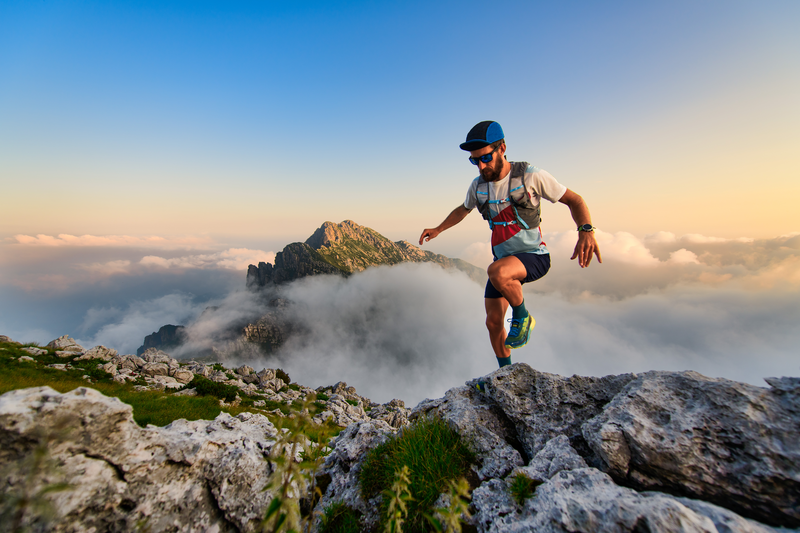
94% of researchers rate our articles as excellent or good
Learn more about the work of our research integrity team to safeguard the quality of each article we publish.
Find out more
ORIGINAL RESEARCH article
Front. Pediatr.
Sec. Pediatric Cardiology
Volume 13 - 2025 | doi: 10.3389/fped.2025.1528490
This article is part of the Research Topic Surgical and Non-Surgical Intervention of Congenital Heart Disease Management in Developing and Developed Countries View all 8 articles
The final, formatted version of the article will be published soon.
You have multiple emails registered with Frontiers:
Please enter your email address:
If you already have an account, please login
You don't have a Frontiers account ? You can register here
Transcatheter device closure of ventricular septal defects (VSDs) offers an appealing and effective alternative to surgical repair. The Lifetech™ Konar-Multifunctional Occluder (MFO) VSD occluder has gained increasing application due to its versatility and promising outcomes.We aim to evaluate our experience with the MFO device for VSD closure.We conducted a prospective analysis of clinical data from 151 patients who underwent percutaneous closure of muscular and perimembranous VSDs using the MFO device at our institution between November 2018 and September 2023.Comprehensive assessments of safety and procedural outcomes were performed.The patient's mean age was 55.4±51.6 months (range, 6 months to 31 years), and the mean weight was 17.6±11.9 kg (range, 5-86). Among the patients, 94 (62.3%) had perimembranous defects, while the remaining had muscular VSDs. The mean defect diameter was 4.8±1.5 mm (range, 2-10). The retrograde approach was applied in 133 patients (88.7%). Device implantation was successful in 98.7% of patients. One procedure (0.7%) failed due to device migration, requiring surgical retrieval and VSD closure, and another patient with a significant residual shunt needed placement of an additional device in another session. The mean procedure time was 44±2 minutes, with a mean fluoroscopy time of 12.8±7.7 minutes. The mean follow-up duration was 11±9.7 months (range, 6-35). Non-significant shunts were found in 32 patients (21.2%). Newly acquired valve regurgitation was observed in 16 patients (10.6%), including 11 patients (7.3%) with trivial-to-mild aortic regurgitation and 5(3.3%) with moderate-to-severe tricuspid regurgitation. Electrophysiological adverse events occurred in 5 patients (3.3%), including nodal rhythm (n=3, 2%), intermittent heart block (n=1, 0.7%), and severe bradycardia (n=1, 0.7%). Vascular complications were documented in 13 patients (8.6%) including one developing chronic vascular occlusion.Percutaneous VSD closure with the MFO device is a safe, effective, and feasible procedure via both antegrade and retrograde approaches.
Keywords: VSD, percutaneous VSD closure, konar MF, Ventricular septal defect, Perimembranous VSD, Muscular VSD
Received: 14 Nov 2024; Accepted: 14 Mar 2025.
Copyright: © 2025 Afifi, Kotit, Shehata, Deyaa, Ramadan and Tawfik. This is an open-access article distributed under the terms of the Creative Commons Attribution License (CC BY). The use, distribution or reproduction in other forums is permitted, provided the original author(s) or licensor are credited and that the original publication in this journal is cited, in accordance with accepted academic practice. No use, distribution or reproduction is permitted which does not comply with these terms.
* Correspondence:
Susy Kotit, Magdi Yacoub Heart Foundation-Aswan Heart Centre, Cairo, Egypt
Disclaimer: All claims expressed in this article are solely those of the authors and do not necessarily represent those of their affiliated organizations, or those of the publisher, the editors and the reviewers. Any product that may be evaluated in this article or claim that may be made by its manufacturer is not guaranteed or endorsed by the publisher.
Research integrity at Frontiers
Learn more about the work of our research integrity team to safeguard the quality of each article we publish.