- 1 Center for Diagnostic Nanosystems, Marshall University, Huntington, WV, USA
- 2 Department of Biological Sciences, Marshall University, Huntington, WV, USA
- 3 Department of Exercise Science, Sport and Recreation, College of Education and Human Services, Marshall University, Huntington, WV, USA
- 4 Department of Pharmacology, Physiology and Toxicology, Marshall University, Huntington, WV, USA
- 5 Department of Cardiology, Marshall University, Huntington, WV, USA
- 6 Department of Internal Medicine, Joan C. Edwards School of Medicine, Marshall University, Huntington, WV, USA
Acetaminophen, also known as APAP or paracetamol, is one of the most widely used analgesics (pain reliever) and antipyretics (fever reducer). According to the U.S. Food and Drug Administration, currently there are 235 approved prescription and over-the-counter drug products containing acetaminophen as an active ingredient. When used as directed, acetaminophen is very safe and effective; however when taken in excess or ingested with alcohol hepatotoxicity and irreversible liver damage can arise. In addition to well known use pain relief and fever reduction, recent laboratory and pre-clinical studies have demonstrated that acetaminophen may also have beneficial effects on blood glucose levels, skeletal muscle function, and potential use as cardioprotective and neuroprotective agents. Extensive laboratory and pre-clinical studies have revealed that these off-label applications may be derived from the ability of acetaminophen to function as an antioxidant. Herein, we will highlight these novel applications of acetaminophen, and attempt, where possible, to highlight how these findings may lead to new directions of inquiry and clinical relevance of other disorders.
Introduction
Acetaminophen (N-Acetyl-4-aminophenol) also known as “APAP” or “paracetamol” is one of the most widely used medicines in the United States. According to the data from IMS Health, ∼24.6 billion doses of acetaminophen were sold in 2008. The market demand for acetaminophen is growing. Recent data has demonstrated a 28% growth in the market sales of acetaminophen between 2004 and 2008 with sales approaching approximately $2.6 billion in 2008 alone (Data from IMS Health).
Acetaminophen exhibits both analgesic and antipyretic properties and has been widely used as an active ingredient in many approved drugs. According to the U.S. Food and Drug Administration (FDA), 479 drugs contain acetaminophen (Table 1). As of August 2011, 235 out of the 479 acetaminophen-containing drugs exhibit active approval status, which include 214 prescription drug products owned by 31 companies and 21 over-the-counter (OTC) drug products produced by nine companies (Tables 1 and 2). Given its wide use and easy availability, scientists have recently begun to examine acetaminophen for off-label applications. Herein, we will investigate these potential applications, and attempt, where possible, to highlight how these findings may lead to new directions of inquiry and clinical relevance of other disorders.
Chronic Acetaminophen Ingestion Can Improve Blood Glucose Control
Although a toxic dose of acetaminophen (3 h following 500 mg APAP/kg body weight) can rapidly induce hyperglycemia (Hinson et al., 1984) and acute liver failure secondary to clinical acetaminophen overdose can further impair the peripheral uptake of glucose (Clark et al., 2001), recent animal studies have demonstrated that acetaminophen, when taken at lower dosages (20–30 mg/kg body weight) exhibits an ability to lower blood glucose levels in several animal disease models, including diabetes, high-fat (HF) diet induced obesity, and aging.
Using a streptozotocin (STZ)-induced diabetic mice model, Shertzer et al. (2008) showed that acetaminophen at 20 mg/kg body weight is able to normalize STZ-induced increases in blood glucose levels. This effect appears to be associated with protection against STZ-induced destruction of pancreatic beta-cells given the finding that acetaminophen injection appears to be associated with the maintenance of pancreatic insulin synthesis (Shertzer et al., 2008). Like that observed in the STZ model, acetaminophen at 20 or 30 mg/kg body weight has also been reported to prevent hyperglycemia in animals fed a HF diet (Shertzer et al., 2008, 2010). In addition to preventing hyperglycemia, acetaminophen ingestion is also associated with the restoration of fasting insulin levels, improvements in glucose tolerance, and an increased ability of the HF-fed mice to boost blood insulin levels after a glucose challenge (Shertzer et al., 2008, 2010). Although the exact mechanism(s) remains unclear, these effects, like that seen in the rat STZ model, have been postulated to arise from improvements in pancreatic insulin synthesis/secretion.
Research using both humans and animal models have demonstrated that the incidence of insulin resistance increases with age (Houmard et al., 1995; Wu et al., 2009a). Wu et al. (2009a) using Fisher 344 × Brown Norway (F344BN) aging rat model have shown that age-associated increases in blood glucose can be corrected by chronic acetaminophen treatment at the dosage of 30 mg/kg body weight. It has been postulated that this effect was predicated, at least in part, on the ability of acetaminophen to diminish age-related losses in amount of muscle glucose transporter-4 (Glut4) expression (Wu et al., 2009a). Further study demonstrated that acetaminophen treatment was also associated with an attenuation of muscle reactive oxygen species (ROS) levels and in the amount of proteins that become oxidatively modified (Wu et al., 2009a). It has been shown that prolonged mitogen activated protein kinase (MAPK) activation is associated with decreased Glut4 expression (Fujishiro et al., 2001; Carlson et al., 2003) and Glut4 translocation in response to insulin (Bandyopadhyay et al., 2001; Izawa et al., 2005; D’Alessandris et al., 2007). Interestingly, works done by Wu et al. (2009a) showed that acetaminophen treatment could normalize the marked increases in p38- and ERK–MAPK activation seen in aged muscle. Taken together, these findings suggests that acetaminophen might function to improve blood glucose levels by employing multiple mechanisms including decreases in intracellular ROS levels, diminished aging-associated MAPK hyperphosphorylation and by increasing muscle Glut4 expression (Wu et al., 2009a).
Long Term Acetaminophen Ingestion Can Improve Skeletal Muscle Structure and Function
In addition to the beneficial effect of acetaminophen on relieving muscle soreness and pain (Prior et al., 2011), recent studies have suggested that acetaminophen can improve aged skeletal muscle structure and function (sarcopenia). Wu et al. (2009b) firstly reported that chronic acetaminophen treatment at 30 mg/kg body weight is able to decrease the amount of aging-associated myocyte apoptosis and increase myocyte size (muscle fiber cross sectional area), with this latter effect occurring most likely associated with an increase in myosin and actin expression in aged muscle. These effects are believed to be mediated, at least in part, via reductions in the amount of oxidative and nitrosative stress as acetaminophen intervention lowers the amount of superoxide and the abundance of oxidatively modified proteins (Wu et al., 2009a). It also appears that acetaminophen treatment reduces the phosphorylation of eukaryotic initiation factor 2a (eIF2a; Wu et al., 2010), a key protein translational factor which when phosphorylated in response to stress leads to the inhibition of protein translational initiation (Kimball, 1999). Other data has demonstrated that acetaminophen can decrease muscle reactive nitrogen species (RNS) as evidenced by reduced expression of inducible NOS (iNOS) and S-nitrosylation of Akt (Wu et al., 2009b). The Akt/protein kinase B is critical regulator of cellular homeostasis and functions to control cellular anabolism (protein synthesis, glucose uptake, and metabolism) and cell fate (proliferation, apoptosis; Wu et al., 2011). Akt S-nitrosylation impairs Akt kinase activity (Carvalho-Filho et al., 2005; Yasukawa et al., 2005; Wu et al., 2009b), which if not corrected can lead to a dysregulation of Akt signaling. Importantly, the restoration of Akt function by acetaminophen is associated with improvements in protein translational signaling [increased phosphorylation/activation of S6 ribosomal protein (Ser235/236) and translation initiation factor eIF4E (Ser209)], increases in the amount of muscle Glut4, myosin, and actin, along with a decrease in myocyte apoptosis and the prevention of age-associated hyperglycemia (Wu et al., 2009a,b, 2010).
The positive effects of acetaminophen on skeletal muscle structure and function have also been proved in other experimental sitting. Shertzer et al. (2008) reported that acetaminophen is able to prevent HF diet induced decreases in lean muscle volume and body water retention. Trappe et al. (2011) demonstrated during 12 weeks of knee extensor progressive resistance exercise training that acetaminophen (4 g/day) can increase exercise-induced muscle (quadriceps) hypertrophy and strength in older adults when compared to that seen with placebo, although muscle (vastus lateralis) protein content, muscle water content, and myosin heavy chain distribution did not increase. Interestingly, increased muscle volume and strength by acetaminophen appears not to be mediated by cyclooxygenase (COX), as the expression of COX-1 and COX-2 was not changed with acetaminophen consumption (Trappe et al., 2011). Importantly, acetaminophen at 4 g/day for 12 weeks when used in combination with resistance training did not alter blood creatinine and ALT levels (Trappe et al., 2011), a finding which suggests that this dosage does not cause liver or kidney damage. Interestingly, the effect of acetaminophen and exercise combination appears dependent on type of exercise as acetaminophen has been reported to suppress the protein synthesis response in skeletal muscle after eccentric resistance exercise (Trappe et al., 2002), which appears to be mediated through decreasing exercise-induced PGF(2alpha) expression and hence affecting the anabolic response of muscle to eccentric resistance exercise (Trappe et al., 2001). Whether a similar finding exists for other types of exercise modalities (e.g., aerobic) is currently unclear.
Acetaminophen Possesses Cardioprotective and Neuroprotective Properties
Clinical studies have suggested that the risk for major cardiovascular events will be increased if acetaminophen is taken frequently (≥22 days/month) or at high doses (15 tablets/week; Chan et al., 2006). Nonetheless, when used properly, acetaminophen has been shown to exhibit cardioprotective effects. Using an isolated guinea pig heart model, several studies have shown that acetaminophen (0.35 mM) can increase coronary vascular resistance and positive inotropy (Merrill et al., 2001), attenuate ischemia-reduced monophasic action potentials (Merrill and Goldberg, 2001), and improve left ventricular contractility (rate of developed pressure) during post ischemia–reperfusion (Merrill et al., 2001; Merrill, 2002). Further examination using electron microscopy has suggested that acetaminophen can preserve left ventricular myofibrillar ultrastructure in the reperfused myocardium, including protection against ischemia/reperfusion-induced diffused and blurred Z lines, the presence of contraction bands, and swollen, sparsely packed mitochondria (Merrill et al., 2001). Using two dog models of ventricular arrhythmias induced by regional myocardial ischemia/reperfusion or ouabain (25 μg/kg), Merrill et al. (2007) demonstratedan anti-arrhythmic effect for acetaminophen, and found that acetaminophen (15 mg/kg, i.v.) significantly reduce the number of ventricular ectopic beats during ischemia and reperfusion, the amount of ouabain-induced ventricular premature beats, ventricular salvo, ventricular bigeminy, and non-sustained ventricular arrhythmia. In the iron-overloaded gerbil, Walker et al. (2007) have demonstrated that acetaminophen is able to prevent iron overload-induced cardiac structural and functional changes, including alterations in cardiac rhythm, ventricular distension, reductions in left ventricular ejection fraction, decreases in fractional shortening, and decreases in mortality (Walker et al., 2009). Mauger et al. (2010) reported that ingestion of acetaminophen (1.5 g) can improve the performance of a 10-mile cycle time trial (TT) with no difference in exertion or perceived pain, and that cyclists who ingested acetaminophen had a higher mean power output and heart rate. Merrill et al. (2004) using a myocardial infarction dog model showed that acetaminophen at 30 mg/kg body weight is able to decrease infarct size. These researchers also showed acetaminophen treatment can reduce cardiac damage, including swollen mitochondria and fragmented nucleus (Merrill et al., 2004). Conversely, others using different animal models did not show beneficial effects on infarct size in non-preconditioned rats (Dai and Kloner, 2003) or in coronary artery occlusion/reperfusion rabbits (Hale and Kloner, 2004), however all suggest that acetaminophen is a safe drug in the postmyocardial infarction setting (Dai and Kloner, 2003; Hale and Kloner, 2004; Leshnower et al., 2006). Further studies defining detailed conditions are needed to verify the protective effect of acetaminophen on infarct size.
It has also been reported that acetaminophen has neuroprotective effects. Maharaj et al. (2004) reported that acetaminophen (0.25–1 mM) treatment ex vivo can inhibit cyanide-induced superoxide anion generation and lipid peroxidation in rat brain homogenates. Further animal study has suggested the acetaminophen (100 mg/kg/day, i.p.) can inhibit quinolinic acid (QA)-induced lipid peroxidation, superoxide anion generation, and cell damage in the rat hippocampus (Maharaj et al., 2006). Naziroglu et al. (2009) also reported acetaminophen (5–100 mg/kg) can reduce brain and microsomal lipid peroxidation, while it also increases brain vitamin E levels and microsomal glutathione peroxidase (GSH-Px) activity. In addition, Bisaglia et al. (2002) used rat primary hippocampal neurons and rat pheochromocytoma cells demonstrated that acetaminophen (100 μM) can protect against amyloid beta-fragment-induced impairment of mitochondrial redox activity, increases in phospholipid peroxidation, and apoptotic nuclear fragmentation, suggesting a possible therapeutic effect of acetaminophen on Alzheimer’s disease.
Acetaminophen Exhibits Potent Antioxidant Activity
It is well known that acetaminophen overdose can lead to oxidative stress and induce hepatic and renal damage (Ghosh et al., 2010; Agarwal et al., 2011). Acetaminophen is initially metabolized in the liver, and generates the toxic metabolite, N-acetyl-p-benzoquinone imine (NAPQI). NAPQI can be efficiently detoxified by glutathione (GSH) which is an important cellular antioxidant for detoxification of drugs and foreign chemicals. When overdosed with acetaminophen, intracellular glutathione can be depleted within 1–4 h (Al-Turk and Stohs, 1981; Porubek et al., 1987; Lores Arnaiz et al., 1995), resulting in accumulation of intracellular reactive oxygen and nitrogen species (ROS/RNS), and oxidative/nitrosative stress will occur. The over-produced NAPQI can covalently bind to the cysteinyl thiol groups of cellular proteins and form protein-(cystein-S-yl)-APAP adducts (Hoffmann et al., 1985), which may impair protein function. Given this mechanism, antioxidant therapy using N-acetylcysteine (NAC) is commonly used to attenuate acetaminophen-induced hepatotoxicity.
Interestingly, extensive animal and in vitro studies have suggested that acetaminophen possesses remarkable antioxidant properties when used within the therapeutic dosage. Acetaminophen is phenolic in structure with a substituent at the para position relative to the hydroxyl group (Figure 1) which allows it to react with reactive species (Dinis et al., 1994; Shertzer et al., 2008). For example, Shertzer et al. (2008) using cell-free assay systems demonstrated that acetaminophen at a concentrations of 2–10 μM is able to directly scavenge reactive oxygen. Nam et al. (2009) found that acetaminophen has higher reactivity with peroxyl radicals than many widely used phenolic antioxidants, including ubiquitous butylated hydroxytoluene (BHT). Other in vitro studies showed that acetaminophen can significantly inhibit hemoprotein-induced lipid peroxidation by its ability to reducing ferryl heme to its ferric state and the quenching globin radicals (Boutaud et al., 2010). Acetaminophen has also be shown to reduce the degree of low-density lipoproteins (LDL) hydroperoxides induced by Cu2+ ions (Ozsoy and Pabuccuoglu, 2007) or myeloperoxidase in presence of nitrite and hydrogen peroxide (Chou and Greenspan, 2002). Several studies have also demonstrated that acetaminophen can directly scavenge peroxynitrite (Van Dyke et al., 1998; Rork et al., 2006; Schildknecht et al., 2008), a highly reactive oxidant and nitrating agent that can oxidize lipids, proteins, and nucleic acids. Nam et al. (2009) showed that when nitrogen is incorporated into the phenolic ring the antioxidant activity of acetaminophen is greatly reduced and that this finding seems to be associated with increased O–H bond dissociation enthalpy. However, this structural change increases the efficacy of acetaminophen to act as an inhibitor of lipid hydroperoxide biosynthesis by soybean lipoxygenases-1 (sLOX-1) (Nam et al., 2009) and further evidences suggested that altered acidity of the phenolic O–H may lead to chelation of the catalytic non-heme iron atom in sLOX-1 (Nam et al., 2009). Taken together, it appears that the structure of the acetaminophen phenolic ring is critical for its pharmacological and antioxidant properties.
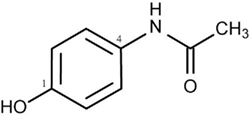
Figure 1. Structure of acetaminophen (N-Acetyl-4-aminophenol). The phenolic structure with a substituent at the para position relative to the hydroxyl group allows acetaminophen to react with reactive species and possesses antioxidant activity.
Ex vivo and in vivo animal studies have suggested that acetaminophen can effectively reduce ROS/RNS in multiple tissue types. Back in 1983, DuBois et al. (1983) reported that acetaminophen had antioxidant effects in the rat liver. Shertzer et al. (2008) also showed that acetaminophen (20 mg/kg) can decrease liver mitochondrial H2O2 formation in both control and HF diet fed mice. Wu et al. (2009a,b, 2010) demonstrated that acetaminophen treatment at 30 mg/kg for 6 months can attenuate aging-increased skeletal muscle ROS content, the amount of proteins that are oxidatively modified, and protein S-nitrosylation, suggesting acetaminophen can decrease oxidative/nitrosative stress during aging. Using a rhabdomyolysis-induced renal failure animal model, Boutaud et al. (2010) showed that acetaminophen (100 mg/kg, i.p.) can significantly decrease myoglobin-derived radical species and that this finding was associated with reduced renal damage and improved renal function. Usinga HF fed animal model, Shertzer et al. (2010) demonstrated that acetaminophen (30 mg/kg/day) was able to inhibit production of reactive oxygen species (at least partially via inhibition of NADPH oxidase activity) and lipid peroxidation in white adipose tissue (WAT) which improved glucose tolerance and insulin sensitivity in HF animals.
Although not yet well understood, the cardioprotective effects of acetaminophen seem to relate to its antioxidant property (Merrill and Goldberg, 2001; Merrill et al., 2001; Merrill, 2002; Rork et al., 2006; Hadzimichalis et al., 2007; Walker et al., 2007, 2009; Kakarla et al., 2010). Using Langendorff-perfused guinea pig hearts, Hadzimichalis et al. (2007) reported that acetaminophen (0.35 mM) can reduce mitochondrial swelling, and inhibit the mitochondrial permeability transition pore-induced apoptotic pathway and mitochondrial cytochrome C release in heart with induced low-flow global myocardial ischemia. Merrill et al. (2001) demonstrated that acetaminophen can significantly attenuate the burst of hydroxyl radicals during the first 10 min of reperfusion, and block 3-morpholinosydnominine (SIN-1)-induced peroxynitrite generation. Kakarla et al. (2010) have found that chronic acetaminophen treatment at 30 mg/kg body weight is able to attenuate aging-associated increases in cardiac oxidative (superoxide) and nitrosative (protein nitrotyrosylation) stresses, caspase-3 activation, and apoptosis in F344BN rats. Rork et al. (2006) reported that acetaminophen (0.35 mM) can reduce peroxynitrite in the isolated guinea pig myocardium and that this finding was associated with an attenuated activation of MMP-2 and decreased cleavage of troponin I (TnI) following ischemia/reperfusion. Therefore, cardioprotective effects of acetaminophen are at least partially mediated by reducing tissue reactive oxygen and nitrogen species.
Conclusion and Perspectives
Recent experimental data suggests that acetaminophen may have several remarkable effects other than its well known analgesic/antipyretic properties. Thus far, acetaminophen has been shown to improve blood glucose control, improve skeletal muscle structure and function in the aged, and that this agent exhibits cardioprotective and neuroprotective effects (Figure 2). Current laboratory and pre-clinical studies have revealed that many of these findings can be linked to its incredible antioxidant properties. It is also worth noting that since acetaminophen overdose or ingestion with alcohol can cause hepatotoxicity and death, well controlled clinical studies must be conducted to ensure the safety and efficiency of acetaminophen before its clinical application for off-label application.
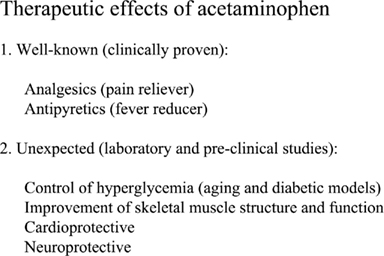
Figure 2. Summary of acetaminophen therapeutic effects. In addition to the clinically proven analgesic/antipyretic properties, laboratory and pre-clinical studies demonstrated that acetaminophen has other beneficial effects that would increase clinical application of acetaminophen. However, clinical studies are needed to ensure its safety, efficiency, and proper dosage.
Conflict of Interest Statement
The authors declare that the research was conducted in the absence of any commercial or financial relationships that could be construed as a potential conflict of interest.
Acknowledgments
The authors would like to acknowledge the support of the Huntington VA Medical Center for laboratory space and equipment.
References
Agarwal, R., Macmillan-Crow, L. A., Rafferty, T. M., Saba, H., Roberts, D. W., Fifer, E. K., James, L. P., and Hinson, J. A. (2011). Acetaminophen-induced hepatotoxicity in mice occurs with inhibition of activity and nitration of mitochondrial manganese superoxide dismutase. J. Pharmacol. Exp. Ther. 337, 110–116.
Al-Turk, W. A., and Stohs, S. J. (1981). Hepatic glutathione content and aryl hydrocarbon hydroxylase activity of acetaminophen-treated mice as a function of age. Drug Chem. Toxicol. 4, 37–48.
Bandyopadhyay, G., Sajan, M. P., Kanoh, Y., Standaert, M. L., Quon, M. J., Reed, B. C., Dikic, I., and Farese, R. V. (2001). Glucose activates protein kinase C-zeta/lambda through proline-rich tyrosine kinase-2, extracellular signal-regulated kinase, and phospholipase D: a novel mechanism for activating glucose transporter translocation. J. Biol. Chem. 276, 35537–35545.
Bisaglia, M., Venezia, V., Piccioli, P., Stanzione, S., Porcile, C., Russo, C., Mancini, F., Milanese, C., and Schettini, G. (2002). Acetaminophen protects hippocampal neurons and PC12 cultures from amyloid beta-peptides induced oxidative stress and reduces NF-kappaB activation. Neurochem. Int. 41, 43–54.
Boutaud, O., Moore, K. P., Reeder, B. J., Harry, D., Howie, A. J., Wang, S., Carney, C. K., Masterson, T. S., Amin, T., Wright, D. W., Wilson, M. T., Oates, J. A., and Roberts, L. J. II. (2010). Acetaminophen inhibits hemoprotein-catalyzed lipid peroxidation and attenuates rhabdomyolysis-induced renal failure. Proc. Natl. Acad. Sci. U.S.A. 107, 2699–2704.
Carlson, C. J., Koterski, S., Sciotti, R. J., Poccard, G. B., and Rondinone, C. M. (2003). Enhanced basal activation of mitogen-activated protein kinases in adipocytes from type 2 diabetes: potential role of p38 in the downregulation of GLUT4 expression. Diabetes 52, 634–641.
Carvalho-Filho, M. A., Ueno, M., Hirabara, S. M., Seabra, A. B., Carvalheira, J. B., De Oliveira, M. G., Velloso, L. A., Curi, R., and Saad, M. J. (2005). S-nitrosation of the insulin receptor, insulin receptor substrate 1, and protein kinase B/Akt: a novel mechanism of insulin resistance. Diabetes 54, 959–967.
Chan, A. T., Manson, J. E., Albert, C. M., Chae, C. U., Rexrode, K. M., Curhan, G. C., Rimm, E. B., Willett, W. C., and Fuchs, C. S. (2006). Nonsteroidal antiinflammatory drugs, acetaminophen, and the risk of cardiovascular events. Circulation 113, 1578–1587.
Chou, T. M., and Greenspan, P. (2002). Effect of acetaminophen on the myeloperoxidase-hydrogen peroxide-nitrite mediated oxidation of LDL. Biochim. Biophys. Acta 1581, 57–63.
Clark, S. J., Shojaee-Moradie, F., Croos, P., Seed, P. T., Umpleby, A. M., Wendon, J. A., and Miell, J. (2001). Temporal changes in insulin sensitivity following the development of acute liver failure secondary to acetaminophen. Hepatology 34, 109–115.
Dai, W., and Kloner, R. A. (2003). Effects of acetaminophen on myocardial infarct size in rats. J. Cardiovasc. Pharmacol. Ther. 8, 277–284.
D’Alessandris, C., Lauro, R., Presta, I., and Sesti, G. (2007). C-reactive protein induces phosphorylation of insulin receptor substrate-1 on Ser307 and Ser 612 in L6 myocytes, thereby impairing the insulin signalling pathway that promotes glucose transport. Diabetologia 50, 840–849.
Dinis, T. C., Maderia, V. M., and Almeida, L. M. (1994). Action of phenolic derivatives (acetaminophen, salicylate, and 5-aminosalicylate) as inhibitors of membrane lipid peroxidation and as peroxyl radical scavengers. Arch. Biochem. Biophys. 315, 161–169.
DuBois, R. N., Hill, K. E., and Burk, R. F. (1983). Antioxidant effect of acetaminophen in rat liver. Biochem. Pharmacol. 32, 2621–2622.
Fujishiro, M., Gotoh, Y., Katagiri, H., Sakoda, H., Ogihara, T., Anai, M., Onishi, Y., Ono, H., Funaki, M., Inukai, K., Fukushima, Y., Kikuchi, M., Oka, Y., and Asano, T. (2001). MKK6/3 and p38 MAPK pathway activation is not necessary for insulin-induced glucose uptake but regulates glucose transporter expression. J. Biol. Chem. 276, 19800–19806.
Ghosh, J., Das, J., Manna, P., and Sil, P. C. (2010). Acetaminophen induced renal injury via oxidative stress and TNF-alpha production: therapeutic potential of arjunolic acid. Toxicology 268, 8–18.
Hadzimichalis, N. M., Baliga, S. S., Golfetti, R., Jaques, K. M., Firestein, B. L., and Merrill, G. F. (2007). Acetaminophen-mediated cardioprotection via inhibition of the mitochondrial permeability transition pore-induced apoptotic pathway. Am. J. Physiol. Heart Circ. Physiol. 293, H3348–H3355.
Hale, S. L., and Kloner, R. A. (2004). Acetaminophen and experimental acute myocardial infarction. Cardiovasc. Drugs Ther. 18, 121–125.
Hinson, J. A., Han-Hsu, H., Mays, J. B., Holt, S. J., Mclean, P., and Ketterer, B. (1984). Acetaminophen-induced alterations in blood glucose and blood insulin levels in mice. Res. Commun. Chem. Pathol. Pharmacol. 43, 381–391.
Hoffmann, K. J., Streeter, A. J., Axworthy, D. B., and Baillie, T. A. (1985). Identification of the major covalent adduct formed in vitro and in vivo between acetaminophen and mouse liver proteins. Mol. Pharmacol. 27, 566–573.
Houmard, J. A., Weidner, M. D., Dolan, P. L., Leggett-Frazier, N., Gavigan, K. E., Hickey, M. S., Tyndall, G. L., Zheng, D., Alshami, A., and Dohm, G. L. (1995). Skeletal muscle GLUT4 protein concentration and aging in humans. Diabetes 44, 555–560.
Izawa, Y., Yoshizumi, M., Fujita, Y., Ali, N., Kanematsu, Y., Ishizawa, K., Tsuchiya, K., Obata, T., Ebina, Y., Tomita, S., and Tamaki, T. (2005). ERK1/2 activation by angiotensin II inhibits insulin-induced glucose uptake in vascular smooth muscle cells. Exp. Cell Res. 308, 291–299.
Kakarla, S. K., Fannin, J. C., Keshavarzian, S., Katta, A., Paturi, S., Nalabotu, S. K., Wu, M., Rice, K. M., Manzoor, K., Walker, E. M. Jr., and Blough, E. R. (2010). Chronic acetaminophen attenuates age-associated increases in cardiac ROS and apoptosis in the Fischer Brown Norway rat. Basic Res. Cardiol. 105, 535–544.
Leshnower, B. G., Sakamoto, H., Zeeshan, A., Parish, L. M., Hinmon, R., Plappert, T., Jackson, B. M., Gorman, J. H. III., and Gorman, R. C. (2006). Role of acetaminophen in acute myocardial infarction. Am. J. Physiol. Heart Circ. Physiol. 290, H2424–H2431.
Lores Arnaiz, S., Llesuy, S., Cutrin, J. C., and Boveris, A. (1995). Oxidative stress by acute acetaminophen administration in mouse liver. Free Radic. Biol. Med. 19, 303–310.
Maharaj, D. S., Saravanan, K. S., Maharaj, H., Mohanakumar, K. P., and Daya, S. (2004). Acetaminophen and aspirin inhibit superoxide anion generation and lipid peroxidation, and protect against 1-methyl-4-phenyl pyridinium-induced dopaminergic neurotoxicity in rats. Neurochem. Int. 44, 355–360.
Maharaj, H., Maharaj, D. S., and Daya, S. (2006). Acetylsalicylic acid and acetaminophen protect against oxidative neurotoxicity. Metab. Brain Dis. 21, 189–199.
Mauger, A. R., Jones, A. M., and Williams, C. A. (2010). Influence of acetaminophen on performance during time trial cycling. J. Appl. Physiol. 108, 98–104.
Merrill, G., Mcconnell, P., Vandyke, K., and Powell, S. (2001). Coronary and myocardial effects of acetaminophen: protection during ischemia-reperfusion. Am. J. Physiol. Heart Circ. Physiol. 280, H2631–H2638.
Merrill, G. F. (2002). Acetaminophen and low-flow myocardial ischemia: efficacy and antioxidant mechanisms. Am. J. Physiol. Heart Circ. Physiol. 282, H1341–H1349.
Merrill, G. F., and Goldberg, E. (2001). Antioxidant properties of acetaminophen and cardioprotection. Basic Res. Cardiol. 96, 423–430.
Merrill, G. F., Merrill, J. H., Golfetti, R., Jaques, K. M., Hadzimichalis, N. S., Baliga, S. S., and Rork, T. H. (2007). Antiarrhythmic properties of acetaminophen in the dog. Exp. Biol. Med. (Maywood) 232, 1245–1252.
Merrill, G. F., Rork, T. H., Spiler, N. M., and Golfetti, R. (2004). Acetaminophen and myocardial infarction in dogs. Am. J. Physiol. Heart Circ. Physiol. 287, H1913–H1920.
Nam, T. G., Nara, S. J., Zagol-Ikapitte, I., Cooper, T., Valgimigli, L., Oates, J. A., Porter, N. A., Boutaud, O., and Pratt, D. A. (2009). Pyridine and pyrimidine analogs of acetaminophen as inhibitors of lipid peroxidation and cyclooxygenase and lipoxygenase catalysis. Org. Biomol. Chem. 7, 5103–5112.
Naziroglu, M., Uguz, A. C., Kocak, A., and Bal, R. (2009). Acetaminophen at different doses protects brain microsomal Ca2+-ATPase and the antioxidant redox system in rats. J. Membr. Biol. 231, 57–64.
Ozsoy, M. B., and Pabuccuoglu, A. (2007). The effect of acetaminophen on oxidative modification of low-density lipoproteins in hypercholesterolemic rabbits. J. Clin. Biochem. Nutr. 41, 27–31.
Porubek, D. J., Rundgren, M., Harvison, P. J., Nelson, S. D., and Moldeus, P. (1987). Investigation of mechanisms of acetaminophen toxicity in isolated rat hepatocytes with the acetaminophen analogues 3,5-dimethylacetaminophen and 2,6-dimethylacetaminophen. Mol. Pharmacol. 31, 647–653.
Prior, M. J., Lavins, B. J., and Cooper, K. (2011). A randomized, placebo-controlled trial of acetaminophen extended release for treatment of post-marathon muscle soreness. Clin. J. Pain. PMID: 21760499. [Epub ahead of print].
Rork, T. H., Hadzimichalis, N. M., Kappil, M. A., and Merrill, G. F. (2006). Acetaminophen attenuates peroxynitrite-activated matrix metalloproteinase-2-mediated troponin I cleavage in the isolated guinea pig myocardium. J. Mol. Cell. Cardiol. 40, 553–561.
Schildknecht, S., Daiber, A., Ghisla, S., Cohen, R. A., and Bachschmid, M. M. (2008). Acetaminophen inhibits prostanoid synthesis by scavenging the PGHS-activator peroxynitrite. FASEB J. 22, 215–224.
Shertzer, H. G., Kendig, E. L., Nasrallah, H. A., Johansson, E., and Genter, M. B. (2010). Protection from olanzapine-induced metabolic toxicity in mice by acetaminophen and tetrahydroindenoindole. Int. J. Obes. (Lond.) 34, 970–979.
Shertzer, H. G., Schneider, S. N., Kendig, E. L., Clegg, D. J., D’alessio, D. A., and Genter, M. B. (2008). Acetaminophen normalizes glucose homeostasis in mouse models for diabetes. Biochem. Pharmacol. 75, 1402–1410.
Trappe, T. A., Carroll, C. C., Dickinson, J. M., Lemoine, J. K., Haus, J. M., Sullivan, B. E., Lee, J. D., Jemiolo, B., Weinheimer, E. M., and Hollon, C. J. (2011). Influence of acetaminophen and ibuprofen on skeletal muscle adaptations to resistance exercise in older adults. Am. J. Physiol. Regul. Integr. Comp. Physiol. 300, R655–R662.
Trappe, T. A., Fluckey, J. D., White, F., Lambert, C. P., and Evans, W. J. (2001). Skeletal muscle PGF(2)(alpha) and PGE(2) in response to eccentric resistance exercise: influence of ibuprofen acetaminophen. J. Clin. Endocrinol. Metab. 86, 5067–5070.
Trappe, T. A., White, F., Lambert, C. P., Cesar, D., Hellerstein, M., and Evans, W. J. (2002). Effect of ibuprofen and acetaminophen on postexercise muscle protein synthesis. Am. J. Physiol. Endocrinol. Metab. 282, E551–E556.
Van Dyke, K., Sacks, M., and Qazi, N. (1998). A new screening method to detect water-soluble antioxidants: acetaminophen (Tylenol) and other phenols react as antioxidants and destroy peroxynitrite-based luminol-dependent chemiluminescence. J. Biolumin. Chemilumin. 13, 339–348.
Walker, E. M. Jr., Epling, C. P., Parris, C., Cansino, S., Ghosh, P., Desai, D. H., Morrison, R. G., Wright, G. L., Wehner, P., Mangiarua, E. I., Walker, S. M., and Blough, E. R. (2007). Acetaminophen protects against iron-induced cardiac damage in gerbils. Ann. Clin. Lab. Sci. 37, 22–33.
Walker, E. M. Jr., Morrison, R. G., Dornon, L., Laurino, J. P., Walker, S. M., Studeny, M., Wehner, P. S., Rice, K. M., Wu, M., and Blough, E. R. (2009). Acetaminophen combinations protect against iron-induced cardiac damage in gerbils. Ann. Clin. Lab. Sci. 39, 378–385.
Wu, M., Desai, D. H., Kakarla, S. K., Katta, A., Paturi, S., Gutta, A. K., Rice, K. M., Walker, E. M. Jr., and Blough, E. R. (2009a). Acetaminophen prevents aging-associated hyperglycemia in aged rats: effect of aging-associated hyperactivation of p38-MAPK and ERK1/2. Diabetes Metab. Res. Rev. 25, 279–286.
Wu, M., Katta, A., Gadde, M. K., Liu, H., Kakarla, S. K., Fannin, J., Paturi, S., Arvapalli, R. K., Rice, K. M., Wang, Y., and Blough, E. R. (2009b). Aging-associated dysfunction of akt/protein kinase B: s-nitrosylation and acetaminophen intervention. PLoS ONE 4, e6430. doi: 10.1371/journal.pone.0006430
Wu, M., Falasca, M., and Blough, E. R. (2011). Akt/protein kinase B in skeletal muscle physiology and pathology. J. Cell. Physiol. 226, 29–36.
Wu, M., Liu, H., Fannin, J., Katta, A., Wang, Y., Arvapalli, R. K., Paturi, S., Karkala, S. K., Rice, K. M., and Blough, E. R. (2010). Acetaminophen improves protein translational signaling in aged skeletal muscle. Rejuvenation Res. 13, 571–579.
Keywords: acetaminophen, antioxidant, hyperglycemia, skeletal muscle, cardiac protection
Citation: Blough ER and Wu M (2011) Acetaminophen: beyond pain and fever-relieving. Front. Pharmacol. 2:72. doi: 10.3389/fphar.2011.00072
Received: 19 September 2011; Paper pending published: 10 October 2011;
Accepted: 24 October 2011; Published online: 09 November 2011.
Edited by:
Mauro Martins Teixeira, Universidade Federal de Minas Gerais, BrazilReviewed by:
Thiago Mattar Cunha, School of Medicine of Ribeirao Preto University of Sao Paulo, BrazilMarco AurélioMartins, Oswaldo Cruz Foundation, Brazil
Copyright: © 2011 Blough and Wu. This is an open-access article subject to a non-exclusive license between the authors and Frontiers Media SA, which permits use, distribution and reproduction in other forums, provided the original authors and source are credited and other Frontiers conditions are complied with.
*Correspondence: Miaozong Wu, Translational Research Division, Center for Diagnostic Nano systems, Marshall University, Room 217 BBSC, 1700 3rd Avenue, Huntington, WV 25755, USA. e-mail:d3VtQG1hcnNoYWxsLmVkdQ==