- 1Novel Bacteria and Drug Discovery Research Group, School of Pharmacy, Monash University Malaysia, Bandar Sunway, Malaysia
- 2Biomedical Research Laboratory, Jeffrey Cheah School of Medicine and Health Sciences, Monash University Malaysia, Bandar Sunway, Malaysia
- 3Division of Genetic and Molecular Biology, Faculty of Science, Institute of Biological Sciences, University of Malaya, Kuala Lumpur, Malaysia
- 4Center of Health Outcomes Research and Therapeutic Safety (Cohorts), School of Pharmaceutical Sciences, University of Phayao, Phayao, Thailand
- 5Pharmaceutical Outcomes Research Center, Faculty of Pharmaceutical Sciences, Naresuan University, Phitsanulok, Thailand
- 6Division of Physiology, School of Medical Sciences, University of Phayao, Phayao, Thailand
Epimedium (family Berberidaceae), commonly known as Horny Goat Weed or Yin Yang Huo, is commonly used as a tonic, aphrodisiac, anti-rheumatic and anti-cancer agent in traditional herbal formulations in Asian countries such as China, Japan, and Korea. The major bioactive compounds present within this plant include icariin, icaritin and icariside II. Although it is best known for its aphrodisiac properties, scientific and pharmacological studies suggest it possesses broad therapeutic capabilities, especially for enhancing reproductive function and osteoprotective, neuroprotective, cardioprotective, anti-inflammatory and immunoprotective effects. In recent years, there has been great interest in scientific investigation of the purported anti-cancer properties of icariin and its derivatives. Data from in vitro and in vivo studies suggests these compounds demonstrate anti-cancer activity against a wide range of cancer cells which occurs through various mechanisms such as apoptosis, cell cycle modulation, anti-angiogenesis, anti-metastasis and immunomodulation. Of note, they are efficient at targeting cancer stem cells and drug-resistant cancer cells. These are highly desirable properties to be emulated in the development of novel anti-cancer drugs in combatting the emergence of drug resistance and overcoming the limited efficacy of current standard treatment. This review aims to summarize the anti-cancer mechanisms of icariin and its derivatives with reference to the published literature. The currently utilized applications of icariin and its derivatives in cancer treatment are explored with reference to existing patents. Based on the data compiled, icariin and its derivatives are shown to be compounds with tremendous potential for the development of new anti-cancer drugs.
Introduction
Cancer is a growing health problem, representing one of the leading causes of mortality worldwide (Goh and Kadir, 2011). The current standard of care includes conventional medicine, surgery, radiotherapy, and chemotherapy (Hsiao and Liu, 2010) however the limitations of existing care are clear as even after completion of the standard treatment regimens, there are high rates of metastasis and relapse (Liang et al., 2010). In addition, there are significant problems associated with the standard treatments such as significant toxicity levels and the emergence of resistance toward anti-cancer drugs, making treatment of cancer even more challenging (Crespo-Ortiz and Wei, 2012). Consequently, the annual death rates due to cancer cannot be significantly lowered (Chan et al., 2015) unless new treatment strategies can be devised. Scientific exploration of medicinal plants and herbs is now of increasing interest in the hope that they will yield novel compounds with properties that can overcome the limitations of the drugs currently available (Lau et al., 2015; Supriady et al., 2015). Plant based compounds are also of interest due to their multitargeting properties, low cost, relative absence of toxicity and high availability (Gupta et al., 2011; Tan et al., 2015; Sayyad et al., in press).
Epimedium (family Berberidaceae), (Latin name Herba Epimedii) commonly known as Horny Goat Weed or Yin Yang Huo in Chinese, is a genus consisting of approximately 52 species of herbaceous flowering plants (Ma et al., 2011). In recent years, there has been a significant volume of research on the anti-cancer effect of the main active compounds from Herba Epimedii such as icariin, icaritin, and icariside II. Icariin and its derivatives, icaritin, and icariside II seem to be promising compounds for cancer treatment, with studies having shown that they exhibit anti-cancer activity against a wide range of cancer cell types such as osteosarcoma (Geng et al., 2014), prostate (Lee et al., 2009), lung (Zheng et al., 2014), gastric (Wang et al., 2010), and kidney cancer cells (Li et al., 2013b). These compounds exert their anticancer action via a multitude of cellular targets and through a variety of pathways including apoptosis inducing effect, cell-cycle modulation, anti-angiogenesis, anti-metastasis, and immunomodulation. Of particular interest, they effectively target cancer stem cells and drug resistant cancer cells. Research also suggests they are able to potentiate the current standard cancer treatments. The purpose of this review is to provide an up-to-date of the anti-cancer mechanisms of icariin and its derivatives; and to provide scientific evidence that there is a basis to support the efficacy of Herba Epimedii-based traditional herbal formulations for the treatment of cancer. Overall, the existing literature highlights the remarkable potential of these compounds for the development of novel drugs for cancer treatment.
Plant Description and Traditional Uses
Epimedium is a low-growing, deciduous plant with leathery leaves that spreads by underground stems. The flowers of Epimedium vary in color and they have eight sepals. A tough and long-lived perennial species, Epimedium is found mainly on cliffs in moist forests, near streams and wet lands at altitudes of between 200 and 3700 m (Ma et al., 2011). Epimedium is widely distributed from Japan to Algeria but it is mostly found in the East Asian and Mediterranean region (Arief et al., 2015).
Epimedium species have a long history of use in traditional medicine as they have been used in botanical supplements for more than 2000 years. The extracts of Epimedium plants are included in traditional herbal formulations for the treatment of infertility, rheumatism and cancer in Asian countries such as China, Japan, and Korea. In China, Epimedium is taken as a supplement for prevention of chronic diseases and to strengthen the body (Cassileth et al., 2010; Ma et al., 2011). Today, Herba Epimedii is still popular in the treatment of cancers; it has been commonly used as one of the main ingredients together with other herbs, for the preparation of traditional Chinese formulations to treat various cancers such as digestive system cancers, hepatocarcinomas, lung cancers, breast cancers and cervical cancers (Zhang, 1991; Qi and Qi, 2002; Wang, 2003; Teng, 2010). With reference to formal research, the extracts of Herba Epimedii were reported to display anti-cancer activity in cancer cell lines such as colon cancer cells, hepatoma and leukemia cells (Lin et al., 1999; Guon and Chung, 2014). Given the apparent efficacy of Herba Epimedii in treating cancers, phytochemical analyses have also been carried out to identify the bioactive components responsible for its pharmacological activities.
Icariin and Its Derivatives
More than 260 moieties can be detected in Herba Epimedii, including flavonoids, lignins, ionones, phenol glycosides, phenylethanoid glycosides, sesquiterpenes, and other types of compounds (Ma et al., 2011). Among the compounds identified, icariin is thought to be the principal bioactive compound in Epimedium extracts. Aside from icariin, derivatives such as icaritin, icariside I, icariside II, and desmethylicaritin can also be found in Herba Epimedii (Ma et al., 2011). Metabolic and pharmacokinetic studies have shown that these derivatives can also be obtained through the metabolism of icariin by intestinal flora, by converting icariin to icaritin, icariside I, icariside II, and desmethylicaritin (Liu et al., 2005; Xu et al., 2007). As shown in Figure 1, icariin (1) is a prenylated flavonol glycoside with rhamnosyl, glucosyl, and methoxy groups. Deglycosylation or demethylation of icariin will form different metabolites. For instance, icariside I will be formed when the rhamnose residue is removed whereas icariside II (3) will be formed when the glucose residue is removed. Deglycosylation of icariin will result in icaritin (2) and demethylation of icaritin will result in desmethylicaritin (Shen et al., 2009; Khan et al., 2015).
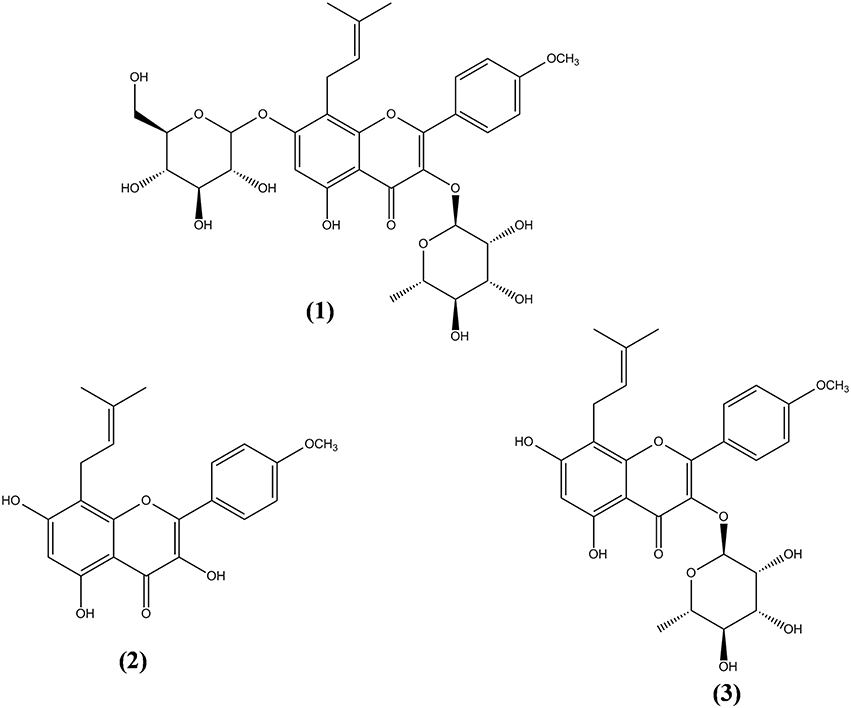
Figure 1. Chemical structures of icariin and its derivatives isolated from Herba Epimedii. Icariin (1), icaritin (2), and icariside II (3).
Scientific studies have provided evidence of the broad therapeutic capabilities of icariin, especially with reference to its effects on reproductive function (Chen et al., 2014), osteoprotective (Qin et al., 2008), neuroprotective (Nie et al., 2010), cardioprotective (Ding et al., 2007), anti-inflammatory (Xu et al., 2010), and immunoprotective effects (Li et al., 2011). Icariin's derivatives have also been shown to possess significant biological activities. For example, icaritin was shown to possess osteopromotive (Yao et al., 2012), neuroprotective (Wang et al., 2007) and cardioprotective effect (Lei et al., 2015). The number of studies on icariside I are limited but it was reported to alleviate sexual dysfunction (Lenoble et al., 2002). Icariside II, is reported to possess anti-hepatotoxic (Cho et al., 1995), anti-osteoporosis effects (Liu et al., 2014) and the ability to ameliorate diabetic neuropathy (Tian et al., 2015). Icariin, icaritin and icariside II, have also been reported to possess anticancer activity. Results of both in vitro and in vivo experiments have suggested these compounds hold promise for cancer treatment because they are effective against a wide range of cancer cell types including osteosarcoma (Geng et al., 2014), prostate (Lee et al., 2009), lung (Zheng et al., 2014), gastric (Wang et al., 2010), kidney (Li et al., 2013b). Furthermore, these compounds seem to exert their anticancer actions through different cellular targets and pathways. It is crucial to attain a more complete understanding of the underlying mechanisms of the anticancer properties of icariin and its derivatives to provide direction for future research in cancer drug discovery.
Anticancer Properties
Apoptotic Activity
Over the past decade, triggering apoptosis in tumor cells has been utilized as an effective approach for treatment of cancer (Han et al., 2015). Apoptosis occurs through two different pathways: the extrinsic or death receptor pathway and the intrinsic or mitochondrial pathway (Goh et al., 2014). Both pathways of apoptosis involve the activation of initiator caspases, followed by activation of effector caspases (caspase-3, -6, and -7) which the major executioners of apoptosis (Mongiat et al., 2007; Ahmed and Othman, 2013). With reference to the intrinsic pathway, mitochondrial outer membrane permeabilization is the crucial event for initiator caspase activation (Chan et al., 2012). Herba Epimedii was shown to induce apoptosis of cancer cells; 70% ethyl alcohol extracts of Epimedium koreanum Nakai has caused activation of mitochondria-dependent apoptotic pathway in colon cancer cells (Guon and Chung, 2014). This led to further studies on the apoptotic activity induced by the bioactive components isolated from this herb. Generally, the apoptotic effect of icariin and its derivatives has been associated with the activation of the intrinsic pathway of apoptosis. Treatment with icariin and icaritin has resulted in increase in Bax/Bcl2 ratio, release of cytochrome c, cleavage of poly (ADP-ribose) polymerase and activation of caspases in a wide range of cancer cells (He et al., 2010; Li et al., 2010, 2013a, 2014b; Tong et al., 2011; Wang et al., 2011a; Zhu et al., 2011; Sun et al., 2015b; Wu et al., 2015b); these can be regulated by various signaling pathways, as shown in Table 1. Similarly, icariside II has been shown to increase the ratio of Bax/Bcl2, causing transposition of cytochrome c and activation of caspase-3 and -9 in cancer cells including lung cancer cells and acute myeloid leukemia cells. It also causes degradation of poly ADP-ribose polymerase (PARP) and reactive oxygen species (ROS) over-production (Kang et al., 2012; Song et al., 2012). Additionally, apoptosis of esophageal squamous cell carcinoma treated with icariside II has been reported to be associated with lowered expression of β-catenin and downregulation of expression of survivin and cyclin D1, suggesting that icariside II is capable of simultaneously inducing apoptosis while also inhibiting proliferation of cancer cells (Wang et al., 2011b). Other mechanisms involved include inhibition of epidermal growth factor receptor (EGFR) pathway activation (Wu et al., 2013b) which then prevents the downstream activation of the JAK/STAT3 pathway (Kim et al., 2011; Wu et al., 2013a). This shows the ability of icariside II to overcome the survival signals of tumor cells because STAT3 is one of the STAT proteins that is constitutively active in cancer cells where it promotes the expression of antiapoptotic proteins (Wu et al., 2013a). Icariside II also helps to overcome the survival signals of tumor cells by inhibiting cyclooxygenase-2 (COX-2)/PGE2 pathway (Lee et al., 2009); expression of COX-2 has been associated with tumor-cell resistance to apoptosis (Greenhough et al., 2009).
The extrinsic pathway, on the other hand, is activated by specific receptors of the tumor necrosis factor receptor superfamily, such as Fas, DR4 (TRAIL-R1), and DR5 (TRAIL-R2). The binding of their specific ligands will result in the production of death-inducing signaling complex and activation of caspase-8 and caspase-10 which will then activate the effector caspases (Mongiat et al., 2007). In some studies, both intrinsic and extrinsic pathways seem to be involved in the induction of apoptosis by icariin and its derivatives. For example, the treatment of human hepatocellular carcinoma with icaritin not only triggered mitochondrial/caspase apoptotic pathway but also induced apoptosis through Fas-mediated caspase-dependent pathway as evidenced by increased levels of Fas and cleaved caspase-8 (Sun et al., 2015b). Similar results were obtained in breast cancer cells treated with Icariside II (Huang et al., 2012).
Aside from mitochondria, organelles such as endoplasmic reticulum and lysosomes also play an important role in apoptosis of cancer cells (Geng et al., 2015). The rapid rate of glucose metabolism and growth in cancer cells causes endoplasmic reticulum stress (ERS) and activation of the unfolded protein response (UPR). As the UPR is one of the resistance mechanisms against cancer therapy, it can often enable cancer cells to adapt to ERS and evade apoptotic pathways (Yadav et al., 2014). However, some studies have shown that therapeutic induction of ERS-induced apoptosis can be useful in eradicating cancer cells if there is forced activation of ERS (Di et al., 2015; Fan et al., 2016). Recently, the role of ERS signaling in the anticancer activity of icariin has been studied on human adenocarcinoma and esophageal cancer cells. Icariin treatment upregulated ERS-related molecules such as p-PERK, ATF, GRP78, p-eIF2α, CHOP as well as apoptosis-related protein PUMA. At the same time, there was downregulation of anti-apoptosis-related protein Bcl2. This demonstrated that the apoptotic activity of icariin in cancer cells might also be due to activation of ERS signaling (Di et al., 2015; Fan et al., 2016).
Lysosomes are acidic organelles containing various hydrolytic enzymes for recycling of intracellular proteins. When the alteration in lysosomal membrane permeabilization occurred, there will be release of its hydrolytic enzymes, leading to a change in mitochondrial membrane potential and subsequent pro-apoptotic events of cells (Geng et al., 2015). In a recent study, it was shown that treatment with icariside II induced both mitochondrial and lysosomal dysfunction through mitochondrial membrane and lysosomal membrane permeabilization in human hepatoblastoma cells, leading to caspase activation and cell apoptosis (Geng et al., 2015). This shows that icariside II is able to induce cancer cell apoptosis by causing both mitochondrial and lysosomal damage.
Therefore, icariin and its derivatives appear to be effective in inducing apoptosis in a wide range of cancer cell types through a variety of cell signaling mechanisms (Figure 2).
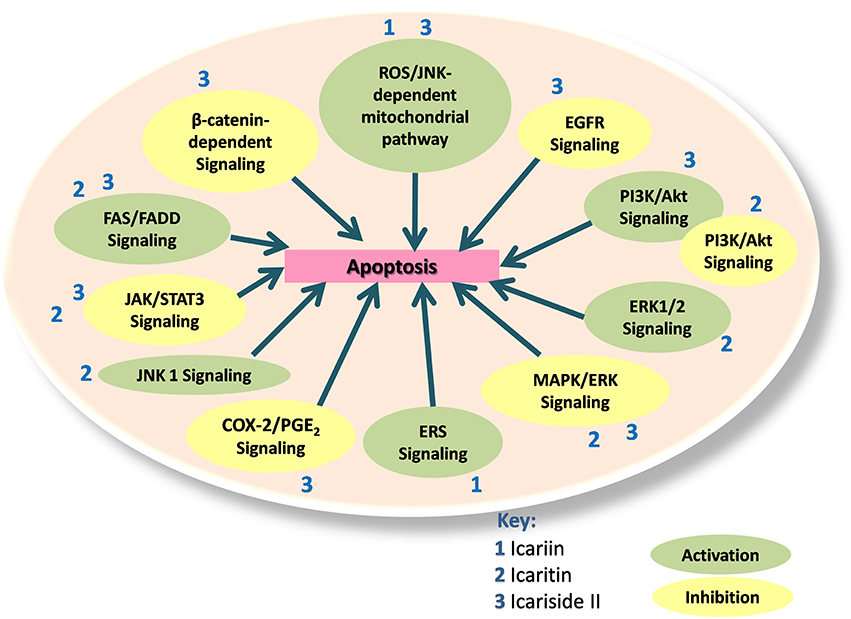
Figure 2. Icariin, icaritin, and icariside II exert apoptotic effects through multiple mechanisms, which include the inhibition of β-catenin-dependent signaling, EGFR signaling, MAPK/ERK signaling, PI3K/Akt signaling, JAK/STAT3 signaling, and COX-2/PGE2 signaling. The activated pathways are such as ROS/JNK dependent mitochondria pathway, FAS-dependent apoptosis, JNK1 signaling, ERS signaling and ERK1/2 signaling. PI3K/Akt signaling can also be activated to inactivate JAK/STAT pathway.
Cell-Cycle Modulation
The cell cycle consists of phases known as G0, G1, S, and G2M. Cyclin-dependent kinases (CDK) are the protein translating signals that push the cells through the cell cycle and the growth of cells is dependent on their capability to translate these signals for effective replication and division. When the normal regulation of cell-cycle progression and division is disrupted, uncontrolled proliferation can occur which may result in the development of cancer (Crespo-Ortiz and Wei, 2012; Dinicola et al., 2014).
Icariin and its derivatives inhibit the growth of cancer cells by causing cell cycle arrest. Icariin induces cell cycle arrest at G0/G1 and G2/M phases in gallbladder carcinoma cells and colorectal cancer cells respectively, through the inhibition of NF-κB activity (Zhang et al., 2013, 2014). Icaritin inhibits growth of human prostate carcinoma cells through G1 cell cycle arrest which is attributed to induction of p27Kip1, p16Ink4a, and pRb, as well as the down-regulation of expression of phosphorylated pRb, cyclin D1 and CDK4 (Huang et al., 2007). In other work, icaritin caused cell cycle arrest at the G2/M phase in extranodal NK/T-cell lymphoma cells (Wu et al., 2015b). Similarly, treatment of breast cancer cells with icaritin resulted in cell cycle arrest at the G2/M phase, accompanied by down-regulation of the expression of G2/M phase regulatory proteins including cyclin B, cdc2 as well as cdc25c (Guo et al., 2011). The cell cycle arrest was associated with sustained extracellular signal-regulated kinases (ERK) activation, consistent with the findings of Tong et al. (2011). Aside from these, icaritin has been shown to induce cell cycle arrest in the S phase associated with down-regulated expression levels of S regulatory proteins including Cyclin A, Cyclin B, and CDK2 in lung cancer and chronic myeloid leukemia cells (Zheng et al., 2014; Zhu et al., 2015). As shown in Figure 3, the cell cycle modulation of icaritin seems to vary from study to study and this might be due to the cell type species-differences of the cancer cell lines (Zheng et al., 2014). Icariside II also inhibits cancer cell proliferation through cell cycle modulation (Wang et al., 2011b); it was able to induce cell cycle arrest at G0/G1 and G2/M transitions. The underlying mechanism was suggested to be due to the generation of reactive oxygen species as well as activation of p38 and p53 (Wu et al., 2015a). In a study on osteosarcoma, the inhibition of cancer cell proliferation by icariside II was associated with its ability to inactivate epidermal growth factor receptor (EGFR)/mammalian target of rapamycin (mTOR) pathway, showing that icariside II might target mTOR, the master switch of tumor cell proliferation (Geng et al., 2014).
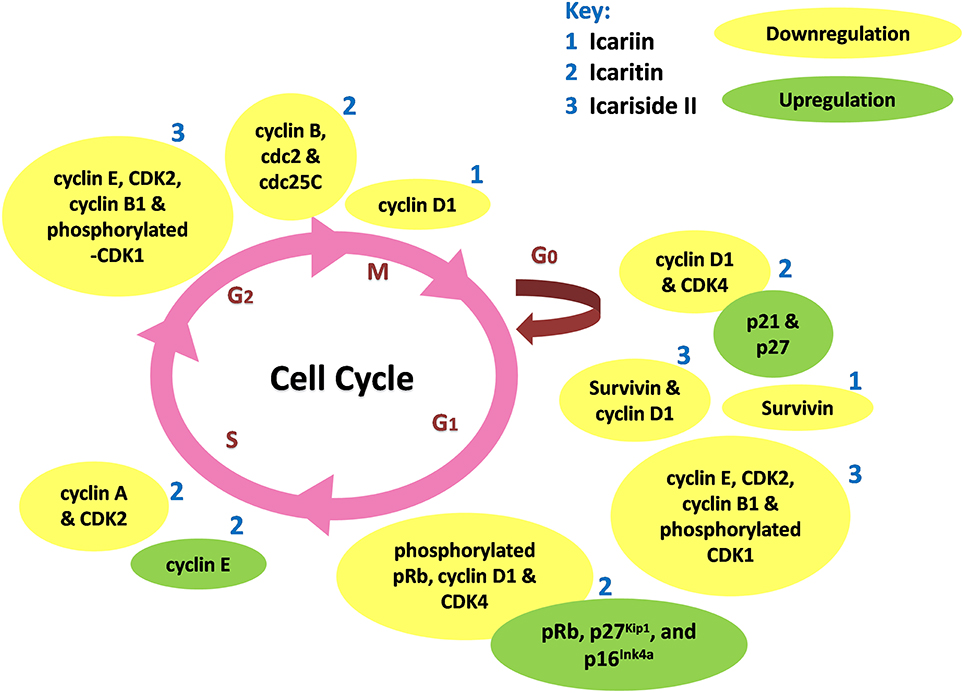
Figure 3. Cell cycle modulation by icariin and its derivatives. Icariin, icaritin, and icariside II induce cell cycle arrest through modulation of expression of cell cycle regulatory proteins at different stages of cell cycle, resulted in inhibition of tumor growth.
Based on these studies, icariin and its derivatives were demonstrated to exert cell cycle modulation at different phases resulting in an anti-proliferative effect on cancer cells; mainly attributed to the downregulation of cell cycle regulatory proteins, as summarized in Table 2.
Angiogenesis Inhibition
Angiogenesis is the vital physiological process of formation of new blood vessels; it is a normal process in growth and granulation tissue formation. Angiogenesis is crucial for cancer development because blood vessels supply the nutrition and transfer channels at every stage of the growth of tumor (Zhang, 2014). Therefore, disruption of angiogenesis can cause inhibition of tumor invasion and metastasis (Tan et al., 2016). Currently, anti-angiogenesis treatment represents one of the strategies in cancer therapy but the currently known effective anti-angiogenesis agents are prohibitively expensive.
The potential of Herba Epimedii to inhibit angiogenesis was investigated in vitro and in vivo, as summarized in Table 3. Anti-angiogenic effects of the ethyl acetate fraction of Herba Epimedii extract was demonstrated in both experimental models and might be due to inhibition of ERK signaling pathway (Yu et al., 2013). Preliminary studies on the effect of active compounds of Herba Epimedii such as icariin and icaritin on angiogenesis have been carried out by using human umbilical vein endothelial cells as a cell model of angiogenesis. Both icariin and icaritin were found to inhibit the proliferation, migration as well as tube formation of the cells (Zhang, 2014; Ye et al., 2015). In a chick embryo chorioallantoic assay, there was significant reduction in newly-formed blood vessels as well as reduced area and length of blood vessels that occurred in a dose-dependent manner following treatment of icariin and icaritin (Hong et al., 2013; Ye et al., 2015). Icariin and its derivatives have also exhibited their anti-angiogenic activity in vivo in different xenograft models of tumors such as hepatocellular carcinoma and renal cell carcinoma. CD31 is a pan-endothelial marker which is specifically expressed on the endothelial cells surfaces; in tumors of mice treated with icariin and icaritin, there was a significant decrease in the areas positive for CD31 (Yang et al., 2009; Li et al., 2013b). The anti-angiogenic effect appears to be moderated by down-regulation of vascular endothelial growth factor (VEGF), a crucial growth factor that acts as the fundamental regulator of angiogenesis (Figure 4). The results of both in vitro and in vivo studies showed a decrease in VEGF levels in both the icaritin-and icariside-II treated groups (Choi et al., 2008; Li et al., 2013b). Based on the study reported by Choi et al. (2008), the decreased level of VEGF is probably due to the reduction in transcriptional activity of hypoxia inducible factor 1-alpha, the primary regulator of the expression of VEGF. Taking all of the above into account, inhibition of VEGF is likely to be the target of icariin and its derivatives with respect their anti-angiogenic effect.
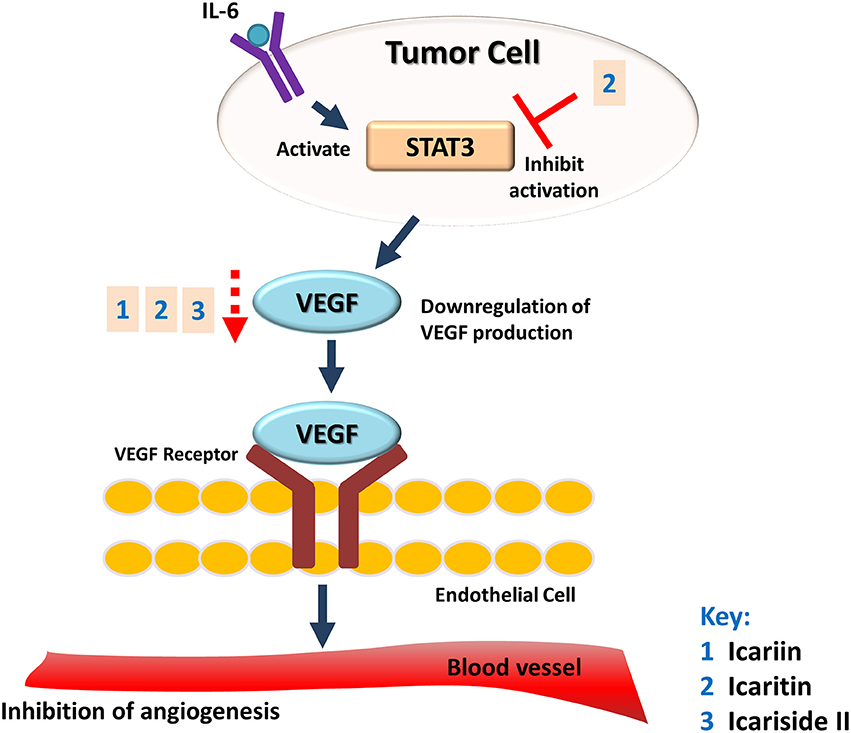
Figure 4. Anti-angiogenic effect of icariin and its derivatives. The treatment of icariin, icaritin, and icariside II have resulted in anti-angiogenic effect. This can be due to the reduction in expression of VEGF, a crucial growth factor that acts as the fundamental regulator of angiogenesis.
Inhibition of Metastasis
The malignancy of cancers is mainly due to the ability of cancer cells to invade their neighboring tissues. This process of invasion allows neoplastic cells to enter the blood stream and lymphatic system and reach other organs where secondary tumors may form. The development of metastases is the cause of treatment failure and death in cancer patients. The key steps for metastases are cell attachment, local proteolysis and cell migration (Harlozinska, 2005). Icariin and its derivatives have also been investigated with reference to their anti-metastatic activity. A study on highly metastatic human lung main cancer cells demonstrated a reduced ability of these cells to invade and migrate following treatment with icariin (Mao et al., 2000). In another study, icariin suppressed the adhesion of lung adenocarcinoma (Di et al., 2015). The possible mode of action includes effects on vasodilator-stimulated phosphoprotein (VASP) which is reported to be crucial in cell migration as well tumor metastasis. VASP function in turn is regulated by Rac, a member of the Rho family proteins (Schlegel et al., 2000). The study has also revealed the inhibition of human gastric cancer cell invasion and cell migration by icariin and the underlying mechanism was suggested to be the suppression of expression of cell motility-related genes Rac1 and VASP (Wang et al., 2010).
Icaritin was also tested on glioblastoma cell line. There was suppression of adhesion, migration and invasion of glioblastoma cells, due to downregulation of extracellular matrix metalloproteinase via PTEN/Akt/HIF-1α pathway (Xu et al., 2015). Icariside II was also found to exert anti-metastatic effects as it inhibited the migration of human osteosarcoma cells by suppressing the transcription of hypoxia-inducible genes involved in tumor cell invasion, including urokinase plasminogen activator receptor, adrenomedullin, and matrix metalloproteinase 2 (Choi et al., 2008). The CXCR4/CXCL12 signaling axis is known to be involved in the metastasis of cancer cells and CXCR4 chemokine receptors are highly expressed in various tumors compared to normal cells (Kim and Park, 2014). In a study, the anti-metastatic activity of icariside II was shown to be correlated with downregulation of CXCR4 at transcriptional level through the suppression of NF-κB activation (Kim and Park, 2014). The chronic inflammation that occurs during cancer treatment is an important factor that induces tumor metastasis because an inflammatory microenvironment is favorable for cancer invasion and metastasis through epithelial-mesenchymal transition (EMT). Studies have been done on the effect of exposure to icariside II on the migration of non-small cell lung cancer cells in an inflammatory microenvironment; the exposure of icariside II resulted in the inhibition of TNF-α-boosted EMT, migration and invasion of cancer cells, probably through the inhibition of Akt/ NF-κB pathway. This is supported by the results of an in vivo study that showed anti-metastastic effect of icariside II on tumor in nude mice (Song et al., 2016).
The findings of these studies revealed that icariin and its derivatives inhibit the metastasis of cancer cells through downregulation of proteins that are crucial for cancer metastasis, as summarized in Table 4 and Figure 5.
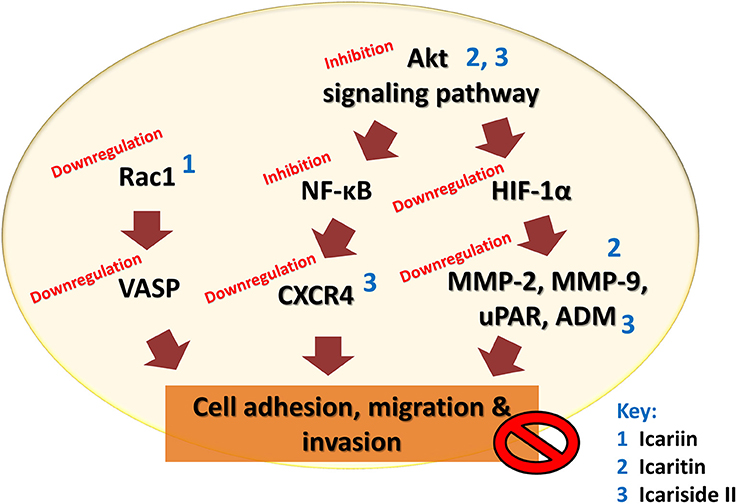
Figure 5. Anti-metastatic effect of icariin and its derivatives. Icariin, icaritin, and icariside II inhibit metastasis of tumor by inhibiting cell adhesion, migration, and invasion through multiple mechanisms that resulted in downregulation of VASP, CXCR4, uPAR, ADM, and matrix metalloproteinases.
Inhibition of Hormone Dependent Cancers
Breast and prostate cancers are the commonest invasive hormone dependent cancers in females and males, respectively. Although these two types of cancers have different physiological features depending on the organs where they arise, they have common pathological features of “hormonal-dependence” (Belev and Vrbanec, 2013). The initiation and progression of these diseases are based on the modulation of hormone effects through specific steroid hormone receptors including estrogen receptors, progesterone receptors and androgen receptors (Rau et al., 2005).
Estrogens are known to stimulate the growth of breast cancer and most breast cancers have overexpression of estrogen receptor alpha (ERα). It has been found that some ERα –positive tumors can develop resistance toward hormone therapy. These indicate a role of ERα in initiation and progression of cancer (Ali and Coombes, 2000). Icaritin has been reported to inhibit the estradiol-stimulated growth of ERα-positive breast cancer cells by destabilizing ERα protein. Icaritin activates the aryl hydrocarbon (Ahr)-mediated pathways by acting as an Ahr agonist; resulting in Ahr-mediated proteosomal pathways and degradation of ERα protein which ultimately reduces the maximal estrogen responsiveness. In an athymic nude mouse model, icaritin also restricted estradiol-stimulated breast cancer xenograft growth and caused strong reduction in ERα protein levels (Tiong et al., 2012). This finding is consistent with another recent study which showed undetectable levels of ERα protein in breast cancer tumors of nude mice due to the presence of icaritin and desmethylicaritin in serum after the administration of Epimedium bervicornum extract (Indran et al., 2014).
Androgens play a crucial role for survival and growth of prostatic carcinoma cells; hence, androgen deprivation therapy has been used as the mainstay of treatment for prostate cancer. This includes the reduction of gonadal androgen synthesis as well as disruption of androgen receptor (AR) signaling axis. However, the mechanism of cancer development has evolved and some variants have developed into castration-resistant prostate cancer (CRPC) (Belev and Vrbanec, 2013). This occurs where there is persistent AR signaling which is postulated to be produced by AR COOH-terminal truncated splice variants (ARvs) (Sun et al., 2015a). Recently, icaritin has been tested on CRPC cells and was found to exert similar effects to those seen in estrogen responsive breast cancer, with promotion of degradation of AR and ARvs through the binding of icaritin to Ahr. Consequently, the transcription of genes regulated by AR and ARvs such as KLK3 and UBE2C are suppressed, inducing apoptosis of cancer cells. In an in vivo study, reduction of tumor growth was seen in murine models implanted with androgen-sensitive and CRPC cells, due to inhibition of AR signaling by icaritin (Sun et al., 2015a). In another study, the treatment of androgen receptor-positive prostate cancer cells with icariside II resulted in downregulation of expression of KLK3 gene, showing the role of icariside II in inhibition of AR signaling (Miura et al., 2015).
Based on the research, icaritin and its derivative are effective against hormone dependent cancers that are resistant toward hormone therapy by promoting the degradation of hormone receptors through Ahr-dependent pathway.
Inhibition of Cancer Stem Cells
Cancer stem cells (CSCs), also known as cancer/tumor-initiating cells, can be defined as a small population of cancer cells that are able to self-renew and differentiate into a wide range of progeny cells that make up the tumor as well as reform the original tumor following xenotransplantation (Kim et al., 2009). Although conventional cancer therapy—including radio and chemotherapy—destroy the bulk of cancer cells, they often fail to eradicate the critical CSCs due to inherent resistance mechanisms. Consequently, CSCs form new tumors, which results in relapse of the cancers (Vinogradov and Wei, 2012). The inhibitory effect of icaritin on CSCs has been evaluated with one study reporting more effective inhibition of growth of breast cancer stem/progenitor cells following treatment with icaritin compared to the standard chemopreventive agent tamoxifen (Guo et al., 2011). Recently, the inhibition of malignant growth of hepatocellular carcinoma initiating cells (HCICs) by icaritin has been demonstrated (Zhao et al., 2015). The hepatosphere cells (HCIC that formed spherical clusters) were inhibited from forming secondary passage of hepatospheres due to the effect of icaritin which negatively regulated the self-renewal of HCICs. In addition, the hepatosphere cells lost their ability to initiate tumor. In in vivo work, growth of primary and secondary xenografts in NOD/SCID mice were also suppressed. The underlying mechanism for these observed effects was proposed to be the attenuation of IL-6 receptors/Jak2/Stat3 signaling (Zhao et al., 2015). These recent findings show the potential of icaritin in eradicating CSCs; and seem to suggest that it may be worthwhile conducting further studies on icariin and its other derivatives to evaluate their effectiveness against other types of CSCs.
Inhibition of Resistant Cancer Cells
Another huge challenge for anticancer therapy is the emergence of drug resistance over the course of treatment which has led to drug-limiting toxicities and failure of chemotherapy (Crespo-Ortiz and Wei, 2012; Chan et al., 2016). The resistance of cancer cells can develop via biological mechanisms such as reduced uptake of drugs, changes in cellular pathways and increased drug efflux. The most common mechanism is overexpression of ATP-binding cassette (ABC) transporters such as P-glycoprotein (P-gp) that extrudes the chemotherapeutic agents from the cells (Liu et al., 2009). In the study by Song et al. (2014), the combined treatment of Epimedium koreanum Nakai extract with gefitinib overcame the resistance of non-small cell lung cancer cells to gefitinib, showing the potential of bioactive components in Herba epimedii to treat cancers with drug-resistance. Hence, studies have been carried out to unravel the reversal of drug resistance of tumor cells by icariin and its derivatives. One study using multiple drug-resistant (MDR) human hepatocellular carcinoma/Adriamycin (ADR) cell line revealed the capability of icaritin to reverse the cytotoxicity of ADR in a dose-dependent manner, which was due to the decrease in expression of MDR1 gene, decrease in P-gp level and increased intracellular accumulation of ADR (Sun et al., 2013). Similar results were obtained in a study on MDR reversal activities of a series of semi-synthesized icariin derivatives using ADR-resistant breast carcinoma cells (Liu et al., 2009). In another study, the treatment of icariin resulted in the inhibition of the P-gp-mediated active transport process. In addition, there was enhanced doxorubicin (DOX)-induced apoptosis in DOX-resistant human osteosarcoma cells and this was due to the inhibition of PI3K activity and reduced phosphorylation of Akt in the cells (Wang et al., 2015b). Recently, icaritin has been shown to overcome the resistance of human glioblastoma cells to apoptosis caused by tumor necrosis factor-related apoptosis-inducing ligand (TRAIL). Treatment with icaritin has been shown to cause the inhibition of NF-κB signaling which in turn leads to the suppression of the expression of cellular PLICE-inhibitory protein (c-FLIP), the inhibitor of apoptotic signaling. Consequently, TRAIL-induced apoptosis in glioblastoma cells can be sensitized (Han et al., 2015). Overall, icariin and its derivatives are able to reverse the drug resistance of cancer cells, mainly by inhibiting the activity of P-gp and promoting the accumulation of drug in cancer cells.
Immunomodulation
There is increased interest in focusing the application of tumor immunology for cancer therapy (Ménard et al., 2008). Myeloid-derived suppressor cells (MDSCs) are part of the myeloid-cell lineage and they are the heterogenous population of cells which consist of myeloid-cell progenitors and precursors of myeloid cells. In healthy individuals, MDSCs quickly differentiate into mature granulocytes, macrophages, or dendritic cells (DCs). However, in pathological conditions such as cancer, differentiation of MDSCs and subsequent expansion of the cell population is blocked (Gabrilovich and Nagaraj, 2009). As MDSCs possess immunosuppressive functions, their accumulation is thought to be crucial in protecting the tumor cells from immune-mediated killing which then facilitates tumor growth and metastasis, especially in solid cancers. Therefore, targeting MDSCs could be another approach for cancer therapy (Marvel and Gabriolovich, 2015). In a study, in vitro treatment of MDSCs with icaritin led to a reduced proportion of MDSCs with induction of differentiation of the cells into macrophages and DCs. There have been in vivo experiments with tumor bearing mice where treatment with icaritin and icariin has delayed tumor progression and reduced the percentage of MDSCs. The levels of arginase, nitric oxide and ROS were determined as they are involved in MDSC-mediated T-cell suppression and the results showed significantly lower production of nitric oxide and ROS as well as restoration of CD8+ T cells functional capacity. In addition to that, there was downregulation of expression of S100A8 and S100A9, the pro-inflammatory mediators that inhibit the differentiation of MDSCs. There was also inhibition of activation of STAT and AKT (Zhou et al., 2011). Based on these studies, the immunomodulatory effect of icariin and icaritin indicates that they are not only effective in killing tumor cells but they are also involved in antitumor immunity for tumor regression.
Applications in Medicines
Besides the studies on icariin and its derivatives alone as potential anticancer agents, there is tremendous interest in using these compounds in combination with standard anticancer chemotherapy, as shown in Table 5. This is to enhance the efficacy of the chemotherapy while reducing the side effects and complications (Wang et al., 2015c). While treatment with either icariin or temozolomide resulted in inhibition of cell proliferation of glioblastoma cells, cell apoptosis and cell migration inhibition; a combination of icariin and temozolomide led to significant enhancement of these antitumor activities compared to the treatment using either agent alone. The synergistic effect was correlated with the suppression of NF-κB activity (Yang et al., 2015). This is consistent with synergistic effects that were observed in other in vitro and in vivo studies using different anticancer drugs such as gemcitabine, 5-florouracil and arsenic trioxide. Other than inhibition of NF-κB activity, an increase in the generation of intracellular ROS was observed (Zhang et al., 2013; Li et al., 2014a; Shi et al., 2014; Wang et al., 2015c). Recently, the effect of icaritin used in combination with epirubicin against bladder cancer cells was investigated. The results showed that icaritin acted synergistically with epirubicin via suppression of autophagy (Pan et al., 2016). For extranodal NK/T-cell lymphoma cells, icaritin potentiated ganciclovir-induced apoptosis through induction of lytic Epstein–Barr virus infection (Wu et al., 2015b). With reference to studies on icariside II, a synergistic effect was also observed in different cell lines when it was used in combination with drugs such as bortezomib, thalidomide and paclitaxel. There was an increase in the expression of cleaved caspase 3, showing that icariside II potentiated the apoptosis of cancer cells induced by these chemotherapeutic agents with the possible mechanisms involved being inhibition of STAT3 signaling pathways as well as TLR4-MyD88-ERK signaling (Kim et al., 2011; Wu et al., 2012).
Other than chemotherapy, ionizing radiation (IR) therapy is also used as a main treatment of many types of malignancies. However, there are some drawbacks of IR therapy such as treatment-induced resistance, epidermitis and higher risk of cardiovascular disease. Hence, there is a need for an agent or radiosensitizer that can overcome radioresistance and sensitize the malignant cells to radiation. This will also help to enhance the tumor response facilitating the use of lower therapeutic doses of radiation which will help reduce toxicity to other organs (Hong et al., 2013; Zhang et al., 2014). The synergistic effect of icaritin with IR has been demonstrated (Hong et al., 2013) through three main pathways. Firstly, icaritin blocks the activation of survival signaling such as ERK1/2 and AKT pathways of breast cancer cells that are caused by IR stress. Secondly, icaritin enhances the accumulation of cells in G2/M phase, causing more cells to die. Thirdly, the induction of necrosis and apoptosis of cancer cells by icaritin occurs in a dose-dependent manner, preventing the IR-insulted cells from repairing the damage. Similar results were obtained in a study that used icariin as a radiosensitizer of colorectal cancer cells (Zhang et al., 2014). One of the possible underlying mechanisms for these observed effects is the suppression of NF-κB activity by icaritin, which then prevents the activation of pro-proliferative genes such as ERK1/2 and AKT as well as causing cell cycle arrest in G2/M phase. Based on the reported studies, co-treatment using icariin and its derivatives in combination with standard cancer therapies results in positive effects because they potentiate apoptosis of cancer cells induced by chemotherapy through their action on different signaling pathways. In addition to that, they also help to overcome the radioresistance of cancer cells.
There are a number of patents registered related to the application of icariin and its derivatives in cancer treatment. For example, icariin has been used to treat melanoma and breast cancers (Dong et al., 2011). Icaritin has been utilized in the preparation of medicaments for primary liver cancer and inhibition of liver cancer stem cells (Meng et al., 2014, 2015); while Zhang and Calderwood (2008) reported the application of desmethylicaritin as the active ingredient of an antitumor drug. Broad-spectrum antitumor effects have been shown and it is found to be maintained at a particular concentration in plasma, which is a desirable property for antitumor effect. Icaritin has also been used together with juglanin B for the treatment of hepatocellular carcinoma (Zhao et al., 2014). Other derivatives such as icariinaglycon and cyclo-icariin have also been utilized in treatment of different cancer cell lines (Wang et al., 2011c; Jia et al., 2014). The preparations of icariin and its derivatives for cancer treatment appear to be advantageous because they show low toxicity to normal cells and no obvious adverse effects (Meng et al., 2014; Wei et al., 2015).
Comparison of Potency of Icariin Derivatives and Challenges in Clinical Usage
In a comparative study done by Huang et al. (2007), icariin and icaritin exert anticancer effect against PC-3 cells to different extents. Icaritin had a stronger effect on PC-3 cells by causing G1 and G2/M arrest whereas icariin only caused weak G1 arrest. The different level of potency of the two compounds in inhibiting cell growth could be due to the difference in their chemical structures. The possible structure-activity relationship was further demonstrated by another study with icariin and icaritin. Although both icariin and icaritin exerted immunomodulatory and antitumor effects, icariin showed weaker action as it did not affect the proportion of MDSCs, macrophages and DCs in vitro and furthermore, had no effect on the expression of S100A8/9 in MDSCs in vitro (Zhou et al., 2011).
Pharmacological studies have clearly shown that icariin, icaritin and icariside II possess anticancer activity but the challenge for their experimental studies is the small amount of natural icaritin and icariside II present in Herba Epimedii. In order to increase the yield of icaritin and icariside II from icariin, enzyme hydrolysis methods have been used to prepare higher amounts of these metabolites for pharmacological studies (Xia et al., 2010; Jin et al., 2012). Another impediment to the clinical application of icariin, icaritin and icariside II is their low solubility in water which will lead to low in vivo bioavailability (Cai et al., 2011; Wang et al., 2015a). In order to enhance their water solubility, other derivatives have also been synthesized. For example, Wang et al. (2015a) prepared a series of icaritin derivatives bearing carboxylic acid or carboxylic ester groups and their enhanced cytotoxic activity against breast and lung cancer cells was demonstrated. Song et al. (2015) demonstrated another approach for the enhancement of solubility, which is by incorporating icariside II into phospholipid/d-α-tocopheryl polyetheyene glycol 1000 succinate (TPGS) mixed micelles. The mixed micelles loaded with icariside II showed stronger inhibition action on the proliferation of breast cancer cells compared to icariside II alone. Similar effects were observed in lung cancer cells treated with lecithin/Solutol HS 15 loaded with icariside II (Yan et al., 2015). These results demonstrate that icariin and its derivatives have real potential given that one of the main potential limitations of low solubility can be overcome via the application of novel drug delivery systems.
Conclusion
Overall, the existing research provides supporting scientific-based evidence that substantiates the use of Herba Epimedii-containing herbal medicine for anticancer treatment. The active compound in Herba Epimedii, icariin and its derivatives possess tremendous potential for treatment of many different cancers—including solid and non-solid tumors. They were found to act through a wide variety of mechanisms as shown in Figure 6. The tantalizing promise of these compounds as suggested by the current work is the ability of these substances to overcome the limitations of current anticancer therapy—including the ability to effectively kill cancer stem cells and help overcome hormone therapy resistance in hormone dependent cancers. Their anticancer activity which goes beyond cytotoxicity to include more novel mechanisms such as immunotherapy is also a fascinating aspect which begs further study. Given that icariin and its various derivatives seem to have different levels of potency with reference to their anticancer effects, work remains to be done to identify the ones with the strongest anticancer activity; this is at the moment challenging as the full details of their mechanisms of action are still not known and the amount of comparative studies at present are limited. The safety profile of these compounds appears promising as clinical trials and a patents study suggest that they can safely be used without adverse effects. All in all, icariin and its derivatives have tremendous potential as candidates for research in the development of new anti-cancer agents.
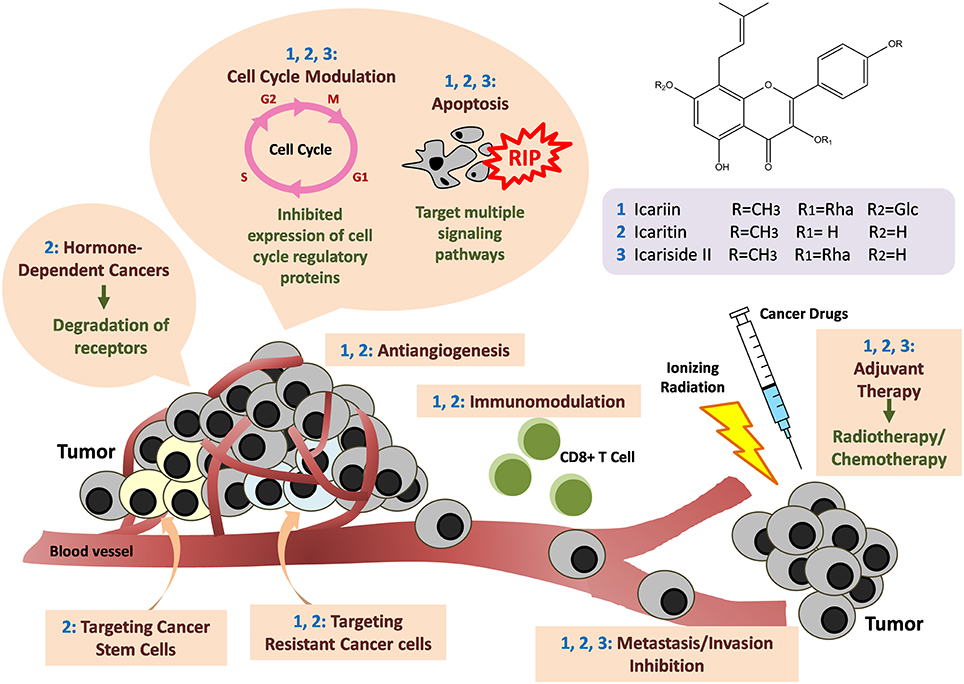
Figure 6. Anti-cancer mechanisms of icariin and its derivatives. Icariin and its derivatives mainly inhibit the growth of tumor through induction of apoptosis by targeting multiple signaling pathways. Cell cycle arrests also occur through downregulation of the expression of cell cycle regulatory proteins. Besides, there are anti-angiogenesis, anti-metastasis and immunomodulation. The compounds are also effective in targeting hormone-dependent cancers, cancer stem cells, and drug-resistant cancer cells. There are synergistic effects when icariin and its derivatives are used together with standard cancer therapy such as chemotherapy or radiotherapy.
Author Contributions
All authors listed, have made substantial, direct and intellectual contribution to the work, and approved it for publication.
Conflict of Interest Statement
The authors declare that the research was conducted in the absence of any commercial or financial relationships that could be construed as a potential conflict of interest.
Acknowledgments
This work was supported by the MONASH PVC Award Grant (Project Q7 No. PVC-ECR-2016), Monash University Malaysia ECR Grant (5140077-000-00), External Industry Grant (Biotek Abadi Vote No. GBA-808813), Fundamental Research Grant Scheme (FRGS/1/2014/SKK01/MUSM/03/2) of Malaysia Ministry of Higher Education, MOSTI eScience Funds (02-02-10-SF0215 and 06-02-10-SF0300), University of Malaya for High Impact Research Grant (UM-MOHE HIR Nature Microbiome Grant No. H-50001-A000027 and No. A000001-50001).
Abbreviations
ADM, adrenomedullin; AIF, Apoptosis inducing factor; Apaf-1, Apoptotic protease activating factor 1; ATF, activating transcription factor; Bad, Bcl-2-associated agonist of cell death; Bax, Bcl-2-associated X protein; Bcl, B-cell lymphoma 2; CAM, Chick chorioallantoic membrane; CDK, cyclin-dependent kinases; CHOP, C/EBP homologous protein; COX-2, cyclooxygenase-2; c-src, Proto-oncogene tyrosine-protein kinase Src; CXCR4, chemokine (C-X-C Motif) receptor 4; EBV, Epstein-Barr virus; eIF2α, α -subunit of eukaryotic translational initiation factor 2; EGFR, Epidermal growth factor receptor; EMMPRIN, extracellular matrix metalloproteinase; EMT, epithelial-mesenchymal transition; ERK, extracellular signal-regulated kinases; ERS, endoplasmic reticulum stress; FADD, Fas-associated protein with death domain; GRP, glucose regulated protein; HIF-1α, hypoxia-inducible factor 1-alpha; HUVECs, human umbilical vein endothelial cells; iNOS, Inducible nitric oxide synthase; IR, Ionizing radiation; JNK, Jun N-terminal kinases; LMP1, Latent membrane protein 1; MAPK, Mitogen-activated protein kinases; MMP, matrix metalloproteinase; mTOR, mechanistic target of rapamycin; NF-κB, nuclear factor-kappa beta; PARP, poly ADP-ribose polymerase; PGE2, prostaglandin E2; PI3K, phosphoinositide 3-kinase; Piwil4, piwi-like protein 4; pRb, retinoblastoma protein; PTEN, phosphatase and tensin homolog; PUMA, p53 upregulated modulator of apoptosis; ROS, reactive oxygen species; STAT3, signal transducer and activator of transcription 3; TLR4, toll-like receptor 4; uPAR, urokinase plasminogen activator receptor; VASP, Vasodilator-stimulated phosphoprotein; VEGF, vascular endothelial growth factor.
References
Ahmed, S., and Othman, N. H. (2013). Honey as a potential natural anticancer agent: a review of its mechanisms. Evid. Based Complement. Alternat. Med. 2013, 7. doi: 10.1155/2013/829070
Ali, S., and Coombes, R. C. (2000). Estrogen receptor alpha in human breast cancer: occurrence and significance. J. Mammary Gland Biol. Neoplasia 5, 271–281. doi: 10.1023/A:1009594727358
Arief, Z. M., Munshi, A. H., and Shawl, A. S. (2015). Evaluation of medicinal value of Epimedium elatum on the basis of pharmacologically active constituents, Icariin and Icariside-II. Pak. J. Pharm. Sci. 28, 1665–1669. Available online at: http://www.pjps.pk/wp-content/uploads/pdfs/28/5/Paper-15.pdf
Belev, B., and Vrbanec, D. (2013). Hormonal resistance in breast- and prostate cancer. Period. Biol. 114, 511–517. Available online at: hrcak.srce.hr/file/149266
Cai, W. J., Huang, J. -H., Zhang, S. -Q., Wu, B., Kapahi, P., Zhang, X. -M., et al. (2011). Icariin and its derivative icariside II extend healthspan via Insulin/IGF-1 pathway in C. elegans. PLoS ONE 6:e28835. doi: 10.1371/journal.pone.0028835
Cassileth, B. R., Yeung, K. S., and Gubili, J. (2010). Herb-Drug Interactions in Oncology. Shelton, CT: People's Medical Publishing House-USA.
Chan, C. K., Goh, B. H., Kamarudin, M. N. A., and Kadir, H. A. (2012). Aqueous fraction of Nephelium ramboutan-ake rind induces mitochondrial-mediated apoptosis in HT-29 human colorectal adenocarcinoma cells. Molecules 17, 6633–6657. doi: 10.3390/molecules17066633
Chan, C. K., Supriady, H., Goh, B. H., and Kadir, H. A. (2015). Elephantopus scaber induces apoptosis through ROS-dependent mitochondrial signaling pathway in HCT116 human colorectal carcinoma cells. J. Ethnopharm. 168, 291–304. doi: 10.1016/j.jep.2015.03.072
Chan, W. K., Tan, L. T. H., Chan, K. G., Lee, L. H., and Goh, B. H. (2016). Nerolidol: a sesquiterpene alcohol with multi-faceted pharmacological and biological activities. Molecules 21:529. doi: 10.3390/molecules21050529
Chen, M., Hao, J., Yang, Q., and Li, G. (2014). Effects of icariin on reproductive functions in male rats. Molecules 19, 9502–9514. doi: 10.3390/molecules19079502
Cho, N. J., Sung, S. H., Lee, H. S., Jeon, M. H., and Kim, Y. C. (1995). Anti-hepatotoxic activity of icariside II, a constituent of Epimedium koreanum. Arch. Pharmacal. Res. 18, 289–292. doi: 10.1007/BF02976415
Choi, H. J., Eun, J. S., Kim, D. K., Li, R. H., Shin, T. Y., Park, H., et al. (2008). Icariside II from Epimedium koreanum inhibits hypoxia-inducible factor-1alpha in human osteosarcoma cells. Eur. J. Pharmacol. 579, 58–65. doi: 10.1016/j.ejphar.2007.10.010
Crespo-Ortiz, M. P., and Wei, M. Q. (2012). Antitumor activity of artemisinin and its derivatives: from a well-known antimalarial agent to a potential anticancer drug. J. Biomed. Biotechnol. 2012:247597. doi: 10.1155/2012/247597
Di, S., Fan, C., Yang, Y., Jiang, S., Liang, M., Wu, G., et al. (2015). Activation of endoplasmic reticulum stress is involved in the activity of icariin against human lung adenocarcinoma cells. Apoptosis 20, 1229–1241. doi: 10.1007/s10495-015-1142-0
Ding, L., Liang, X. G., Zhu, D. Y., and Lou, Y. J. (2007). Icariin promotes expression of PGC-1α, PPARα, and NRF-1 during cardiomyocyte differentiation of murine embryonic stem cells in vitro. Acta Pharmacol. Sin. 28, 1541–1549. doi: 10.1111/j.1745-7254.2007.00648.x
Dinicola, S., Cucina, A., Antonacci, D., and Bizzarri, M. (2014). Anticancer effects of grape seed extract on human cancers: a review. J. Carcinog. Mutagen S8:005. doi: 10.4172/2157-2518.S8-005
Dong, J., Wu, J., Xu, Y., Le, J., Cai, C., and Du, W. (2011). Medical Application of Icariin in Preparing Medicament for Antagonizing Myeloid-Derived Suppressor Cells. CN. Patent No102106864. Beijing: State Intellectual Property Office of the P.R.C.
Fan, C., Yang, Y., Liu, Y., Jiang, S., Di, S., Hu, W., et al. (2016). Icariin displays anticancer activity against human esophageal cancer cells via regulating endoplasmic reticulum stress-mediated apoptotic signaling. Sci. Rep. 19:21145. doi: 10.1038/srep21145
Gabrilovich, D. I., and Nagaraj, S. (2009). Myeloid-derived-suppressor cells as regulators of the immune system. Nat. Rev. Immunol. 9, 162–174. doi: 10.1038/nri2506
Geng, Y. D., Yang, L., Zhang, C., and Kong, L. Y. (2014). Blockade of epidermal growth factor receptor/mammalian target of rapamycin pathway by Icariside II results in reduced cell proliferation of osteosarcoma cells. Food Chem. Toxicol. 73, 7–16. doi: 10.1016/j.fct.2014.08.002
Geng, Y. D., Zhang, C., Shi, Y. M., Xia, Y. Z., Guo, C., Yang, L., et al. (2015). Icariside II-induced mitochondrion and lysosome mediated apoptosis is counterbalanced by an autophagic salvage response in hepatoblastoma. Cancer Lett. 366, 19–31. doi: 10.1016/j.canlet.2015.05.032
Goh, B. H., Chan, C. K., Kamarudin, M. N. A., and Abdul Kadir, H. (2014). Swietenia macrophylla King induces mitochondrial-mediated apoptosis through p53 upregulation in HCT116 colorectal carcinoma cells. J. Ethnopharm. 153, 375–385. doi: 10.1016/j.jep.2014.02.036
Goh, B. H., and Kadir, H. A. (2011). In vitro cytotoxic potential of Swietenia macrophylla King seeds against human carcinoma cell lines. J. Med. Plants Res 5, 1395–1404. Available online at: http://www.academicjournals.org/journal/JMPR/article-full-text-pdf/5C6A11016968
Greenhough, A., Smartt, H. J., Moore, A. E., Roberts, H. R., Williams, A. C., Paraskeva, C., et al. (2009). The COX-2/PGE2 pathway: key roles in the hallmarks of cancer and adaptation to the tumour microenvironment. Carcinogenesis 30, 377–386. doi: 10.1093/carcin/bgp014
Guo, Y., Zhang, X., Meng, J., and Wang, Z. Y. (2011). An anticancer agent icaritin induces sustained activation of the extracellular signal-regulated kinase (ERK) pathway and inhibits growth of breast cancer cells. Eur. J. Pharmacol. 658, 114–122. doi: 10.1016/j.ejphar.2011.02.005
Guon, T., and Chung, H. (2014). Effect of Epimedium koreanum Nakai on apoptosis in HCT116 human colon cancer cells. Food Eng. Prog. 18, 154–159. doi: 10.13050/foodengprog.2014.18.2.154
Gupta, S. C., Kannappan, R., Reuter, S., Kim, J. H., and Aggarwal, B. B. (2011). Chemosensitization of tumors by resveratrol. Ann. N.Y. Acad. Sci. 1215, 150–160. doi: 10.1111/j.1749-6632.2010.05852.x
Han, H., Xu, B., Hou, P., Jiang, C., Liu, L., Tang, M., et al. (2015). Icaritin sensitizes human glioblastoma cells to TRAIL-induced apoptosis. Cell Biochem. Biophys. 72, 533–542. doi: 10.1007/s12013-014-0499-y
Harlozinska, A. (2005). Progress in molecular mechanisms of tumor metastasis and angiogenesis. Anticancer Res. 25, 3327–3333. Available online at: http://ar.iiarjournals.org/content/25/5/3327.full.pdf
He, J., Wang, Y., Duan, F., Jiang, H., Chen, M., and Tang, S. (2010). Icaritin induces apoptosis of HepG2 cells via the JNK1 signaling pathway independent of the estrogen receptor. Planta Med. 76, 1834–1839. doi: 10.1055/s-0030-1250042
Hong, J., Zhang, Z., Lv, W., Zhang, M., Chen, C., Yang, S., et al. (2013). Icaritin synergistically enhances the radiosensitivity of 4T1 breast cancer cells. PLoS ONE 8:e71347. doi: 10.1371/journal.pone.0071347
Hsiao, W. L., and Liu, L. (2010). The role of traditional Chinese herbal medicines in cancer therapy–from TCM theory to mechanistic insights. Planta Med. 76, 1118–1131. doi: 10.1055/s-0030-1250186
Huang, C., Chen, X., Guo, B., Huang, W., Shen, T., Sun, X., et al. (2012). Induction of apoptosis by Icariside II through extrinsic and intrinsic signaling pathways in human breast cancer MCF7 cells. Biosci. Biotechnol. Biochem. 76, 1322–1328. doi: 10.1271/bbb.120077
Huang, X., Zhu, D., and Lou, Y. (2007). A novel anticancer agent, icaritin, induced cell growth inhibition, G1 arrest and mitochondrial transmembrane potential drop in human prostate carcinoma PC-3 cells. Eur. J. Pharmacol. 564, 26–36. doi: 10.1016/j.ejphar.2007.02.039
Indran, I. R., Zhang, S. J., Zhang, Z. W., Sun, F., Gong, Y., Wang, X., et al. (2014). Selective estrogen receptor modulator effects of epimedium extracts on breast cancer and uterine growth in nude mice. Planta Med. 80, 22–29. doi: 10.1055/s-0033-1360112
Jia, X., Jiang, J., Zhang, Z., and Song, J. (2014). Application of Cyclo-Icariin in Producing Antitumor Composition. CN. Patent No 103860542. Beijing: State Intellectual Property Office of the P.R.C.
Jin, X., Zhang, Z., Sun, E., Li, S., and Jia, X. (2012). Statistically designed enzymatic hydrolysis of an icariin/β-cyclodextrin inclusion complex optimized for production of icaritin. Acta Pharm. Sin. B 2, 83–89. doi: 10.1016/j.apsb.2011.12.004
Kang, S. -H., Jeong, S. -J., Kim, S. -H., Kim, J. -H., Jung, J. -H., Koh, W., et al. (2012). Icariside II induces apoptosis in U937 acute myeloid leukemia cells: role of inactivation of STAT3-related signaling. PLoS ONE 7:e28706. doi: 10.1371/journal.pone.0028706
Khan, M., Maryam, A., Qazi, J. I., and Ma, T. (2015). Targeting apoptosis and multiple signaling pathways with icariside II in cancer cells. Int. J. Biol. Sci. 11, 1100–1112. doi: 10.7150/ijbs.11595
Kim, B., and Park, B. (2014). Baohuoside I suppresses invasion of cervical and breast cancer cells through the downregulation of CXCR4 chemokine receptor expression. Biochemistry 53, 7562–7569. doi: 10.1021/bi5011927
Kim, S. H., Ahn, K. S., Jeong, S. J., Kwon, T. R., Jung, J. H., Yun, S. M., et al. (2011). Janus activated kinase 2/signal transducer and activator of transcription 3 pathway mediates icariside II-induced apoptosis in U266 multiple myeloma cells. Eur. J. Pharmacol. 654, 10–16. doi: 10.1016/j.ejphar.2010.11.032
Kim, Y., Joo, K. M., Jin, J., and Nam, D. H. (2009). Cancer stem cells and their mechanism of chemo-radiation resistance. Int. J. Stem Cells 2, 109–114. doi: 10.15283/ijsc.2009.2.2.109
Lau, W. K., Goh, B. H., Kadir, H. A., Shu-Chien, A. C., and Muhammad, T. S. (2015). Potent PPARγ ligands from Swietenia macrophylla are capable of stimulating glucose uptake in muscle cells. Molecules 20, 22301–22314. doi: 10.3390/molecules201219847
Lee, K. S., Lee, H. J., Ahn, K. S., Kim, S. H., Nam, D., Kim, D. K., et al. (2009). Cyclooxygenase-2/prostaglandin E2 pathway mediates icariside II induced apoptosis in human PC-3 prostate cancer cells. Cancer Lett. 280, 93–100. doi: 10.1016/j.canlet.2009.02.024
Lei, S. W., Cui, G., Leung, G. P. H., Luk, S. C. W., Hoi, M. P. M., Wang, L., et al. (2015). Icaritin protects against oxidative stress-induced injury in cardiac H9c2 cells via Akt/Nrf2/HO-1 and calcium signalling pathways. J. Funct. Foods 18, 213–223. doi: 10.1016/j.jff.2015.06.054
Lenoble, R., Richheimer, S. L., Bailey, D. T., Mannila, C. G., and Nichol, R. L. (2002). Compositions Comprising Icariside I and Anhydroicaritin and Methods for Making the Same. US. Patent No US 6399579. Washington, DC: U.S. Patent and Trademark Office.
Li, L., Peng, L., Miao, J., Qiu, Y., Zhou, Y., Gao, X., et al. (2011). Icariin induces the expression of toll-like receptor 9 in Ana-1 murine macrophages. Phytother. Res. 25, 1732–1735. doi: 10.1002/ptr.3514
Li, Q., Huai, L., Zhang, C., Wang, C., Jia, Y., Chen, Y., et al. (2013a). Icaritin induces AML cell apoptosis via the MAPK/ERK and PI3K/AKT signal pathways. Int. J. Hematol. 97, 617–623. doi: 10.1007/s12185-013-1317-9
Li, S., Dong, P., Wang, J., Zhang, J., Gu, J., Wu, X., et al. (2010). Icariin, a natural flavonol glycoside, induces apoptosis in human hepatoma SMMC-7721 cells via a ROS/JNK-dependent mitochondrial pathway. Cancer Lett. 298, 222–230. doi: 10.1016/j.canlet.2010.07.009
Li, S., Priceman, S. J., Xin, H., Zhang, W., Deng, J., Liu, Y., et al. (2013b). Icaritin inhibits JAK/STAT3 signaling and growth of renal cell carcinoma. PLoS ONE 8:e81657. doi: 10.1371/journal.pone.0081657
Li, W., Wang, M., Wang, L., Ji, S., Zhang, J., and Zhang, C. (2014a). Icariin synergizes with arsenic trioxide to suppress human hepatocellular carcinoma. Cell Biochem. Biophys. 68, 427–436. doi: 10.1007/s12013-013-9724-3
Li, Z. J., Yao, C., Liu, S. F., Chen, L., Xi, Y. M., Zhang, W., et al. (2014b). Cytotoxic effect of icaritin and its mechanisms in inducing apoptosis in human Burkitt lymphoma cell line. Biomed. Res. Int. 2014:391512. doi: 10.1155/2014/391512
Liang, X. J., Chen, C., Zhao, Y., and Wang, P. C. (2010). Circumventing tumor resistance to chemotherapy by nanotechnology. Methods Mol. Biol. 596, 467–488. doi: 10.1007/978-1-60761-416-6_21
Lin, X., Li, W., and Xiao, P. (1999). Effects of icariside II from Epimedium koreanum on tumor cell lines in vitro. Pharm. Pharmacol. Commun. 5, 701–703. doi: 10.1211/146080899128734424
Liu, D. F., Li, Y. P., Ou, T. M., Huang, S. L., Gu, L. Q., Huang, M., et al. (2009). Synthesis and antimultidrug resistance evaluation of icariin and its derivatives. Bioorg. Med. Chem. Lett. 19, 4237–4240. doi: 10.1016/j.bmcl.2009.05.103
Liu, J., Ye, H., and Lou, Y. (2005). Determination of rat urinary metabolites of icariin in vivo and estrogenic activities of its metabolites on MCF-7 cells. Die Pharmazie 60, 120–125. Available online at: http://www.ingentaconnect.com/content/govi/pharmaz/2005/00000060/00000002/art00006
Liu, Y. Q., Yang, Q. X., Cheng, M. C., and Xiao, H. B. (2014). Synergistic inhibitory effect of Icariside II with Icaritin from Herba Epimedii on pre-osteoclastic RAW264.7 cell growth. Phytomedicine 15, 1633–1637. doi: 10.1016/j.phymed.2014.07.016
Ma, H., He, X., Yang, Y., Li, M., Hao, D., and Jia, Z. (2011). The genus Epimedium: an ethnopharmacological and phytochemical review. J. Ethnopharm. 134, 519–541. doi: 10.1016/j.jep.2011.01.001
Mao, H., Zhang, L., Wang, Y., and Li, X. (2000). Experimental studies of icariin on anticancer mechanism. Zhong Yao Cai 23, 554–556. Available online at: http://en.cnki.com.cn/Article_en/CJFDTOTAL-ZYCA200009021.htm
Marvel, D., and Gabriolovich, D. I. (2015). Myeloid-derived suppresor cells in the tumor microenvironment: expect the unexpected. J. Clin. Invest. 125, 3356–3364. doi: 10.1172/JCI80005
Ménard, C., Martin, F., Apetoh, L., Bouyer, F., and Ghiringhelli, F. (2008). Cancer chemotherapy: not only a direct cytotoxic effect, but also an adjuvant for antitumor immunity. Cancer Immunol. Immunother. 57, 1579–1587. doi: 10.1007/s00262-008-0505-6
Meng, K., Guo, Y., Wang, Y., Zhu, H., and He, Y. (2015). Application of Icaritin in Preparation of Medicines for Inhibiting Liver Cancer Stem Cells. CN. Patent No 105106196. Beijing: State Intellectual Property Office of the P.R.C.
Meng, K., Li, S., Ding, H., Guo, Y., Xu, Y., and Shen, Y. (2014). Application of Icaritin in Preparing Medicament for Treating Primary Liver Cancer. WO. Patent No 2014172857. Geneva: World Intellectual Property Organization.
Miura, Y., Oyama, M., Iguchi, K., Ito, T., Baba, M., Shikama, Y., et al. (2015). Anti-androgenic activity of icarisid II from Epimedium Herb in prostate cancer LNCaP cells. J. Nutr. Sci. Vitaminol. 61, 201–204. doi: 10.3177/jnsv.61.201
Mongiat, M., Ligresti, G., Marastoni, S., Lorenzon, E., Doliana, R., and Colombatti, A. (2007). Regulation of the extrinsic apoptotic pathway by the extracellular matrix glycoprotein EMILIN2. Mol. Cell. Biol. 27, 7176–7187. doi: 10.1128/MCB.00696-07
Nie, J., Luo, Y., Huang, X. N., Gong, Q. H., Wu, Q., and Shi, J. S. (2010). Icariin inhibits beta-amyloid peptide segment 25-35 induced expression of beta-secretase in rat hippocampus. Eur. J. Pharmacol. 626, 213–218. doi: 10.1016/j.ejphar.2009.09.039
Pan, X. W., Li, L., Huang, Y., Huang, H., Xu, D. F., Gao, Y., et al. (2016). Icaritin acts synergistically with epirubicin to suppress bladder cancer growth through inhibition of autophagy. Oncol. Rep. 35, 334–342. doi: 10.3892/or.2015.4335
Qi, J., and Qi, Y. (2002). An Anti-Cancer Composition, and Its Preparation Method. CN. Patent No 1380089. Beijing: State Intellectual Property Office of the P.R.C.
Qin, L., Han, T., Zhang, Q., Cao, D., Nian, H., Rahman, K., et al. (2008). Antiosteoporotic chemical constituents from Er-Xian Decoction, a traditional Chinese herbal formula. J. Ethnopharmacol. 118, 271–279. doi: 10.1016/j.jep.2008.04.009
Rau, K. M., Kang, H. Y., Cha, T. L., Miller, S. A., and Hung, M. C. (2005). The mechanisms and managements of hormone-therapy resistance in breast and prostate cancers. Endocr. Relat. Cancer 12, 511–532. doi: 10.1677/erc.1.01026
Sayyad, M., Tiang, N., Kumari, Y., Goh, B. H., Jaiswal, Y., Rosli, R., et al (in press). Acute toxicity profiling of the ethyl acetate fraction of Swietenia macrophylla seeds in-vitro neuroprotection studies. Saudi Pharm. J. doi: 10.1016/j.jsps.2016.05.002.
Schlegel, N., Burger, S., Golenhofen, N., Walter, U., Walter, D., Drenckhahn, D., et al. (2000). The role of VASP in regulation of cAMP- and Rac 1-mediated endothelial barrier stabilization. Am. J. Physiol. Cell Physiol. 294, C178–C188. doi: 10.1152/ajpcell.00273.2007
Shen, P., Wong, S. P., Li, J., and Yong, E. L. (2009). Simple and sensitive liquid chromatography-tandem mass spectrometry assay for simultaneous measurement of five Epimedium prenylflavonoids in rat sera. J. Chromatogr. B Analyt. Technol. Biomed. Life Sci. 877, 71–78. doi: 10.1016/j.jchromb.2008.11.030
Shi, D. B., Li, X. X., Zheng, H. T., Li, D. W., Cai, G. X., Peng, J. J., et al. (2014). Icariin-mediated inhibition of NF-κB activity enhances the in vitro and in vivo antitumour effect of 5-fluorouracil in colorectal cancer. Cell Biochem. Biophys. 69, 523–530. doi: 10.1007/s12013-014-9827-5
Song, J., Feng, L., Zhong, R., Xia, Z., Zhang, L., Cui, L., et al. (2016). Icariside II inhibits the EMT of NSCLC cells in inflammatory microenvironment via down-regulation of Akt-NF-κB signaling pathway. Mol. Carcinog. doi: 10.1002/mc.22471. [Epub ahead of print].
Song, J., Huang, H., Xia, Z., Wei, Y., Yao, N., Zhang, L., et al. (2015). TPGS/phospholipids mixed micelles for delivery of icariside II to multidrug-resistant breast cancer. Integr. Cancer. Ther. doi: 10.1177/1534735415596571. [Epub ahead of print].
Song, J., Shu, L., Zhang, Z., Tan, X., Sun, E., Jin, X., et al. (2012). Reactive oxygen species-mediated mitochondrial pathway is involved in Baohuoside I-induced apoptosis in human non-small cell lung cancer. Chem. Biol. Interact. 199, 9–17. doi: 10.1016/j.cbi.2012.05.005
Song, J., Zhong, R., Huang, H., Zhang, Z., Ding, D., Yan, H., et al. (2014). Combined treatment with Epimedium koreanum Nakai extract and gefitinib overcomes drug resistance caused by T790M mutation in non-small cell lung cancer cells. Nutr. Cancer 66, 682–689. doi: 10.1080/01635581.2014.895392
Sun, F., Indran, I. R., Zhang, Z. W., Tan, M. H., Li, Y., Lim, Z. L., et al. (2015a). A novel prostate cancer therapeutic strategy using icaritin-activated arylhydrocarbon-receptor to co-target androgen receptor and its splice variants. Carcinogenesis 36, 757–768. doi: 10.1093/carcin/bgv040
Sun, L., Chen, W., Qu, L., Wu, J., and Si, J. (2013). Icaritin reverses multidrug resistance of HepG2/ADR human hepatoma cells via downregulation of MDR1 and Pglycoprotein expression. Mol. Med. Rep. 8, 1883–1887. doi: 10.3892/mmr.2013.1742
Sun, L., Peng, Q., Qu, L., Gong, L., and Si, J. (2015b). Anticancer agent icaritin induces apoptosis through caspase-dependent pathways in human hepatocellular carcinoma cells. Mol. Med. Rep. 11, 3094–3100. doi: 10.3892/mmr.2014.3007
Supriady, H., Kamarudin, M. N. A., Chan, C. K., Goh, B. H., and Kadir, H. A. (2015). SMEAF attenuates the production of pro-inflammatory mediators through the inactivation of Akt-dependent NF-κB, p38 and ERK1/2 pathways in LPS-stimulated BV-2 microglial cells. J. Funct. Food. 17, 434–448. doi: 10.1016/j.jff.2015.05.042
Tan, H. L., Chan, K. G., Pusparajah, P., Lee, L. H., and Goh, B. H. (2016). Gynura procumbens: an overview of the biological activities. Front. Pharmacol. 7:52. doi: 10.3389/fphar.2016.00052
Tan, L. T. H., Lee, L. H., Yin, W. F., Chan, C. K., Kadir, H. A., Chan, K. G., et al. (2015). Traditional uses, phytochemistry, and bioactivities of Cananga odorata (Ylang-Ylang). Evid. Based Complement Alternat Med. 2015:896314. doi: 10.1155/2015/896314
Teng, S. (2010). Method for Manufacturing Anticancer Traditional Chinese Medicine Powder. CN. Patent No 101804175. Beijing: State Intellectual Property Office of the P.R.C.
Tian, W., Lei, H., Guan, R., Xu, Y., Li, H., Wang, L., et al. (2015). Icariside II ameliorates diabetic nephropathy in streptozotocin-induced diabetic rats. Drug Des. Devel. Ther. 9, 5147–5157. doi: 10.2147/DDDT.S90060
Tiong, C. T., Chen, C., Zhang, S. J., Li, J., Soshilov, A., Denison, M. S., et al. (2012). A novel prenylflavone restricts breast cancer cell growth through AhR-mediated destabilization of ERalpha protein. Carcinogenesis 33, 1089–1097. doi: 10.1093/carcin/bgs110
Tong, J. S., Zhang, Q. H., Huang, X., Fu, X. Q., Qi, S. T., Wang, Y. P., et al. (2011). Icaritin causes sustained ERK1/2 activation and induces apoptosis in human endometrial cancer cells. PLoS ONE 6:e16781. doi: 10.1371/journal.pone.0016781
Vinogradov, S., and Wei, X. (2012). Cancer stem cells and drug resistance: the potential of nanomedicine. Nanomedicine 7, 597–615. doi: 10.2217/nnm.12.22
Wang, C., Wu, P., Shi, J. -F., Jiang, Z. -H., and Wei, X. -Y. (2015a). Synthesis and cancer cell growth inhibitory activity of icaritin derivatives. Eur. J. Med. Chem. 100, 139–150. doi: 10.1016/j.ejmech.2015.06.006
Wang, J. (2003). A Natural Anticancer Preparation, and Its Preparation Method. CN. Patent No 1406626. Beijing: State Intellectual Property Office of the P.R.C.
Wang, L., Lu, A., Liu, X., Sang, M., Shan, B., Meng, F., et al. (2011b). The flavonoid Baohuoside-I inhibits cell growth and downregulates survivin and cyclin D1 expression in esophageal carcinoma via β-catenin-dependent signaling. Oncol. Rep. 26, 1149–1156. doi: 10.3892/or.2011.1400
Wang, Q., Hao, J., Pu, J., Zhao, L., Lü, Z., Hu, J., et al. (2011a). Icariin induces apoptosis in mouse MLTC-10 Leydig tumor cells through activation of the mitochondrial pathway and down-regulation of the expression of piwil4. Int. J. Oncol. 39, 973–980. doi: 10.3892/ijo.2011.1086
Wang, X. F., and Wang, J. (2014). Icaritin suppresses the proliferation of human osteosarcoma cells in vitro by increasing apoptosis and decreasing MMP expression. Acta. Pharmacol. Sin. 35, 531–539. doi: 10.1038/aps.2013.178
Wang, Y., Dong, H., Zhu, M., Ou, Y., Zhang, J., Luo, H., et al. (2010). Icariin exterts negative effects on human gastric cancer cell invasion and migration by vasodilator-stimulated phosphoprotein via Rac1 pathway. Eur. J. Pharmacol. 635, 40–48. doi: 10.1016/j.ejphar.2010.03.017
Wang, Y., Yu, P., Yi, P., Cheng, Q., and Ren, J. (2011c). Icariin Aglycon Derivatives and Their Preparation, Pharmaceutical Compositions and Use in Preventing and Treating Cancer and Protecting Myocardium. CN. Patent No 102167690. Beijing: State Intellectual Property Office of the P.R.C.
Wang, Z., Yang, L., Xia, Y., Guo, C., and Kong, L. (2015b). Icariin enhances cytotoxicity of doxorubicin in human multidrug-resistant osteosarcoma cells by inhibition of ABCB1 and down-regulation of the PI3K/Akt pathway. Biol. Pharm. Bull. 38, 277–284. doi: 10.1248/bpb.b14-00663
Wang, Z., Zhang, H., Dai, L., Song, T., Li, P., Liu, Y., et al. (2015c). Arsenic trioxide and icariin show synergistic anti-leukemic activity. Cell Biochem. Biophys. 73, 213–219. doi: 10.1007/s12013-015-0660-2
Wang, Z., Zhang, X., Wang, H. M., and Lou, Y. (2007). Neuroprotective effects of icaritin against beta amyloid-induced neurotoxicity in primary cultured rat neuronal cells via estrogen-dependent pathway. Neuroscience 30, 911–922. doi: 10.1016/j.neuroscience.2006.12.059
Wei, X., Wang, C., Wu, P., Jiang, Z., and Xu, L. (2015). Preparation of Icaritin Derivatives for Treating Breast Cancer or Lung Cancer. CN. Patent No 104610212. Beijing: State Intellectual Property Office of the P.R.C.
Wu, J., Guan, M., Wong, P. F., Yu, H., Dong, J., and Xu, J. (2012). Icariside II potentiates paclitaxel-induced apoptosis in human melanoma A375 cells by inhibiting TLR4 signaling pathway. Food Chem. Toxicol. 50, 3019–3024. doi: 10.1016/j.fct.2012.06.027
Wu, J., Song, T., Liu, S., Li, X., Li, G., and Xu, J. (2015a). Icariside II inhibits cell proliferation and induces cell cycle arrest through the ROS-p38-p53 signaling pathway in A375 human melanoma cells. Mol. Med. Rep. 11, 410–416. doi: 10.3892/mmr.2014.2701
Wu, J., Xu, J., Eksioglu, E. A., Chen, X., Zhou, J., Fortenbery, N., et al. (2013a). Icariside II induces apoptosis of melanoma cells through the downregulation of survival pathways. Nutr. Cancer 65, 110–117. doi: 10.1080/01635581.2013.741745
Wu, J., Zuo, F., Du, J., Wong, P. F., Qin, H., and Xu, J. (2013b). Icariside II induces apoptosis via inhibition of the EGFR pathways in A431 human epidermoid carcinoma cells. Mol. Med. Rep 8, 597–602. doi: 10.3892/mmr.2013.1557
Wu, T., Wang, S., Wu, J., Lin, Z., Sui, X., Xu, X., et al. (2015b). Icaritin induces lytic cytotoxicity in extranodal NK/T-cell lymphoma. J. Exp. Clin. Cancer Res. 34, 1–11. doi: 10.1186/s13046-015-0133-x
Xia, Q., Xu, D., Huang, Z., Liu, J., Wang, X., Wang, X., et al. (2010). Preparation of icariside II from icariin by enzymatic hydrolysis method. Fitoterapia 81, 437–442. doi: 10.1016/j.fitote.2009.12.006
Xu, B., Jiang, C., Han, H., Liu, H., Tang, M., Liu, L., et al. (2015). Icaritin inhibits the invasion and epithelial-to-mesenchymal transition of glioblastoma cells by targeting EMMPRIN via PTEN/AKt/HIF-1α signalling. Clin. Exp. Pharmacol. Physiol. 42, 1296–1307. doi: 10.1111/1440-1681.12488
Xu, C. Q., Liu, B. J., Wu, J. F., Xu, Y. C., Duan, X. H., Cao, Y. X., et al. (2010). Icariin attenuates LPS-induced acute inflammatory responses: involvement of PI3K/Akt and NF-kappaB signaling pathway. Eur. J. Pharmacol. 642, 146–153. doi: 10.1016/j.ejphar.2010.05.012
Xu, W., Zhang, Y., Yang, M., Shen, Z., Zhang, X., Zhang, W., et al. (2007). LC–MS/MS method for the simultaneous determination of icariin and its major metabolites in rat plasma. J. Pharm. Biomed. Ana. 45, 667–672. doi: 10.1016/j.jpba.2007.07.007
Yadav, R. K., Chae, S., Kim, H., and Chae, H. J. (2014). Endoplasmic reticulum stress and cancer. J. Cancer Prev. 19, 75–88. doi: 10.15430/JCP.2014.19.2.75
Yan, H., Song, J., Zhang, Z., and Jia, X. (2015). Optimization and anticancer activity in vitro and in vivo of baohuoside I incorporated into mixed micelles based on lecithin and Solutol HS 15. Drug Deliv. 8, 1–8. doi: 10.3109/10717544.2015.1120365
Yang, J. X., Fichtner, I., Becker, M., Lemm, M., and Wang, X. M. (2009). Anti-proliferative efficacy of icariin on HepG2 hepatoma and its possible mechanism of action. Am. J. Chin. Med. 37, 1153–1165. doi: 10.1142/S0192415X09007569
Yang, L., Wang, Y., Guo, H., and Guo, M. (2015). Synergistic anti-cancer effects of icariin and temozolomide in glioblastoma. Cell Biochem. Biophys. 71, 1379–1385. doi: 10.1007/s12013-014-0360-3
Yao, D., Xie, X. H., Wang, X. L., Wan, C., Lee, Y. W., Chen, S. H., et al. (2012). Icaritin, an exogenous phytomolecule, enhances osteogenesis but not angiogenesis—an in vitro efficacy study. PLoS ONE 7:e41264. doi: 10.1371/journal.pone.0041264
Ye, Y., Hu, F., Zou, J., Zhan, Y., Wang, S., and Liu, J. (2015). In vivo and in vitro inhibitory effects of icariin on angiogenesis. Zhongguo Yi Xue Ke Xue Yuan Xue Bao 37, 264–268. doi: 10.3881/j.issn.1000-503X.2015.03.003
Yu, X., Tong, Y., Han, X. Q., Kwok, H. F., Yue, G. G., Lau, C. B., et al. (2013). Anti-angiogenic activity of Herba Epimedii on zebrafish embryos in vivo and HUVECs in vitro. Phytother. Res. 27, 1368–1375. doi: 10.1002/ptr.4881
Zhang, D. A. (2014). Suppresive effect of icaritin on angiogenesis and its mechanisms. J. Int. Transl. Med. 2, 385–388. doi: 10.11910/2227-6394.2014.02.03.07
Zhang, D. C., Liu, J. L., Ding, Y. B., Xia, J. G., and Chen, G. Y. (2013). Icariin potentiates the antitumor activity of gemcitabine in gallbladder cancer by suppressing NF-κB. Acta Pharmacol. Sin. 34, 301–308. doi: 10.1038/aps.2012.162
Zhang, N., and Calderwood, S. K. (2008). Icaritin and Desmethylicaritin As Anti-Cancer Agents. US. Patent No 20080214844. Washington, DC: U.S. Patent and Trademark Office.
Zhang, S. (1991). Chinese Herbal Composition with Anticancer Effect, and Preparation Method Thereof. CN. Patent No 1056814. Beijing: State Intellectual Property Office of the P.R.C.
Zhang, Y., Wei, Y., Zhu, Z., Gong, W., Liu, X., Hou, Q., et al. (2014). Icariin enhances radiosensitivity of colorectal cancer cells by suppressing NF-κB activity. Cell Biochem. Biophys. 69, 303–310. doi: 10.1007/s12013-013-9799-x
Zhao, H., Guo, Y., Li, S., Han, R., Ying, J., Zhu, H., et al. (2015). A novel anti-cancer agent Icaritin suppresses hepatocellular carcinoma initiation and malignant growth through the IL-6/Jak2/Stat3 pathway. Oncotarget 6, 31927–31943. doi: 10.1093/annonc/mdv523.86
Zhao, Y., Xiao, X., Wang, G., Chen, Z., and Zheng, Q. (2014). Pharmaceutical Composition Containing Juglanin B and Icaritin and Its Application for Treating Hepatocellular Carcinoma. CN. Patent No 104147025. Beijing: State Intellectual Property Office of the P.R.C.
Zheng, Q., Liu, W. W., Li, B., Chen, H. J., Zhu, W. S., Yang, G. X., et al. (2014). Anticancer effect of icaritin on human lung cancer cells through inducing S phase cell cycle arrest and apoptosis. J. Huazhong Univ. Sci. Technol. Med. Sci. 34, 497–503. doi: 10.1007/s11596-014-1305-1
Zhou, J., Wu, J., Chen, X., Fortenberry, N., Eksioglu, E., Kodumudi, K. N., et al. (2011). Icariin and its derivative, ICT, exert anti-inflammatory, anti-tumor effects and modulate myeloid derived suppressive cells (MDSCs) functions. Int. Immunopharmacol. 11, 890–898. doi: 10.1016/j.intimp.2011.01.007
Zhu, J. F., Li, Z. J., Zhang, G. S., Meng, K., Kuang, W. Y., Li, J., et al. (2011). Icaritin shows potent anti-leukemia activity on chronic myeloid leukemia in vitro and in vivo by regulating MAPK/ERK/JNK and JAK2/STAT3/AKT signalings. PLoS ONE 6:e23720. doi: 10.1371/journal.pone.0023720
Keywords: icariin, icaritin, icariside II, Yin Yang Huo, anti-cancer, ethnopharmacology
Citation: Tan H-L, Chan K-G, Pusparajah P, Saokaew S, Duangjai A, Lee L-H and Goh B-H (2016) Anti-Cancer Properties of the Naturally Occurring Aphrodisiacs: Icariin and Its Derivatives. Front. Pharmacol. 7:191. doi: 10.3389/fphar.2016.00191
Received: 01 March 2016; Accepted: 16 June 2016;
Published: 29 June 2016.
Edited by:
Lyndy Joy McGaw, University of Pretoria, South AfricaReviewed by:
Fang-Rong Chang, Kaohsiung Medical University, TaiwanAmit Kumar Tyagi, The University of Texas MD Anderson Cancer Center, USA
Copyright © 2016 Tan, Chan, Pusparajah, Saokaew, Duangjai, Lee and Goh. This is an open-access article distributed under the terms of the Creative Commons Attribution License (CC BY). The use, distribution or reproduction in other forums is permitted, provided the original author(s) or licensor are credited and that the original publication in this journal is cited, in accordance with accepted academic practice. No use, distribution or reproduction is permitted which does not comply with these terms.
*Correspondence: Learn-Han Lee, bGVlLmxlYXJuLmhhbkBtb25hc2guZWR1;
Bey-Hing Goh, Z29oLmJleS5oaW5nQG1vbmFzaC5lZHU=