G. (2006). Nanomedicine: techniques, potentials, and ethical implications. J. Biomed. Biotechnol. 2006:51516. doi: 10.1155/JBB/2006/51516
Eloy, J. O., Petrilli, R., Trevizan, L. N. F., and Chorilli, M. (2017). Immunoliposomes: a review on functionalization strategies and targets for drug delivery. Colloids Surf. B Biointerfaces 159, 454–467. doi: 10.1016/j.colsurfb.2017.07.085
Erdogar, N., Iskit, A. B., Eroglu, H., Sargon, M. F., Mungan, N. A., and Bilensoy, E. (2014). Cationic core-shell nanoparticles for intravesical chemotherapy in tumor-induced rat model: Safety and efficacy. Int. J. Pharm. 471, 1–9. doi: 10.1016/j.ijpharm.2014.05.014
Erdogar, N., Iskit, A. B., Mungan, N. A., and Bilensoy, E. (2012). Prolonged retention and in vivo evaluation of cationic nanoparticles loaded with Mitomycin C designed for intravesical chemotherapy of bladder tumours. J. Microencapsul. 29, 576–582. doi: 10.3109/02652048.2012.668957
Ferlay, J., Steliarova-Foucher, E., Lortet-Tieulent, J., Rosso, S., Coebergh, J. W. W., Comber, H., et al. (2013). Cancer incidence and mortality patterns in Europe: estimates for 40 countries in 2012. Eur. J. Cancer 49, 1374–1403. doi: 10.1016/j.ejca.2012.12.027
Gan, C., Mostafid, H., Khan, M. S., and Lewis, D. J. M. (2013). BCG immunotherapy for bladder cancer—the effects of substrain differences. Nat. Rev. Urol. 10, 580–588. doi: 10.1038/nrurol.2013.194
Gontero, P., Bohle, A., Malmstrom, P. U., O'Donnell, M. A., Oderda, M., Sylvester, R., et al. (2010). The role of bacillus calmette-guérin in the treatment of non-muscle-invasive bladder cancer. Eur. Urol. 57, 410–429. doi: 10.1016/j.eururo.2009.11.023
Halachmi, S., Moskovitz, B., Maffezzini, M., Conti, G., Verweij, F., Kedar, D., et al. (2011). Intravesical mitomycin C combined with hyperthermia for patients with T1G3 transitional cell carcinoma of the bladder. Urol. Oncol. Semin. Orig. Investig. 29, 259–264. doi: 10.1016/j.urolonc.2009.02.012
Hall, M. C., Chang, S. S., Dalbagni, G., Pruthi, R. S., Seigne, J. D., Skinner, E. C., et al. (2007). Guideline for the management of nonmuscle invasive bladder cancer (stages Ta, T1, and Tis): 2007 update. J. Urol. 178, 2314–2330. doi: 10.1016/j.juro.2007.09.003
Hayashi, A., Nishida, Y., Yoshii, S., Kim, S. Y., Uda, H., and Hamasaki, T. (2009). Immunotherapy of ovarian cancer with cell wall skeleton of Mycobacterium bovis Bacillus Calmette-Guérin: effect of lymphadenectomy. Cancer Sci. 100, 1991–1995. doi: 10.1111/j.1349-7006.2009.01271.x
Homhuan, A., Kogure, K., Akaza, H., Futaki, S., Naka, T., and Fujita, Y. (2007). New packaging method of mycobacterial cell wall using octaarginine-modified liposomes: enhanced uptake by and immunostimulatory activity of dendritic cells. J. Control. Release 120, 60–69. doi: 10.1016/j.jconrel.2007.03.017
Hussain, M. H., Wood, D. P., Bajorin, D. F., Bochner, B. H., Dreicer, R., Lamm, D. L., et al. (2009). Bladder cancer: narrowing the gap between evidence and practice. J. Clin. Oncol. 27, 5680–5684. doi: 10.1200/JCO.2009.23.6901
Jemal, A., Bray, F., and Ferlay, J. (1999). Global Cancer Statistics: 2011. CA Cancer J. Clin. 49, 33–64. doi: 10.3322/canjclin.49.1.33
Joraku, A., Homhuan, A., Kawai, K., Yamamoto, T., Miyazaki, J., Kogure, K., et al. (2008). Immunoprotection against murine bladder carcinoma by octaarginine-modified liposomes incorporating cell wall of Mycobacterium bovis bacillus Calmette-Guérin. BJU Int. 103, 686–693. doi: 10.1111/j.1464-410X.2008.08235.x
Kaasinen, E., Rintala, E., Hellström, P., Viitanen, J., Juusela, H., Rajala, P., et al. (2002). Factors explaining recurrence in patients undergoing chemoimmunotherapy regimens for frequently recurring superficial bladder carcinoma. Eur. Urol. 42, 167–174. doi: 10.1016/S0302-2838(02)00260-9
Kamat, A. M., Flaig, T. W., Grossman, H. B., Konety, B., Lamm, D., O'Donnell, M. A., et al. (2015). Expert consensus document: Consensus statement on best practice management regarding the use of intravesical immunotherapy with BCG for bladder cancer. Nat. Rev. Urol. 12, 225–235. doi: 10.1038/nrurol.2015.58
Kamat, A. M., Hahn, N. M., Efstathiou, J. A., Lerner, S. P., Malmström, P. U., Choi, W., et al. (2016). Bladder cancer. Lancet 388, 2796–2810. doi: 10.1016/S0140-6736(16)30512-8
Kang, M. R., Yang, G., Place, R. F., Charisse, K., Epstein-Barash, H., Manoharan, M., et al. (2012). Intravesical delivery of small activating RNA formulated into lipid nanoparticles inhibits orthotopic bladder tumor growth. Cancer Res. 72, 5069–5079. doi: 10.1158/0008-5472.CAN-12-1871
Kang, S. H., Revuri, V., Lee, S.-J., Cho, S., Park, I.-K., Cho, K. J., et al. (2017). Oral siRNA delivery to treat colorectal liver metastases. ACS Nano 11, 10417–10429. doi: 10.1021/acsnano.7b05547
Kassouf, W., Black, P. C., Tuziak, T., Bondaruk, J., Lee, S., Brown, G. A., et al. (2008). Distinctive expression pattern of erbb family receptors signifies an aggressive variant of bladder cancer. J. Urol. 179, 353–358. doi: 10.1016/j.juro.2007.08.087
Kates, M., Date, A., Yoshida, T., Afzal, U., Kanvinde, P., Babu, T., et al. (2017). Preclinical evaluation of intravesical cisplatin nanoparticles for non-muscle-invasive bladder cancer. Clin. Cancer Res. 23, 6592–6601. doi: 10.1158/1078-0432.CCR-17-1082
Kato, T., Bilim, V., Yuuki, K., Naito, S., Yamanobe, T., Nagaoka, A., et al. (2010). Bacillus Calmette-Guérin and BCG cell wall skeleton suppressed viability of bladder cancer cells in vitro. Anticancer Res. 30, 4089–4096.
Kresowik, T. (2009). Bacillus Calmette-Guerin immunotherapy for urothelial carcinoma of the bladder. Immunotherapy 1, 281–288. doi: 10.2217/1750743X.1.2.281
Lamm, D. L., and Allaway, M. (2000). Current trends in bladder cancer treatment. Ann. Chir. Gynaecol. 89, 234–241.
Li, L.-C., Okino, S. T., Zhao, H., Pookot, D., Place, R. F., Urakami, S., et al. (2006). Small dsRNAs induce transcriptional activation in human cells. Proc. Natl. Acad. Sci. U.S.A. 103, 17337–17342. doi: 10.1073/pnas.0607015103
Lightfoot, A. J., Rosevear, H. M., and O'Donnell, M. A. (2011). Recognition and treatment of BCG failure in bladder cancer. ScientificWorldJournal 11, 602–613. doi: 10.1100/tsw.2011.30
Lu, S., Xu, L., Kang, E. T., Mahendran, R., Chiong, E., and Neoh, K. G. (2016). Co-delivery of peptide-modified cisplatin and doxorubicin via mucoadhesive nanocapsules for potential synergistic intravesical chemotherapy of non-muscle-invasive bladder cancer. Eur. J. Pharm. Sci. 84, 103–115. doi: 10.1016/j.ejps.2016.01.013
Malmström, P. U., Sylvester, R. J., Crawford, D. E., Friedrich, M., Krege, S., Rintala, E., et al. (2009). An individual patient data meta-analysis of the long-term outcome of randomised studies comparing intravesical mitomycin C versus Bacillus Calmette-Guérin for non-muscle-invasive bladder cancer. Eur. Urol. 56, 247–256. doi: 10.1016/j.eururo.2009.04.038
McKiernan, J. M., Barlow, L. J., Laudano, M. A., Mann, M. J., Petrylak, D. P., and Benson, M. C. (2011). A phase I trial of intravesical nanoparticle albumin-bound paclitaxel in the treatment of bacillus Calmette-Guérin refractory nonmuscle invasive bladder cancer. J. Urol. 186, 448–451. doi: 10.1016/j.juro.2011.03.129
Mckiernan, J. M., Holder, D. D., Ghandour, R. A., Barlow, L. J., Ahn, J. J., Kates, M., et al. (2014). Phase II trial of intravesical nanoparticle albumin bound paclitaxel for the treatment of nonmuscle invasive urothelial rin carcinoma of the bladder after bacillus Calmette-Guérin treatment failure. J. Urol. 192, 1633–1638. doi: 10.1016/j.juro.2014.06.084
Melamed, J. R., Riley, R. S., Valcourt, D. M., Billingsley, M. M., Kreuzberger, N. L., and Day, E. S. (2017). Quantification of siRNA duplexes bound to gold nanoparticle surfaces. Methods Mol. Biol. 1570, 1–15. doi: 10.1007/978-1-4939-6840-4_1
Miyazaki, J., Kawai, K., Kojima, T., Oikawa, T., Joraku, A., Shimazui, T., et al. (2011a). The liposome-incorporating cell wall skeleton of Mycobacterium bovis bacillus Calmette-Guérin can directly enhance the susceptibility of cancer cells to lymphokine-activated killer cells through up-regulation of natural-killer group 2, member D ligands. BJU Int. 108, 1520–1526. doi: 10.1111/j.1464-410X.2010.10056.x
Miyazaki, J., Nishiyama, H., Yano, I., Nakaya, A., Kohama, H., Kawai, K., et al. (2011b). The therapeutic effects of R8-liposome-BCG-CWS on BBN-induced rat urinary bladder carcinoma. Anticancer Res. 31, 2065–2071.
Moch, H., Cubilla, A. L., Humphrey, P. A., Reuter, V. E., and Ulbright, T. M. (2016). The 2016 WHO classification of tumours of the urinary system and male genital organs—part A: renal, penile, and testicular tumours. Eur. Urol. 70, 93–105. doi: 10.1016/j.eururo.2016.02.029
Morales, A., Eidinger, D., and Bruce, A. W. (1976). Intracavitary bacillus Calmette-Guerin in the treatment of superficial bladder tumors. J. Urol. 116, 180–182. doi: 10.1016/S0022-5347(17)58737-6
Morales, A., Phadke, K., and Steinhoff, G. (2009). Intravesical mycobacterial cell wall-DNA complex in the treatment of carcinoma in situ of the bladder after standard intravesical therapy has failed. J. Urol. 181, 1040–1045. doi: 10.1016/j.juro.2008.11.019
Müller, K., Klein, P. M., Heissig, P., Roidl, A., and Wagner, E. (2016). EGF receptor targeted lipo-oligocation polyplexes for antitumoral siRNA and miRNA delivery. Nanotechnology 27, 1–15. doi: 10.1088/0957-4484/27/46/464001
Nakamura, T., Fukiage, M., Higuchi, M., Nakaya, A., Yano, I., Miyazaki, J., et al. (2014a). Nanoparticulation of BCG-CWS for application to bladder cancer therapy. J. Control. Release 176, 44–53. doi: 10.1016/j.jconrel.2013.12.027
Nakamura, T., Fukiage, M., Suzuki, Y., Yano, I., Miyazaki, J., Nishiyama, H., et al. (2014b). Mechanism responsible for the antitumor effect of BCG-CWS using the LEEL method in a mouse bladder cancer model. J. Control. Release 196, 161–167. doi: 10.1016/j.jconrel.2014.10.007
Nowicka, A. M., Kowalczyk, A., Jarzebinska, A., Donten, M., Krysinski, P., Stojek, Z., et al. (2013). Progress in targeting tumor cells by using drug-magnetic nanoparticles conjugate. Biomacromolecules 14, 828–833. doi: 10.1021/bm301868f
Pan, J., Li, X., Wu, W., Xue, M., Hou, H., Zhai, W., et al. (2016). Long non-coding RNA UCA1 promotes cisplatin/gemcitabine resistance through CREB modulating miR-196a-5p in bladder cancer cells. Cancer Lett. 382, 64–76. doi: 10.1016/j.canlet.2016.08.015
Poletajew, S., Zapała, P., and Radziszewski, P. (2017). Safety and efficacy of intravesical Bacillus Calmette-Guérin immunotherapy in patients with non-muscle-invasive bladder cancer presenting with asymptomatic bacteriuria: a systematic review. Urol. Int. 99, 1–5. doi: 10.1159/000477673
Rebouissou, S., Bernard-Pierrot, I., de Reyniès, A., Lepage, M.-L., Krucker, C., Chapeaublanc, E., et al. (2014). EGFR as a potential therapeutic target for a subset of muscle-invasive bladder cancers presenting a basal-like phenotype. Sci. Transl. Med. 6:244ra91. doi: 10.1126/scitranslmed.3008970
Rezaei, G., Habibi-Anbouhi, M., Mahmoudi, M., Azadmanesh, K., Moradi-Kalbolandi, S., Behdani, M., et al. (2017). Development of anti-CD47 single-chain variable fragment targeted magnetic nanoparticles for treatment of human bladder cancer. Nanomedicine 12, 597–613. doi: 10.2217/nnm-2016-0302
Rübben, H., Lutzeyer, W., Fischer, N., Deutz, F., Lagrange, W., and Giani, G. (1988). Natural history and treatment of low and high risk superficial bladder tumors. J. Urol. 139, 283–285. doi: 10.1016/S0022-5347(17)42387-1
Seth, S., Matsui, Y., Fosnaugh, K., Liu, Y., Vaish, N., Adami, R., et al. (2011). RNAi-based therapeutics targeting survivin and PLK1 for treatment of bladder cancer. Mol. Ther. 19, 928–935. doi: 10.1038/mt.2011.21
Shelley, M. D., Kynaston, H., Court, J., Wilt, T. J., Coles, B., Burgon, K., et al. (2001). A systematic review of intravesical bacillus Calmette-Guérin plus transurethral resection vs transurethral resection alone in Ta and T1 bladder cancer. BJU Int. 88, 209–216. doi: 10.1046/j.1464-410x.2001.02306.x
Skinner, E. C., Goldman, B., Sakr, W. A., Petrylak, D. P., Lenz, H. J., Lee, C. T., et al. (2013). SWOG S0353: Phase II trial of intravesical gemcitabine in patients with nonmuscle invasive bladder cancer and recurrence after 2 prior courses of intravesical bacillus calmette-guérin. J. Urol. 190, 1200–1204. doi: 10.1016/j.juro.2013.04.031
Sobin, L., and Gospodarowicz, M. W. C. (eds.). (2009). TNM: Classification of Malignant Tumours. Oxford, UK: Wiley-Blackwell.
Stapf, M., Teichgräber, U., and Hilger, I. (2017). Methotrexate-coupled nanoparticles and magnetic nanochemothermia for the relapse-free treatment of T24 bladder tumors. Int. J. Nanomedicine 12, 2793–2811. doi: 10.2147/IJN.S120969
Steinberg, G., Bahnson, R., Brosman, S., Middleton, R., Wajsman, Z., and Wehle, M. (2000). Efficacy and safety of valrubicin for the treatment of Bacillus Calmette-Guerin refractory carcinoma in situ of the bladder. The Valrubicin Study Group. J. Urol. 163, 761–767. doi: 10.1016/S0022-5347(05)67799-3
Sudha, T., Bharali, D. J., Yalcin, M., Darwish, N. H., Coskun, M. D., Keating, K. A., et al. (2017). Targeted delivery of cisplatin to tumor xenografts via the nanoparticle component of nano-diamino-tetrac. Nanomedicine 12:nnm-2016-0315. doi: 10.2217/nnm-2016-0315
Sweeney, A. E. (2015). Nanomedicine concepts in the general medical curriculum: initiating a discussion. Int. J. Nanomed. 10, 7319–7331. doi: 10.2147/IJN.S96480
Sylvester, R. J. (2008). Editorial comment on: prognostic factors in patients with non-muscle-invasive bladder cancer treated with bacillus Calmette-Guérin: multivariate analysis of data from four randomized CUETO trials. Eur. Urol. 53, 1002. doi: 10.1016/j.eururo.2007.10.007
Sylvester, R. J., van der Meijdena, A. P., and Lamm, D. L. (2002). Intravesical bacillus Calmette-Guerin reduces the risk of progression in patients with superficial bladder cancer: a meta-analysis of the published results of randomized clinical trials. J. Urol. 168, 1964–1970. doi: 10.1016/S0022-5347(05)64273-5
Uenishi, Y., Kawabe, K., Nomura, T., Nakai, M., and Sunagawa, M. (2009). Morphological study on Mycobacterium bovis BCG Tokyo 172 cell wall skeleton (SMP-105). J. Microbiol. Methods 77, 139–144. doi: 10.1016/j.mimet.2009.01.019
Uenishi, Y., Okada, T., Okabe, S., and Sunagawa, M. (2007). Study on the cell wall skeleton derived from Mycobacterium bovis BCG Tokyo 172 (SMP-105): establishment of preparation and analytical methods. Chem. Pharm. Bull. (Tokyo) 55, 843–852. doi: 10.1248/cpb.55.843
van der Heijden, A. G., Kiemeney, L. A., Gofrit, O. N., Nativ, O., Sidi, A., Leib, Z., et al. (2004). Preliminary European results of local microwave hyperthermia and chemotherapy treatment in intermediate or high risk superficial transitional cell carcinoma of the bladder. Eur. Urol. 46, 65–71; discussion 71–72. doi: 10.1016/j.eururo.2004.01.019
Wang, X., Zhang, F., and Wu, X.-R. (2017). Inhibition of pyruvate kinase M2 markedly reduces chemoresistance of advanced bladder cancer to cisplatin. Sci. Rep. 7:45983. doi: 10.1038/srep45983
Wei, Y., Gao, L., Wang, L., Shi, L., Wei, E., Zhou, B., et al. (2017). Polydopamine and peptide decorated doxorubicin-loaded mesoporous silica nanoparticles as a targeted drug delivery system for bladder cancer therapy. Drug Deliv. 24, 681–691. doi: 10.1080/10717544.2017.1309475
Xin, Y., Huang, M., Guo, W. W., Huang, Q., Zhang, L. Z., and Jiang, G. (2017). Nano-based delivery of RNAi in cancer therapy. Mol. Cancer 16, 134. doi: 10.1186/s12943-017-0683-y
Yang, X., Su, L.-J., La Rosa, F. G., Smith, E. E., Schlaepfer, S. K., Cho, S. K., et al. (2015). The antineoplastic activity of photothermal ablative therapy with targeted gold nanorods in an orthotopic urinary bladder. Bladder Cancer 3, 201–210. doi: 10.3233/BLC-170096
Yao, S., Li, X., Liu, J., Sun, Y., Wang, Z., and Jiang, Y. (2017). Maximized nanodrug-loaded mesenchymal stem cells by a dual drug-loaded mode for the systemic treatment of metastatic lung cancer. Drug Deliv. 24, 1372–1383. doi: 10.1080/10717544.2017.1375580
Yurgel, V. C., Oliveira, C. P., Begnini, K. R., Schultze, E., Thurow, H. S., Leon, P. M. M., et al. (2014). Methotrexate diethyl ester-loaded lipid-core nanocapsules in aqueous solution increased antineoplastic effects in resistant breast cancer cell line. Int. J. Nanomed. 9, 1583–1591. doi: 10.2147/IJN.S56506
Zhang, D., Sun, P., Li, P., Xue, A., Zhang, X., Zhang, H., et al. (2013). A magnetic chitosan hydrogel for sustained and prolonged delivery of Bacillus Calmette-Guérin in the treatment of bladder cancer. Biomaterials 34, 10258–10266. doi: 10.1016/j.biomaterials.2013.09.027
Zhang, Q., Neoh, K. G., Xu, L., Lu, S., Kang, E. T., Mahendran, R., et al. (2014). Functionalized mesoporous silica nanoparticles with mucoadhesive and sustained drug release properties for potential bladder cancer therapy. Langmuir 30, 6151–6161. doi: 10.1021/la500746e
Keywords: bladder cancer, nanotechnology, BCG, Nano-BCG, monoclonal antibody, EGFR
Citation: Buss JH, Begnini KR, Bender CB, Pohlmann AR, Guterres SS, Collares T and Seixas FK (2018) Nano-BCG: A Promising Delivery System for Treatment of Human Bladder Cancer. Front. Pharmacol. 8:977. doi: 10.3389/fphar.2017.00977
Received: 21 October 2017; Accepted: 21 December 2017;
Published: 12 January 2018.
Edited by:
Susan Hua, University of Newcastle, AustraliaReviewed by:
Grannum R. Sant, Tufts University School of Medicine, United StatesKaisar Raza, Central University of Rajasthan, India
Copyright © 2018 Buss, Begnini, Bender, Pohlmann, Guterres, Collares and Seixas. This is an open-access article distributed under the terms of the Creative Commons Attribution License (CC BY). The use, distribution or reproduction in other forums is permitted, provided the original author(s) or licensor are credited and that the original publication in this journal is cited, in accordance with accepted academic practice. No use, distribution or reproduction is permitted which does not comply with these terms.
*Correspondence: Fabiana Kömmling Seixas, c2VpeGFzLmZrQGdtYWlsLmNvbQ==