- 1 Department of Pharmaceutical Technology, Jadavpur University, Kolkata, India
- 2 Radiation Biology and Health Sciences Division, Bhabha Atomic Research Centre, Mumbai, India
Treatment of cancer often requires exposure to radiation, which has several limitations involving non-specific toxicity toward normal cells, reducing the efficacy of treatment. Efforts are going on to find chemical compounds which would effectively offer protection to the normal tissues after radiation exposure during radiotherapy of cancer. In this regard, plant-derived compounds might serve as “leads” to design ideal radioprotectors/radiosensitizers. This article reviews some of the recent findings on prospective medicinal plants, phytochemicals, and their analogs, based on both in vitro and in vivo tumor models especially focused with relevance to cancer radiotherapy. Also, pertinent discussion has been presented on the molecular mechanism of apoptotic death in relation to the oxidative stress in cancer cells induced by some of these plant samples and their active constituents.
Introduction
Cancer is now the third leading cause of death worldwide, with an estimated 12 million new cases and 7.6 million cancer deaths reported in American Cancer Society (2007). It is projected that by 2030 there would be about 26 million new cases, while a majority of deaths due to cancer will occur in developing countries (Bray and Moller, 2006; World Cancer Report, 2008). In the meantime, the global distribution of cancer along with the predominating types of the disease continues to change. Thus, cancers of the lung, breast, colon/rectum, and prostate are no longer confined to the Western industrialized countries but are among the most common cancers occurring all over the world. As for the therapy and management of cancer, newer strategies comprising multi-faceted and integrative approach involving surgery, followed by chemotherapy along with radiation is currently gaining consensus (Oehler et al., 2007). At the same time, advancement in the understanding of the disease processes at molecular level has offered novel targets for prevention, detection, control, and elimination of cancer.
Application of ionizing radiation, over and above surgery, and chemotherapy, has been the treatment of choice in case of solid malignancies (Kinsella, 2011). However, a substantial fraction of such tumors would fail to respond well to the radiation treatment, and require a very high dose to get killed, posing a severe limitation to the radiotherapy. Additionally, undesirable complications would occur owing to radiation injury to the surrounding normal tissues and to the skin, brain, heart, lung, kidney, liver, or gastrointestinal system of the cancer patient. Also, symptoms like tissue fibrosis, hair loss, xerostomia, xerophthalmia, etc., considerably restrict the application of a high dose of radiation aimed at the tumor-bearing organs (Dest, 2006).
Moreover, co-administration of radiation (delivered in the range of 40–80 Gy) along with the chemotherapeutic regimen might aggravate these complications (Curry and Curran, 2003). Patients undergoing treatment with taxol or vincristine often suffer from peripheral neuropathy as a side effect, while anthracycline drugs like doxorubicin (adriamycin), epirubicin, and mitoxantrone, etc., might lead to cardiac dysfunction (Choy, 2001). Abnormal kidney function and hearing loss were some of the common adverse effects occurring upon radiotherapy given to patients under treatment with platinum compounds (Amorino et al., 2000). Combination with some of the alkylating agents, like cyclophosphamide, ifosfamide, and leukeran (chlorambucil), might cause infertility (Verma et al., 2007). Again, a long term problem might emerge due to the post-radiotherapy incidence of a second tumor appearing either at the site of irradiation or away from it (Ng et al., 2002). Hence, it is a major challenge to radiation oncologists and researchers to develop alternative approaches to minimize the dosage through selective sensitization of tumor cells to respond to the radiation treatment, and thereby evade the detrimental consequences of radiotherapy (Rosenberg and Knox, 2006).
The exposure to radiation would primarily generate intracellular reactive oxygen species (ROS, viz., superoxide and hydroxyl radicals), which in turn would lead to DNA strands breaks and conformational alterations of biomolecules (Halliwell and Gutteridge, 1989). This will inevitably cause damage to surrounding normal cells. Hence, certain compounds/formulations could be envisaged to effectively scavenge the free radicals and thereby protect the surrounding normal cells from radiation induced injury. Historically, the seminal findings on the radioprotective ability of naturally occurring amino-metabolites like cysteine and cysteamine triggered the search for other thiolamines which would protect us from the acute effects of radiation (Patt et al., 1949). Thus, amifostine ([S-2-[3-aminopropylamino] ethylphosphorothioic acid) was developed as a potential radioprotector molecule (Weiss and Landauer, 2000; Grdina et al., 2002; Bensadoun et al., 2006). Nevertheless, its wider applicability has been restricted due to the major limitations associated with nausea, diarrhea, hypotension, hypocalcaemia, sleeplessness, dizziness, nephro- and neuro-toxic effects. Other non-protein thiols possessing antioxidative properties, viz. captopril ([S]-1-[3-mercapto-2-methyl-1-oxo-propyl]-L-proline), mesna (sodium-2-mercapto-ethanesulfonate), and N-acetyl-L-cysteine (NAC), were also relevant in this regard (Murley et al., 2004). However, these exogenous antioxidants proved to be ineffective if administered at the post-irradiation stage. These scavengers, acting only for a limited period of time (15 min to 1 h under in vivo conditions), must be administered shortly before the radiation exposure as the generation of highly reactive free radicals following radiation is a very rapid process, spanning less than 10−3 s (Grdina et al., 2002). Hence, there is an urgent need to look for more suitable molecules to be used in combination with chemo- and radio-therapy of cancer in order to minimize the adverse effects, and to enhance the overall curative outcome in the patients.
Screening and testing of compounds from natural as well as synthetic sources have been carried out over the last few decades in order to find effective radioprotectors capable of inhibiting radiation damage not only during radiotherapy of cancer patients, but also to healthy individuals undergoing occupational and accidental exposures to radiation (Stone et al., 2003). In this context, several authors have reviewed the prospective application of traditional medicinal plants which are known to contain anti-inflammatory, antioxidant, and immunomodulatory compounds (Arora et al., 2005; Venkatachalam and Chattopadhyay, 2005; Jagetia, 2007). Again, plant-derived polyphenolic compounds with radiosensitizing property have been extensively reviewed elsewhere (Garg et al., 2005). Incidentally, some of these antioxidants and plant products were also shown to be effective in prevention of cancer incidence (Suresh and Vasudevan, 1994; Zhao et al., 1997; Lee et al., 2002; Girdhani et al., 2005). The present article would be following up these developments during the last 5 years, and aim to highlight the current endeavor (since 2006) to identify phytochemicals and secondary metabolites of medicinal plants with relevance to cancer radiotherapy, and at the same time, attempt to elucidate the mechanistic premise in the light of available reports.
Potential Plant Products for Application in Cancer Radiotherapy
The global search for naturally occurring phytochemicals as potential radiotherapeutic agents has unearthed a host of plant products broadly categorized as (i) “radioprotectors”- to ameliorate the undesired damages caused to the normal cells, hence, minimize the side effects of radiation therapy; and (ii) “radiosensitizers”- to enhance the radiation-induced cell death inflicted to the tumor, and thereby minimize the dose of radiation treatment. In the present article, some of the major findings on traditional medicinal plants and active phytochemicals with promising radioprotective or radiosensitizing efficacy have been briefly summarized in a tabular form (Tables 1 and 2; post-2006).
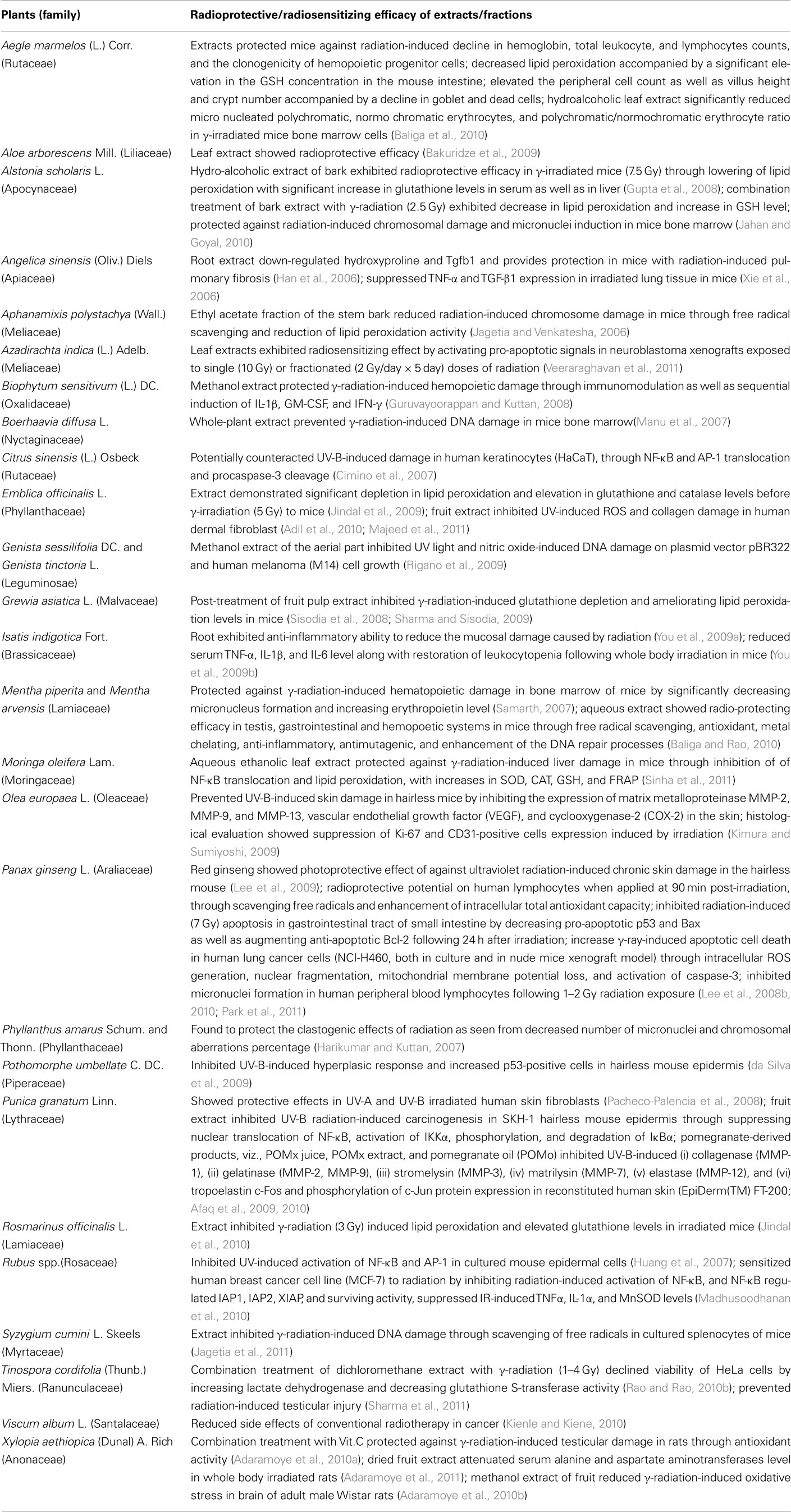
Table 1. Traditional medicinal plants and/or their bioactive constituents with prospective radioprotective/radiosensitizing efficacy (2006–2011).
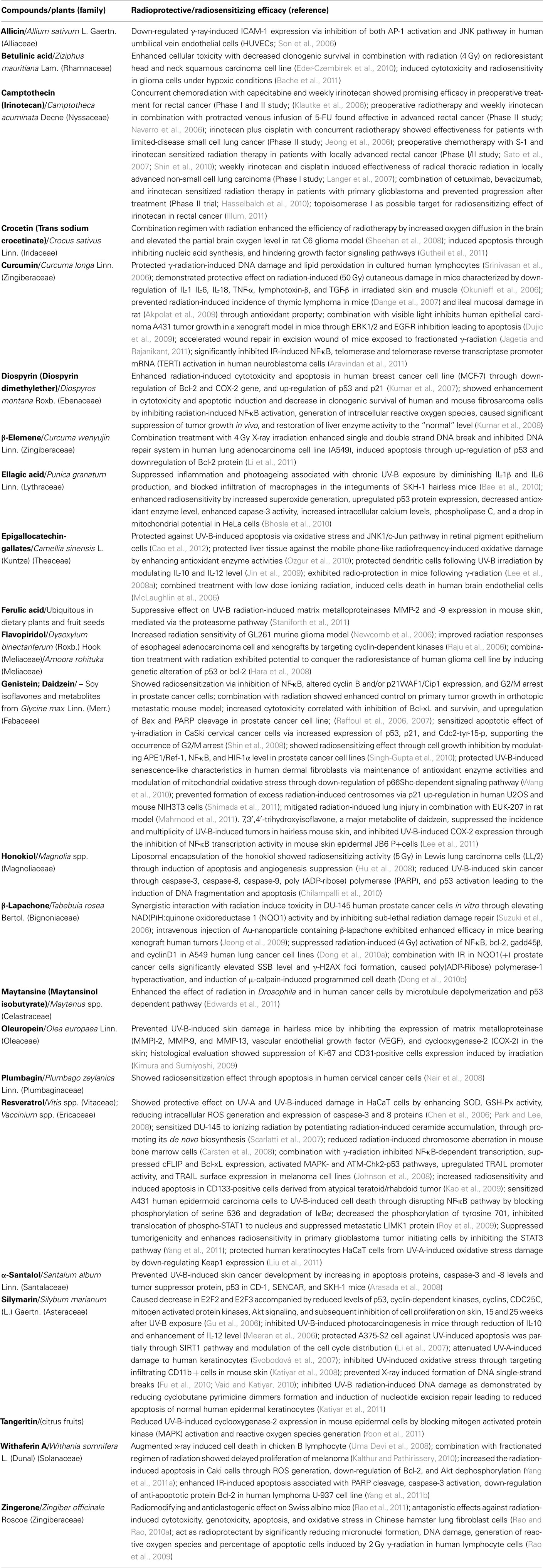
Table 2. Phytochemicals with prospective radioprotective/radiosensitizing efficacy: reports from last 5 years study.
In Table 1, we have enlisted the reports on the crude extracts/semi-purified fractions of plant samples which demonstrated substantial prospect to enhance the clinical success of radiotherapy through a combination treatment. It is to be noted that 14 out of the twenty six plants in this list, viz. Aloe arborescens, Angelica sinensis, Azadirachta indica, Biophytum sensitivum, Boerhaavia diffusa, Citrus sinensis, Genista sessilifolia, Grewia asiatica, Isatis indigotica, Moringa oleifera, Olea europaea, Rosmarinus officinalis, Rubus spp., and Xylopia aethiopica have not been investigated prior to 2006. Most of the reports were obtained from the in vivo study on mouse models, where the radio-protecting activity was found to be associated with significant scavenging of free radicals, and depletion in lipid peroxidation with elevation in the glutathione, catalase, and lactate dehydrogenase enzyme levels. Also, several of these studies were conducted on propagatory cell lines and primary cultures to unravel the underlying mode of action at the molecular level (Table 1; Kimura and Sumiyoshi, 2009; Lee et al., 2010; Park et al., 2011).
In Table 2, we have presented the pure plant constituents and/or their analogs which could be considered as emerging candidates to be developed for the aforesaid application in future. Here, it is to be noted that the radiotherapeutic prospect of several plant-derived compounds, viz. allicin, betulinic acid, crocetin, diospyrin, honokiol, maytansine, oleuropein, α-santalol, tangeritin, withaferin A, and zingerone have been reported for the first time during the last 5 years.
Here, in Table 2, it has to be mentioned that we have not included the established plant-derived anticancer drugs, viz. etoposide, pactitaxel, and Vinca alkaloids, which have not only been recognized as potential radiosensitizers, but already under clinical application in association with cancer radiotherapy (Burris and Hurtig, 2010). Nevertheless, these drugs and their analogs are also under continuous appraisal for further development in this regard (Hiro et al., 2010; Orditura et al., 2010; Lillo et al., 2011; Schwarzenberger et al., 2011).
Again, prospective radiotherapeutic application of herbal formulations composed of traditional medicinal plants, and marketed as Triphala, Abana, Mentat, Septilin, Chyavanaprasha, Oligonol, HemoHIM, Fuzheng zengxiao formula, etc., have been reported by Sandhya et al. (2006), Jagetia (2007), Kundu et al. (2008), Park et al. (2010), Huang et al. (2011). In fact, the potent radioprotective property of Triphala, a mixture of three plants, might actually be attributed to the presence of Emblica officinalis (vide Table 1), which is also a major constituent of some of the other Oriental rejuvenators (Chyavanaprasha, Septilin, etc.) found to offer protection against radiation damage. Likewise, some herbal products, like HemoHIM from Far-East countries, contain rhizhomes of Angelica spp., a prospective source of radioprotectants (vide Table 1), while Oligonol is composed of modified plant phenolics. Therefore, these commercial formulations comprising poly-herbal mixtures have been kept outside the purview of the present article in order to focus on the search for new plants and phytochemicals with prospective radiotherapeutic property, and not included in our Tables.
Molecular Mechanism of Natural Radioprotectors/Radiosensitizers
Over the years, multi-modal therapy involving more than one anticancer agent applied in combination has been found to be favorable in the management of cancer. The precise efficacy and degree of tumor control exhibited by combination regimen, however, remains variable. Although the reasons for variability remain unclear, discovery of additional novel drugs that synergize with an existing radiation therapy would allow multiple combinations to choose from, thereby increasing the likelihood of clinical success. Recently, Edwards et al. (2011) has developed an interesting Drosophila larvae model which could be used to screen and identify molecules that would act in conjunction with radiation therapy (Edwards et al., 2011). On the whole, a number of phytochemicals with anti-/pro-oxidant, or immunomodulatory activity hold greater promise in pre-clinical/clinical trials. Emerging data also demonstrated that many phytochemicals, especially camptothecin, epigallocatechin gallate (EGCG), paclitaxel, etoposide, curcumin, etc., have potent growth inhibitory and apoptosis inducing effects on human as well as animal cancer cells by targeting multiple cellular signaling pathways in vitro. Therefore, these compounds could be useful in combination with conventional chemotherapeutic agents/radiation for the treatment of cancer, and expected to have lower toxicity but higher effectiveness. Also, recent in vivo pre-clinical studies and clinical trials have provided increasing evidence in support of multi-targeted therapies in combination with natural products. A comprehensive view on the molecular mechanisms to rationalize the prospective role of such phytochemicals acting on relevant signaling pathways has been given in Table 2.
Scavenging of Reactive Oxygen Species
Natural products in conjunction with irradiation are likely to exert the protective action through several mechanisms. Scavenging of free radicals generated during radiolysis would be a credible mode of action. Hence, naturally occurring polyphenolic compounds and antioxidant vitamins, primarily retinoids, would be the plausible candidates to offer radio-protection. It is a fact that the chances of developing cancer could be minimized through optimum nutritional supplementation by consuming a variety of fruits and vegetables, some of which have displayed chemopreventive activity by inhibiting tumorigenesis induced by chemical carcinogens and other genotoxic agents (Loo, 2003).
However, clinical reports on application of plant extracts with antioxidant property as adjuvants in cancer radiotherapy are still sparse in literature. Apparently, there is an apprehension that the antioxidants would protect not only the normal cells, but also the tumors, from the attack of free radicals generated during the course of treatment with ionizing radiation and anticancer agents. The lack of strong experimental evidences to address this concern resulted in poor enthusiasm from radiation oncologists to recommend their patients to consume such antioxidant products during the course of therapy. However, the pros and cons of this aspect have been critically reviewed, based on in vitro and in vivo experimentations (Prasad, 2005; Prasad and Cole, 2006).
In this context, hydrogen peroxide (H2O2) has been known to play a crucial role in the proliferation of cancer cells. In fact, many human cancers, like melanoma, neuroblastoma, colon carcinoma, and ovarian carcinoma, were found to constitutively generate a high amount of H2O2 (Szatrowski and Nathan, 1991). This indicated that the tumor cells would require a certain level of oxidative stress for maintaining a balance to undergo either proliferation or apoptotic death, and a minor fluctuation in the concentration of ROS might be critical to the intracellular signaling mechanism (Droge, 2002). The production of a larger amount of H2O2 was demonstrated by transforming NIH3T3 cells with Ras oncogene to cancer cells (Benhar et al., 2001), and a similar observation was noted in our laboratory when thymus cells in mice were transformed to thymic lymphoma after whole body radiation exposure (Pandey et al., unpublished data). The elevated production of ROS in cancer cells might be routed through mitochondrial electron transport chain, peroxisomes or NAD(P)H oxidase pathways, but the involvement of some direct mechanism of H2O2 generation may also be suggested. The constitutive production of ROS caused sub-lethal DNA damage in tumors, which was evidenced by a higher level of 8-hydroxy-2′-deoxyguanosine, while the increase of 4-hydroxy-2-non-enal, the lipid peroxidation product, indicated damage in cell membrane of carcinoma tissues (Toyokuni et al., 1995; Kondo et al., 1999). The cancer cells, in spite of having some amount of sub-lethal DNA damage, are generally adapted to survive in such stress conditions, and do not undergo cell cycle arrest or apoptosis (Elledge and Lee, 1995). In fact, at the basal level, the ROS would provide a stimulatory environment conducive to the proliferation of cancer cells, and become somewhat vital to their survival, rather than being merely useless cytotoxic products. Therefore, it may be hypothesized that the phenolic compounds with antioxidant properties could induce cell cycle arrest and apoptosis through scavenging of H2O2, presumably by depriving the cancer cells of an essential factor vital to their sustenance. It could also be speculated that a relatively lower level of constitutive ROS in normal cells would make them less vulnerable to the phenolic antioxidants (Simone et al., 2007).
Generation of Reactive Oxygen Species
Another category of phytochemicals showing antitumor activity are those which would enhance the generation of ROS, instead of scavenging the cellular free radicals. Such ROS-generators would apparently sensitize cancer cells endowed with persistent oxidative stress to undergo apoptotic death. We have already discussed about the critical maintenance of constitutively produced ROS, which is probably just below the threshold level required to induce apoptosis in tumors, the basal level being much lower in case of normal cells (Droge, 2002). Therefore, ROS-generating quinones could presumably create the requisite imbalance to lead the tumors cells, rather than the normal ones, to apoptotic death. Thus, the pro-oxidant quinones like β-lapachone (Suzuki et al., 2006; Dong et al., 2010a,b), plumbagin (Nair et al., 2008), and diospyrin derivatives (Hazra et al., 2007; Kumar et al., 2007, 2008) have been found to induce apoptosis in tumor cells through DNA damage, lipid peroxidation, mitochondrial membrane depolarization, and related signaling events. Incidentally, a few other anticancer plant products, such as paclitaxel, vinca alkaloids, and maytansinol, have been found to enhance the effect of radiation in human cancer cells through the involvement of microtubule interference to inhibit the proliferation of tumor (Edwards et al., 2011).
Induction of Apoptotic Pathway
Further, the phytochemicals could also interact directly with molecular pathways involving kinase networks, like mitogen activated protein kinases (MAPK), phosphatidylinositol-3-kinase (PI-3K), etc., thereby showing the potential to inhibit tumor growth in combination with anticancer drugs and radiation therapy by inducing programmed cell death or apoptosis (Garg et al., 2005).
Again, the higher level of ROS could result in the increase in protein tyrosine kinase (PTK)-mediated phosphorylation of epidermal growth factor receptor (EGF-R; Kamata et al., 2000), sequentially activating the Ras- and MAPK-signaling pathways (Loo, 2003). Further, the over-activated MAPK would trigger expression of transcription factors, like nuclear factor kappa B (NF-κB), activator protein-1 (AP-1), and c-myc (a proto-oncoprotein; Meyer et al., 1994; Muller et al., 1997). It is to be noted that these transcription factors by themselves are redox-sensitive (Meyer et al., 1994; Muller et al., 1997; Loo, 2003), and may be regulated directly by the higher level of ROS.
The molecular mechanism of anticancer property of plant-derived polyphenolic compounds, such as EGCG, resveratrol, quercetin, genistein, etc., has been primarily attributable to their ability to scavenge the constitutively expressed endogenous redox modulators (H2O2/OH.). Further studies revealed that EGCG inhibited the phosphorylation of MAPK-enzymes, viz. ERK (extracellular signal regulated kinases), JNK (c-Jun N-terminal kinases), and p38-MAPK (p38 mitogen activated protein kinases), activated by UV-B radiation in human epidermal keratinocytes (Katiyar et al., 2001). Similar observation was obtained in UV-C-irradiated HeLa cells, pretreated with resveratrol, a polyphenolic constituent present in grapes and berries (Yu et al., 2001). Resveratrol was also found to check NF-κB activation induced by tumor necrosis factor (TNF) in U-937, Jurkat, HeLa, and H4 glioma cells in vitro (Manna et al., 2000). A few other plant phenolics, such as quercetin and genistein, have been reported to initiate apoptosis in pancreatic carcinoma cells, by inducing mitochondrial depolarization, cytochrome c release, and activation of caspases (Mouria et al., 2002).
In our laboratory, we are investigating the radiomodulating potential of a diethtylether derivative (D7) of diospyrin, an antitumor quinonoid plant-product, in human breast carcinoma cells (MCF-7). It was observed that D7, in combination with radiation, could increase the apoptosis in tumor cells through down-regulation of the anti-apoptotic Bcl-2 and COX-2 gene expression, and up-regulation of pro-apoptotic genes, like p53 and p21. The higher expression of PUMA (p53 upregulated modulator of apoptosis), a pro-apoptotic protein, was also observed in the combination treatment. Further, the up-regulation of p21 expression in irradiated MCF-7 cells was found to be concomitant with the cell cycle arrest in the G1 phase (Kumar et al., 2007). Further studies in mouse and human fibrosarcoma cells (viz., Wehi164 and HT1080, respectively) showed marked enhancement of cytotoxicity with decreased clonogenic survival following treatment with D7 in combination with radiation. Moreover, increased radiosensitivity of tumor cells by D7 was found to occur through inhibition of radiation-induced NF-κB activation with substantial generation of intracellular ROS, ultimately leading to programmed cell death. Further, a combination regimen of D7 with 5 Gy radiation administered in two fractionated doses (2.5 Gy each) could cause a significant inhibition of tumor growth and increased life span of experimental mice bringing the liver enzyme activity to the normal level (Kumar et al., 2008).
Conclusion
Cancer patients need to go through extensive treatment involving chemotherapy, surgical intervention, recurring exposure to gamma-irradiation, or a combination therapy. Some traditionally popular medicinal plants have recently gained attention for their ability to modulate a number of signaling pathways that could initiate and facilitate the proliferation of cancer. In many cases, the potency of these compounds/formulations to sensitize the cancer cells to radiotherapy could be corroborated with the inhibition/activation of the relevant molecular markers. However, the literature citations on supporting clinical trials showing similar observations are quite limited. Nevertheless, a number of reports are available on antioxidants being able to protect against radiation-induced oncogenic transformations in experimental systems. Based on these information it has been presumed that supplementation of vitamins in a good measure, and intake of health promoting plant products in the diet might reduce the harmful side effects of standard therapeutic modalities and enhance their selective toxicity toward malignant cells, leading to an overall improvement in the efficacy of anticancer treatment.
Furthermore, the underlying mechanism of survival and proliferation in some types of cancer would reveal the inherent dependence of these cells on their constitutive oxidative stress. This mechanistic interpretation, in the light of the well-studied role of plant-polyphenolics in scavenging cellular free radical species, might resolve the prevailing dilemma on whether antioxidants would provide the desirable relief, to some extent, to the normal cells in preference to the tumor-bearing ones. Again, this hypothesis would be relevant to the radiosensitizing effect exhibited by a few ROS-generating antitumor agents as well. Thus, it is hoped that future research would add up positively, and would bring more of the aforesaid phytochemicals from “bench to bedside” of the suffering humanity seeking relief from the awful maladies of cancer.
Conflict of Interest Statement
The authors declare that the research was conducted in the absence of any commercial or financial relationships that could be construed as a potential conflict of interest.
Acknowledgments
Banasri Hazra is grateful to the Board of Radiation and Nuclear Sciences, Department of Atomic Energy, Mumbai (Grant No. 2008/37/30/BRNS), and the University Grants Commission, New Delhi, for financial support. Subhalakshmi Ghosh is a Research Associate under this program.
References
Adaramoye, O. A., Adedara, I. A., Popoola, B., and Farombi, E. O. (2010a). Extract of Xylopia aethiopica (Annonaceae) protects against gamma-radiation induced testicular damage in Wistar rats. J. Basic. Clin. Physiol. Pharmacol. 21, 295–313.
Adaramoye, O. A., Okiti, O. O., and Farombi, E. O. (2011). Dried fruit extract from Xylopia aethiopica (Annonaceae) protects Wistar albino rats from adverse effects of whole body radiation. Exp. Toxicol. Pathol. 63, 635–643.
Adaramoye, O. A., Popoola, B. O., and Farombi, E. O. (2010b). Effects of Xylopia aethiopica (Annonaceae) fruit methanol extract on gamma-radiation-induced oxidative stress in brain of adult male Wistar rats. Acta Biol. Hung. 61, 250–261.
Adil, M. D., Kaiser, P., Satti, N. K., Zargar, A. M., Vishwakarma, R. A., and Tasduq, S. A. (2010). Effect of Emblica officinalis (fruit) against UVB-induced photo-aging in human skin fibroblasts. J. Ethnopharmacol. 132, 109–114.
Afaq, F., Khan, N., Syed, D. N., and Mukhtar, H. (2010). Oral feeding of pomegranate fruit extract inhibits early biomarkers of UVB radiation-induced carcinogenesis in SKH-1 hairless mouse epidermis. Photochem. Photobiol. 86, 1318–1326.
Afaq, F., Zaid, M. A., Khan, N., Dreher, M., and Mukhtar, H. (2009). Protective effect of pomegranate-derived products on UVB-mediated damage in human reconstituted skin. Exp. Dermatol. 18, 553–561.
Akpolat, M., Kanter, M., and Uzal, M. C. (2009). Protective effects of curcumin against gamma radiation-induced ileal mucosal damage. Arch. Toxicol. 83, 609–617.
American Cancer Society. (2007). Global Cancer Facts, and Figures 2007. Atlanta, GA: American Cancer Society.
Amorino, G. P., Mohr, P. J., Hercules, S. K., Pyo, H., and Choy, H. (2000). Combined effects of the orally active cisplatin analog, JM216, and radiation in antitumor therapy. Cancer Chemother. Pharmacol. 46, 423–426.
Arasada, B. L., Bommareddy, A., Zhang, X., Bremmon, K., and Dwivedi, C. (2008). Effects of alpha-santalol on proapoptotic caspases and p53 expression in UVB irradiated mouse skin. Anticancer Res. 28, 129–132.
Aravindan, N., Veeraraghavan, J., Madhusoodhanan, R., Herman, T. S., and Natarajan, M. (2011). Curcumin regulates low-linear energy transfer γ-radiation-induced NFκB-dependent telomerase activity in human neuroblastoma cells. Int. J. Radiat. Oncol. Biol. Phys. 79, 1206–1215.
Arora, R., Gupta, D., Chawla, R., Sagar, R., Sharma, A., and Kumar, R. (2005). Radioprotection by plant products: present status and future prospects. Phytother. Res. 19, 1–22.
Bache, M., Zschornak, M. P., Passin, S., Kessler, J., Wichmann, H., Kappler, M., Paschke, R., Kaluderovic, G. N., Kommera, H., Taubert, H., and Vordermark, D. (2011). Increased betulinic acid induced cytotoxicity and radiosensitivity in glioma cells under hypoxic conditions. Radiat. Oncol. 6, 111.
Bae, J. Y., Choi, J. S., Kang, S. W., Lee, Y. J., Park, J., and Kang, Y. H. (2010). Dietary compound ellagic acid alleviates skin wrinkle and inflammation induced by UV-B irradiation. Exp. Dermatol. 19, e182–e190.
Bakuridze, A. D., Nikolaev, S. M., Berashvili, D. T., Bakuridze, K. A., and Tsomaia, I. V. (2009). Radio protective drug production from fresh leaves of Aloe arborescens mill. Georgian Med. News 171, 80–83.
Baliga, M. S., Bhat, H. P., Pereira, M. M., Mathias, N., and Venkatesh, P. (2010). Radioprotective effects of Aegle marmelos (L.) Correa (Bael): a concise review. J. Altern. Complement. Med. 16, 1109–1116.
Baliga, M. S., and Rao, S. (2010). Radioprotective potential of mint: a brief review. J. Cancer. Res. Ther. 6, 255–262.
Benhar, M., Dalyot, I., Engelberg, D., and Levitzki, A. (2001). Enhanced ROS production in oncogenically transformed cells potentiates c-Jun N-terminal kinase and p38 mitogen-activated protein kinase activation and sensitization to genotoxic stress. Mol. Cell. Biol. 21, 6913–6926.
Bensadoun, R. J., Schubert, M. M., Lalla, R. V., and Keefe, D. (2006). Amifostine in the management of radiation-induced and chemo-induced mucositis. Support. Care Cancer 14, 566–572.
Bhosle, S. M., Ahire, V. R., Henry, M. S., Thakur, V. S., Huilgol, N. G., and Mishra, K. P. (2010). Augmentation of radiation-induced apoptosis by ellagic acid. Cancer Invest. 28, 323–330.
Cao, G., Chen, M., Song, Q., Liu, Y., Xie, L., Han, Y., Liu, Z., Ji, Y., and Jiang, Q. (2012). EGCG protects against UVB-induced apoptosis via oxidative stress and the JNK1/c-Jun pathway in ARPE19 cells. Mol. Med. Report. 5, 54–59.
Carsten, R. E., Bachand, A. M., Bailey, S. M., and Ullrich, R. L. (2008). Resveratrol reduces radiation-induced chromosome aberration frequencies in mouse bone marrow cells. Radiat. Res. 169, 633–638.
Chen, M. L., Li, J., Xiao, W. R., Sun, L., Tang, H., Wang, L., Wu, L. Y., Chen, X., and Xie, H. F. (2006). Protective effect of resveratrol against oxidative damage of UVA irradiated HaCaT cells. Zhong Nan Da Xue Xue Bao Yi Xue Ban 31, 635–639.
Chilampalli, S., Zhang, X., Fahmy, H., Kaushik, R. S., Zeman, D., Hildreth, M. B., and Dwivedi, C. (2010). Chemopreventive effects of honokiol on UVB-induced skin cancer development. Anticancer Res. 30, 777–783.
Choy, H. (2001). Taxanes in combined modality therapy for solid tumors. Crit. Rev. Oncol. Hematol. 37, 237–247.
Cimino, F., Cristani, M., Saija, A., Bonina, F. P., and Virgili, F. (2007). Protective effects of a red orange extract on UVB-induced damage in human keratinocytes. Biofactors 30, 129–138.
Curry, H. A., and Curran, W. J. (2003). “Chemoradiation strategies for patients with malignant gliomas,” in Chemoradiation in Cancer Therapy, ed. H. Choy (Totowa, NJ: Humana Press Inc.), 129.
da Silva, V. V., Ropke, C. D., Miranda, D. V., de Almeida, R. L., Sawada, T. C., Rivelli, D. P., and Barros, S. B. (2009). Photoprotective effect of Pothomorphe umbellata on UVB radiation-induced biomarkers involved in carcinogenesis of hairless mouse epidermis. Cutan. Ocul. Toxicol. 28, 54–60.
Dange, P., Sarma, H., Pandey, B. N., and Mishra, K. P. (2007). Radiation-induced incidence of thymic lymphoma in mice and its prevention by antioxidants. J. Environ. Pathol. Toxicol. Oncol. 26, 273–279.
Dest, V. M. (2006). Radioprotectants: adding quality of life to survivorship? Semin. Oncol. Nurs. 22, 249–256.
Dong, G. Z., Oh, E. T., Lee, H., Park, M. T., Song, C. W., and Park, H. J. (2010a). Beta-lapachone suppresses radiation-induced activation of nuclear factor-kappaB. Exp. Mol. Med. 42, 327–334.
Dong, Y., Bey, E. A., Li, L. S., Kabbani, W., Yan, J., Xie, X. J., Hsieh, J. T., Gao, J., and Boothman, D. A. (2010b). Prostate cancer radiosensitization through poly(ADP-Ribose) polymerase-1 hyperactivation. Cancer Res. 70, 8088–8096.
Droge, W. (2002). Free radicals in the physiological control of cell function. Physiol. Rev. 82, 47–95.
Dujic, J., Kippenberger, S., Ramirez-Bosca, A., Diaz-Alperi, J., Bereiter-Hahn, J., Kaufmann, R., Bernd, A., and Hofmann, M. (2009). Curcumin in combination with visible light inhibits tumor growth in a xenograft tumor model. Int. J. Cancer 124, 1422–1428.
Eder-Czembirek, C., Erovic, B. M., Czembirek, C., Brunner, M., Selzer, E., Pötter, R., and Thurnher, D. (2010). Betulinic acid a radiosensitizer in head and neck squamous cell carcinoma cell lines. Strahlenther. Onkol. 186, 143–148.
Edwards, A., Gladstone, M., Yoon, P., Raben, D., Frederick, B., and Su, T. T. (2011). Combinatorial effect of maytansinol and radiation in Drosophila and human cancer cells. Dis. Models Mech. 4, 496–503.
Fu, H., Lin, M., Katsumura, Y., Yokoya, A., Hata, K., Muroya, Y., Fujii, K., and Shikazono, N. (2010). Protective effects of silybin and analogues against X-ray radiation-induced damage. Acta Biochim. Biophys. Sin. (Shanghai) 42, 489–495.
Garg, A. K., Buchholz, T. A., and Aggarwal, B. B. (2005). Chemosensitization and radiosensitization of tumors by plant polyphenols. Antioxid. Redox Signal. 7, 1630–1647.
Girdhani, S., Bhosle, S. M., Thulasidas, S. A., Kumar, A., and Mishra, K. P. (2005). Potential of radio-sensitizing agents in cancer chemo-radiotherapy. J. Cancer Res. Ther. 1, 129–131.
Grdina, D. J., Murley, J. S., and Kataoka, Y. (2002). Radioprotectants: current status and new directions. Oncology 63, 2–10.
Gu, M., Singh, R. P., Dhanalakshmi, S., Mohan, S., and Agarwal, R. (2006). Differential effect of silibinin on E2F transcription factors and associated biological events in chronically UVB-exposed skin versus tumors in SKH-1 hairless mice. Mol. Cancer Ther. 5, 2121–2129.
Gupta, U., Jahan, S., Chaudhary, R., and Goyal, P. K. (2008). Amelioration of radiation-induced hematological and biochemical alterations by Alstonia scholaris (a medicinal plant) extract. Integr. Cancer Ther. 7, 155–161.
Guruvayoorappan, C., and Kuttan, G. (2008). Protective effect of Biophytum sensitivum (L.) DC on radiation-induced damage in mice. Immunopharmacol. Immunotoxicol. 30, 815–835.
Gutheil, W. G., Reed, G., Ray, A., and Dhar, A. (2011). Crocetin: an agent derived from saffron for prevention and therapy for cancer. Curr. Pharm. Biotechnol. PMID: 21466430. [Epub ahead of print].
Halliwell, B., and Gutteridge, J. M. C. (1989). Free Radicals in Biology and Medicine, 2nd Edn. Oxford: Clarendon Press.
Han, G., Zhou, Y. F., Zhang, M. S., Cao, Z., Xie, C. H., Zhou, F. X., Peng, M., and Zhang, W. J. (2006). Angelica sinensis down-regulates hydroxyproline and Tgfb1 and provides protection in mice with radiation-induced pulmonary fibrosis. Radiat. Res. 165, 546–552.
Hara, T., Omura-Minamisawa, M., Kang, Y., Cheng, C., and Inoue, T. (2008). Flavopiridol potentiates the cytotoxic effects of radiation in radioresistant tumor cells in which p53 is mutated or Bcl-2 is overexpressed. Int. J. Radiat. Oncol. Biol. Phys. 71, 1485–1495.
Harikumar, K. B., and Kuttan, R. (2007). An extract of Phyllanthus amarus protects mouse chromosomes and intestine from radiation induced damages. J. Radiat. Res. 48, 469–476.
Hasselbalch, B., Lassen, U., Hansen, S., Holmberg, M., Sørensen, M., Kosteljanetz, M., Broholm, H., Stockhausen, M. T., and Poulsen, H. S. (2010). Cetuximab, bevacizumab, and irinotecan for patients with primary glioblastoma and progression after radiation therapy and temozolomide: a phase II trial. Neuro-oncology 12, 508–516.
Hazra, B., Das, S. M., Kumar, B., Basu, S., Das, K., Pandey, B. N., and Mishra, K. P. (2007). Cytotoxicity of diospyrin and its derivatives in relation to the generation of reactive oxygen species in tumor cells in vitro and in vivo. Chemotherapy 53, 173–176.
Hiro, J., Inoue, Y., Toiyama, Y., Yoshiyama, S., Tanaka, K., Mohri, Y., Miki, C., and Kusunoki, M. (2010). Possibility of paclitaxel as an alternative radiosensitizer to 5-fluorouracil for colon cancer. Oncol. Rep. 24, 1029–1034.
Hu, J., Chen, L. J., Liu, L., Chen, X., Chen, P. L., Yang, G., Hou, W. L., Tang, M. H., Zhang, F., Wang, X. H., Zhao, X., and Wei, Y. Q. (2008). Liposomal honokiol, a potent anti-angiogenesis agent, in combination with radiotherapy produces a synergistic antitumor efficacy without increasing toxicity. Exp. Mol. Med. 40, 617–628.
Huang, C., Zhang, D., Li, J., Tong, Q., and Stoner, G. D. (2007). Differential inhibition of UV-induced activation of NF kappa B and AP-1 by extracts from black raspberries, strawberries, and blueberries. Nutr. Cancer 58, 205–212.
Huang, J. C., Zhao, P. C., Zhang, H. Z., and Wang, H. (2011). A proteomical study on the radiosensitized target molecules of fuzheng zengxiao formula in pulmonary adenocarcinoma nude mice model. J. Tradit. Chin. Med. 31, 3–6.
Jagetia, G. C. (2007). Radioprotective potential of plants and herbs against the effects of ionizing radiation. J. Clin. Biochem. Nutr. 40, 74–81.
Jagetia, G. C., and Rajanikant, G. K. (2011). Acceleration of wound repair by curcumin in the excision wound of mice exposed to different doses of fractionated γ radiation. Int. Wound J. PMID: 21733986. [Epub ahead of print].
Jagetia, G. C., Shetty, P. C., and Vidyasagar, M. S. (2011). Inhibition of radiation-induced DNA damage by Jamun, Syzygium cumini, in the cultured splenocytes of mice exposed to different doses of gamma-radiation. Integr. Cancer Ther. [Epub ahead of print].
Jagetia, G. C., and Venkatesha, V. A. (2006). Treatment of mice with stem bark extract of Aphanamixis polystachya reduces radiation-induced chromosome damage. Int. J. Radiat. Biol. 82, 197–209.
Jahan, S., and Goyal, P. K. (2010). Protective effect of Alstonia scholaris against radiation-induced clastogenic and biochemical alterations in mice. J. Environ. Pathol. Toxicol. Oncol. 29, 101–111.
Jeong, H. C., Lee, S. Y., Lee, S. Y., Kim, J. H., Shin, C., Shim, J. J., In, K. H., Kang, K. H., and Yoo, S. H. (2006). Phase II study of irinotecan plus cisplatin with concurrent radiotherapy for the patients with limited-disease small-cell lung cancer. Lung Cancer 53, 361–366.
Jeong, S. Y., Park, S. J., Yoon, S. M., Jung, J., Woo, H. N., Yi, S. L., Song, S. Y., Park, H. J., Kim, C., Lee, J. S., Lee, J. S., and Choi, E. K. (2009). Systemic delivery and preclinical evaluation of Au nanoparticle containing beta-lapachone for radiosensitization. J. Control Release. 139, 239–245.
Jin, S. L., Zhou, B. R., and Luo, D. (2009). Protective effect of epigallocatechin gallate on the immune function of dendritic cells after ultraviolet B irradiation. J. Cosmet. Dermatol. 8, 174–180.
Jindal, A., Agrawal, A., and Goyal, P. K. (2010). Influence of Rosemarinus officinalis extract on radiation-induced intestinal injury in mice. J. Environ. Pathol. Toxicol. Oncol. 29, 169–179.
Jindal, A., Soyal, D., Sharma, A., and Goyal, P. K. (2009). Protective effect of an extract of Emblica officinalis against radiation-induced damage in mice. Integr. Cancer Ther. 8, 98–105.
Johnson, G. E., Ivanov, V. N., and Hei, T. K. (2008). Radiosensitization of melanoma cells through combined inhibition of protein regulators of cell survival. Apoptosis 13, 790–802.
Kalthur, G., and Pathirissery, U. D. (2010). Enhancement of the response of B16F1 melanoma to fractionated radiotherapy and prolongation of survival by withaferin A and/or hyperthermia. Integr. Cancer Ther. 9, 370–377.
Kamata, H., Shibukawa, Y., Oka, S. I., and Hirata, H. (2000). Epidermal growth factor receptor is modulated by redox through multiple mechanisms. Effects of reductants and H2O2. Eur. J. Biochem. 267, 1933–1944.
Kao, C. L., Huang, P. I., Tsai, P. H., Tsai, M. L., Lo, J. F., Lee, Y. Y., Chen, Y. J., Chen, Y. W., and Chiou, S. H. (2009). Resveratrol-induced apoptosis and increased radiosensitivity in CD133-positive cells derived from atypical teratoid/rhabdoid tumor. Int. J. Radiat. Oncol. Biol. Phys. 74, 219–228.
Katiyar, S. K., Afaq, F., Azizuddin, K., and Mukhtar, H. (2001). Inhibition of UVB-induced oxidative stress-mediated phosphorylation of mitogen-activated protein kinase signaling pathways in cultured human epidermal keratinocytes by green tea polyphenol (-)-epigallocatechin-3-gallate. Toxicol. Appl. Pharmacol. 176, 110–117.
Katiyar, S. K., Mantena, S. K., and Meeran, S. M. (2011). Silymarin protects epidermal keratinocytes from ultraviolet radiation-induced apoptosis and DNA damage by nucleotide excision repair mechanism. PLoS ONE 6, e21410. doi:10.1371/journal.pone.0021410
Katiyar, S. K., Meleth, S., and Sharma, S. D. (2008). Silymarin, a flavonoid from milk thistle (Silybum marianum L.), inhibits UV-induced oxidative stress through targeting infiltrating CD11b+ cells in mouse skin. Photochem. Photobiol. 84, 266–271.
Kienle, G. S., and Kiene, H. (2010). Review article: influence of Viscum album L (European mistletoe) extracts on quality of life in cancer patients: a systematic review of controlled clinical studies. Integr. Cancer Ther. 9, 142–157.
Kimura, Y., and Sumiyoshi, M. (2009). Olive leaf extract and its main component oleuropein prevent chronic ultraviolet B radiation-induced skin damage and carcinogenesis in hairless mice. J. Nutr. 139, 2079–2086.
Kinsella, T. J. (2011). Radiation oncology: today and tomorrow. Front. Oncol. 1:1–2. doi:10.3389/fonc.2011.00007
Klautke, G., Küchenmeister, U., Foitzik, T., Ludwig, K., Prall, F., Klar, E., and Fietkau, R. (2006). Concurrent chemoradiation with capecitabine and weekly irinotecan as preoperative treatment for rectal cancer: results from a phase I/II study. Br. J. Cancer 94, 976–981.
Kondo, S., Toyokuni, S., Iwasa, Y., Tanaka, T., Onodera, H., and Hiai, H. (1999). Persistent oxidative stress in human colorectal carcinoma, but not in adenoma. Free Radic. Biol. Med. 27, 401–410.
Kumar, B., Joshi, J., Kumar, A., Pandey, B. N., Hazra, B., and Mishra, K. P. (2007). Radiosensitization by diospyrin diethylether in MCF-7 breast carcinoma cell line. Mol. Cell. Biochem. 304, 287–296.
Kumar, B., Kumar, A., Pandey, B. N., Hazra, B., and Mishra, K. P. (2008). Increased cytotoxicity by the combination of radiation and diospyrin diethylether in fibrosarcoma in culture and in tumor. Int. J. Radiat. Biol. 84, 429–440.
Kundu, J. K., Chang, E. J., Fujii, H., Sun, B., and Surh, Y. J. (2008). Oligonol inhibits UVB-induced COX-2 expression in HR-1 hairless mouse skin – AP-1 and C/EBP as potential upstream targets. Photochem. Photobiol. 84, 399–406.
Langer, C. J., Somer, R., Litwin, S., Feigenberg, S., Movsas, B., Maiale, C., Sherman, E., Millenson, M., Nicoloau, N., Huang, C., and Treat, J. (2007). Phase I study of radical thoracic radiation, weekly irinotecan, and cisplatin in locally advanced non-small cell lung carcinoma. J. Thorac. Oncol. 2, 203–209.
Lee, D. E., Lee, K. W., Byun, S., Jung, S. K., Song, N., Lim, S. H., Heo, Y. S., Kim, J. E., Kang, N. J., Kim, B. Y., Bowden, G. T., Bode, A. M., Lee, H. J., and Dong, Z. (2011). 7,3′,4′-Trihydroxyisoflavone, a metabolite of the soy isoflavone daidzein, suppresses ultraviolet B-induced skin cancer by targeting Cot and MKK4. J. Biol. Chem. 286, 14246–14256.
Lee, H. J., Kim, J. S., Moon, C., Kim, J. C., Lee, Y. S., Jang, J. S., Jo, S. K., and Kim, S. H. (2008a). Modification of gamma-radiation response in mice by green tea polyphenols. Phytother. Res. 22, 1380–1383.
Lee, T. K., Wang, W., O’Brien, K. F., Johnke, R. M., Wang, T., Allison, R. R., and Diaz, A. L. (2008b). Effect of North American ginseng on 137Cs-induced micronuclei in human lymphocytes: a comparison with WR-1065. Phytother. Res. 22, 1614–1622.
Lee, H. J., Kim, J. S., Song, M. S., Seo, H. S., Moon, C., Kim, J. C., Jo, S. K., Jang, J. S., and Kim, S. H. (2009). Photoprotective effect of red ginseng against ultraviolet radiation-induced chronic skin damage in the hairless mouse. Phytother. Res. 23, 399–403.
Lee, S. M., Li, M. L., Tse, Y. C., Leung, S. C., Lee, M. M., and Tsui, S. K. (2002). Paeoniae Radix, a Chinese herbal extract, inhibit hepatoma cells growth by inducing apoptosis in a p53 independent pathway. Life Sci. 71, 2267–2277.
Lee, T. K., O’Brien, K. F., Wang, W., Johnke, R. M., Sheng, C., Benhabib, S. M., Wang, T., and Allison, R. R. (2010). Radioprotective effect of American ginseng on human lymphocytes at 90 minutes postirradiation: a study of 40 cases. J. Altern. Complement. Med. 16, 561–567.
Li, L. H., Wu, L. J., Tashiro, S. I., Onodera, S., Uchiumi, F., and Ikejima, T. (2007). Activation of the SIRT1 pathway and modulation of the cell cycle were involved in silymarin’s protection against UV-induced A375-S2 cell apoptosis. J. Asian Nat. Prod. Res. 9, 245–252.
Li, L. J., Lai-Fu, Z., Li-Ping, J., Cheng-Yan, G., and Li-Juan, Z. (2011). β-Elemene Radiosensitizes Lung Cancer A549 Cells by Enhancing DNA Damage and Inhibiting DNA Repair. Phytother. Res. 25, 1095–1097.
Lillo, O., Bracesco, N., and Nunes, E. (2011). Lethal and mutagenic interactions between γ-rays, cisplatin and etoposide at the cellular and molecular levels. Int. J. Radiat. Biol. 87, 222–230.
Liu, Y., Chan, F., Sun, H., Yan, J., Fan, D., Zhao, D., An, J., and Zhou, D. (2011). Resveratrol protects human keratinocytes HaCaT cells from UVA-induced oxidative stress damage by downregulating Keap1 expression. Eur. J. Pharmacol. 650, 130–137.
Loo, G. (2003). Redox-sensitive mechanisms of phytochemical-mediated inhibition of cancer cell proliferation. J. Nutr. Biochem. 14, 64–73.
Madhusoodhanan, R., Natarajan, M., Singh, J. V., Jamgade, A., Awasthi, V., Anant, S., Herman, T. S., and Aravindan, N. (2010). Effect of black raspberry extract in inhibiting NFkappa B dependent radioprotection in human breast cancer cells. Nutr. Cancer 62, 93–104.
Mahmood, J., Jelveh, S., Calveley, V., Zaidi, A., Doctrow, S. R., and Hill, R. P. (2011). Mitigation of radiation-induced lung injury by genistein and EUK-207. Int. J. Radiat. Biol. 87, 889–901.
Majeed, M., Bhat, B., Anand, S., Sivakumar, A., Paliwal, P., and Geetha, K. G. (2011). Inhibition of UV-induced ROS and collagen damage by Phyllanthus emblica extract in normal human dermal fibroblasts. J. Cosmet. Sci. 62, 49–56.
Manna, S. K., Mukhopadhyay, A., and Aggarwal, B. B. (2000). Resveratrol suppresses TNF-induced activation of nuclear transcription factors NF-kappa B, activator protein-1, and apoptosis: potential role of reactive oxygen intermediates and lipid peroxidation. J. Immunol. 164, 6509–6519.
Manu, K. A., Leyon, P. V., and Kuttan, G. (2007). Studies on the protective effects of Boerhaavia diffusa L. against gamma radiation induced damage in mice. Integr. Cancer. Ther. 6, 381–388.
McLaughlin, N., Annabi, B., Lachambre, M. P., Kim, K. S., Bahary, J. P., Moumdjian, R., and Béliveau, R. (2006). Combined low dose ionizing radiation and green tea-derived epigallocatechin-3-gallate treatment induces human brain endothelial cells death. J. Neurooncol. 80, 111–121.
Meeran, S. M., Katiyar, S., Elmets, C. A., and Katiyar, S. K. (2006). Silymarin inhibits UV radiation-induced immunosuppression through augmentation of interleukin-12 in mice. Mol. Cancer Ther. 5, 1660–1668.
Meyer, M., Pahl, H. L., and Baeuerle, P. A. (1994). Regulation of the transcription factors NF-kappa B and AP-1 by redox changes. Chem. Biol. Interact. 91, 91–100.
Mouria, M., Gukovskaya, A. S., Jung, Y., Buechler, P., Hines, O. J., and Reber, H. A. (2002). Food-derived polyphenols inhibit pancreatic cancer growth through mitochondrial cytochrome c release and apoptosis. Int. J. Cancer 98, 761–769.
Muller, J. M., Rupec, R. A., and Baeuerle, P. A. (1997). Study of gene regulation by NF-kappa B and AP-1 in response to reactive oxygen intermediates. Methods 11, 301–312.
Murley, J. S., Kataoka, Y., Cao, D., Li, J. J., Oberley, L. W., and Grdina, D. J. (2004). Delayed radioprotection by NFkappaB-mediated induction of Sod2 (MnSOD) in SA-NH tumor cells after exposure to clinically used thiol-containing drugs. Radiat. Res. 162, 536–546.
Nair, S., Nair, R. R., Srinivas, P., Srinivas, G., and Pillai, M. R. (2008). Radiosensitizing effects of plumbagin in cervical cancer cells is through modulation of apoptotic pathway. Mol. Carcinog. 47, 22–33.
Navarro, M., Dotor, E., Rivera, F., Sánchez-Rovira, P., Vega-Villegas, M. E., Cervantes, A., García, J. L., Gallén, M., and Aranda, E. (2006). A Phase II study of preoperative radiotherapy and concomitant weekly irinotecan in combination with protracted venous infusion 5-fluorouracil, for resectable locally advanced rectal cancer. Int. J. Radiat. Oncol. Biol. Phys. 66, 201–205.
Newcomb, E. W., Lymberis, S. C., Lukyanov, Y., Shao, Y., Schnee, T., Devitt, M., Rosenstein, B. S., Zagzag, D., and Formenti, S. C. (2006). Radiation sensitivity of GL261 murine glioma model and enhanced radiation response by flavopiridol. Cell Cycle 5, 93–99.
Ng, A. K., Bernardo, M. V., Weller, E., Backstrand, K., Silver, B., Marcus, K. C., Tarbell, N. J., Stevenson, M. A., Friedberg, J. W., and Mauch, P. M. (2002). Second malignancy after Hodgkin disease treated with radiation therapy with or without chemotherapy: long-term risks and risk factors. Blood 100, 1989–1996.
Oehler, C., Dickinson, D. J., Broggini-Tenzer, A., Hofstetter, B., Hollenstein, A., Riesterer, O., Vuong, V., and Pruschy, M. (2007). Current concepts for the combined treatment modality of ionizing radiation with anticancer agents. Curr. Pharm. Des. 13, 519–535.
Okunieff, P., Xu, J., Hu, D., Liu, W., Zhang, L., Morrow, G., Pentland, A., Ryan, J. L., and Ding, I. (2006). Curcumin protects against radiation-induced acute and chronic cutaneous toxicity in mice and decreases mRNA expression of inflammatory and fibrogenic cytokines. Int. J. Radiat. Oncol. Biol. Phys. 65, 890–898.
Orditura, M., Galizia, G., Napolitano, V., Martinelli, E., Pacelli, R., Lieto, E., Aurilio, G., Vecchione, L., Morgillo, F., Catalano, G., Ciardiello, F., Del Genio, A., Di Martino, N., and De Vita, F. (2010). Weekly chemotherapy with cisplatin and paclitaxel and concurrent radiation therapy as preoperative treatment in locally advanced esophageal cancer: a phase II study. Cancer Invest. 28, 820–827.
Ozgur, E., Güler, G., and Seyhan, N. (2010). Mobile phone radiation-induced free radical damage in the liver is inhibited by the antioxidants N-acetyl cysteine and epigallocatechin-gallate. Int. J. Radiat. Biol. 86, 935–945.
Pacheco-Palencia, L. A., Noratto, G., Hingorani, L., Talcott, S. T., and Mertens-Talcott, S. U. (2008). Protective effects of standardized pomegranate (Punica granatum L.) polyphenolic extract in ultraviolet-irradiated human skin fibroblasts. J. Agric. Food Chem. 56, 8434–8441.
Park, E., Hwang, I., Song, J. Y., and Jee, Y. (2011). Acidic polysaccharide of Panax ginseng as a defense against small intestinal damage by whole-body gamma irradiation of mice. Acta Histochem. 113, 19–23.
Park, H. R., Ju, E. J., Jo, S. K., Jung, U., and Kim, S. H. (2010). HemoHIM enhances the therapeutic efficacy of ionizing radiation treatment in tumor-bearing mice. J. Med. Food 13, 47–53.
Park, K., and Lee, J. H. (2008). Protective effects of resveratrol on UVB-irradiated HaCaT cells through attenuation of the caspase pathway. Oncol. Rep. 19, 413–417.
Patt, H. M., Tyree, E. B., Straube, R. L., and Smith, D. E. (1949). Cysteine protection against X-irradiation. Science 110, 213–214.
Prasad, K. N. (2005). Rationale for using multiple antioxidants in protecting humans against low doses of ionizing radiation. Br. J. Radiol. 78, 485–492.
Raffoul, J. J., Banerjee, S., Che, M., Knoll, Z. E., Doerge, D. R., Abrams, J., Kucuk, O., Sarkar, F. H., and Hillman, G. G. (2007). Soy isoflavones enhance radiotherapy in a metastatic prostate cancer model. Int. J. Cancer 120, 2491–2498.
Raffoul, J. J., Wang, Y., Kucuk, O., Forman, J. D., Sarkar, F. H., and Hillman, G. G. (2006). Genistein inhibits radiation-induced activation of NF-kappa B in prostate cancer cells promoting apoptosis and G2/M cell cycle arrest. BMC Cancer 6, 107. doi:10.1186/1471-2407-6-107
Raju, U., Ariga, H., Koto, M., Lu, X., Pickett, J., Valdecanas, D., Mason, K. A., and Milas, L. (2006). Improvement of esophageal adenocarcinoma cell and xenograft responses to radiation by targeting cyclin-dependent kinases. Radiother. Oncol. 80, 185–191.
Rao, B. N., Archana, P. R., Aithal, B. K., and Rao, B. S. (2011). Protective effect of zingerone, a dietary compound against radiation induced genetic damage and apoptosis in human lymphocytes. Eur. J. Pharmacol. 657, 59–66.
Rao, B. N., and Rao, B. S. (2010a). Antagonistic effects of Zingerone, a phenolic alkanone against radiation-induced cytotoxicity, genotoxicity, apoptosis and oxidative stress in Chinese hamster lung fibroblast cells growing in vitro. Mutagenesis 25, 577–587.
Rao, B. N., Rao, B. S., Aithal, B. K., and Kumar, M. R. (2009). Radiomodifying and anticlastogenic effect of Zingerone on Swiss albino mice exposed to whole body gamma radiation. Mutat. Res. 677, 33–41.
Rao, S. K., and Rao, P. S. (2010b). Alteration in the radiosensitivity of HeLa cells by dichloromethane extract of guduchi (Tinospora cordifolia). Integr. Cancer Ther. 9, 378–384.
Rigano, D., Cardile, V., Formisano, C., Maldini, M. T., Piacente, S., Bevelacqua, Y., Russo, A., and Senatore, F. (2009). Genista sessilifolia DC. and Genista tinctoria L. inhibit UV light and nitric oxide-induced DNA damage and human melanoma cell growth. Chem. Biol. Interact. 180, 211–219.
Rosenberg, A., and Knox, S. (2006). Radiation sensitization with redox modulators: a promising approach. Int. J. Radiat. Oncol. Biol. Phys. 64, 343–354.
Roy, P., Madan, E., Kalra, N., Nigam, N., George, J., Ray, R. S., Hans, R. K., Prasad, S., and Shukla, Y. (2009). Resveratrol enhances ultraviolet B-induced cell death through nuclear factor-kappaB pathway in human epidermoid carcinoma A431 cells. Biochem. Biophys. Res. Commun. 384, 215–220.
Samarth, R. M. (2007). Protection against radiation induced hematopoietic damage in bone marrow of Swiss albino mice by Mentha piperita (Linn). J. Radiat. Res. 48, 523–528.
Sandhya, T., Lathika, K. M., Pandey, B. N., Bhilwade, H. N., Chaubey, R. C., Priyadarsini, K. I., and Mishra, K. P. (2006). Protection against radiation oxidative damage in mice by Triphala. Mutat. Res. 609, 17–25.
Sato, T., Kokuba, Y., Koizumi, W., Hayakawa, K., Okayasu, I., and Watanabe, M. (2007). Phase I trial of neoadjuvant preoperative chemotherapy with S-1 and irinotecan plus radiation in patients with locally advanced rectal cancer. Int. J. Radiat. Oncol. Biol. Phys. 69, 1442–1447.
Scarlatti, F., Sala, G., Ricci, C., Maioli, C., Milani, F., Minella, M., Botturi, M., and Ghidoni, R. (2007). Resveratrol sensitization of DU145 prostate cancer cells to ionizing radiation is associated to ceramide increase. Cancer Lett. 253, 124–130.
Schwarzenberger, P., Fariss, A., Linares, L., Nedzi, L., and Salazar, O. M. (2011). Dose escalation of once weekly oral vinorelbine concurrent with weekly split dose hypofractionated chest radiation for palliation of advanced non-small cell lung cancer: a phase I/II study. Am. J. Med. Sci. 341, 454–459.
Sharma, K. V., and Sisodia, R. (2009). Evaluation of the free radical scavenging activity and radioprotective efficacy of Grewia asiatica fruit. J. Radiol. Prot. 29, 429–443.
Sharma, P., Parmar, J., Sharma, P., Verma, P., and Goyal, P. K. (2011). Radiation-induced testicular injury and its amelioration by Tinospora cordifolia (An Indian Medicinal Plant) Extract. Evid. Based Complement. Alternat. Med. 2011, 643847.
Sheehan, J., Ionescu, A., Pouratian, N., Hamilton, D. K., Schlesinger, D., Oskouian, R. J. Jr., and Sansur, C. (2008). Use of trans sodium crocetinate for sensitizing glioblastoma multiforme to radiation: laboratory investigation. J. Neurosurg. 108, 972–978.
Shimada, M., Kato, A., Habu, T., and Komatsu, K. (2011). Genistein, isoflavonoids in soybeans, prevents the formation of excess radiation-induced centrosomes via p21 up-regulation. Mutat Res. 716, 27–32.
Shin, J. I., Shim, J. H., Kim, K. H, Choi, H. S., Kim, J. W., Lee, H. G., Kim, B. Y., Park, S. N., Park, O. J., and Yoon, D. Y. (2008). Sensitization of the apoptotic effect of gamma-irradiation in genistein-pretreated CaSki cervical cancer cells. J. Microbiol. Biotechnol. 18, 523–531.
Shin, S. J., Kim, N. K., Keum, K. C., Kim, H. G., Im, J. S., Choi, H. J., Baik, S. H., Choen, J. H., Jeung, H. C., Rha, S. Y., Roh, J. K., Chung, H. C., and Ahn, J. B. (2010). Phase II study of preoperative chemoradiotherapy (CRT) with irinotecan plus S-1 in locally advanced rectal cancer. Radiother. Oncol. 95, 303–307.
Simone, C. B., Simone, N. L., Simone, V., and Simone, C. B. (2007). Antioxidants and other nutrients do not interfere with chemotherapy or radiation therapy and can increase kill and increase survival, Part 2. Altern. Ther. Health Med. 13, 40–47.
Singh-Gupta, V., Zhang, H., Yunker, C. K., Ahmad, Z., Zwier, D., Sarkar, F. H., and Hillman, G. G. (2010). Daidzein effect on hormone refractory prostate cancer in vitro and in vivo compared to genistein and soy extract: potentiation of radiotherapy. Pharm. Res. 27, 1115–1127.
Sinha, M., Das, D. K., Bhattacharjee, S., Majumdar, S., and Dey, S. (2011). Leaf extract of Moringa oleifera prevents ionizing radiation-induced oxidative stress in mice. J. Med. Food 14, 1167–1172.
Sisodia, R., Singh, S., Sharma, K. V., and Ahaskar, M. (2008). Post treatment effect of Grewia asiatica against radiation-induced biochemical alterations in Swiss albino mice. J. Environ. Pathol. Toxicol. Oncol. 27, 113–121.
Son, E. W., Mo, S. J., Rhee, D. K., and Pyo, S. (2006). Inhibition of ICAM-1 expression by garlic component, allicin, in gamma-irradiated human vascular endothelial cells via downregulation of the JNK signaling pathway. Int. Immunopharmacol. 6, 1788–1795.
Srinivasan, M., Rajendra, P. N., and Menon, V. P. (2006). Protective effect of curcumin on gamma-radiation induced DNA damage and lipid peroxidation in cultured human lymphocytes. Mutat. Res. 611, 96–103.
Staniforth, V., Huang, W. C., Aravindaram, K., and Yang, N. S. (2011). Ferulic acid, a phenolic phytochemical, inhibits UVB-induced matrix metalloproteinases in mouse skin via posttranslational mechanisms. J. Nutr. Biochem. PMID: 21543204. [Epub ahead of print].
Stone, H. B., Coleman, C. N., Anscher, M. S., and McBride, W. H. (2003). Effects of radiation on normal tissue: consequences and mechanisms. Lancet Oncol. 4, 529–536.
Suresh, K., and Vasudevan, D. M. (1994). Augmentation of murine natural killer cell and antibody dependent cellular cytotoxicity activities by Phyllanthus emblica, a new immunomodulator. J. Ethnopharmacol. 44, 55–60.
Suzuki, M., Amano, M., Choi, J., Park, H. J., Williams, B. W., Ono, K., and Song, C. W. (2006). Synergistic effects of radiation and beta-lapachone in DU-145 human prostate cancer cells in vitro. Radiat. Res. 165, 525–531.
Svobodová, A., Zdarilová, A., Malisková, J., Mikulková, H., Walterová, D., and Vostalová, J. (2007). Attenuation of UVA-induced damage to human keratinocytes by silymarin. J. Dermatol. Sci. 46, 21–30.
Szatrowski, T. P., and Nathan, C. F. (1991). Production of large amounts of hydrogen peroxide by human tumor cells. Cancer Res. 51, 794–798.
Toyokuni, S., Okamoto, K., Yodoi, J., and Hiai, H. (1995). Persistent oxidative stress in cancer. FEBS Lett. 358, 1–3.
Uma Devi, P., Utsumiz, H., Takata, M., and Takeda, S. (2008). Enhancement of radiation induced cell death in chicken B lymphocytes by withaferin A. Indian J. Exp. Biol. 46, 437–442.
Vaid, M., and Katiyar, S. K. (2010). Molecular mechanisms of inhibition of photocarcinogenesis by silymarin, a phytochemical from milk thistle (Silybum marianum L. Gaertn.). Int. J. Oncol. 36, 1053–1060.
Veeraraghavan, J., Aravindan, S., Natarajan, M., Awasthi, V., Herman, T. S., and Aravindan, N. (2011). Neem leaf extract induces radiosensitization in human neuroblastoma xenograft through modulation of apoptotic pathway. Anticancer Res. 31, 161–170.
Venkatachalam, S. R., and Chattopadhyay, S. (2005). Natural radioprotective agents: an overview, Curr. Org. Chem. 9, 389–404.
Verma, S., Younus, J., Stys-Norman, D., Haynes, A. E., and Blackstein, M. (2007). Ifosfamide-based combination chemotherapy in advanced soft-tissue sarcoma: a practice guideline. Curr. Oncol. 14, 144–148.
Wang, Y. N., Wu, W., Chen, H. C., and Fang, H. (2010). Genistein protects against UVB-induced senescence-like characteristics in human dermal fibroblast by p66Shc down-regulation. J. Dermatol. Sci. 58, 19–27.
Weiss, J. F., and Landauer, M. R. (2000). Radioprotection by antioxidants. Ann. N. Y. Acad. Sci. 899, 44–60.
World Cancer Report. (2008). eds P. Boyle and B. E. Levin (Lyon: IARC Press). Available at: http://www.iarc.fr/en/publications/pdfs-online/wcr/2008/wcr_2008.pdf
Xie, C. H., Zhang, M. S., Zhou, Y. F., Han, G., Cao, Z., Zhou, F. X., Zhang, G., Luo, Z. G., Wu, J. P., Liu, H., Chen, J., and Zhang, W. J. (2006). Chinese medicine Angelica sinensis suppresses radiation-induced expression of TNF-alpha and TGF-beta1 in mice. Oncol. Rep. 15, 1429–1436.
Yang, E. S., Choi, M. J., Kim, J. H., Choi, K. S., and Kwon, T. K. (2011a). Withaferin A enhances radiation-induced apoptosis in Caki cells through induction of reactive oxygen species, Bcl-2 downregulation and Akt inhibition. Chem. Biol. Interact. 190, 9–15.
Yang, E. S., Choi, M. J., Kim, J. H., Choi, K. S., and Kwon, T. K. (2011b). Combination of withaferin A and X-ray irradiation enhances apoptosis in U937 cells. Toxicol. In vitro. 25, 1803–1810.
Yang, Y. P., Chang, Y. L., Huang, P. I., Chiou, G. Y., Tseng, L. M., Chiou, S. H., Chen, M. H., Chen, M. T., Shih, Y. H., Chang, C. H., Hsu, C. C., Ma, H. I., Wang, C. T., Tsai, L. L., Yu, C. C., and Chang, C. J. (2011). Resveratrol suppresses tumorigenicity and enhances radiosensitivity in primary glioblastoma tumor initiating cells by inhibiting the STAT3 axis. J. Cell. Physiol. doi:10.1002/jcp.22806. [Epub ahead of print].
Yoon, J. H., Lim, T. G., Lee, K. M., Jeon, A. J., Kim, S. Y., and Lee, K. W. (2011). Tangeretin reduces ultraviolet B (UVB)-induced cyclooxygenase-2 expression in mouse epidermal cells by blocking mitogen-activated protein kinase (MAPK) activation and reactive oxygen species (ROS) generation. J. Agric. Food Chem. 59, 222–228.
You, W. C., Hsieh, C. C., and Huang, J. T. (2009a). Effect of extracts from indigowood root (Isatis indigotica Fort.) on immune responses in radiation-induced mucositis. J. Altern. Complement. Med. 15, 771–778.
You, W. C., Lin, W. C., Huang, J. T., and Hsieh, C. C. (2009b). Indigowood root extract protects hematopoietic cells, reduces tissue damage and modulates inflammatory cytokines after total-body irradiation: does Indirubin play a role in radioprotection? Phytomedicine 16, 1105–1111.
Yu, R., Hebbar, V., Kim, D. W., Mandlekar, S., Pezzuto, J. M., and Kong, A. N. (2001). Resveratrol inhibits phorbol ester and UV-induced activator protein 1 activation by interfering with mitogen-activated protein kinase pathways. Mol. Pharmacol. 60, 217–224.
Keywords: medicinal plants, phytochemicals, radioprotectors, radiosensitizers, cancer radiotherapy, ionizing radiation
Citation: Hazra B, Ghosh S, Kumar A and Pandey BN (2012) The prospective role of plant products in radiotherapy of cancer: a current overview. Front. Pharmacol. 2:94. doi: 10.3389/fphar.2011.00094
Received: 04 November 2011;
Accepted: 22 December 2011;
Published online: 09 January 2012.
Edited by:
Xue-Jun Sun, Taishan Medical College, ChinaReviewed by:
Wenwu Liu, Second Military Medical University, ChinaSheng Chen, Zhejiang University, China
Copyright: © 2012 Hazra, Ghosh, Kumar and Pandey. This is an open-access article distributed under the terms of the Creative Commons Attribution Non Commercial License, which permits non-commercial use, distribution, and reproduction in other forums, provided the original authors and source are credited.
*Correspondence: Banasri Hazra, Department of Pharmaceutical Technology, Jadavpur University, Kolkata 700 032, India. e-mail: banasrihazra@yahoo.co.in