- 1Department of Cell Physiology, Ruhr University Bochum, Bochum, Germany
- 2Department of Biophysics, Ruhr University Bochum, Bochum, Germany
- 3Department of Biophysics, Key Laboratory of Computational Biology, CAS-MPG Partner Institute for Computational Biology, Shanghai Institutes for Biological Sciences, Chinese Academy of Sciences, Shanghai, China
Histamine is a neurotransmitter involved in a number of physiological and neuronal functions. In mammals, such as humans, and rodents, the histaminergic neurons found in the tuberomamillary nucleus project widely throughout the central nervous system. Histamine acts as positive modulator of GABAA receptors (GABAARs) and, in high concentrations (10 mM), as negative modulator of the strychnine-sensitive glycine receptor. However, the exact molecular mechanisms by which histamine acts on GABAARs are unknown. In our study, we aimed to identify amino acids potentially involved in the modulatory effect of histamine on GABAARs. We expressed GABAARs with 12 different point mutations in Xenopus laevis oocytes and characterized the effect of histamine on GABA-induced currents using the two-electrode voltage clamp technique. Our data demonstrate that the amino acid residues β2(N265) and β2(M286), which are important for modulation by propofol, are not involved in the action of histamine. However, we found that histamine modulation is dependent on the amino acid residues α1(R120), β2(Y157), β2(D163), β3(V175), and β3(Q185). We showed that the amino acid residues β2(Y157) and β3(Q185) mediate the positive modulatory effect of histamine on GABA-induced currents, whereas α1(R120) and β2(D163) form a potential histamine interaction site in GABAARs.
Introduction
The GABAA receptor (GABAAR) is the most important inhibitory receptor channel complex in the central nervous system (CNS). It is a pentameric ligand-gated ion channel, which is activated by γ-aminobutyric acid (GABA) and is modulated by various compounds. GABAAR modulators include benzodiazepines, barbiturates, propofol, ethanol, neurosteroids, cations (e.g., zinc ions), herbal components and odorants as well as the neurotransmitter histamine (Bureau and Olsen, 1991; McDonald and Olsen, 1994; Thompson et al., 1996; Grobin et al., 1998; Hosie et al., 2003; Kim and McDonald, 2003; Belelli and Lambert, 2005; Li et al., 2006; Saras et al., 2008; Sergeeva et al., 2010; Rudolph and Knoflach, 2011; Kletke et al., 2013; Yip et al., 2013). Since 2008, it has been known that histamine could directly activate homomeric GABAARs composed of β subunits and modulate the heteromeric GABAARs α1β2 and α1β2γ2 (Saras et al., 2008). The modulatory effects of histamine on heteromeric GABAARs depend on the subunit composition of the receptors (Bianchi et al., 2011). Today, 19 subunits are known (Simon et al., 2004). These subunits are α1–6, β1–3, γ1–3, δ, ε, 𝜃, π, and ρ1–3. Bianchi et al. (2011) showed α subunit preference of histamine under low GABA concentrations.
Histamine is a neurotransmitter in the brain and a cytokine in the periphery. It is produced and released by mast cells, basophiles, enterochromaffin-like cells, and neurons (Haas et al., 2008). In the brain histamine is released from the tuberomammillary nucleus which is involved in the wake-sleep regulation. Metabotropic histamine receptors (H1–H4) mediate the physiological functions of histamine but at least three different types of neuronal ligand-gated ion channels are modulated by histamine (Bekkers, 1993; Saras et al., 2008; Bianchi et al., 2011; Kletke et al., 2013). The possible mechanism of the modulation of GABAARs in the brain is still speculative (Saras et al., 2008; Bianchi et al., 2011).
Currently, the interaction site for histamine on the GABAAR is unknown. There are many known modulation sites on the GABAARs for various compounds. These sites include the amino acids β2(N265) and β2(M286). These two amino acid residues are involved in the propofol and etomidate interaction with the GABAARs (Krasowski et al., 2001; Siegwart et al., 2002; Jurd et al., 2003; Reynolds et al., 2003). These amino acid residues represent some of the different modulation mechanisms of the receptor. Propofol and etomidate are positive modulators of the GABAARs. In addition to these described modulation sites, there are other identified sites of functional relevance. These sites are, e.g., the GABA-binding site, which involves the amino acid residue F64 of the α subunit and the amino acid residues Y157 and Y205 of the β subunits (Sigel et al., 1992; Amin and Weiss, 1993). Recent studies with mutated amino acids V175, Q185, and D163 of the β subunit and R120 of the α subunit, which are components of loop nine or are involved in a salt-bridge interaction, show that these amino acids are involved in stabilizing the closed state or involved in a state-dependent salt-bridge of the GABAARs (Williams et al., 2010; Laha and Wagner, 2011).
While binding sites on the GABAARs have already been described for some allosteric modulators, the molecular basis of positive allosteric receptor modulation by histamine is unknown. Therefore, we plan to identify the amino acids that are involved in the modulatory effect of histamine at the GABAARs. For that purpose, we investigated site-directed mutated GABAARs expressed in a Xenopus laevis expression system using the two-electrode voltage clamp technique, and analyzed the structural impact of the chosen amino acids by creating a homology model of a 2x α1 – 3x β3 GABAARs heteropentamer.
Materials and Methods
GABAAR cDNA
Expression plasmids based on the pSGEM vector (courtesy of M. Hollmann, Bochum, Germany) for rat α1, β2, mouse γ2L, and human β3 were described by Saras et al. (2008). Rat α2 cDNA was kindly provided by ImaGenes, Berlin, Germany, and subcloned into pSGEM by using PCR and standard molecular biology methods.
The point mutations were made using overlap-extension PCRs, as described by Heckman and Pease (2007). For overlap-extension PCRs, the plasmids for α1, β2, and β3 subunits were used as templates. Overlap-extension PCR was performed using a mixture of taq-DNA- and pwo-DNA-polymerase (20:1) (Biotherm) in a volume of 50 μl with 20 pmol of each primer (Supplementary Table S3). The following temperature cycle profile was used: 5 min at 95°C; followed by 30 cycles of 45 s at 95°C, 45 s at 60°C, 60 s at 72°C (120 s for the fusion PCR); and a final extension of 10 min at 72°C. The mutated DNA was subcloned into pSGEM using standard molecular biology methods.
Expression of Receptor cRNA in Xenopus laevis oocytes
The cRNAs of wt as well as point mutated GABAAR subunit cRNAs were synthesized by using the AmpliCap T7 high yield message maker kit (Epicentre, Madison, WI, USA) as described by Sergeeva et al. (2010). The oocytes were prepared from Xenopus laevis using standard methods. Five to twenty nanograms of cRNA was injected, 24 h after surgery, into one stage V–VI oocyte. The incubation took place at 12–16°C in ND96 [96 mM NaCl, 2 mM KCl, 1.8 mM CaCl2, 1 mM MgCl2, 5 mM HEPES, pH 7.2; 100 U/ml penicillin, 100 U/ml streptomycin from Antibiotic Antimycotic Solution (100x)] (Sigma–Aldrich, St. Louis, MO, USA). After 2–4 days, the oocytes were measured by two-electrode voltage clamp, as briefly described by Sergeeva et al. (2010). Each of the tested substances was diluted with Frog-Ringer’s solution (115 mM NaCl, 2.5 mM KCl, 1.8 mM CaCl2, 10 mM HEPES, pH 7.2). The agonists were dissolved in ND96 and applied in a volume of 200 μl into the entrance tube of the recording chamber, totally exchanging the bath solution within a second. Therefore, due to the relative slow desensitization kinetics in our investigated GABA receptors and the fact that we compare the same receptor ± histamine, desensitization will not interfere with the determination of the histamine potentiation. The pH of GABA- or histamine-containing solutions was monitored to ensure a pH of 7.2 for all histamine concentrations. All measurements were taken with a membrane potential of -40 mV.
Analysis and Statistics
During the measurements, the currents were recorded using CellWorks software and were analyzed using pCLAMP 10 software. The statistical evaluation and curve fitting (3-parameter Hill equation) was performed using SigmaPlot V8.0 (Systat Software, San Jose, CA, USA). All mean values are ± SEM, and the significant data were marked with ∗p ≤ 0.05, ∗∗p ≤ 0.01, and ∗∗∗p ≤ 0.001.
GABAAR Homology Modeling and Histamine Docking
The 2.97 Å crystal structure of the GABA β3 homopentamer (Miller and Aricescu, 2014) was used as basis for homology modeling. We carried out a sequence alignment of the α1 sequence (Uniprot entry P14867, The UniProt Consortium, 2013) and the β3 subunit sequences from the crystal structure with MUSCLE (Edgar, 2004; default settings). The resulting alignment showed 35.3% sequence identity and 61.9% sequence similarity and is included in the Supplementary Material. As we know from GPCR modeling, an identity >35% is a good basis for homology modeling of transmembrane proteins (Wolf et al., 2008; Kufareva et al., 2011). Based on this alignment, we created and optimized a model of an α1 subunit homopentamer with the SWISS-MODEL server (Biasini et al., 2014), based on the structure of the β3 homopentamer from the crystal structure mentioned above. We then created a 2x α1 – 3x β3 GABAAR heteropentamer by substituting two β3 subunits in the crystal structure (chain A and C) by the respective α1 subunits in PyMol (Schrödinger, 2010). We then carried out a WHATIF protein check, showing that the model is reliable. The checkfile can be found in the Supplementary Material. Binding site analysis was carried out with TRIDOCK (te Heesen et al., 2007). In short, TRIDOCK searches for putative binding positions for small organic molecules following Congreve’s rule-of-three (Congreve et al., 2003) and marks these positions with a “bead.” Docking calculations on histamine binding were carried out with Autodock Vina (Trott and Olson, 2010), using a 20 × 20 × 20 Å box (grid spacing 1 Å) centered between Asp163(β) and R120(α), and an exhaustiveness parameter of 80 (Schneider et al., 2011). A histamine topology was obtained from the PRODRG server (Schuttelkopf and van Aalten, 2004). Polar atoms and Kollman charges were added to the heteropentameric protein, while histamine was docked with Gasteiger charges.
Results
Screening of Mutated GABAARs for Altered Histamine Potentiation
Saras et al. (2008) showed that homomeric β2 or β3 receptors were activated by histamine in a recombinant expression system. Our intention was to analyze the effect of histamine on point mutated GABAAR β2/3 subunits. First, we generated mutations in β2/3 subunits. In contrast to the wild-type (wt) subunits, functional expression of mutated GABAARs as homomeric receptors failed in most instances so that a systematic screening of homomeric receptors was not possible. Therefore, we had to determine the impact of mutated amino acids in heteromeric receptors by analyzing the potentiating effect of histamine on the GABA-induced currents. We were mostly interested in the effect of histamine on the two most important synaptic GABAARs of the CNS composed of α1β2γ2L and α2β3γ2L subunits (Pirker et al., 2000; Nutt, 2006; Olsen and Sieghart, 2008).
To screen the modulating effect of histamine, we investigated the action of 3 mM histamine on currents evoked by GABA (typically EC10-30). To determine suitable GABA concentrations for screening experiments, the EC50 values of GABA for all of the investigated subunit combinations were determined (Supplementary Table S1). In addition, we tested histamine concentrations up to 10 mM to demonstrate the absence of homomeric GABAARs composed of β subunits. For all of our analyzed GABAARs, histamine alone did not induce any currents (data not shown).
First, we analyzed the wt GABAARs α1β2γ2L, α1β3γ2L, and α2β3γ2L, to demonstrate a robust and reproducible potentiation of GABA-induced currents by histamine under our experimental conditions. This analysis also served as control for our experiments with the point mutated GABAARs. With our experiments, we could show, as Saras et al. (2008) had previously, that histamine could increase the GABA-induced current of wt receptors in a dose-dependent manner. Three millimolar histamine significantly potentiated the GABA-induced currents of GABAARs α1β2γ2L by approximately 1.4-fold, α1β3γ2L by approximately 0.6-fold and α2β3γ2L by approximately 0.7-fold (Figure 1).
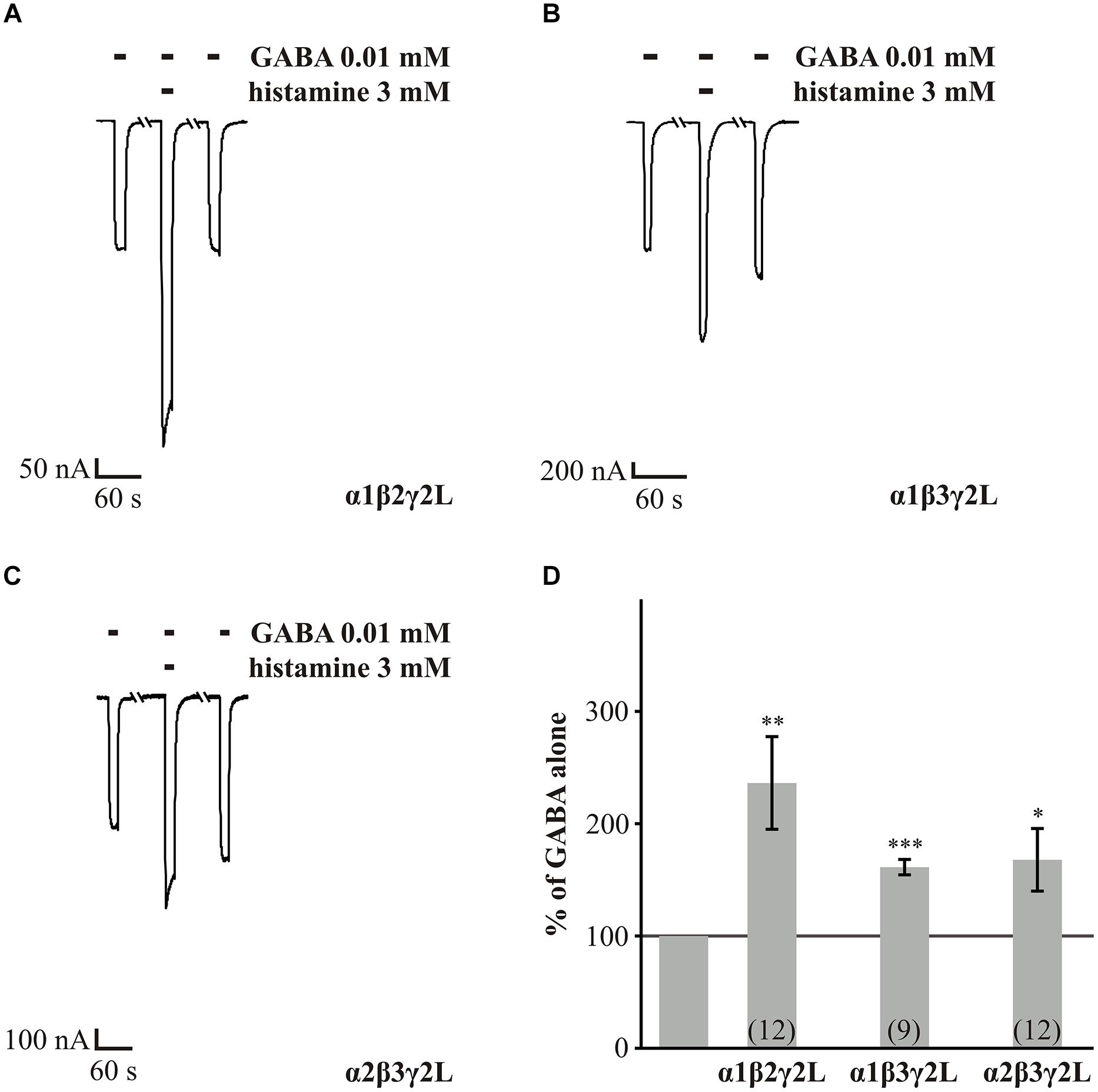
FIGURE 1. GABA potentiated by histamine in wt receptors. GABA-induced (EC10-30) currents are potentiated by 3 mM histamine. (A–C) Xenopus oocytes expressing the abg isoforms: α1β2γ2L, α1β3γ2L, and α2β3γ2L were voltage clamped, and GABA at a concentration of 10 μM was bath-applied with or without 3 mM histamine. (D) Average potentiation of GABA-induced currents calculated from measurements as shown in (A–C). Bars two to four show the mean enhancement ± SEM, as a percentage of the amplitude of GABA alone (gray line), for the αβγ isoforms: α1β2γ2L, α1β3γ2L, and α2β3γ2L, (n = 9–12). Significant data were marked with ∗p ≤ 0.05, ∗∗p ≤ 0.01, and ∗∗∗p ≤ 0.001.
Next, we screened all point mutated GABAARs using the same conditions: a GABA concentration of typical EC10-30 and a histamine concentration of 3 mM. The first amino acids to be analyzed for their action in the histamine modulation of the GABAAR were the point mutants β(N265M) and β(M286W). These amino acids are involved in the action of etomidate and propofol on the GABAARs (Krasowski et al., 2001; Siegwart et al., 2002; Jurd et al., 2003; Reynolds et al., 2003). Our experiments showed that the GABA-induced currents (10 μM GABA) on GABAARs that contained the point mutated subunits β(N265M) or β(M286W) were increased by histamine (Figure 2). However, the histamine modulation of the mutated receptors was not significantly different from the modulation of the wt GABAARs α1β2γ2L. Therefore, these mutations do not influence histamine modulation.
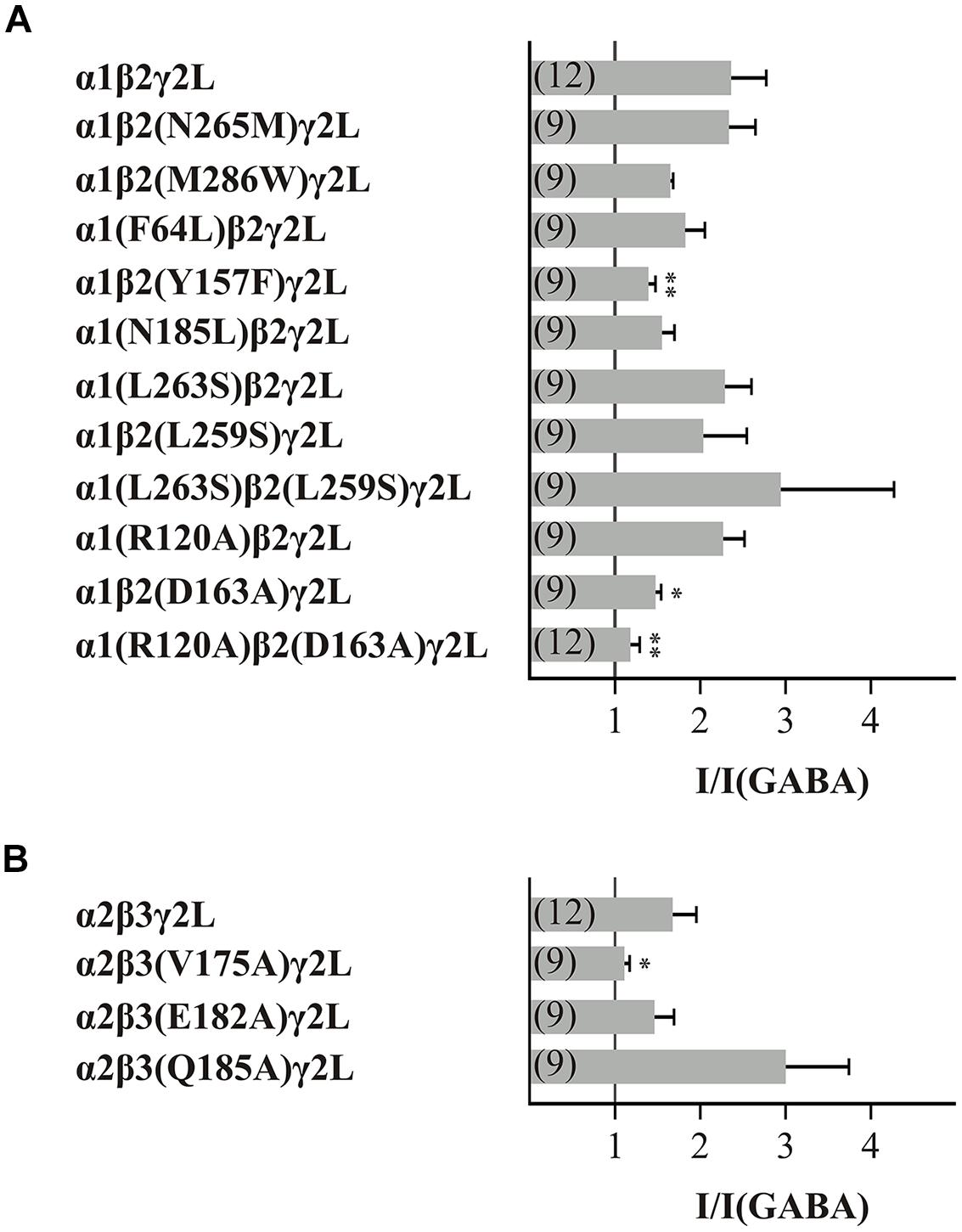
FIGURE 2. Effect of 3 mM histamine on the GABA-induced current of wt or mutated GABAARs. The bars show the increase of the GABA-induced (typically EC10-30) currents by 3 mM histamine of the GABAAR subunit combination (A) α1β2γ2L and (B) α2β3γ2L. Significant data were marked with ∗p ≤ 0.05 and ∗∗p ≤ 0.01.
The next 10 amino acids that we analyzed are important for binding GABA, for forming a state-dependent salt-bridge or for stabilizing the closed state of the GABAARs (Amin and Weiss, 1993; Williams et al., 2010; Laha and Wagner, 2011). Furthermore, we were interested in the influence of the conserved leucine in the pore-forming TM2 segment that is involved in the gating-mechanism of the GABAARs (Chang and Weiss, 1999). Because this amino acid, β2(L259), is also present in α subunits, we investigated point mutations of both a and β subunits. Receptors with the mutations β2(Y157F), β2(D163A), β3(V175A), and the combination of α1(R120A) with β2(D163A) showed significantly reduced potentiation of the GABA-induced current by histamine (Figure 2).
It can be suggested that the amino acids Y157, D163, and V175 of the β subunit and the amino acid R120 of the α subunit are involved in the action of histamine. The analyzed GABAAR α2β3(Q185A)γ2L showed a stronger potentiation than the wt demonstrating that the amino acid Q185 also potentially affects histamine action on GABAARs.
Dose-Dependent Action of Histamine on Mutated GABAARs
To investigate the impact of the identified amino acids on histamine modulation in more detail, we next analyzed the effect of different histamine concentrations (0.1 to 10 mM) on the GABA-induced current (typically EC10-30).
The first amino acid residue we analyzed was Y157 of the β subunit. This amino acid residue is involved in the conformational changes in the GABAARs induced by GABA (Amin and Weiss, 1993). GABAAR α1β2(Y157F)γ2L showed reduced potentiation of the GABA-induced current by 3 mM histamine (Figure 2A) in comparison to the wt α1β2γ2L receptor. Histamine concentrations of 0.1 to 10 mM potentiated the GABA-induced current in a dose-dependent manner (Figures 3A,B). However, for all of the tested concentrations, the potentiating effect was smaller than the effect observed with the wt receptor (Figures 3B,C, and Supplementary Table S2). To analyze the influence of the γ2L subunit, we co-expressed the subunits α1 and β2(Y157F). Histamine caused a dose-dependent potentiation of the mutated GABAAR (Figures 3D,E) that was not significantly different from the wt α1β2 receptor (Figures 3E,F, and Supplementary Table S2). Interestingly, the influence of the point mutations is dependent on the presence of the γ2L subunit. These results show that Y157 is not essential for potentiation by histamine.
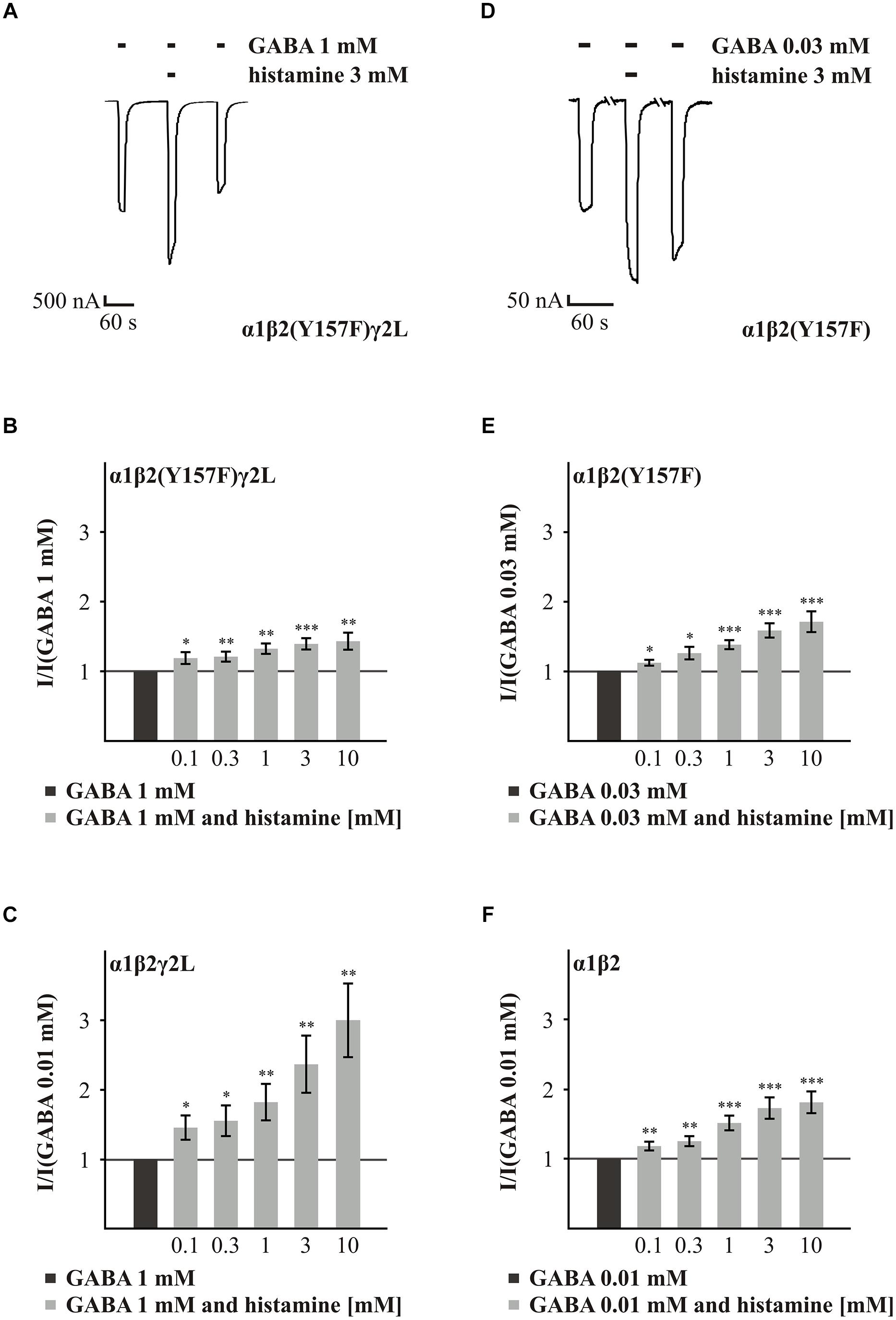
FIGURE 3. Histamine action on GABA-induced currents of GABAARs with the point mutated β2(Y157F) subunit. (A,D) Original traces of the histamine (3 mM) action on the GABA-induced currents of the GABAARs α1β2(Y157F)γ2L and α1β2(Y157F). (B,C,E,F) Bar diagrams show the action of various histamine concentrations on the GABA-induced currents of the GABAARs α1β2(Y157F)γ2L, α1β2(Y157F), α1β2γ2L, and α1β2. Significant data were marked with ∗p ≤ 0.05, ∗∗p ≤ 0.01, and ∗∗∗p ≤ 0.001.
Next, we analyzed the effect of histamine on the amino acids α1(R120) and β2(D163), which form a state-dependent salt-bridge of the GABAARs (Laha and Wagner, 2011). We investigated the ability of different histamine concentrations (0.1 to 10 mM) to potentiate the GABA response (typically EC10-30) on the GABAARs α1(R120A)β2γ2L, α1β2(D163A)γ2L, and α1(R120A)β2(D163A)γ2L (Figure 4). Histamine shows a dose-dependent potentiation on the GABAARs α1(R120A)β2γ2L and α1β2(D163A)γ2L (Figures 4A,B,D,E). However, at a receptor composed of both mutated subunits in combination with the γ2L subunit, the increasing effect of histamine is nearly abolished (Figures 4C,F). In comparison to wt, the mutation α1(R120A) alone had no significant effect on histamine potentiation (Supplementary Table S2). At GABAARs α1β2(D163A)γ2L and GABAAR α1(R120A)β2(D163A)γ2L (Figure 3C), histamine potentiation was significantly less than at the wt for most concentrations tested (Supplementary Table S2). To ensure that this effect is specific to histamine and does not abolish potentiation in general, we tested the potentiation of the GABA-induced currents by 10 μM propofol (Yip et al., 2013), which was able to potentiate the GABA-induced current (data not shown). To verify the influence of the γ2L subunit, we attempted to express the subunit combinations α1(R120A)β2, α1β2(D163A), and α1(R120A)β2(D163A), but none of these combinations produced a functional receptor.
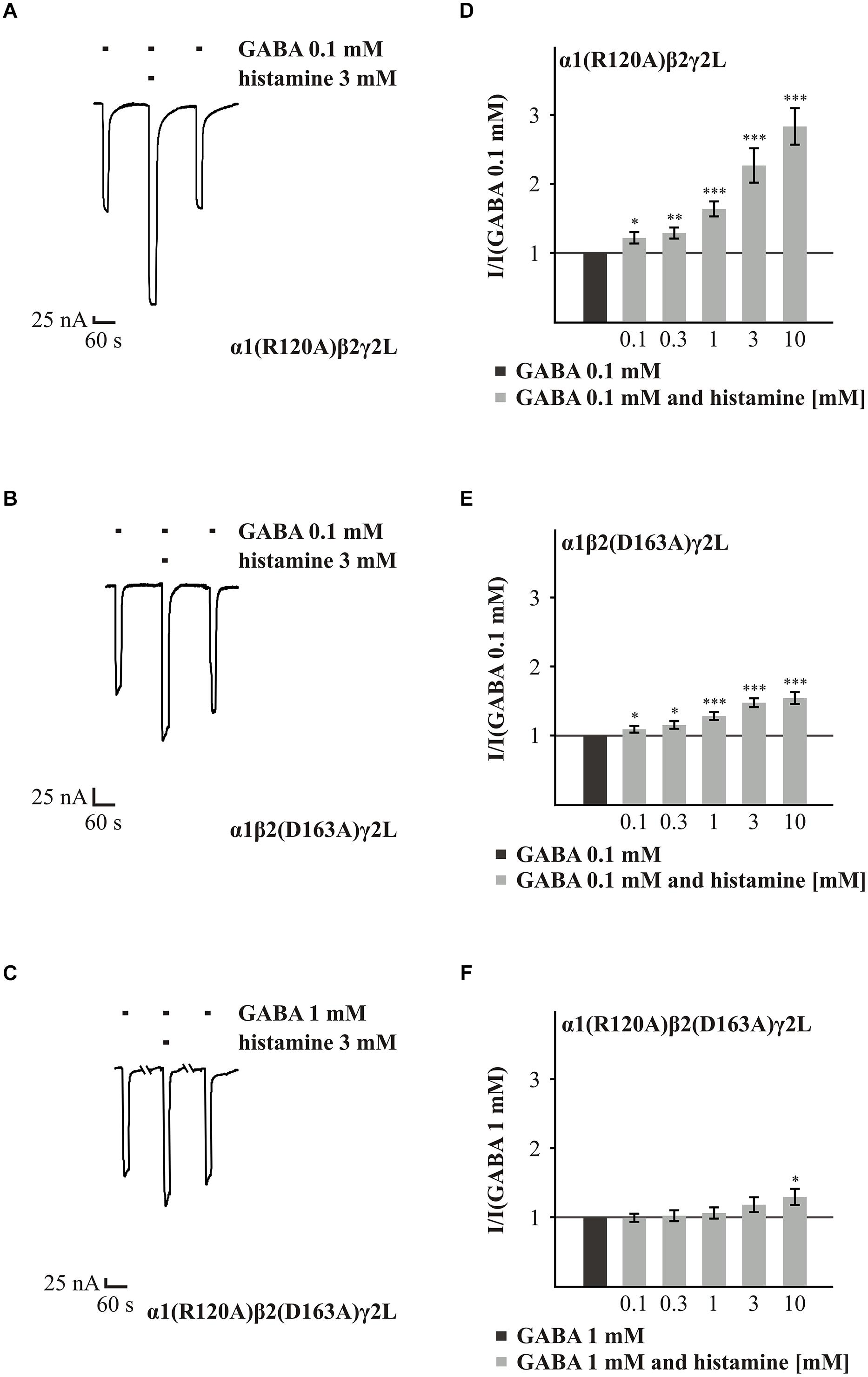
FIGURE 4. Histamine action on GABA-induced currents of GABAARs with point mutated subunits α1(R120A) and β2(D163A). (A–C) Original traces of the modulatory effect of 3 mM histamine on GABA-induced currents from GABAARs α1(R120A)β2γ2L, α1β2(D163A)γ2L, and α1(R120A)β2(D163A)γ2L. (D–F) Bar diagrams show the effect of 0.1 to 10 mM histamine on the GABA-induced (typically EC10-30) current. Significant data were marked with ∗p ≤ 0.05, ∗∗p ≤ 0.01, and ∗∗∗p ≤ 0.001.
The last two amino acids that we analyzed were V175 and Q185 on the β subunit. These amino acids are located in loop 9, which is in close proximity to the salt-bridge formed by α1(R120A) and β2(D163A). The experiments with the GABAAR α2β3(V175A)γ2L showed that the GABA-induced current was not potentiated by histamine concentrations up to 3 mM (0.1 to 3 mM; Figure 5). Only the highest concentration, 10 mM, significantly changed the GABA-induced (EC16) current, increasing it 1.4-fold (Figure 5B). In comparison to the wt (Figure 6C), the potentiation effected by histamine is drastically reduced. This suggests that V175 is involved in the action of histamine on the GABAARs. To demonstrate that this effect is not dependent on the presence of the α2 subunit, we tested the α1β3(V175A)γ2L combination and also found a significant reduction in potentiation (Supplementary Figure S1). To ensure that the loss of potentiation is specific for histamine, we tested this mutated receptor with 10 μM propofol, which caused a 1.8-fold potentiation (data not show) demonstrating that GABA-induced currents at this receptor can be potentiated by other modulators.
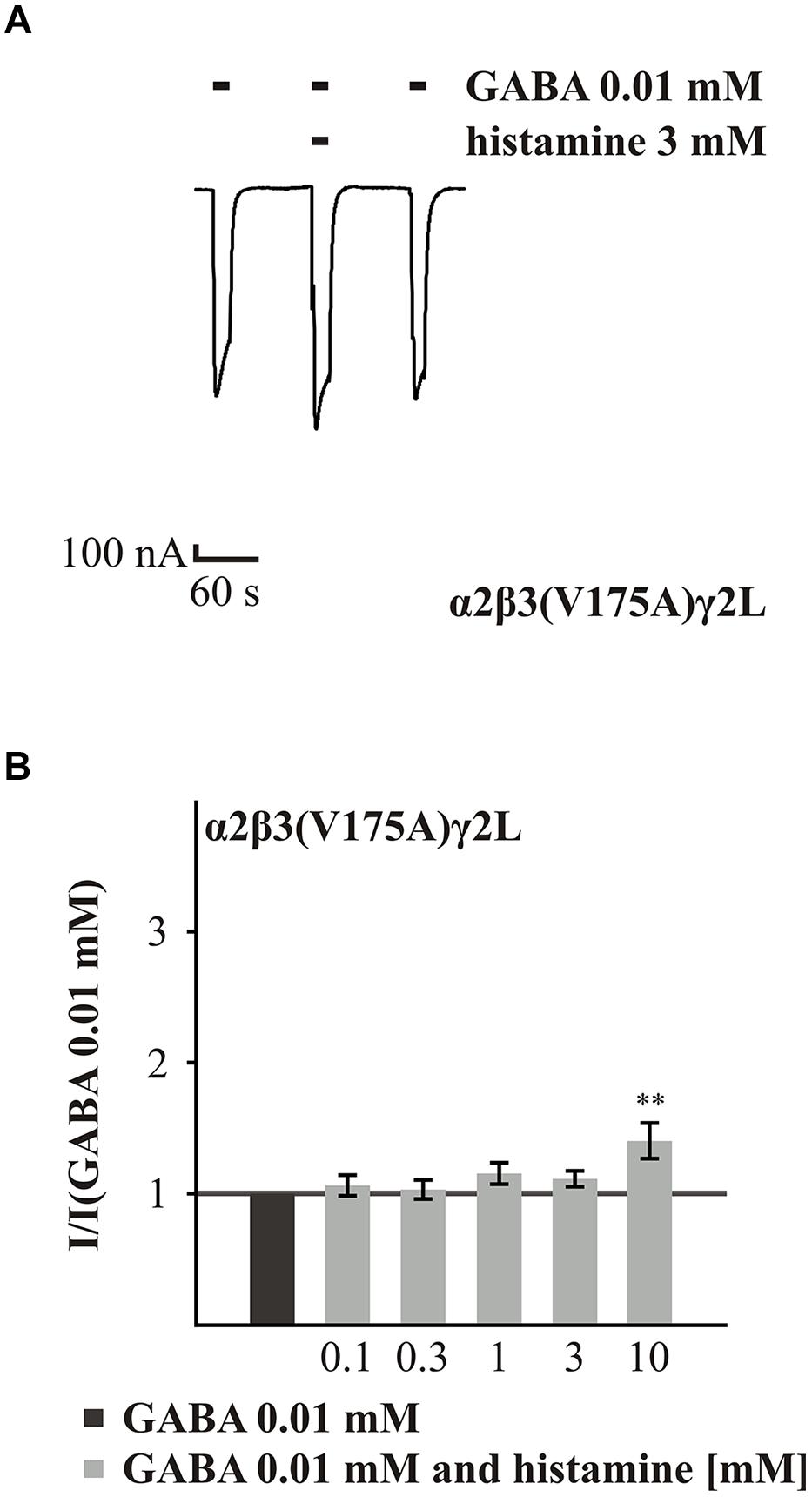
FIGURE 5. Histamine action on the GABA-induced currents of GABAARs with point mutated β3(V175A). (A) Original trace of the effect of 3 mM histamine on the GABA-induced currents of the GABAAR α2β3(V175A)γ2L. (B) Bar diagram show the effect of various histamine concentrations up to 10 mM on the GABA-induced currents of the GABAAR α2β3(V175A)γ2L. Significant data were marked with ∗∗p ≤ 0.01.
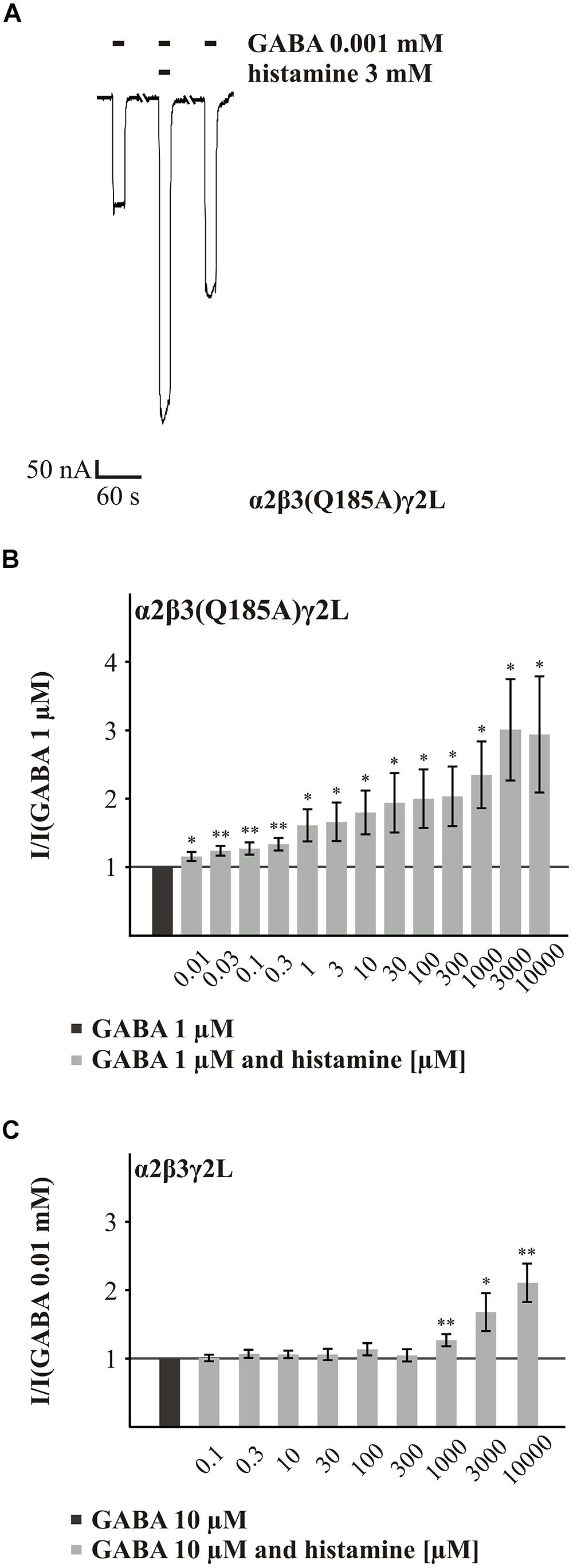
FIGURE 6. Histamine action on the GABA-induced currents of GABAARs with the point mutated β3(Q185A) subunit. (A) Original trace of the histamine (3 mM) action on the GABA-induced (EC11) currents of the GABAAR α2β3(Q185A)γ2L. (B,C) Bar diagrams that show the action of different histamine concentrations on the GABA-induced currents of the GABAARs α2β3(Q185A)γ2L and α2β3γ2L. Significant data were marked with ∗p ≤ 0.05 and ∗∗p ≤ 0.01.
In the initial screening, the potentiating effect of histamine appeared to be increased at GABAAR α2β3(Q185A)γ2L. The investigation of various histamine concentrations (0.01 to 10 mM) revealed a dose-dependent potentiation of the GABA (EC11) response (Figures 6A,B). In comparison to the wt receptor (Figure 6C), where the first significant potentiation was observed at 1 μM histamine, concentrations as low as 10 μM histamine produced significant potentiation at the α2β3(Q185A)γ2L receptor. For most concentrations up to 1 mM, histamine is a significantly better potentiator at the mutated receptor (Supplementary Table S2). At higher concentrations, the effect is no longer significant, which is an indication that the potency but not the efficacy of histamine is enhanced by this mutation. To demonstrate that this effect is not dependent on the presence of the α2 subunit, we tested the α1β3(Q185A)γ2L subunit combination and also found a significant increase in potentiation (Supplementary Figure S1).
Discussion
Our study confirms the recent finding (Saras et al., 2008; Bianchi et al., 2011) that there is a robust modulatory effect of histamine on GABAARs with different subunit combinations. The screening of point mutated GABAAR subunits revealed that most mutations, which encompass several amino acids conserved in β subunits, did not influence histamine potentiation. Our results demonstrate that the point mutations N265M and M286W of amino acids in the β subunit that are important for the modulation by etomidate and propofol (Siegwart et al., 2002; Jurd et al., 2003; Reynolds et al., 2003) had no effect on the modulation of the GABA-induced current by histamine. Our results indicate that histamine does not act through these known modulation mechanisms.
In our work, we identified amino acid residues important for the modulatory action of histamine on GABAARs. Four amino acid residues are located on the β subunit, consistent with the idea proposed by Saras et al. (2008) that the β subunit is involved in the action of histamine at the GABAARs. Most recently, the crystal structure of the human ß3 homopentameric receptor was resolved (Miller and Aricescu, 2014). To assess the potential function of the mutated amino acids, we build a homology model of an α1/β3 heteropentamer (Figure 7).
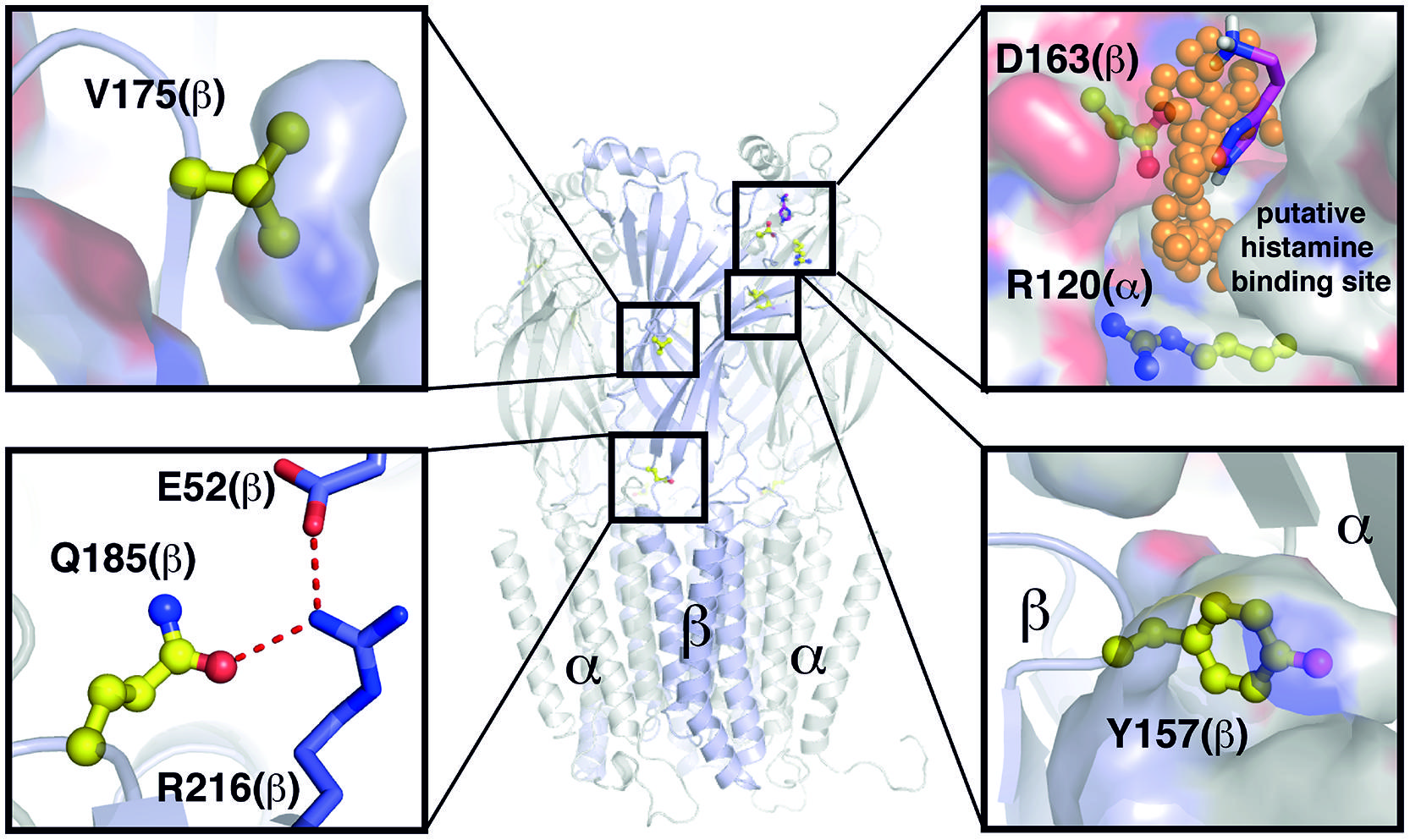
FIGURE 7. Overview of positions and functions of mutated residues. Structural model based on PDB ID 4COF (Miller and Aricescu, 2014). Backbone displayed as cartoon, van der Waals surface as surface, selected residues as sticks, or balls and sticks, respectively. Extracellular domains visible as sheets, transmembrane domains as helical domains. Putative binding positions of small organic molecules according to TRIDOCK analysis (te Heesen et al., 2007) as orange spheres and histamine in magenta. Y157 is actively taking part in formation of the interface between the two subdomains. D163(β) and R120(α) form a putative histamine binding site at the interface between subdomains α and β. Q185 at the interface between transmembrane and extracellular domains is part of a hydrogen bonding network with the highly conserved residues E52 and R216. The V175 side chain is buried within the extracellular subdomains, and thus important for their correct folding. None of the investigated residues except D163(β) and R120(α) show a putative small molecule binding site.
The point mutation β2(Y157F) was only effective in receptors containing the γ2L subunit and did not alter histamine’s action on receptors composed solely of a and β subunits. This indicates that histamine does not directly interact with the amino acid Y157. The presence of the γ2 subunit alters the potentiation by histamine (Saras et al., 2008) and propofol (Siegwart et al., 2002). An indirect participation could be caused by an influence of amino acid Y157 on the interaction of γ2 with other subunits in a heteromeric receptor. This is supported by the location of Y157 in the homology model, as it is actively taking part in formation of the interface between the two extracellular subdomains (Figure 7).
A further amino acid residue that is involved in the modulatory effect of histamine is β3(Q185). This amino acid has a potentially negative impact on histamine potentiation, and the Q185A mutation enhances the effect of lower concentrations of histamine on the GABAAR. Q185 at the interface between transmembrane and extracellular domains is part of a hydrogen bonding network with the highly conserved residues E52 and R216 (Figure 7). The amino acid V175 resides inside the extra cellular domain and is of potential structural importance (Figure 7). Therefore, the Q185A or V175A mutations influence the histamine potentiation possibly by allosteric effects and are not part of a binding site.
The two amino acid residues that we identified as possibly being directly involved in the action of histamine on the GABAAR are R120 on the α subunit and D163 on the β subunit. Amino acid R120 is conserved in all α subunits; therefore, our data could be valid for all α subunits. Laha and Wagner (2011) showed that these amino acids could be part of a state-dependent salt-bridge. This potential salt-bridge between R120 on the α subunit and D163 on the β subunit stabilizes the binding of GABA (Laha and Wagner, 2011). Disruption of the salt-bridge on the α subunit by the R120A mutation greatly increases the EC50 of GABA. The D163A mutation on the β subunit clearly reduces histamine potentiation, and histamine potentiation is nearly absent in receptors with the double mutation. Based on our homology model, these two amino acid residues are not close enough to form a salt bridge directly (Figure 7). However, the crystal structure is derived from a homopentameric receptor in a ligand bound state with the artificial agonist benzamidine. Though this benzamidine binding site is found in the vicinity of Y157 (Miller and Aricescu, 2014), it might influence the overall structure of the extracellular domain, including the position of R120. However, an analysis with TRIDOCK (te Heesen et al., 2007) revealed that a putative small molecule ligand binding site exists between D163 and R120 (Figure 7). Furthermore, our docking analysis revealed that histamine can bind in various ways at this site (see Figure 7 and Supplementary Figure 2) with a comparably low predicted binding affinity [ΔG(bind) = -3.9 to –3.7 kcal/mol], which is in agreement with our comparably low histamine affinities measured experimentally. At the found docking positions, the histamine ammonium headgroup interacts majorly with D163(β), and thus probably weakens the R120/D163 interaction, which is in agreement with our experimental results. Furthermore, the docking positions found are in good agreement with the binding positions found by TRIDOCK. This position therefore could be a binding site for histamine, as well.
On the basis of our investigations, we suggest that histamine interacts with D163 on the β subunit and weakens the interaction of D163 with R120, which could lead to enhanced or prolonged GABA binding, a left shift of the GABA EC50 and thereby to potentiation. Consequently, we propose that the amino acid D163 is essential for the modulation of GABAARs by histamine and possibly a part of the histamine binding site. We demonstrate with our results a further function of the interaction of the α and β subunit mediated by D163 with R120, which is vital for both histamine potentiation and GABA affinity. The amino acid D163 is conserved in all β subunits of the GABAARs. In addition, amino acids homologous to D163 could be detected in the α1 subunit of the GlyR and α7 subunit of the nAChRs (Galzi et al., 1996; Newell et al., 2004). The homologous amino acid in the nAChR is involved in the change of the affinity for calcium and acetylcholine (Galzi et al., 1996).
Conclusion
Our data show that histamine’s potentiation depends on amino acid D163 of the β subunit and R120 of the α subunit. Interaction of these amino acids lowers the EC50 for GABA (Laha and Wagner, 2011). We propose that histamine potentiates GABAARs by influencing this interaction and that these amino acids are part of a potential histamine binding site.
Conflict of Interest Statement
The authors declare that the research was conducted in the absence of any commercial or financial relationships that could be construed as a potential conflict of interest.
Acknowledgments
We thank J. Gerkrath, T. Lichtleitner, and U. Müller and for excellent technical assistance. This work was funded by a grant (SFB 642) from the German Research Foundation (Deutsche Forschungsgemeinschaft) to HH.
Supplementary Material
The Supplementary Material for this article can be found online at: http://journal.frontiersin.org/article/10.3389/fphar.2015.00106/abstract
References
Amin, J., and Weiss, D. S. (1993). GABAA receptor needs two homologous domains of the β-subunit for activation by GABA but not by pentobarbital. Nature 366, 565–569. doi: 10.1038/366565a0
Bekkers, J. M. (1993). Enhancement by histamine of NMDA-mediated synaptic transmission in the hippocampus. Science 261, 104–106. doi: 10.1126/science.8391168
Belelli, D., and Lambert, J. J. (2005). Neurosteroids: endogenous regulators of the GABAA receptor. Nat. Rev. Neurosci. 6, 565–575. doi: 10.1038/nrn1703
Bianchi, M. T., Clark, A. G., and Fisher, J. L. (2011). The wake-promoting transmitter histamine preferentially enhances α-4 subunit-containing GABAA receptors. Neuropharmacology 61, 747–752. doi: 10.1016/j.neuropharm.2011.05.020
Biasini, M., Bienert, S., Waterhouse, A., Arnold, K., Studer, G., Schmidt, T., et al. (2014). SWISS-MODEL: modelling protein tertiary and quaternary structure using evolutionary information. Nucleic Acids Res. 42, W252–W258. doi: 10.1093/nar/gku340
Bureau, M. H., and Olsen, R. W. (1991). Taurine acts on a subclass of GABAA receptors in mammalian brain in vitro. Eur. J. Pharmacol. 207, 9–16. doi: 10.1016/S0922-4106(05)80031-8
Chang, Y., and Weiss, D. S. (1999). Allosteric-activation mechanism of the α1β2γ2γ-aminobutyric acid type A receptor revealed by mutation of the conserved M2 leucine. Biophys. J. 77, 2542–2551. doi: 10.1016/S0006-3495(99)77089-X
Congreve, M., Carr, R., Murray, C., and Jhoti, H. (2003). A rule of three for fragment- based lead discovery. Drug Discov. Today 8, 876–877. doi: 10.1016/S1359-6446(03)02831-9
Edgar, R. (2004). MUSCLE: multiple sequence alignment with high accuracy and high throughput. Nucleic Acids Res. 32, 1792–1797. doi: 10.1093/nar/gkh340
Galzi, J. L., Bertrand, S., Corringer, P. J., Changeux, J. P., and Bertrand, D. (1996). Identification of calcium binding sites that regulate potentiation of a neuronal nicotinic acetylcholine receptor. EMBO J. 15, 5824–5832.
Grobin, A. C., Matthews, D. B., Devaud, L. L., and Morrow, A. L. (1998). The role of GABAA receptors in the acute and chronic effects of ethanol. Psychopharmacology (Berl.) 139, 2–19. doi: 10.1007/s002130050685
Haas, H. L., Sergeeva, O. A., and Selbach, O. (2008). Histamine in the nervous system. Physiol. Rev. 88, 1183–1241. doi: 10.1152/physrev.00043.2007
Heckman, K. L., and Pease, L. R. (2007). Gene splicing and mutagenesis by PCR-driven overlapextension. Nat. Protoc. 2, 924–932. doi: 10.1038/nprot.2007.132
Hosie, A. M., Dunne, E. L., Harvey, R. J., and Smart, T. G. (2003). Zinc-mediated inhibition of GABAA receptors: discrete binding sites underlie subtype specificity. Nat. Neurosci. 6, 362–369. doi: 10.1038/nn1030
Jurd, R., Arras, M., Lambert, S., Drexler, B., Siegwart, R., Crestani, F., et al. (2003). General anesthetic actions in vivo strongly attenuated by a point mutation in the GABAA receptor β3 subunit. FASEB J. 17, 250–252.
Kim, H., and McDonald, R. L. (2003). An n-terminal histidine is the primary determinant of α subunit-dependent Cu2+ sensitivity of αβ3γ2L GABAA receptors. Mol. Pharmacol. 64, 1145–1152. doi: 10.1124/mol.64.5.1145
Kletke, O., Sergeeva, O. A., Lorenz, P., Oberland, S., Meier, J. C., Hatt, H., et al. (2013). New insights in endogenous modulation of ligand-gated ion channels: histamine is an inverse agonist at strychnine sensitive glycine receptors. Eur. J. Pharmacol. 710, 59–66. doi: 10.1016/j.ejphar.2013.04.002
Krasowski, M. D., Nishikawa, K., Nikolaeva, N., Lin, A., and Harrison, N. L. (2001). Methionine 286 in transmembrane domain 3 of the GABAA receptor β subunit controls a binding cavity for propofol and other alkylphenol general anesthetics. Neuropharmacology 41, 952–964. doi: 10.1016/S0028-3908(01)00141-1
Kufareva, I., Rueda, M., Katritch, V., Stevens, R. C., and Abagyan, R. (2011). Status of GPCR modeling and docking as reflected by community-wide GPCR dock 2010 assessment. Structure 19, 1108–1126. doi: 10.1016/j.str.2011.05.012
Laha, K. T., and Wagner, D. A. (2011). A state-dependent salt-bridge interaction exists across the β/α intersubunit interface of the GABAA receptor. Mol. Pharmacol. 79, 662–671. doi: 10.1124/mol.110.068619
Li, G. D., Chiara, D. C., Sawyer, G. W., Husain, S. S., Olsen, R. W., and Cohen, J. B. (2006). Identification of a GABAA receptor anesthetic binding site at subunit interfaces by photolabeling with an etomidate analog. J. Neurosci. 26, 11599–11605. doi: 10.1523/JNEUROSCI.3467-06.2006
McDonald, R. L., and Olsen, R. W. (1994). GABAA receptor channels. Annu. Rev. Neurosci. 17, 569–602. doi: 10.1146/annurev.ne.17.030194.003033
Miller, P. S., and Aricescu, A. R. (2014). Crystal structure of a human GABAA receptor. Nature 512, 270–275. doi: 10.1038/nature13293
Newell, J. G., McDevitt, R. A., and Czajkowski, C. (2004). Mutation of Glutamate 155 of the GABAA Receptor β2 subunit produces a spontaneously open channel: a trigger for channel activation. J. Neurosci. 24, 11226–11235. doi: 10.1523/JNEUROSCI.3746-04.2004
Nutt, D. (2006). GABAA receptors: subtypes, regional distribution, and function. J. Clin. Sleep Med. 2, 7–11.
Olsen, R. W., and Sieghart, W. (2008). International union of pharmacology. LXX. Subtypes of gamma-aminobutyric acid A receptors: classification on the basis of subunit composition, pharmacology, and function. Update. Pharmacol. Rev. 60, 243–260. doi: 10.1124/pr.108.00505
Pirker, S., Schwarzer, C., Wieselthaler, A., Sieghart, W., and Sperk, G. (2000). GABAA receptors: immuncytochemical distribution of 13 subunits in the adult rat brain. Neuroscience 101, 815–850. doi: 10.1016/S0306-4522(00)00442-5
Reynolds, D. S., Roshal, T. W., Cirone, J., O’Meara, G. F., Haythornthwaite, A., Newman, R. J., et al. (2003). Sedation and anesthesia mediated by distinct GABAA receptor isoforms. J. Neurosci. 23, 8608–8617.
Rudolph, U., and Knoflach, F. (2011). Beyond classical benzodiazepines: novel therapeutic potential of GABAA receptor subtypes. Nat. Rev. Drug Discov. 10, 685–697. doi: 10.1038/nrd3502
Saras, A., Gisselmann, G., Vogt-Eisele, A. K., Erlkamp, K. S., Kletke, O., Pusch, H., et al. (2008). Histamine action on vertebrate GABAA receptors; direct channel gating and potentiation of GABA responses. J. Biol. Chem. 283, 10470–10475. doi: 10.1074/jbc.M709993200
Schneider, M., Wolf, S., Schlitter, J., and Gerwert, K. (2011). The structure of active opsin as a basis for identification of GPCR agonists by dynamic homology modelling and virtual screening assays. FEBS Lett. 585, 3587–3592. doi: 10.1016/j.febslet.2011.10.027
Schuttelkopf, A. W., and van Aalten, D. M. (2004). PRODRG: a tool for high-throughput crystallography of protein-ligand complexes. Acta Crystallogr. D Biol. Crystallogr. 60, 1355–1363. doi: 10.1107/S0907444904011679
Sergeeva, O. A., Kletke, O., Kragler, A., Poppek, A., Fleischer, W., Schubring, S. R., et al. (2010). Fragrant dioxane derivates identify β1-subunit-containing GABAA receptors. J. Biol. Chem. 285, 23985–23993. doi: 10.1074/jbc.M110.103309
Siegwart, R., Jurd, R., and Rudolph, U. (2002). Molecular determinants for the action of general anesthetics at recombinant α2β3γ2γ-aminobutyric acidA receptors. J. Neurochem. 80, 140–148. doi: 10.1046/j.0022-3042.2001.00682.x
Sigel, E., Baur, R., Kellenberger, S., and Malherbel, P. (1992). Point mutations affecting antagonist affinity and agonist dependent gating of GABAA receptor channels. EMBO J. 11, 2017–2023.
Simon, J., Wakimoto, H., Fujita, N., Lalande, M., and Barnard, E. A. (2004). Analysis of the set of GABAA receptor genes in the human genome. J. Biol. Chem. 279, 41422–41435. doi: 10.1074/jbc.M401354200
te Heesen, H. T., Schlitter, A. M., and Schlitter, J. (2007). Empirical rules facilitate the search for binding sites on protein surfaces. J. Mol. Graph. Model. 25, 671–679. doi: 10.1016/j.jmgm.2006.05.005
The UniProt Consortium. (2013). Activities at the Universal Protein Resource (UniProt). Nucleic Acids Res. 42, D191–D198. doi: 10.1093/nar/gkt1140
Thompson, S. A., Whiting, P. J., and Wafford, K. A. (1996). Barbiturate interactions at the human GABAA receptor: dependence on receptor subunit combination. Br. J. Pharmacol. 117, 521–527. doi: 10.1111/j.1476-5381.1996.tb15221.x
Trott, O., and Olson, A. J. (2010). AutoDock vina: improving the speed and accuracy of docking with a new scoring function, efficient optimization, and multithreading. J. Comput. Chem. 31, 455–461.
Williams, C. A., Bell, S. V., and Jenkins, A. (2010). A residue in loop 9 of the β2-subunit stabilizes the closed state of the GABAA receptor. J. Biol. Chem. 285, 7281–7287. doi: 10.1074/jbc.M109.050294
Wolf, S., Böckmann, M., Höweler, U., Schlitter, J., and Gerwert, K. (2008). Simulations of a G protein-coupled receptor homology model predict dynamic features and a ligand binding site. FEBS Lett. 582, 3335–3342. doi: 10.1016/j.febslet.2008.08.022
Keywords: GABAAR, histamine, modulation, site-directed mutagenesis, potentiation, salt-bridge
Citation: Thiel U, Platt SJ, Wolf S, Hatt H and Gisselmann G (2015) Identification of amino acids involved in histamine potentiation of GABAA receptors. Front. Pharmacol. 6:106. doi: 10.3389/fphar.2015.00106
Received: 19 February 2015; Accepted: 01 May 2015;
Published online: 26 May 2015
Edited by:
Jean-François Desaphy, University of Bari Aldo Moro, ItalyReviewed by:
Oscar Moran, Consiglio Nazionale delle Ricerche, ItalyChristoph Fahlke, Forschungszentrum Jülich, Germany
Copyright © 2015 Thiel, Platt, Wolf, Hatt and Gisselmann. This is an open-access article distributed under the terms of the Creative Commons Attribution License (CC BY). The use, distribution or reproduction in other forums is permitted, provided the original author(s) or licensor are credited and that the original publication in this journal is cited, in accordance with accepted academic practice. No use, distribution or reproduction is permitted which does not comply with these terms.
*Correspondence: Günter Gisselmann, Department of Cell Physiology, Ruhr University Bochum, Building ND4-164, Universitätsstraße 150, Bochum 44780, Germany,Z3VlbnRlci5naXNzZWxtYW5uQHJ1Yi5kZQ==