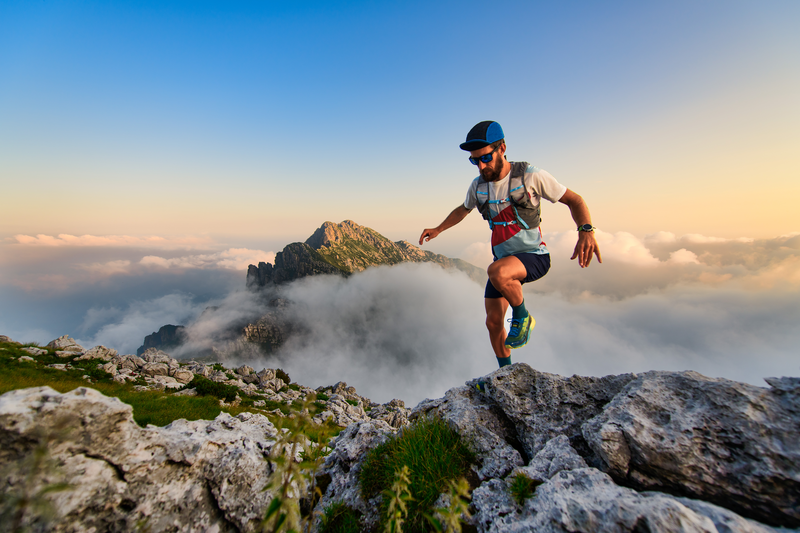
95% of researchers rate our articles as excellent or good
Learn more about the work of our research integrity team to safeguard the quality of each article we publish.
Find out more
ORIGINAL RESEARCH article
Front. Pediatr.
Sec. Pediatric Neurology
Volume 13 - 2025 | doi: 10.3389/fped.2025.1571426
This article is part of the Research Topic New Insights into Pediatric Neurology: Neurological Disorders and Epileptic Encephalopathies View all 3 articles
The final, formatted version of the article will be published soon.
You have multiple emails registered with Frontiers:
Please enter your email address:
If you already have an account, please login
You don't have a Frontiers account ? You can register here
BackgroundBiallelic variants in NARS2 that encodes the mitochondrial asparaginyl-tRNA synthetase are associated with a wide spectrum of clinical phenotypes. Herein, we report on two siblings carrying the same compound heterozygous missense variants in NARS2, to improve the understanding of the phenotypic heterogeneity of NARS2 variants.Case presentationThe two probands, a 3-year-old female (Patient 1) and a 16-month-old male (Patient 2), were clinically suspected of Combined oxidative phosphorylation deficiency 24 (COXPD24). Both presented with neurological manifestations, including refractory epilepsy, developmental delay and motor developmental regression, within the first year of life, accompanied by symmetrical brain lesions identified on magnetic resonance imaging (MRI). To elucidate the underlying genetic etiology, whole-exome sequencing (WES) was performed, followed by Sanger sequencing validation in the patients and their non-consanguineous parents. Genetic analysis revealed that both probands harbored identical compound heterozygous variants in the NARS2 gene: c.1253G>A (p.Arg418His) and c.1163C>T (p.Thr388Met). Notably, the c.1163C>T (p.Thr388Met) variant in NARS2 represents a novel finding, further expanding the genetic spectrum associated with this disorder.ConclusionsOur findings expand the mutational spectrum of NARS2 and highlight the associated phenotypic heterogeneity, underscoring the critical role of NARS2 in epilepsy and neurodevelopmental processes. For pediatric patients with refractory epilepsy, early genetic testing is essential to improve diagnostic accuracy, refine prognostic stratification, and guide personalized treatment strategies. Additionally, mitochondrial drug cocktail therapy may be beneficial for epilepsy caused by NARS2 mutations.
Keywords: Combined oxidative phosphorylation deficiency 24 (COXPD24), NARS2, refractory epilepsy, biallelic variants, pediatric, mitochondrial drug cocktail therapy
Received: 05 Feb 2025; Accepted: 24 Mar 2025.
Copyright: © 2025 Wu, Zhou, Ye, Chen, Lin, Wang, Nie and Zhang. This is an open-access article distributed under the terms of the Creative Commons Attribution License (CC BY). The use, distribution or reproduction in other forums is permitted, provided the original author(s) or licensor are credited and that the original publication in this journal is cited, in accordance with accepted academic practice. No use, distribution or reproduction is permitted which does not comply with these terms.
* Correspondence:
Xing Nie, Department of Pediatric Intensive Care Unit, The Seventh Affiliated Hospital,Sun Yat-sen University, Shenzhen, China
Lidan Zhang, Department of Pediatric Intensive Care Unit, The Seventh Affiliated Hospital,Sun Yat-sen University, Shenzhen, China
Disclaimer: All claims expressed in this article are solely those of the authors and do not necessarily represent those of their affiliated organizations, or those of the publisher, the editors and the reviewers. Any product that may be evaluated in this article or claim that may be made by its manufacturer is not guaranteed or endorsed by the publisher.
Research integrity at Frontiers
Learn more about the work of our research integrity team to safeguard the quality of each article we publish.