- 1Department of Biomedical and Biotechnological Sciences, School of Medicine, University of Catania, Catania, Italy
- 2Sooft Italia S.p.A., Rome, Italy
- 3Bioos s.r.l., Catania, Italy
- 4Eye Clinic, Santa Marta Hospital, University of Catania, Catania, Italy
To investigate the effects of gabapentin, a structural analog of γ-amino butyric acid (GABA), on the inflammatory response of lipopolysaccharide (LPS)-stimulated rabbit corneal cells (SIRC) and on endotoxin-induced uveitis (EIU) in rabbits. We investigated the LPS-induced expression of several inflammatory mediators, such as TNF-α, IL-1β, cPLA2, COX-2, and PGE2 in the SIRC cells with or without gabapentin treatment. Gabapentin treatment significantly (p < 0.05) attenuated cytokines production, cPLA2 activation, COX-2 expression, and PGE2 levels in SIRC. EIU was induced by an intraocular injection of 0.1 μg of LPS in albino rabbit eye. After 7 and 24 h from LPS injection clinical signs of ocular inflammation were examined by slit lamp with or without topical treatment of 0.5% gabapentin. Tears, aqueous, cornea, conjunctiva, and iris-ciliary body were collected and inflammatory biomarkers assessed. Topical treatment with gabapentin significantly (p < 0.05) reduced clinical signs and biomarkers of inflammation compared with the LPS group both at 7 and 24 h. In conclusion, the results generated in the present study suggest that ophthalmic formulation based on gabapentin may be useful in the treatment of inflammatory conditions associated to ocular pain such as uveitis, and that clinical studies to evaluate this possibility may be warranted.
Introduction
Gabapentin, a structural analog of γ-amino butyric acid (GABA), targeting α2δ1 subunit of voltage-sensitive calcium channels, has therapeutic effect for neurological and psychiatric disorders such as epilepsy, anxiety, and neuropathic pain. This latter is also present in the eye and has been reported in patients affected by dry eye disease or diabetes or Sjögren syndrome (Rosenthal et al., 2009). Further, neuropathic ocular pain can be elicited by UV exposure or chemical insults. The effect of gabapentin on pain as recently linked to the anti-inflammatory action of the drug. It has been demonstrated (Lee et al., 2013) that gabapentin is able to reduce pro-inflammatory mediators (e.g., TNF-α, IL-1β, and IL-6) and up-regulates anti-inflammatory cytokine IL-10 in a rat model of neuropathic pain. More recently (Dias et al., 2014) it has been showed that gabapentin reverses inflammatory process in well-known acute mouse models of inflammation.
Inflammation is a non-specific response to injury that includes a variety of functional and molecular mediators, including recruitment and/or activation of inflammatory cells and release of inflammatory mediators such as cytokines (e.g., TNF-α), interleukins (e.g., IL-1β, IL-6), enzymes [e.g., cyclooxygenases (COXs)], and prostaglandins (PGs) (e.g., PGE2). These latter are synthesized by COXs, bifunctional enzymes which contain both cyclooxygenase and peroxidase activity and exist as distinct isoforms referred to as COX-1 and COX-2 (Smith et al., 2000) starting from arachidonic acid (AA), in turn hydrolyzed by phospholipases A2 (PLA2s). Moreover, AA plays a key role in inflammation and neurodegenerative disorders (Sun et al., 2004). Among the three major classes of PLA2s (secretory, calcium-independent and calcium-dependent) in the mammalians, the group IV calcium-dependent cytosolic PLA2α (cPLA2α) has received the most attention because of its expression in all mammalian cells and its active participation in cell metabolism (Sun et al., 2014). Following the Ca++binding to its C-2 domain, cPLA2 undergoes a number of post-translational modifications, such as phosphorylation on Ser-505, -727, and -515 and S-nitrosylation after the NO-interaction (Linkous and Yazlovitskaya, 2010). The aim of the study was to investigate the effects of gabapentin on ocular inflammatory models using lipopolysaccharide (LPS)-induced damage both in vitro and in vivo. In particular, we used rabbit corneal cells [Seruminstitute Rabbit Cornea (SIRC)] and a rabbit model of uveitis. Endotoxin-induced uveitis (EIU) represents a valuable experimental model of acute ocular inflammation characterized by release of several inflammatory biomarkers. We investigated the effect of gabapentin both in corneal cells challenging with LPS and in EIU assessing the levels of TNF-α, IL-6, IL-1β, and PGE2.
Materials and Methods
Materials
Gabapentin was purchased from Santa Cruz Biotechnology (Santa Cruz, CA, USA). LPS (L2880) from Escherichia coli O127:B8 were obtained from Sigma–Aldrich Chemical Co. (St Louis, MO, USA). Anti-rabbit IL-1β and anti-mouse TNF-α antibodies were purchased from Abcam Inc. (Cambridge, MA, USA). Anti-rabbit p-cPLA2, cPLA2, COX-2 antibodies were purchased from Cell Signaling Technology (CST).
Cell Cultures
Statens Seruminstitut rabbit corneal (SIRC) epithelial cells (ATCC CCL-60) were cultured in minimum essential medium with Earle’s salts, L-glutamine, and non-essential amino acids supplemented [Eagle’s Minimum Essential Medium (ATCC® 30-2003TM] with 10% activated fetal bovine serum (FBS, 10108-165, GIBCO), incubated at 37°C in a humidified atmosphere of 5% CO2. SIRC cells were seeded into 6-wells plates for 24 h before the experiment at a density of 1.2 × 105 cells/well in 2.0 ml of medium. Culture medium was exchanged every other day, and cultures were maintained until sub-confluence. For all experiments, cells were pre-incubated in serum-free medium (SFM) for 1 h with 10 μg/ml gabapentin (this concentration was chosen based on cell viability assay) and then, the inflammatory stimulus was induced with LPS (1 μg/ml) for different times. In addition, in order to examine the gabapentin effect on baseline cytokines level, SIRC cells were incubated with the drug alone without LPS.
Analysis of Cell Viability
The MTT assay was employed to assess rabbit corneal cell viability after treatment with gabapentin or LPS. Cells were seeded in 96-well plates at a density 2 × 104 cells per well and incubated overnight at 37°C before experiment. Subsequently, different concentrations of gabapentin (5, 10, 100, 1000 μg/ml) were added to each well except the well with control solution, for 24 and 48 h. In a second set of experiments was also assayed the toxicity of LPS (1–10–100 μg/ml) after 24 h. After incubation with the substances, 10 μl MTT [3-(4,5-dimethylthiazol-2-yl)2,5-diphenyltetrazolium bromide] reagent (5 mg/ml) was added to each well and the plates were incubated for 3 h at 37°C. The formazan crystals were extracted with 100 μl DMSO and plates were shaken for 10 min. The absorbance was measured at 570 nm with plate reader (Biotek Instruments, Elx-800). Cell viability was calculated as a percentage of the control.
Western Blot Analysis
Protein was extracted from SIRC cells as follows: cells were washed with PBS (pH 7.4), centrifuged at 1000 × g for 3 min, suspended in protein extraction buffer and incubated on ice for 30 min. After sonication, extract was centrifuged at 8,000 × g for 15 min. Protein samples (30 μg/lane) were subjected to SDS-PAGE and, after transfer to nitrocellulose membranes, were incubated as described previously (Lupo et al., 2007; Scuderi et al., 2008) with antibody against TNF-α, IL-1β, p-cPLA2, cPLA2, COX-2, β-actin overnight at 4°C followed by incubation with horseradish peroxidase conjugated secondary antibody, goat anti-rabbit IgG for IL-1β, p-cPLA2, cPLA2, COX-2 and goat anti-mouse IgG for TNF-α and β-actin. After washing, with TBS-T, protein expression was visualized with the Super Signal West Pico Chemiluminescence detection system (Thermo Scientific, Rockford, IL, USA). β-actin served as the loading control. Bands were analyzed using Image J software (Version1.43, Broken Symmetry Software, Bethesda, MD, USA).
PGE2 Measurement
PGE2 was measured in culture medium using a competitive binding ELISA, according to the manufacturer’s instructions (Abcam Inc., Cambridge, MA, USA). In particular, the SFM was harvested after incubation of SIRC with gabapentin and LPS (as described in experimental design) for 24 h. The amount of PGE2 was extrapolated from a standard curve (according the manufacturer’s instructions). All experiments were performed in triplicate.
Endotoxin-Induced Uveitis (EIU) Model
Male New Zealand albino rabbits weighing 2–2.5 kg (Harlan, Italy) were used. Animals were housed in single cage upon arrival in the facilities (in a light and temperature controlled room) with tap water and standard chow provided ad libitum. Animal procedures were approved by the Institutional Animal Care and Use Committee (IACUC) of the University of Catania, and conformed to the Association for Research in Vision and Ophthalmology (ARVO) resolution on the use of animals in research. Uveitis was induced by an intravitreal injection of LPS (0.1 μg/10 μl of E. coli 0111:B4; Sigma–Aldrich, Milan, Italy). Before LPS injection, rabbits were anesthetized by intravenous injection of 5 mg/kg Zoletil® (2.5 mg/kg tiletamine HCl and 2.5 mg/kg zolazepam HCl; Virbac, Milan, Italy) and one drop of local anaesthetic (Novesina®, Novartis, Origgio, Italy) was administered to the eye. An ophthalmic formulation of 0.5% gabapentin was prepared in an isotonic buffered solution (pH 7.0; 298 mOsm) and a multiple treatment (50 μl/instillation) was carried out (one instillation 30 min before LPS and four treatments after LPS). The dose used in the present study was chosen based on preliminary dose-ranging study (data not shown). Seven or 24 h after LPS injection tears were obtained with glass capillary tubes (Behring Diagnostics, Marburg, Germany). After that, the animals were killed (Tanax®; Intervet, Milan, Italy) and aqueous, conjunctiva, cornea, and iris-ciliary body collected. Care was taken to obtained tear samples avoiding stimulated tear production. Ten microliters of tears were collected from each eye and stored at -80°C until analysis.
Clinical Score and Aqueous Protein Levels
The clinical signs of ocular inflammation were examined by slit lamp (Sbisà, Firenze, Italy) and were graded on a scale of 0–4, according to the scoring system described by Ruiz-Moreno et al. (1992). Briefly the score was: 0 = no inflammatory reaction; 1 = discrete inflammatory reaction; 2 = moderate dilation of the iris and conjunctival vessels; 3 = intense iridal hyperemia, with flare in the anterior chamber; 4 = the same clinical signs as grade 3 plus the presence of fibrinoid exudation in the pupillary area, with intense flare in the anterior chamber. Clinical signs assessment was performed 15 m before the 7th and 24th h from intravitreal injection of LPS. Aqueous samples were collected from both eyes with a 30-gauge needle and protein levels assessed by a bicinchoninic acid (BCA) protein assay kit (Beyotime Institute of Biotechnology, Jiangsu, China).
Biomarkers Assessment
Ocular tissues (cornea, conjunctiva. and iris-ciliary body) were cut and lysed in 500 μL of tissue extraction reagent containing protease inhibitors (Invitrogen) with ULTRA-TURRAX. The samples were sonicated on ice and centrifuged twice (15,000 × g at 4°C, 20 min). The supernatant was assayed for levels of pro-inflammatory cytokines with commercial ELISA kits for TNF-α, IL-6, IL-1β (Quantikine kit; life technologies); before ELISA assay, total protein content in the ocular tissues was measured using the BCA protein assay kit. The ratio of cytokine to total protein (pg/mg) was calculated. All estimations were performed in duplicate. The TNF-α level was evaluated in tears with commercial ELISA kits (Quantikine kit; life technologies) at 7 and 24 h after injection of LPS; for the analysis were used 5 μL of the sample and values were expressed as pg/mL. In the aqueous humor, the TNF-α level was evaluated at 7 h and 24 h after injection of LPS with commercial ELISA kits (Quantikine kit; MybioSource) using 50 μL of the sample and values were expressed as pg/mL. PGE2 levels in aqueous humor samples were measured using an ELISA kit (Quantikine kit, Abcam) at 7 and 24 h after injection of LPS. The values were expressed as pg/mL according to the instruction manual.
Statistical Analysis
Data are presented as mean ± SD. A statistical analysis was performed with GraphPad Prism (GraphPad Software, Inc., San Diego, CA, USA). One-way ANOVA, followed by Tukey’s test was applied for parametric data; Kruskal–Wallis test, followed by Mann–Whitney test was performed for non-parametric data. A P-value less than 0.05 was considered statistically significant.
Results
Cell Viability
MTT assays were carried out in order to assess the effect of gabapentin on SIRC viability (Figure 1). 5, 10, and 100 μg/ml gabapentin did not affect cell viability, either at 24 h or at 48 h. Gabapentin at 1 mg/ml concentration caused a decrease in viability by 30 and 46% at 24 and 48 h, respectively. The data were confirmed by Trypan blue staining (data not shown). Based on these results, 10 μg/ml has been chosen for all in vitro experiments.
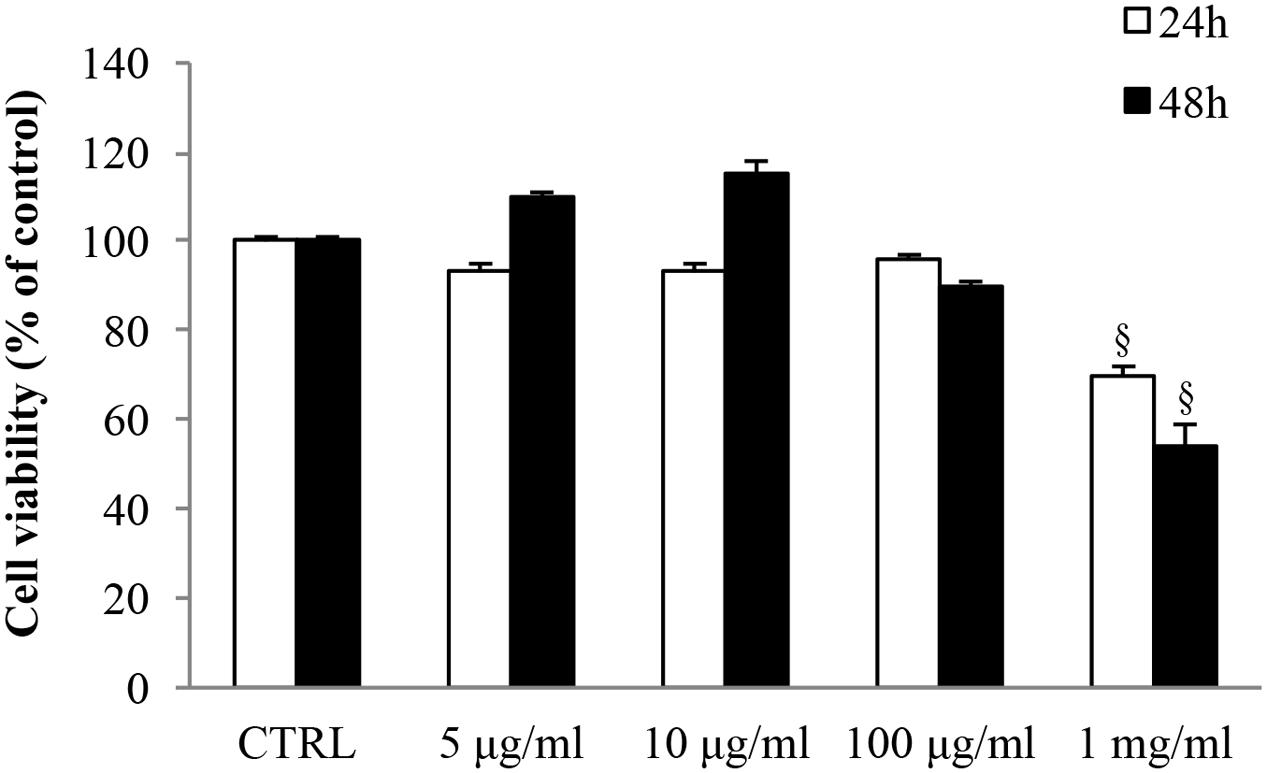
FIGURE 1. Effects of gabapentin on corneal cells viability. SIRC were seeded in 96-well plates at a density of 2 × 104 cells per well and incubated overnight at 37°C before experiment. Subsequently, different doses of gabapentin were added to each well for 24 and 48 h, as described in section “Materials and Methods.” Values represent mean ± SD, xp < 0.01 vs. CTRL. One-way ANOVA, followed by Tukey’s test.
Effects of Gabapentin on LPS-induced TNF-α and IL-1β Protein Expression
Seruminstitute Rabbit Cornea were treated with 1 μg/ml LPS to induce an inflammatory response (Figure 2, see the dose-response MTT viability curve into the box in the left of the figure). LPS increased TNF-α and IL-1β expression by 2.1- and 1.4-fold, respectively (Figure 2). This effect was significantly attenuated by gabapentin treatment (by 52 and 36% for TNF-α and IL-1β, respectively). These data demonstrate a positive effect of gabapentin to counteract inflammatory cytokines elicited by LPS. Gabapentin (10 μg/ml) alone did not elicited expression of TNF-α and IL-1β.
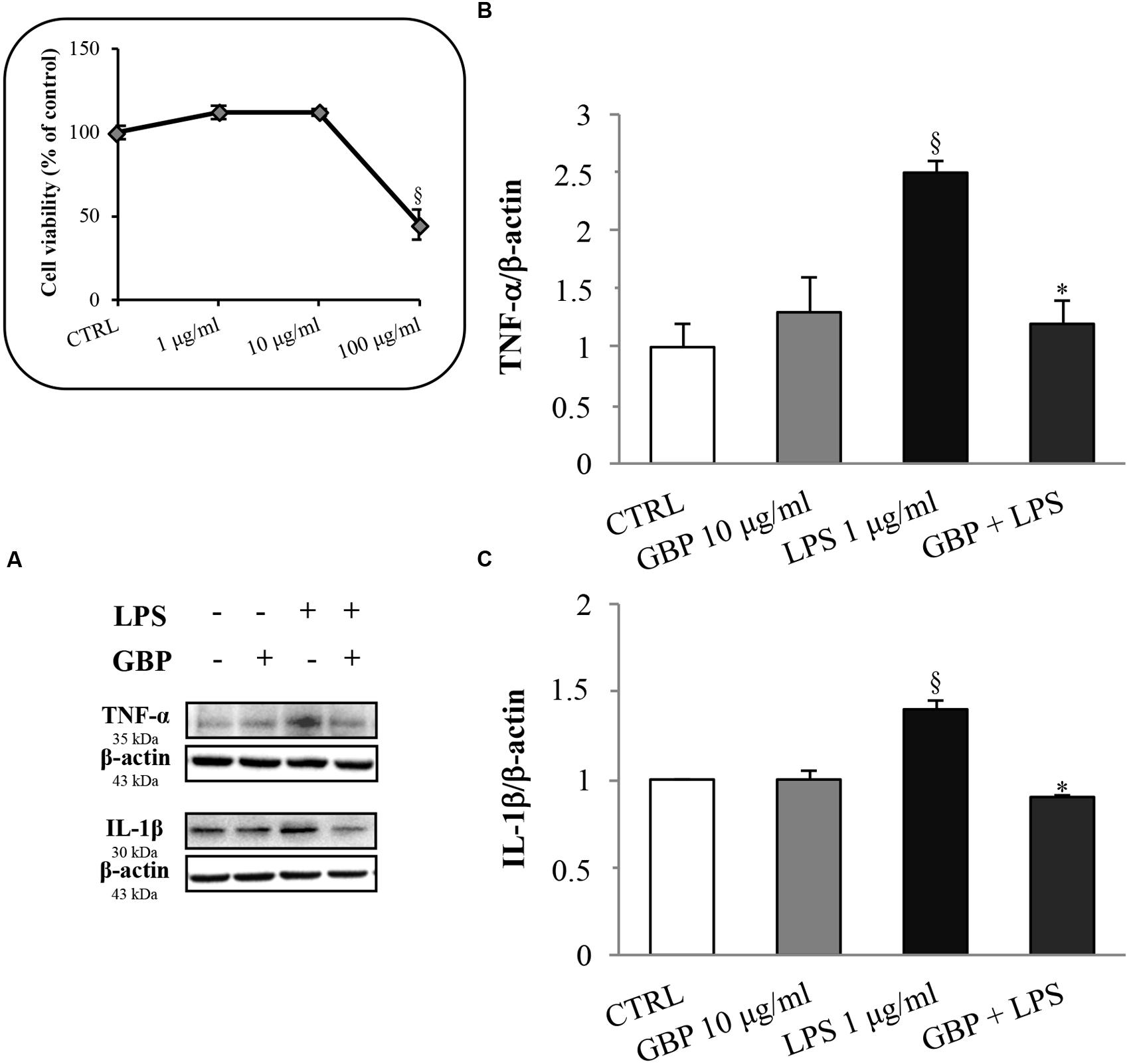
FIGURE 2. Effects of gabapentin on cytokines production by lipopolysaccharide (LPS) in corneal cells. SIRC were pre-incubated in serum-free medium (SFM) for 1 h with gabapentin (10 μg/ml), and the inflammatory stimulus was induced with LPS (1 μg/ml) for 24 h. Lysates were prepared for Western blot analysis as described in section “Materials and Methods.” BOX: Effect of LPS on SIRC viability after 24 h incubation; for the subsequent experiments was chosen the 1 μg/ml not toxic dose. (A) Representative immunoblot images of TNF-α, IL-1β, and β-actin following LPS treatment with or without gabapentin. (B,C) Densitometric analysis of TNF-α and IL-1β protein levels after adjusting for β-actin band intensity. Values represent mean ± SD, ∗p < 0.05 vs. LPS, xp < 0.01 vs. CTRL. One-way ANOVA, followed by Tukey’s test.
PLA2 Protein Expression/Activation
Western blot analyses of cPLA2 and phospho-cPLA2 in SIRC lysates from LPS with or without gabapentin in time-course experiments (5, 15, and 30 min, and 24 h) are reported in Figure 3. Immunoblots revealed the cPLA2 total protein expression was almost comparable in all samples within the same slot of incubation, regardless of LPS or gabapentin presence (panel A). LPS caused significant increases in the expression of the phosphorylated protein (except at 5 min), that is to say the activated form of cPLA2 (1.44, 2.1, and 1.8 p-cPLA2/cPLA2 ratio at 15 and 30 min and 24 h, respectively) and the increase in enzyme activity (Figure 3). Interestingly, by 15 min onward in subsequent incubation time points, gabapentin caused a significant (p < 0.05) reduction of the phosphorylation levels of the protein. These data support the idea that gabapentin is able to modulate the activation of an upstream enzyme in the intracellular cascade of events that lead, through the release of AA to the synthesis of key inflammatory mediators such as eicosanoids.
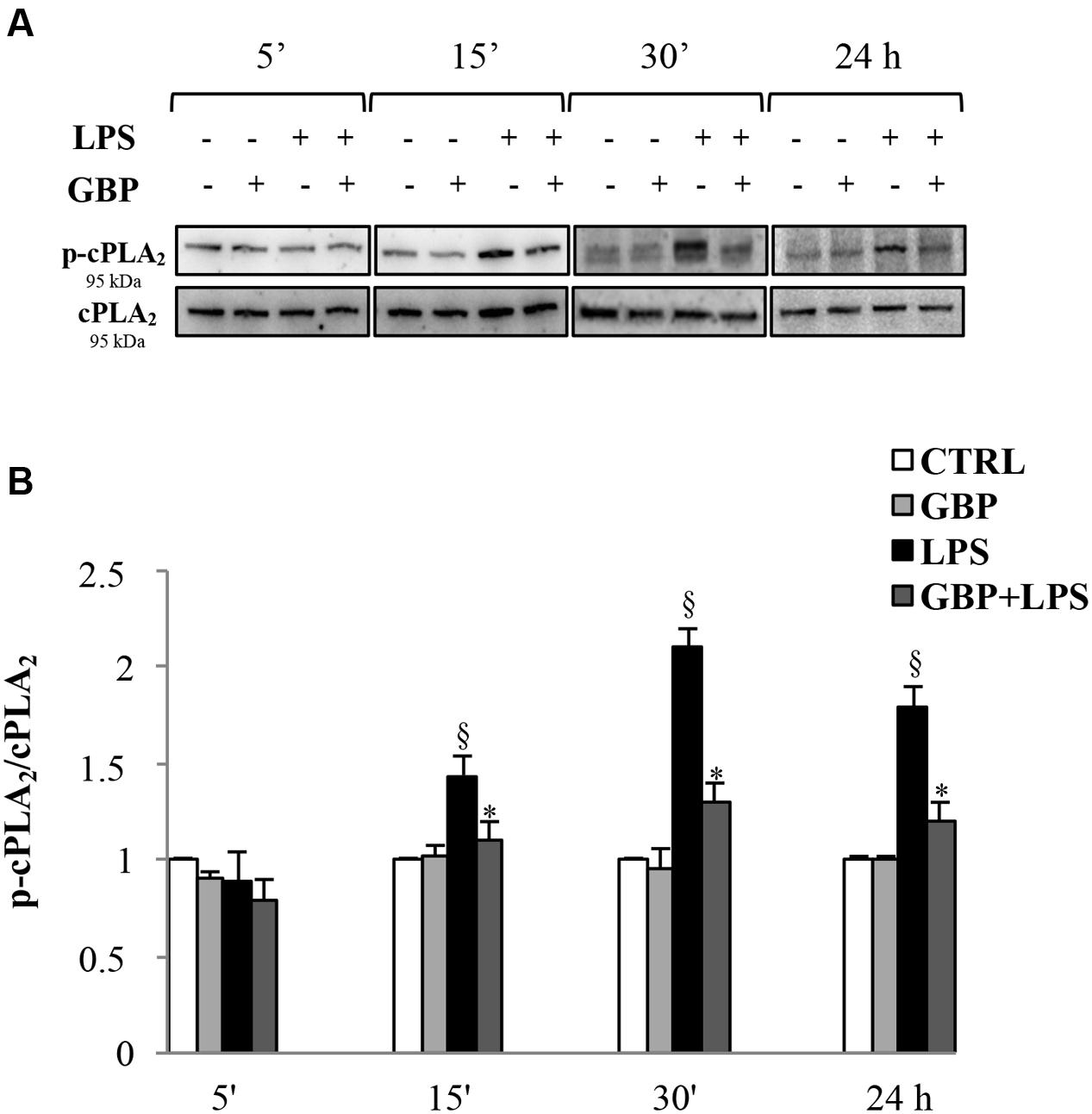
FIGURE 3. Effects of gabapentin on cPLA2 activation by LPS in corneal cells. SIRC were pre-incubated in SFM for 1 h with gabapentin (10 μg/ml) and the inflammatory stimulus was induced with LPS (1 μg/ml) for the different time points reported in figure. Lysated were prepared for Western blot analysis, as described in section “Materials and Methods.” (A) Representative immunoblot images of phospho-cPLA2 (p-cPLA2) and total-cPLA2 after LPS treatment with or without gabapentin. (B) Phospho-cPLA2/cPLA2 band intensity ratios of protein levels. Values represent mean ± SD, ∗p < 0.05 vs. LPS, xp < 0.01 vs. CTRL. One-way ANOVA, followed by Tukey’s test.
COX-2 and PGE2 in Corneal Cells
Cyclooxygenases-2 expression in SIRC (Figures 4A,B) after 30 min, 6 and 18 h from LPS was evaluated (no changes in COX-1 protein expression were observed; data not shown). Conversely, LPS-stimulated SIRC significantly expressed inducible COX-2 total protein by 2.8-, 3.0-, and 3.9-fold at 30 min, 6 and 18 h, respectively, compared to control (no LPS) (Figure 4B). Gabapentin treatment (10 μg/ml) significantly (p < 0.05) reduced the LPS-conditioned SIRC COX-2 overexpression by 43% (30 min), 40% (6 h), and 62% (18 h). These data confirmed the positive effect of gabapentin to contrast the production of inflammatory enzymes such as COX-2. At this regard, we evaluated PGE2 production, measured in supernatants of all culture models incubated for 24 h (Figure 4C). Gabapentin alone in SIRC (without LPS) had no effect on PGE2 production. As expected, LPS-induced a significant increase in PGE2 production by 3.0-fold compared to control. Gabapentin treatment in LPS-stimulated SIRC caused a significant (p < 0.05) reduction in PGE2 levels.
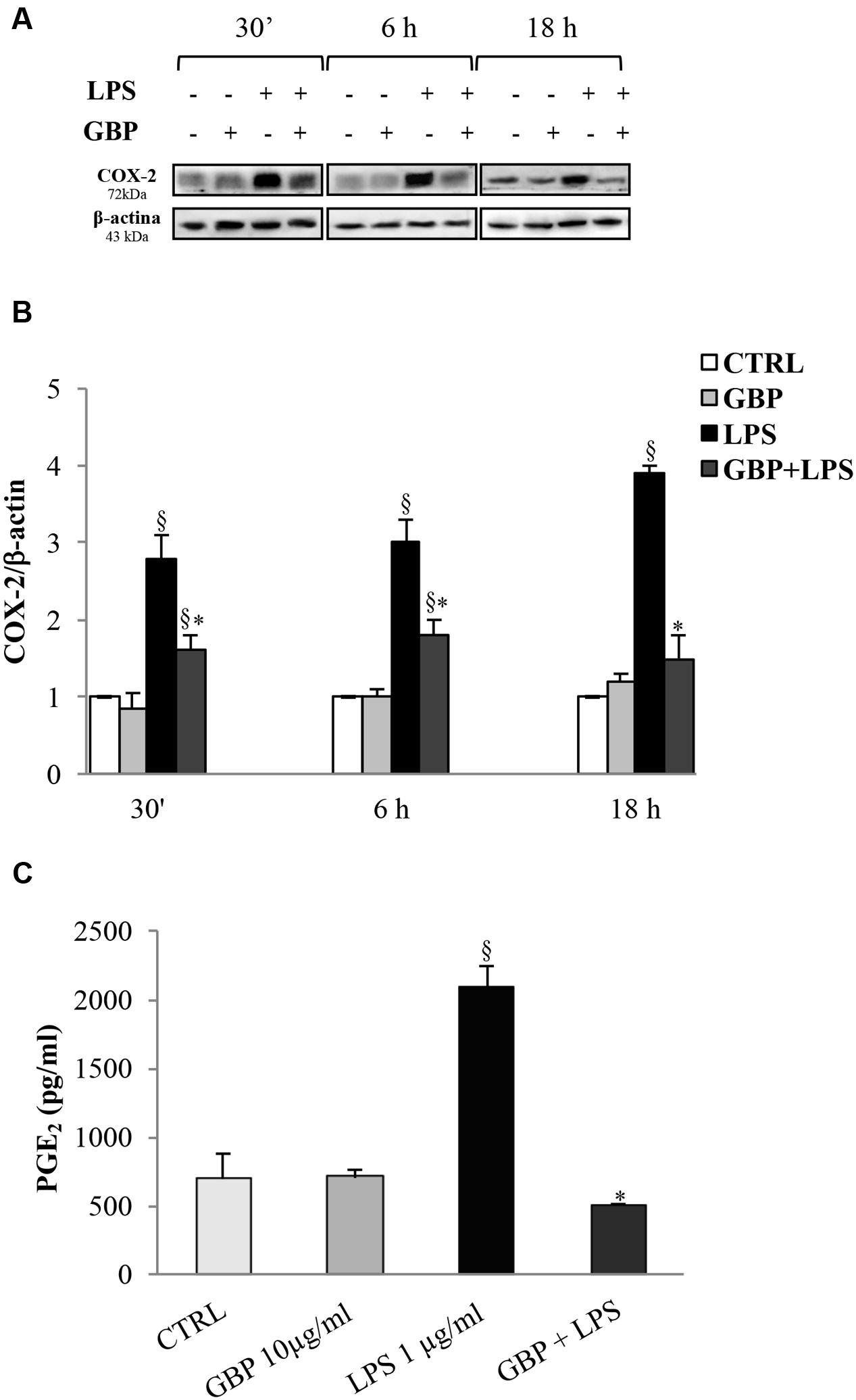
FIGURE 4. Effects of gabapentin on COX-2 and PGE2 in corneal cells. SIRC were pre-incubated in SFM for 1 h with gabapentin (10 μg/ml) and the inflammatory stimulus was induced with LPS (1 μg/ml) for the time points reported in figure. Lysated were prepared for Western blot analysis, as described in section “Materials and Methods.” In a separate set of experiments, the supernatants were collected to assess PGE2 after 24 h, as described in section “Materials and Methods.” Representative immunoblot images of COX-2 after LPS exposure with or without gabapentin (A). Densitometric analysis of COX-2 protein level after adjusting for β-actin band intensity (B). ELISA analysis of PGE2 levels after 24 h of LPS exposure with or without gabapentin (C). Values represent mean ± SD, ∗p < 0.05 vs. LPS, xp < 0.01 vs. CTRL. One-way ANOVA, followed by Tukey’s test.
Clinical Score and Aqueous Protein Levels
Ocular inflammation elicited by LPS caused a significant (p < 0.05) damage of eye’s tissues after 7 h still evident at 24 h (Figure 5A). Topical treatment with gabapentin significantly reduced clinical signs of inflammation compared with the LPS group both at 7 and 24 h (Figure 5A). Furthermore, LPS injection induced a significant (p < 0.01) increase of protein levels in the aqueous humor particularly after 7 h (Figure 5B) which is significantly (p < 0.05) reverted by gabapentin treatment.
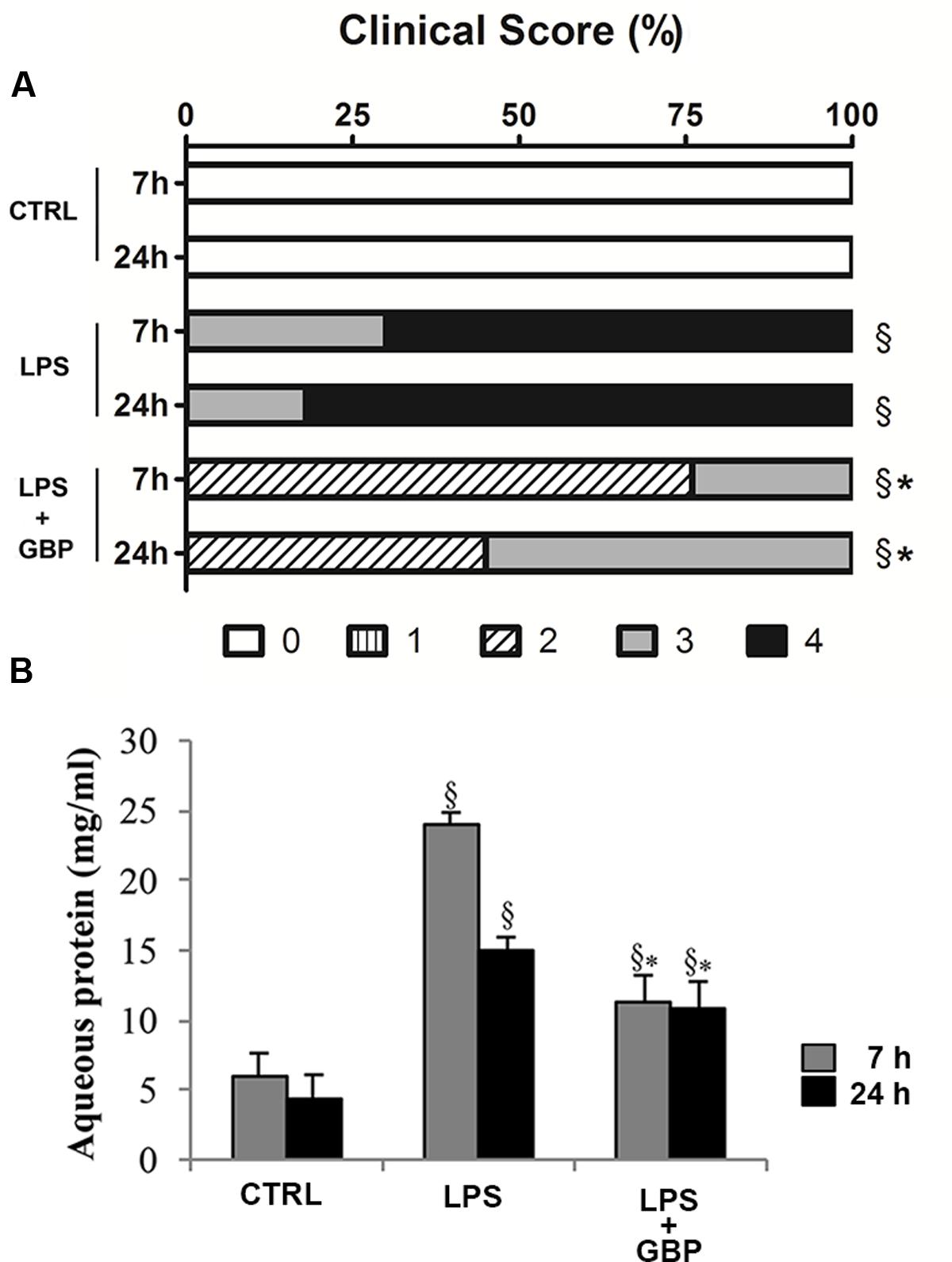
FIGURE 5. Effects of topical gabapentin on clinical score and aqueous protein levels in experimental uveitis. Clinical scores were evaluated at 7 and 24 h after LPS injection (A), see section “Materials and Methods” for more details. Aqueous protein levels from all groups measured at 7 and 24 h after LPS injection (B). Values represent mean ± SD, ∗p < 0.05 vs. LPS, xp < 0.01 vs. CTRL. Kruskal–Wallis test followed by Mann–Whitney test (A); one-way ANOVA followed by Tukey’s test (B).
Inflammatory Biomarkers
At 7 and 24 h after LPS injection we observed a significant (p < 0.01 vs. control) increase of TNF-α levels in tears, cornea, aqueous, and iris-ciliary body that was reversed by topical treatment of gabapentin (Figure 6). Furthermore, the effects of topical gabapentin on IL-6 and IL-1β in ocular tissues were assessed in rabbit with EIU (Figure 7). Previous studies (Mo et al., 1998; Brito et al., 2006) reported that the peak of aqueous IL-1β levels in rabbit with EIU was around 18 h after endotoxin injection, and IL-6 did not change at 24 h. In accordance with these reports we observed a significant (p < 0.01) peak of IL-6 and IL-1β in the aqueous in rabbit with EIU at 7 and 24 h, respectively. Topical treatment with gabapentin significantly (p < 0.05) attenuated the release of IL-6 and IL-1β in the aqueous of rabbit with EIU (Figure 7). Finally, gabapentin was able to significantly reduce the PGE2 levels in the aqueous elicited by endotoxin injection (Figure 8).
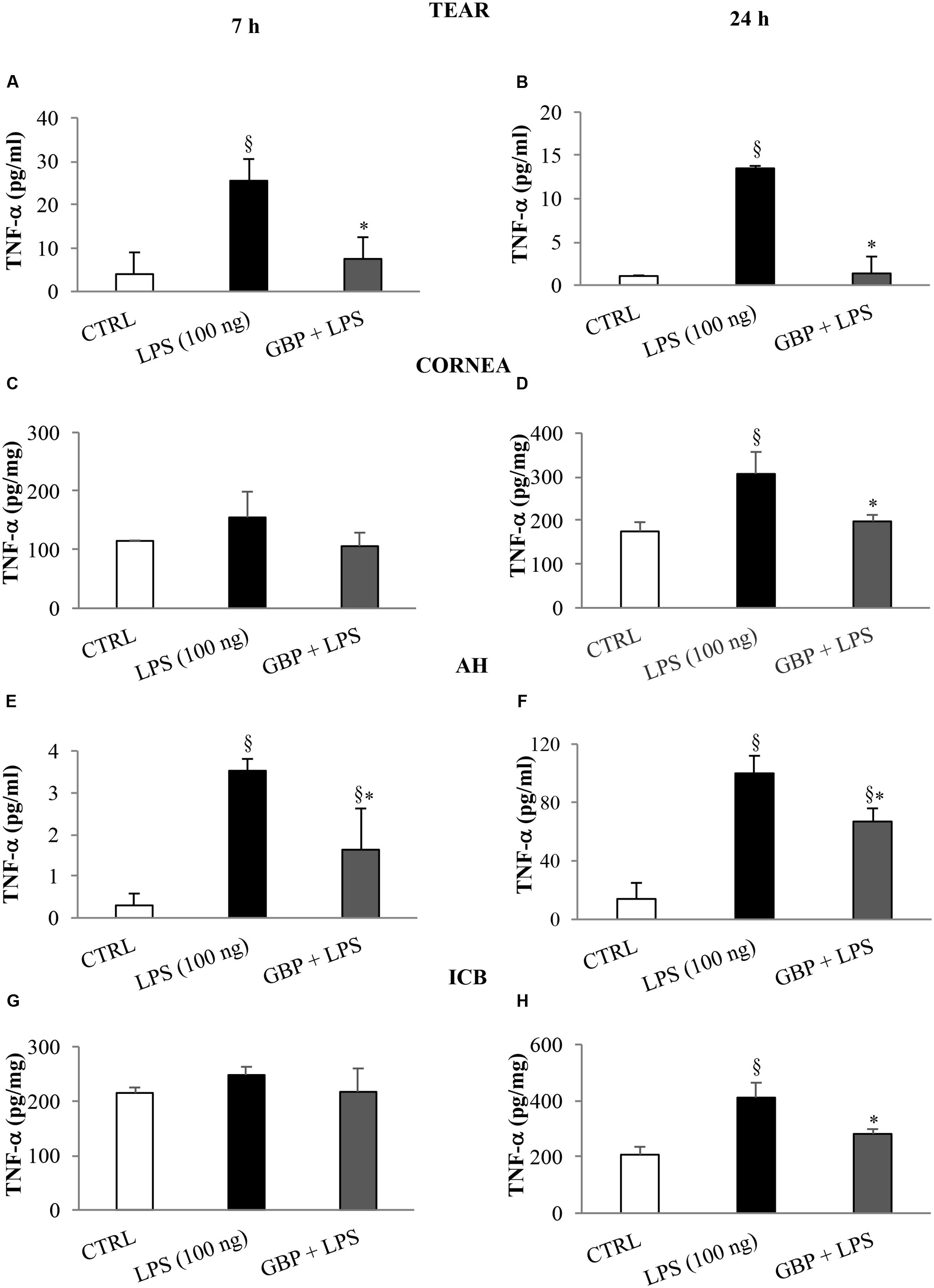
FIGURE 6. Effects of topical gabapentin on TNF-α levels in experimental uveitis. TNF-α levels in tears (A,B), cornea (C,D), aqueous humor (AH) (E,F), and iris-ciliary body (ICB) (G,H) at 7 and 24 h after LPS injection. Values represent mean ± SD, ∗p < 0.05 vs. LPS, xp < 0.01 vs. CTRL. One-way ANOVA, followed by Tukey’s test.
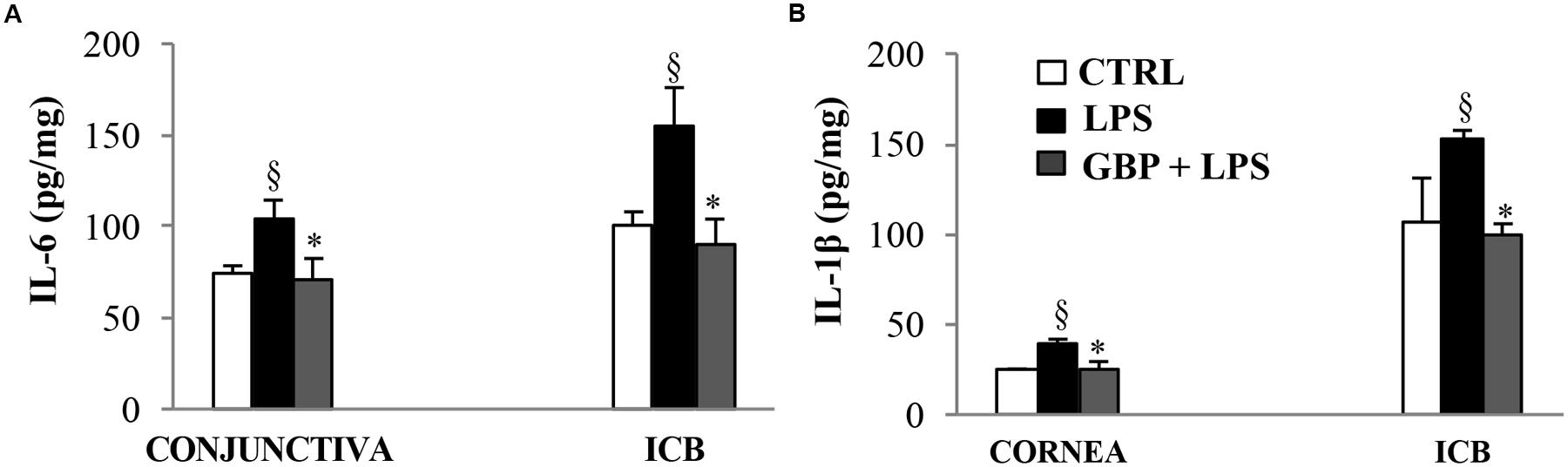
FIGURE 7. Effects of topical gabapentin on IL-6 and IL-1β levels in experimental uveitis. IL-6 (A) and IL-1β (B) levels in cornea, conjunctiva, and ICB (G, H) at 7 and 24 h, respectively, after LPS injection. Values represent mean ± SD, ∗p < 0.05 vs. LPS, xp < 0.01 vs. CTRL. One-way ANOVA, followed by Tukey’s test.
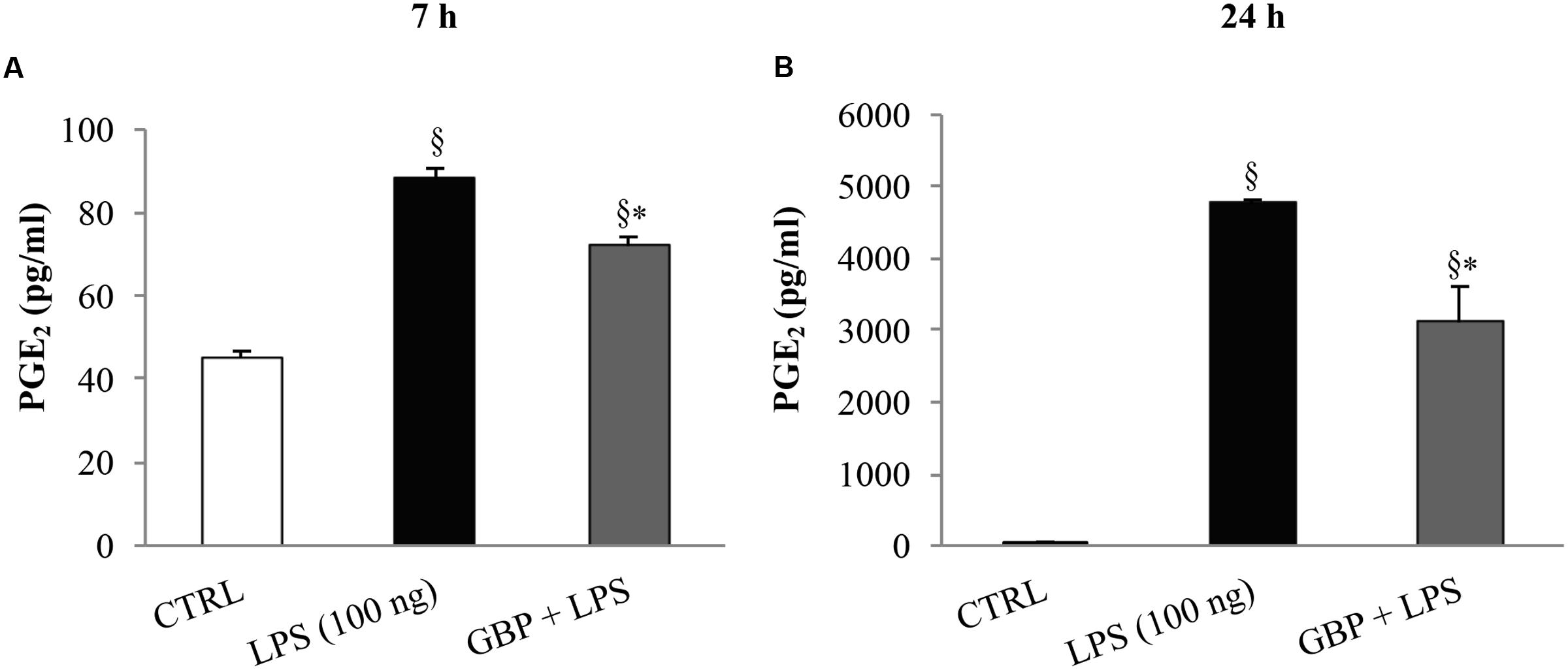
FIGURE 8. Effect of topical gabapentin on aqueous PGE2 levels in experimental uveitis. PGE2 levels in aqueous humor at 7 h (A) and 24 h (B) after LPS injection. Values represent mean ± SD, ∗p < 0.05 vs. LPS, xp < 0.01 vs. CTRL. One-way ANOVA, followed by Tukey’s test.
Discussion
In the present study we demonstrated that gabapentin inhibited ocular inflammation both in vitro and in vivo paradigms. In particular, gabapentin was able to counteract the inflammatory process elicited by LPS in rabbit corneal cells and rabbit’s eye.
Inflammation usually has beneficial effects on an acute basis, but can have unwanted effects if abiding chronically. Uveitis is the fourth cause of blindness in developed countries, and it represents a typical chronic ocular inflammation with a difficult management in terms of pharmacological therapy. Gabapentin is structurally related to the neurotransmitter gamma aminobutyric acid (GABA) but does not bind to the GABA receptors. Gabapentin is a drug with approved indications for epilepsy, seizures and neuropathic pain. Its mechanism of action is through binding to calcium channels and modulating the influx of calcium and thereby bestowing antiepileptic and analgesic effects. It is not clear if the anti-inflammatory effect of gabapentin is related to calcium modulation rather than other mechanisms such as stimulation of endogenous anti-oxidants like GSH, inhibition of NF-kB, block of NMDA receptor or activation of adenosine A1 receptor (Abdel-Salam and Sleem, 2009; Kim et al., 2009; Yang et al., 2012; Dias et al., 2014; Wang et al., 2014; Martins et al., 2015; Xu et al., 2017). Phosphorylation and calcium concentrations are the effectors modulating the activity of cPLA2 and recent studies have highlighted the role of PLAs2 as potential therapeutic target in inflammation and in other serious disorders, and the increase of PLA2 has been linked with the severity of the disease (Yarla et al., 2015). AA is released from phospholipids by the action of different isoforms of phospholipase A2s (PLA2s) and converted to PGs or leukotrienes (LTs) by the action of COXs and 5-lipoxygenase, respectively. These downstream products play key roles in governing cell migration and proliferation, as well as inflammation (Dennis et al., 2011; Anfuso et al., 2014; Lupo et al., 2014). Actually, four main groups of phospholipases are known, which include the secretory, the calcium-independent, the cytosolic and the lipoprotein-associatedphospholipases A2.
Release of AA has been shown to accumulate in response to ischemia in the eye (Birkle and Bazan, 1989; Remé et al., 1994). There is only a little knowledge about PLA2 expression in other parts of the eye, in addition to the retina (Castagnet and Giusto, 1993; Van Themsche et al., 2001; Kolko et al., 2007). cPLA has been identified in the human cornea (Landreville et al., 2004), while, in the conjunctiva, cPLA2 is mainly localized in the surface of the epithelium, probably participating in the protection against risks caused by mechanical wear and tear stress (Helin et al., 2008). In an in vitro retinoblastoma human triple culture model of angiogenesis, tumor cells induced in human retinal endothelium the increase in cPLA2proteinexpression (Lupo et al., 2014). As showed in the in vitro studies, LPS caused an increase expression of the cPLA2 active form and gabapentin significantly lowered the phosphorylation levels of the protein, supporting the idea that gabapentin was able to modulate the intracellular cascade of events that lead to the release of AA for the synthesis of key inflammatory eicosanoids mediators. Two main isoforms of cyclooxygenase exist, COX-1 and COX-2 (Simmons et al., 2004), being COX-3 still largely unknown (Davies et al., 2004). COX-1 is constitutively expressed in many tissues and plays a key role in the management of homeostasis. On the contrary, COX-2 is an inducible isoform and is activated in response to extracellular stimuli such as growth factors and pro-inflammatory cytokines (Simmons et al., 2004). It has been shown that cPLA2 and COX-2 co-localized in the perinuclear area (Pardue et al., 2003). Moreover, IL-1β and TNFα activate COX-2 and stimulate signaling pathways leading to cPLA2 phosphorylation and AA. At this regard, TNFα-stimulated phosphorylation of cPLA2involves the c-Jun and p38 MAP kinase pathways (Hernández et al., 1999). In an endotoxin-induced uveitis rodent model and in human ARPE-19 cells LPS-activated, an increasing of COX-2, IL-6, and IL-8 gene expression were found (Girol et al., 2013). Moreover, mice underwent to adverse environmental conditions showed high COX-2 and PGE synthase mRNA levels on the ocular surface (Shim et al., 2012). In accordance with this data, LPS-stimulated SIRC significantly expressed inducible COX-2 total protein and gabapentin treatment dramatically reduced the COX-2 synthesis induction. Moreover, gabapentin treatment in LPS-stimulated SIRC caused a significant reduction in PGE2 levels.
Several findings have been highlighted the role of gabapentin in reducing inflammation in several experimental paradigms (Dias et al., 2014). Recently, Dias et al. (2014) demonstrated that gabapentin decreases the paw edema induced by carrageenan, dextran, and 48/80 in mice. Furthermore, these authors showed that gabapentin inhibited levels of pro-inflammatory cytokines (TNF-α and IL-1β) and neutrophil infiltration. We showed, for the first time, that gabapentin attenuates ocular inflammation elicited by LPS both rabbit corneal cells culture and in rabbit eye. The effects of gabapentin may be due, at least in part, to the inhibition of inflammatory cytokines such as TNF-α, IL-6, and IL-1β. We also demonstrated that tear TNF-α was inhibited by gabapentin in EIU model. The data on TNF-α, IL-6, and IL-1β are in accordance with the findings generated by Lee et al. (2013) in different inflammatory paradigms. The anti-inflammatory effects of gabapentin may be dependent on a combination of pharmacologic properties of this molecule that could be due to the block of some specific cytokines, particularly TNF-α. Similarly to what we observed in SIRC we showed a significant (p < 0.05) inhibition of PGE2 levels in aqueous humor of rabbit with EIU. It is noteworthy that TNF-α is key actor in ocular inflammation, TNF-α triggers activation of cPLA2 and then enhancing the synthesis of PGE2 (Van Putten et al., 2001). We demonstrated that ocular inflammation elicited by LPS was significantly attenuated by gabapentin treatment both in SIRC and rabbit eye. TNF-α, a well-known hallmark of ocular inflammation, was significantly reduced by gabapentin treatment in vitro and in vivo after endotoxin challenging. In particular, gabapentin reduced TNF-α levels in tears, aqueous, cornea, and iris-ciliary body of rabbit with EIU.
Conclusion
The results generated in the present study suggest that ophthalmic formulation based on gabapentin may be useful in the treatment of inflammatory conditions associated to ocular pain such as uveitis, and that clinical studies to evaluate this possibility may be warranted.
Author Contributions
Authors make substantial contributions to idea and design: CA, MO, AF, GL, DR, SP, FD, and CB. Authors make contribution to acquisition of data: CA, MO, AF, GL, and CB. Authors make contribution to statistical analysis and interpretation of data: CA, MO, GL, CB, and CG. Authors participate in drafting the article and revising it critically: CA, GL, DR, FD, and CB.
Conflict of Interest Statement
Co-authors DR and SP are employees of pharmaceutical companies Sooft and Bioos, respectively.
The other authors declare that the research was conducted in the absence of any commercial or financial relationships that could be construed as a potential conflict of interest.
Acknowledgment
This work was supported by a Ministry of Education, Universities and Research (MIUR) grant PON01-00110.
References
Abdel-Salam, O. M., and Sleem, A. A. (2009). Study of the analgesic, anti-inflammatory, and gastric effects of gabapentin. Drug Discov. Ther. 3, 18–26.
Anfuso, C. D., Motta, C., Giurdanella, G., Arena, V., Alberghina, M., and Lupo, G. (2014). Endothelial PKCα-MAPK/ERK-phospholipase A2 pathway activation as a response of glioma in a triple culture model. A new role for pericytes? Biochimie 99, 77–87. doi: 10.1016/j.biochi.2013.11.013
Birkle, D. L., and Bazan, N. G. (1989). Light exposure stimulates arachidonic acid metabolism in intact rat retina and isolated rod outer segments. Neurochem. Res. 14, 185–190. doi: 10.1007/BF00969637
Brito, B. E., Marcano, J. C., Salazar, E., Cano, M., Baute, L., Bernal, G., et al. (2006). Age as a determinant factor for endotoxin induced uveitis. Ocul. Immunol. Inflamm. 14, 117–124. doi: 10.1080/09273940500328503
Castagnet, P. I., and Giusto, N. M. (1993). Properties of phospholipase A2 activity from bovine retinal rod outer segments. Exp. Eye Res. 56, 709–719. doi: 10.1006/exer.1993.1088
Davies, N. M., Good, R. L., Roupe, K. A., and Yáñez, J. A. (2004). Cyclooxygenase-3: axiom, dogma, anomaly, enigma or splice error?-Not as easy as 1,2, 3. J. Pharm. Pharm. Sci. 7, 217–226.
Dennis, E. A., Cao, J., Hsu, Y. H., Magrioti, V., and Kokotos, G. (2011). Phospholipase A2 enzymes: physical structure, biological function, disease implication, chemical inhibition, and therapeutic intervention. Chem Rev. 111, 6130–6185. doi: 10.1021/cr200085w
Dias, J. M., de Brito, T. V., de Aguiar Magalhães, D., da Silva, Santos, P. W., Batista, J. A., et al. (2014). Gabapentin, a synthetic analogue of gamma aminobutyric acid, reverses systemic acute inflammation and oxidative stress in mice. Inflammation 37, 1826–1836. doi: 10.1007/s10753-014-9913-2
Girol, A. P., Mimura, K. K., Drewes, C. C., Bolonheis, S. M., Solito, E., Farsky, S. H., et al. (2013). Anti-inflammatory mechanisms of the annexin A1 protein and its mimetic peptide Ac2-26 in models of ocular inflammation in vivo and in vitro. J. Immunol. 190, 5689–5701. doi: 10.4049/jimmunol.1202030
Helin, M., Rönkkö, S., Puustjärvi, T., Teräsvirta, M., and Uusitalo, H. (2008). Phospholipases A2 in normal human conjunctiva and from patients with primary open-angle glaucoma and exfoliation glaucoma. Graefes Arch. Clin. Exp. Ophthalmol. 246, 739–746. doi: 10.1007/s00417-007-0757-4
Hernández, M., Bayón, Y., Sánchez Crespo, M., and Nieto, M. L. (1999). Signaling mechanisms involved in the activation of arachidonic acid metabolism in human astrocytoma cells by tumor necrosis factor-alpha: phosphorylation of cytosolic phospholipase A2 and transactivation of cyclooxygenase-2. J. Neurochem. 73, 1641–1649. doi: 10.1046/j.1471-4159.1999.0731641.x
Kim, Y. S., Chang, H. K., Lee, J. W., Sung, Y. H., Kim, S. E., Shin, M. S., et al. (2009). Protective effect of gabapentin on N-methyl-D-aspartete-induced excitotoxicity in rat hippocampal CA1 neurons. J. Pharmacol. Sci. 109, 144–147. doi: 10.1254/jphs.08067SC
Kolko, M., Wang, J., Zhan, C., Poulsen, K. A., Prause, J. U., Nissen, M. H., et al. (2007). Identification of intracellular phospholipases A2 in the human eye: involvement in phagocytosis of photoreceptor outer segments. Invest. Ophthalmol. Vis. Sci. 48, 1401–1409. doi: 10.1167/iovs.06-0865
Landreville, S., Coulombe, S., Carrier, P., Gelb, M. H., Guérin, S. L., and Salesse, C. (2004). Expression of phospholipases A2 and C in human corneal epithelial cells. Invest. Ophthalmol. Vis. Sci. 45, 3997–4003. doi: 10.1167/iovs.04-0084
Lee, B. S., Jun, I. G., Kim, S. H., and Park, J. Y. (2013). Intrathecal gabapentin increases interleukin-10 expression and inhibits pro-inflammatory cytokine in a rat model of neuropathic pain. J. Korean Med. Sci. 28, 308–314. doi: 10.3346/jkms.2013.28.2.308
Linkous, A., and Yazlovitskaya, E. (2010). Cytosolic phospholipase A2 as a mediator of disease pathogenesis. Cell Microbiol. 12, 1369–1377. doi: 10.1111/j.1462-5822.2010.01505.x
Lupo, G., Anfuso, C. D., Ragusa, N., Tirolo, C., Marchetti, B., Gili, E., et al. (2007). Activation of cytosolic phospholipase A2 and 15-lipoxygenase by oxidized low-density lipoproteins in cultured human lung fibroblasts. Biochim. Biophys. Acta 177, 522–532. doi: 10.1016/j.bbalip.2007.01.014
Lupo, G., Motta, C., Salmeri, M., Spina-Purrello, V., Alberghina, M., and Anfuso, C. D. (2014). An in vitro retinoblastoma human triple culture model of angiogenesis: a modulatory effect of TGF-β. Cancer Lett. 354, 181–188. doi: 10.1016/j.canlet.2014.08.004
Martins, D. F., Prado, M. R., Daruge-Neto, E., Batisti, A. P., Emer, A. A., Mazzardo-Martins, L., et al. (2015). Caffeine prevents antihyperalgesic effect of gabapentin in an animal model of CRPS-I: evidence for the involvment of spinal adenosine A1 receptor. J. Peripher. Nerv. Syst. 20, 403–409. doi: 10.1111/jns.12149
Mo, J. S., Matsukawa, A., Ohkawara, S., and Yoshinaga, M. (1998). Involvment of TNFα, IL-1β and IL-1 receptor antagonist in LPS-induced rabbit uveitis. Exp. Eye Res. 66, 547–557.
Pardue, S., Rapoport, S. I., and Bosetti, F. (2003). Co-localization of cytosolic phospholipase A2 and cyclooxygenase-2 in Rhesus monkey cerebellum. Brain Res. Mol. Brain Res. 116, 106–114. doi: 10.1016/S0169-328X(03)00262-6
Remé, C. E., Malnoë, A., Jung, H. H., Wei, Q., and Munz, K. (1994). Effect of dietary fish oil on acute light-induced photoreceptor damage in the rat retina. Invest. Ophthalmol. Vis. Sci. 35, 78–90.
Rosenthal, P., Baran, I., and Jacobs, D. S. (2009). Corneal pain without stain: Is it real? Ocul. Surf. 7, 28–40. doi: 10.1016/s1542-0124(12)70290-2
Ruiz-Moreno, J. M., Thillaye, B., and de Kozak, Y. (1992). Retino-choroidal changes in endotoxin-induced uveitis in the rat. Ophthalmic Res. 24, 162–168. doi: 10.1159/000267163
Scuderi, M. R., Anfuso, C. D., Lupo, G., Motta, C., Romeo, L., Guerra, L., et al. (2008). Expression of Ca(2+)-indipendent and Ca(2+)-dependent phospholipases A(2) and cyclooxygenases in human melanocytes and malignant melanoma cell lines. Biochim. Biophys. Acta 1781, 635–642. doi: 10.1016/j.bbalip.2008.07.007
Shim, J., Park, C., Lee, H. S., Park, M. S., Lim, H. T., Chauhan, S., et al. (2012). Change in prostaglandin expression levels and synthesizing activities in dry eye disease. Ophthalmology 119, 2211–2219. doi: 10.1016/j.ophtha.2012.05.038
Simmons, D. L., Botting, R. M., and Hla, T. (2004). Cyclooxygenase isozymes: the biology of prostaglandin synthesis and inhibition. Pharmacol. Rev. 56, 387–437. doi: 10.1124/pr.56.3.3
Smith, W. L., DeWitt, D. L., and Garavito, R. M. (2000). Cyclooxygenases: structural, cellular, and molecular biology. Annu. Rev. Biochem. 69, 145–182. doi: 10.1146/annurev.biochem.69.1.145
Sun, G. Y., Chuang, D. Y., Zong, Y., Jiang, J., Lee, J. C., Gu, Z., et al. (2014). Role of cytosolic phospholipase A2 in oxidative and inflammatory signaling pathways in different cell types in the central nervous system. Mol. Neurobiol. 50, 6–14. doi: 10.1007/s12035-014-8662-4
Sun, G. Y., Xu, J., Jensen, M. D., and Simonyi, A. (2004). Phospholipase A2 in the central nervous system: implications for neurodegenerative diseases. J. Lipid Res. 45, 205–213. doi: 10.1194/jlr.R300016-JLR200
Van Putten, V., Refaat, Z., Dessev, C., Blaine, S., Wick, M., Butterfield, L., et al. (2001). Induction of cytosolic phospholipaseA2 by oncogenic Ras is mediated through the JNK and ERK pathways in rat epithelial cells. J. Biol. Chem. 276, 1226–1232. doi: 10.1074/jbc.M003581200
Van Themsche, C., Jacob, M., and Salesse, C. (2001). Human retinal pigment epithelium secretes a phospholipase A2 and contains two novel intracellular phospholipases A2. Biochem. Cell Biol. 79, 1–10. doi: 10.1139/o00-088
Wang, X., Zhang, M., Shen, J., and Yang, J. (2014). Gabapentin relieves neuropathic pain and inhibits brain and spinal neuroinflammatory responses in rats after L5 spinal nerve transaction. J. Perioper. Sci. 1:2.
Xu, X., Zheng, S., Xiong, Y., Wang, X., Qin, W., Zhang, H., et al. (2017). Adenosine effectively restores endotoxin-induced inhibition of human neutrophil chemotaxis via A1 receptor-p38 pathway. Inflamm. Res. 66, 353–364. doi: 10.1007/s00011-016-1021-3
Yang, J. L., Xu, B., Li, S. S., Zhang, W. S., Xu, H., Deng, X. M., et al. (2012). Gabapentin reduces CX3CL1 signaling and blocks spinal microglial activation in monoarthritic rats. Mol. Brain. 5:18. doi: 10.1186/1756-6606-5-18
Yarla, N. S., Satyakumar, K., Srinivasu, D., Kaladhar, D. S. V. G. K., Aliev, G., Dharmapuri, G., et al. (2015). Phospholipase A2: a potential therapeutic target in inflammation and cancer (In silico, In vitro, In vivo and clinical approach). J. Cancer Sci. Ther. 7, 249–252. doi: 10.4172/1948-5956.1000357
Keywords: gabapentin, corneal cells, endotoxin-induced uveitis, ocular inflammation, TNF-α
Citation: Anfuso CD, Olivieri M, Fidilio A, Lupo G, Rusciano D, Pezzino S, Gagliano C, Drago F and Bucolo C (2017) Gabapentin Attenuates Ocular Inflammation: In vitro and In vivo Studies. Front. Pharmacol. 8:173. doi: 10.3389/fphar.2017.00173
Received: 15 February 2017; Accepted: 15 March 2017;
Published: 04 April 2017.
Edited by:
Cesare Mancuso, Università Cattolica del Sacro Cuore, ItalyReviewed by:
Giuseppe Maurizio Campo, University of Messina, ItalyDaniele Tomassoni, University of Camerino, Italy
Copyright © 2017 Anfuso, Olivieri, Fidilio, Lupo, Rusciano, Pezzino, Gagliano, Drago and Bucolo. This is an open-access article distributed under the terms of the Creative Commons Attribution License (CC BY). The use, distribution or reproduction in other forums is permitted, provided the original author(s) or licensor are credited and that the original publication in this journal is cited, in accordance with accepted academic practice. No use, distribution or reproduction is permitted which does not comply with these terms.
*Correspondence: Claudio Bucolo, Y2xhdWRpby5idWNvbG9AdW5pY3QuaXQ=
† These authors have contributed equally to this work.