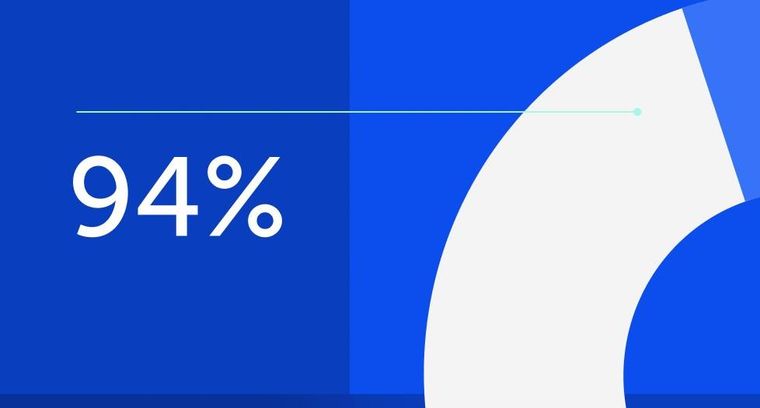
94% of researchers rate our articles as excellent or good
Learn more about the work of our research integrity team to safeguard the quality of each article we publish.
Find out more
ORIGINAL RESEARCH article
Front. Pediatr., 28 February 2025
Sec. Pediatric Surgery
Volume 13 - 2025 | https://doi.org/10.3389/fped.2025.1527880
This article is part of the Research TopicAdvances and Challenges in Neonatal Surgery: Congenital and Acquired ConditionsView all 5 articles
Background: With increased survival of infants born with esophageal atresia (EA), there is a knowledge gap regarding neurodevelopmental outcomes. We aimed to quantify the frequency of (1) documented developmental delay, and (2) implementation of early intervention services in the first and the second year of life following repair of short- and long-gap EA.
Method: We retrospectively analyzed term-born (n = 44) and premature infants (n = 26) following EA repair at a single institution (2009–2020). Infants with anomalies associated with known neurological disorders were excluded. Clinical data was obtained from the electronic medical record, and presented as means and percentages. Developmental delay included clinically documented motor, speech/language, and cognitive delays that were stratified according to a surgical group: short- and long-gap EA.
Results: Nearly half of short-gap (24/54; 44%) and most of long-gap EA patients (12/16; 75%) had documented developmental delay in the first year of life that persisted into the second year of life [52% [28/54] short-gap; 69% [11/16] long-gap EA]. Developmental delay was noted irrespective of gestational age at birth, co-existing cardiac anomalies, or presence of cranial/brain findings on imaging. By age 2, 70% (38/54) of short-gap and 69% (11/16) of long-gap EA patients had received early intervention.
Interpretation: Infants born with EA are at high-risk for developmental delay. Early neurodevelopmental assessments and intervention is recommended for EA patients.
• Evidence that esophageal atresia (EA) may be associated with impaired neurodevelopmental outcomes.
• Half of short-gap and most of long-gap EA had early developmental delay.
• Neurodevelopmental delay in EA is not associated with gestational age at birth.
• Most patients born with EA received early intervention by 2-years of age.
• Early implementation of baseline neurodevelopmental and brain imaging assessments should be considered.
Esophageal atresia (EA), although a rare congenital anomaly, is one of the most common gastrointestinal birth defects (1) with a worldwide prevalence of 1 in 2,500 to 1 in 4,500 live births (2). In addition to anatomical classification in relation to presence and location of tracheoesophageal fistula(e) (types A–D), EA can also be classified as short-gap or long-gap EA based on the esophageal gap length which determines the duration and complexity of perioperative critical care (3). Infants born with a long-gap EA (gap length >3 cm or >2 vertebral bodies in length) (4) undergo multiple surgeries and more complex perioperative care. At our institution, repair of long-gap EA involves tension-induced esophageal growth over a period of several days to weeks as part of the staged Foker process (5, 6). The Foker process involves at least two separate thoracic operations to allow for esophageal growth using either external (5) or, more recently, internal traction (7). As a result, infants undergoing long-gap EA repair require prolonged sedation (5 days or more), which is known to be associated with development of tolerance and physical dependence (8). Similarly to infants born with short-gap EA, infants born with long-gap EA undergo serial follow-up esophagogastroduodenoscopies in the first year of life to evaluate and treat potential esophageal anastomotic strictures (9).
With increased survival of infants born with EA (10), there is a knowledge gap regarding neurodevelopmental outcomes. A recent systematic review of long-term neurodevelopment in children born with EA summarized conflicting results regarding outcomes (11), and the study did not specify the risk related to the surgical types of EA: short- vs. long-gap defect. Literature suggests that surgery for thoracic noncardiac congenital anomalies was associated with high-risk of brain injury leading to delay in neurocognitive and motor development (12), including those with EA (13, 14). Our recent pilot study highlighted potential risks of complex perioperative critical care as part of the Foker process on brain findings (15) and brain growth (16) following repair of long-gap EA.
The primary objective of the current retrospective study was to quantify the frequency of neurodevelopmental delay according to the complexity of perioperative care (short- vs. long-gap EA) in a recently described cohort of infants born with EA (10) that underwent repair at a single institution. We also investigated the frequency and timing of implementation of early intervention (EI) services. We hypothesized that early-to-late premature infants and those with long-gap esophageal atresia are at greater risk of developmental delay than those term-born and presenting with a short-gap EA.
This study extends our previous analysis of a retrospective cohort of infants born with EA that underwent surgical repair at our institution from 2009 to 2020 (10). Institutional Review Board approved the study as a no greater than minimal risk. The study conformed to the standards set by the Declaration of Helsinki and Good Clinical Practice guidelines. Retrospective data were obtained from a prospectively maintained Esophageal and Airway Treatment Center REDCap database established in 2009. Eligibility criteria included: (1) term-born (37–42 weeks of gestation at birth), and (2) premature infants (28 to <37 weeks of gestation at birth (17) born with EA of any type that underwent surgical treatment at our institution. Exclusion criteria included (1) extreme prematurity [<28 weeks of gestation at birth (17)] as this group is already at high risk of neurodevelopmental findings (18), (2) any previous surgical intervention at other institutions, and (3) co-existing genetic or chromosomal abnormalities known to be associated with neurodevelopmental delays (n = 14).
Due to the retrospective study design, all data were obtained from the electronic medical record, Powerchart (Cerner, London, UK) as part of the clinical diagnostics and treatment. As previously described (10), we collected demographic and clinical characteristics including: (1) date of birth, (2) gestational age at birth (weeks), and (3) sex. Table 1 summarizes the characteristics of the retrospective cohort (n = 70) with respect to co-existing anomalies.
We categorized our retrospective cohort into short-gap (n = 54) and long-gap (n = 16) EA surgical groups. At our institution, the latter group undergo the Foker process (5, 6) that involves: (1) Foker I thoracotomy or thoracoscopy to place traction sutures onto the esophageal ends; (2) Time-on-traction with traction system adjustments; (3) Foker II thoracotomy or thoracoscopy to perform esophageal anastomosis; and (4) Postoperative sedation and subsequent weaning of sedation to allow for healing of the esophageal anastomosis (8, 16).
Most patients in this retrospective analysis were born with co-existing cardiac anomalies (10) (Table 1). Due to the known increased risk of neurodevelopmental delays following cardiac repair (19, 20), co-existing cardiac anomalies were categorized into (1) major (requiring cardiac surgical repair), or (2) minor (not requiring surgical correction) as previously summarized [Table 2 in (10)]. Neurological findings of our cohort were obtained from clinically indicated cranial ultrasound and/or brain MRI findings [Table 2 in (21)]. Cranial imaging findings included the presence of abnormal head shape (e.g., plagiocephaly, brachycephaly, etc.), and/or signs of traumatic perinatal injury (e.g., cephalohematoma). Brain imaging findings ranged from likely benign (e.g., a simple cyst) to more serious findings [e.g., cerebral ventriculomegaly, brain atrophy, intracranial hemorrhage; see Figure 5 in (15)].
In this retrospective review, our chart analysis focused on any mention of developmental delay, as documented in clinical notes from a variety of specialties, including developmental medicine, primary care, and physical therapy consultations. We categorized the frequency of neurodevelopmental outcomes in our cohort based on clinical documentation into three categories: (1) documented developmental delay, (2) documented normal development, and (3) not known—in cases when no clinical notes pertaining to neurodevelopmental assessment were available. The term “neurodevelopmental delay” refers to any type of neurodevelopmental impairment documented in the patients' clinical charts (e.g., motor delay, speech/language delay, cognitive delay, or failure to meet age-appropriate milestones) as recorded by a medical professional. Notably, feeding difficulties and/or dysphagia, which are common consequences of EA repair, were not considered a symptom of neurodevelopmental delay. We further categorized frequency of neurodevelopmental delay into three domains: (1) motor delay, (2) speech/language delay, and (3) cognitive delay, based on terminology used in the clinical notes. According to the clinicians’ reports, motor delay was characterized by abnormalities in gross-motor (e.g., large body complex movements and mobility) and/or fine-motor skills (e.g., visual-motor coordination, visual-spatial skills, and hand motor control). Speech/language delay, as noted in clinical reports, referred to delays in speech production, expressive language, and/or receptive language. Cognitive delay findings included memory, learning, and/or problem-solving delays as identified through neurodevelopmental assessments conducted by clinicians. Lastly, we collected the frequency of global developmental delay (GDD) as mentioned in the clinical notes. GDD refers to significant delays in two or more developmental domains (motor, language, cognitive, social interaction, and daily livings skills) (22).
We report the presence of neurodevelopmental delay documented in (i) the first year only, (ii) the second year only, or (iii) both the first and the second years of life. Additionally, we collected the earliest age (in months) of initial clinical documentation of any type of neurodevelopmental delay. For premature infants, we used corrected post-natal age that adjusted for gestational age at birth (in weeks) (23).
At our institution, early intervention (EI) programs (24) that include a range of services and supports, are recommended for pediatric patients who show signs of developmental delays, as identified through neurodevelopmental assessments. Thus, we additionally retrospectively quantified the frequency of EI implementation by 2 years of age, based on clinical documentation. Additionally, we recorded the earliest age (corrected post-natal age in months) at which EI implementation was first documented.
Retrospectively collected data were presented as frequencies and percentages for documented neurodevelopmental delay and EI implementation in the context of (1) surgical classification of EA (short-gap vs. long-gap EA), (2) sex, (3) gestational age at birth (term-born vs. premature), and (4) co-existing cardiac anomalies and known cranial/brain findings. To enhance sample size and statistical power, frequency data were based on multiple assessments per patient and categorized for cross-sectional analysis at both the first and second years of life.
We identified 54/70 (77%) short-gap, and 16/70 (23%) long-gap EA patients in our retrospective cohort (n = 44 term-born; n = 26 early-to-late premature) (10). Details of EA cohort (i) in the context of other congenital anomalies, and (ii) for surgical distribution of EA per sex, gestational age at birth, and gestational age at first EA surgery have been presented previously in Table 1 and Figure 1, respectively (21).
Figure 1. Earliest timing and frequency of documented developmental delay in infants born with esophageal atresia (EA). (Panel A) summarizes the absolute number (#) of infants with documented developmental delay across the first 2 years of life per earliest timing of clinical notes documentation (as corrected post-natal age in months): short-gap (n = 31/54; white bars), and long-gap EA (n = 13/16; black bars). This retrospective cohort includes infants that underwent EA repair at a single institution (2009-2020; n = 70) (10). Line graphs illustrate the change in occurrence of documented developmental delay between the two time points, the first and the second year of life, for infants born with short-gap (n = 54; Panel B) and long-gap EA (n = 16; Panel C). Documented developmental delay data are shown as percent (%) and are classified as: present (black line), absent (gray line), or not known (dotted line).
The earliest documentation of developmental delay of any type was noted in the first 6 months of life (Figure 1A): 48% (15/31) in the short-gap group, and 85% (11/13) in the long-gap group. Indeed, neurodevelopmental delay was first documented over the course of the first year of life for most of the infants born with short-gap (24/31; 77%) and long-gap EA (12/13; 92%). For short-gap EA group (Figure 1B), nearly half had documented developmental delay in the first year (24/54; 44%) and the second year of life (28/54; 52%). For the long-gap EA group (Figure 1C), the majority had neurodevelopmental delay documented in the first (12/16; 75%) and the second year of life (11/16; 69%). The remainder of the cohort had documented normal neurodevelopment throughout the first two years of life, and only one short-gap EA patient lacked documentation regarding neurodevelopment (Figure 1B).
We report equal sex distribution of documented developmental delay by the second year of life: 50% (14/28) male for short-gap, and 55% (6/11) male for long-gap EA. Sex distribution for the remaining infants with no developmental delay and those with incomplete records is also illustrated in Figure 2.
Figure 2. Frequency of documented developmental delay by Sex in infants born with esophageal atresia (EA). Cohort includes infants that underwent EA repair at a single institution from 2009 to 2020 (n = 70) (10). Graphs summarize the occurrence of documented developmental delay distributed by sex at two time points: the 1st year (left graphs), and the 2nd year of life (right graphs). Documented developmental delay data per sex are presented as absolute numbers (#) and are classified as: present (black bars), absent (white bars), or not known (stripes pattern bars) per male and female cohort infants born with short-gap (n = 54; Panels A,B) and long-gap EA (n = 16; Panels C,D). Percentages (%) of developmental delay (black bars) are listed in each graph.
As illustrated in Figure 3, our retrospective cohort (n = 70) has about two-thirds term-born (n = 44) and about one-third premature infants (n = 26). Nearly half of term-born infants with short-gap (16/37; 43%) and long-gap EA (4/7; 57%) had documented developmental delay by the second year of life (Figures 3A,C, respectively). In contrast, the majority of premature patients had documented developmental delay regardless of the surgical type of EA: 71% (12/17) short-gap, and 78% (7/9) long-gap EA (Figures 3B,D). Interestingly, for short-gap EA infants with developmental delay in the first (n = 24/54) and the second year of life (n = 28/54), there is nearly equal distribution between term-born [58% [14/24] in the first; 57% [16/28] in the second year of life] and premature groups. In contrast, long-gap EA patients with developmental delay (n = 12/16 in the first; n = 11/16 in the second year of life) were more frequently premature [67% [8/12] in the first; 64% [7/11] in the second year of life] rather than term-born.
Figure 3. Frequency of documented developmental delay by gestational age at birth in infants born with esophageal atresia (EA). Graphs summarize the occurrence of documented developmental delay in the 1st and the 2nd year of life by gestational age in a retrospective cohort of infants that underwent EA repair at a single institution (2009–2020; n = 70) (10). The occurrence of documented developmental delay data are presented as absolute numbers (#), and are classified as: present (black bars), absent (white bars), or not known (stripes pattern bars) for term-born (37–42 weeks of gestation at birth; left column) and early-to-late premature infants (28 to <37 weeks of gestation of birth; right column) born with short-gap (n = 54; Panels A,B) and long-gap EA (n = 16; Panels C,D). Percentages (%) of developmental delay (black bars) are listed in each graph.
It was previously reported [Table 2 in (10)] that most of the short-gap (38/54; 70%) and long-gap EA patients (13/16; 81%) in our cohort had minor co-existing cardiac anomalies that did not require surgical repair. Here, we report that the majority of short-gap (n = 28/54) and long-gap EA infants (n = 11/16) with documented developmental delay by the second year of life also had minor co-existing cardiac anomalies: 64% (18/28) short-gap (Figures 4A,B), and 91% (10/11) long-gap EA (Figures 4C,D). Interestingly, minor cardiac anomalies were also clinically noted in most of the infants with documented normal development in both surgical groups (Figure 4).
Figure 4. Frequency of documented developmental delay in relation to co-existing cardiac anomalies. Graphs show the incidence of documented developmental delay at two time points: the 1st year (0–12 months old; left graphs), and the 2nd year of life (12–24 months; right graphs) in relation to co-existing cardiac anomalies. The latter are described as either minor (gray bars) that did not require cardiac repair, or major cardiac anomalies (black bars) that required thoracic cardiac surgery. White bars denote isolated EA that did not have co-existing cardiac anomalies. Occurrence of documented developmental delay data are presented as absolute numbers (#) for two surgical groups: short-gap (n = 54; Panels A,B) and long-gap EA (n = 16; Panels C,D).
We previously summarized the type and frequency of neurologic diagnostics and neurological findings of the cohort [Table 2 in (18)]. Nearly half of short-gap EA patients with developmental delay (n = 24/56 in the first; n = 28/56 in the second year of life) had cranial/brain findings: 46% (11/24; Figure 5A) in the first, and 39% (11/28; Figure 5B) in the second year of life. Similar frequency of cranial/brain findings were also identified in long-gap EA patients with documented developmental delay (n = 12/16 in the first; n = 11/16 in the second year of life): 50% (6/12; Figure 5C) in the first, and 45% (5/11; Figure 5D) in the second year of life.
Figure 5. Frequency of documented developmental delay in relation to co-existing cranial/brain findings. Graphs show the occurrence of documented developmental delay at two time points: the 1st year (0–12 months old; left graphs), and the 2nd year of life (12–24 months old; right graphs) in relation to co-existing cranial/brain findings (10). Documented developmental delay data are presented as absolute numbers (#), and are classified as: present, absent, or not known per two surgical groups [short-gap EA, n = 54 [Panels A,B]; long-gap EA, n = 16 [Panels C,D]]. Presence of known co-existing cranial/brain findings in the 1st year of life is illustrated by black bars, while absence of co-existing findings or lack of information on possible cranial/brain findings is illustrated by white bars. Please refer to the text regarding the limitation of the underlying cranial/brain imaging diagnostics.
Neurodevelopmental delay in the first two years of life was clinically documented in over half of short-gap (31/54; 57%) and most of long-gap EA patients (13/16; 81%). Indeed, as summarized in Figure 6A, documented neurodevelopmental delay “of any type” was identified in most of the EA patients in the first year of life and persisted into the second year of life irrespective of surgical type: 68% (21/31) of short-gap, and 77% (10/13) of long-gap EA. Only a few short-gap (3/31; 10%) and long-gap EA patients (2/13; 15%) had documented developmental delay in the first year that resolved by the second year of life. With respect to global developmental delay (GDD), about 35% (19/54) of short-gap and 31% (5/16) of long-gap EA patients had documented GDD only in the first 2 years of life (Figure 6B). Of those, clinical documentation of GDD was made primarily in the second year of life: short-gap (14/19; 74%) and long-gap EA patients (4/5; 80%).
Figure 6. Timing of developmental delay and global developmental delay in esophageal atresia (EA) at a single institution. Graphs show the timing of developmental delay documentation in infants that underwent EA repair at a single institution (2009–2020; n = 70) (10) presented as: any type of developmental delay (A), and global developmental delay (B). See Methods section for definitions of developmental delay types. Graphs show data only for those with documented developmental delay per surgical group: short-gap (n = 31/54 in left graphs; n = 19/54 in right graphs), and long-gap EA (n = 13/16 in A; n = 5/16 in B). Graphs show absolute numbers (#) and percentage (%) per surgical group of each time-point.
Specifically, Figure 7 illustrates that the earliest motor function delay was noted in the first year of life in both short-gap (22/54; 41%) and long-gap EA patients (11/16; 69%), and remained roughly the same in the second year of life [37% [20/54] short-gap; 63% [10/16] long-gap EA]. As language typically develops in the second year of life, we accordingly report nearly half of the patients with documented delayed speech/language development: 46% (25/54) short-gap, and 44% (7/16) long-gap EA patients. Data for cognitive development should be interpreted with caution considering the sources of evaluation included physicians (non-psychologist) lacking formal training in cognitive assessment of young children.
Figure 7. Frequency of documented developmental delay types in esophageal atresia (EA) at a single institution. Graphs summarize the occurrence of documented developmental delay types at two time points: the 1st year (0–12 months old), and the 2nd year of life (12–24 months old). Retrospectively collected measures include documented (i) motor (white bars), (ii) speech/language (gray bars), and (iii) cognitive delay (black bars) as per clinical notes for cohort infants born with short-gap (n = 54/70; A) and long-gap EA (n = 16/70; B). See Methods section for definitions of developmental delay types. Graphs show absolute numbers (#) and percentage (%) per surgical group of each time-point.
In our cohort, EI enrollment was noted at or before six months old for 63% (24/38) of short-gap and 81% (9/11) of long-gap EA patients and continued to be implemented across the first two years of life (see Figure 8A for individual distribution across time). Importantly, we report that 70% (38/54) of short-gap and 69% (11/16) of long-gap EA patients, were enrolled in EI services by two years of age (Figure 8B). Of the infants that underwent EI evaluation, developmental delay was clinically noted in 74% (28/38) of short-gap and 91% (10/11) of long-gap EA patients.
Figure 8. Early intervention implementation in infants that underwent esophageal atresia (EA) repair at a single institution. (Panel A) illustrates the individual timing of early intervention implementation by corrected age (in months) for two different surgical groups: short-gap (n = 54; white dots), and long-gap EA (n = 16; black dots). In contrast, (Panel B) shows the cohort frequency (#) of early intervention implementation by 2 years of age distributed by surgical group in absolute numbers while percentages (%) are summarized per surgical group. As described in Methods section, infants were enrolled in early intervention services either for existing developmental delay or for being at risk of developmental delay.
Our retrospective analysis shows high occurrence of neurodevelopmental delay following both short-gap and long-gap EA repair, documented as early as the first six months of life. Clinical documentation of neurodevelopmental delay was noted irrespective of (1) gestational age at birth (term-born vs. premature), (2) co-existing cardiac anomalies, or (3) presence of cranial/brain imaging findings. Lastly, we report high frequency of EI implementation before two years of age in both short-gap and long-gap EA patients.
Our retrospective data show that infants born with EA, irrespective of the surgical type, are at risk of developmental delay throughout the first and the second year of life, documented as early as the first six months of life. Similarly, a study by Francesca et al. (25) reported development delays at 6 and 12 months, with lower average developmental scores at 12 months compared to 6 months. Another study by Walker et al. (26) reported developmental delay at 12 months of age in 52% of their cohort of EA patients. Neurodevelopmental delay in patients born with EA was also reported between 12 and 36 months in yet another study by Mawlana et al. (27). Neurodevelopmental delay was also noted as early as 12 months of age in other cohorts of infants that underwent complex critical care following repair of non-cardiac congenital anomalies (13), cardiac anomalies (20), and congenital diaphragmatic hernias (28).
In addition to high occurrence of documented developmental delay in long-gap EA patients, nearly half of infants born with short-gap EA in our retrospective cohort had developmental delay documented in the first two years of life. This finding suggests risk of early developmental delay irrespective of the complexity of surgical repair. Literature suggests that surgery for thoracic noncardiac congenital anomalies was associated with high-risk of brain injury (14–16) possibly leading to delay in neurocognitive and motor development (12), including those with EA (13). Given that infants with congenital gastrointestinal anomalies experience multiple stressors early in life, and the fact of improved survival rates of infants born with EA over the recent decade (10), prospective follow up studies are needed to comprehensively examine the impact of complex perioperative critical care on long-term neurodevelopmental outcomes in infants born with EA irrespective of the surgical type (short-gap and long-gap EA).
We report findings of documented motor delay in both short- and long-gap EA groups in the first and the second year of life. This finding is consistent with the most recent reports of motor delays throughout the first two years of life in group of infants following EA repair (25, 26). Early assessment and detection of motor delays before 12 months old is important, as fine and gross motor functions such as grasping, sitting independently, and crawling typically appear during this timeframe. Assessment in the second year is similarly important as delays in gross motor functions such as standing and walking typically emerge between 12 and 15 months old (29). Nearly half of our cohort patients had also documented speech/language delays in the second year of life (Figure 7), which is when expressive and receptive language delays become apparent (29). A study by Mawlana et al. (27) similarly reported evidence of speech/language delays during this timeframe. As evaluation of cognitive functions are difficult to interpret before age two by non-psychologists lacking in specialized cognitive assessment expertise, our data regarding cognitive delays should be interpreted with caution. Previous reports by other groups also failed to detect cognitive developmental delay at this early age (9, 25, 26). Lastly, only a minority of short-gap and long-gap EA patients had documented GDD (Figure 6B). However, the frequency of GDD increased from the first to the second year of life, which in the context of GDD definition (see Methods), reflects increased detection of multiple types of developmental delays over time. Future studies in infants born with EA should involve administered neurobehavioral assessments (e.g., Bayley Scales of Infant and Toddler Development) at several time points in the first 3 years of life.
Equal sex distribution of the cohort (10) and nearly equal sex distribution with respect to the frequency of documented developmental delay per surgical type (Figure 2) implies that there is no sex-specific vulnerability to developmental delay in infants born with EA. As such, future studies could potentially combine sex data with a goal of increasing the study power.
As it is well known that prematurity is a risk factor for neurodevelopmental delay (30), it is not surprising that we report higher frequency of early documented neurodevelopmental delay in premature infants (born 28 to <37 weeks of gestation) compared to term-born infants irrespective of the surgical group of EA (Figure 3). Our report highlights that the frequency of neurodevelopmental delay in term-born patients is higher than that in the general population. As such, our study represents a “call to action” to address the gap in our knowledge regarding the underlying mechanisms that lead to neurodevelopmental vulnerability of term-born infants following EA repair.
It is well known that infants are at risk for adverse neurodevelopmental outcomes following neonatal cardiac surgery (19, 20). As EA often presents with other congenital anomalies that can impact complexity of care (10, 21), the frequency of neurodevelopmental outcomes in relation to co-existing cardiac anomalies represent novel findings. We identified that most EA patients with documented early neurodevelopmental delay had only co-existing minor cardiac anomalies that did not require surgical repair (Figure 4).
Documented neurodevelopmental delay was found both in the presence and absence of known cranial/brain findings irrespective of the surgical group (Figure 5). As we previously reported (21), the frequency of cranial/brain findings in our group (67% of those imaged; 22/33) may be underestimated, as neurologic diagnostic imaging was performed in only about half of the cohort (47%, 33/70). A study by Stolwijk et al. (14) reported that infants born with noncardiac congenital anomalies are at risk of postoperative brain injury following neonatal surgery, potentially contributing to the increased frequency of observed developmental delay in this population (11–13). Furthermore, our recent pilot MRI study following long-gap EA repair with Foker process reported clinically significant incidental imaging findings (15, 16) and reduction of brain volumes (15, 31, 32). Although EA is not known to be associated with neurological imaging findings or specific neurological sequelae (2), future prospective research is warranted to evaluate neurologic vulnerability and its impact on the risk of long-term neurodevelopmental sequelae.
In this report, we show EI implementation in most infants with EA irrespective of the surgical stratification (Figure 8). Similar frequency of EI enrollment was found in a cohort of infants undergoing surgical repair of congenital cardiac anomalies who were also at risk of developmental delay (20). Infants born with congenital cardiac anomalies are typically enrolled in EI programs initiated as early as the first month of life due to the high risk of neurodevelopmental delays (19). Thus, our novel findings highlight the need for timely detection with regular follow-up neurodevelopmental assessments regardless of surgical type, gestational age, or co-existing anomalies of infants born with EA. The more detailed aspects of the EI programs, including but not limited to individualized therapeutic services (e.g., physical therapy, occupational therapy, speech therapy, behavioral and cognitive developmental therapy), family service plans, and educational plans, are beyond the scope of this manuscript's analysis.
In the context of retrospective studies, data obtained from the medical records were originally intended for clinical use alone. Thus, the retrospective study design may lead to incomplete or missing documentation (e.g., unknown status of neurodevelopmental assessment and EI implementation). Despite our exclusion criteria, this study retained a moderate sample size with enough power to quantify early neurodevelopmental outcomes. The sample size n = 16 for infants born with long-gap EA is considered sufficient by literature to obtain both accurate and clinically relevant results (33).
This original retrospective analysis assessed the neurodevelopmental outcomes in a novel manner per surgical group: short- vs. long-gap EA. We report a high occurrence of documented neurodevelopmental delay as early as the first six months of life, regardless of gestational age at birth, surgical type, or co-existing anomalies, as well as a high frequency of EI enrollment. Our data is in support of early and regular neurodevelopmental assessments, EI enrolment, and long-term neurodevelopment follow-up programs of infants born with EA.
The raw data supporting the conclusion of this article will be made available by the authors upon reasonable request.
The studies involving humans were approved by Boston Children's Hospital IRB. The studies were conducted in accordance with the local legislation and institutional requirements. Written informed consent for participation was not required from the participants' legal guardians/next of kin in accordance with the national legislation and institutional requirements.
ML: Conceptualization, Data curation, Formal Analysis, Investigation, Methodology, Visualization, Writing – original draft, Writing – review & editing. DE: Conceptualization, Data curation, Formal Analysis, Investigation, Methodology, Supervision, Writing – review & editing. JW: Conceptualization, Methodology, Resources, Validation, Writing – review & editing. DP: Methodology, Resources, Validation, Writing – review & editing. AS: Methodology, Resources, Validation, Writing – review & editing. BZ: Conceptualization, Resources, Writing – review & editing. DB: Conceptualization, Data curation, Formal Analysis, Funding acquisition, Investigation, Methodology, Project administration, Resources, Supervision, Validation, Visualization, Writing – original draft, Writing – review & editing.
The author(s) declare financial support was received for the research, authorship, and/or publication of this article. This work was supported in part by the Boston Children's Hospital 2019 OFD/BTREC/CTREC Faculty Career Development Fellowship and NIDA R21DA058072-01 grant (DB). The content of this article is solely the responsibility of the authors and does not necessarily represent the official views of the funders.
The authors gratefully acknowledge (1) Quality Improvement Consultants, John J. Bennett, BS, MHA, and Heather B. Riley, MS, for their help with accessing The Esophageal and Airway Treatment Center REDCap data base; (2) Maggie J. McMahon, BS, MS for collecting neurologic data, and (3) Ms. Jennani Durai, Publication Editor from the Office of Medical Writing in the Department of Anesthesiology, Critical Care and Pain Management at Boston Children's Hospital for English language editing support.
The authors declare that the research was conducted in the absence of any commercial or financial relationships that could be construed as a potential conflict of interest.
The authors declare that no Generative AI was used in the creation of this manuscript.
All claims expressed in this article are solely those of the authors and do not necessarily represent those of their affiliated organizations, or those of the publisher, the editors and the reviewers. Any product that may be evaluated in this article, or claim that may be made by its manufacturer, is not guaranteed or endorsed by the publisher.
EA, esophageal atresia; EI, early intervention; GDD, global developmental delay; MRI, magnetic resonance imaging.
1. Aspirot A, Baird R, Faure C, Laberge JM. Second conference on esophageal atresia. Dis Esophagus. (2013) 26(4):353. doi: 10.1111/dote.12052
2. Cassina M, Ruol M, Pertile R, Midrio P, Piffer S, Vicenzi V, et al. Prevalence, characteristics, and survival of children with esophageal atresia: a 32-year population-based study including 1,417,724 consecutive newborns. Birth Defects Res A Clin Mol Teratol. (2016) 106(7):542–8. doi: 10.1002/bdra.23493
3. Rassiwala M, Choudhury SR, Yadav PS, Jhanwar P, Agarwal RP, Chadha R, et al. Determinants of gap length in esophageal atresia with tracheoesophageal fistula and the impact of gap length on outcome. J Indian Assoc Pediatr Surg. (2016) 21(3):126–30. doi: 10.4103/0971-9261.182587
4. Castilloux J, Noble AJ, Faure C. Risk factors for short- and long-term morbidity in children with esophageal atresia. J Pediatr. (2010) 156(5):755–60. doi: 10.1016/j.jpeds.2009.11.038
5. Foker JE, Kendall Krosch TC, Catton K, Munro F, Khan KM. Long-gap esophageal atresia treated by growth induction: the biological potential and early follow-up results. Semin Pediatr Surg. (2009) 18(1):23–9. doi: 10.1053/j.sempedsurg.2008.10.005
6. Bairdain S, Hamilton TE, Smithers CJ, Manfredi M, Ngo P, Gallagher D, et al. Foker process for the correction of long gap esophageal atresia: primary treatment versus secondary treatment after prior esophageal surgery. J Pediatr Surg. (2015) 50(6):933–7. doi: 10.1016/j.jpedsurg.2015.03.010
7. Tainaka T, Uchida H, Tanano A, Shirota C, Hinoki A, Murase N, et al. Two-stage thoracoscopic repair of long-gap esophageal atresia using internal traction is safe and feasible. J Laparoendosc Adv Surg Tech A. (2017) 27(1):71–5. doi: 10.1089/lap.2016.0207
8. Solodiuk JC, Jennings RW, Bajic D. Evaluation of postnatal sedation in full-term infants. Brain Sci. (2019) 9(5):114. doi: 10.3390/brainsci9050114
9. van Hoorn CE, de Graaff JC, Vlot J, Wijnen RM, Stolker RJ, Schnater JM. Primary repair of esophageal atresia is followed by multiple diagnostic and surgical procedures. J Pediatr Surg. (2021) 56(12):2192–9. doi: 10.1016/j.jpedsurg.2021.06.004
10. Evanovich DM, Wang JT, Zendejas B, Jennings RW, Bajic D. From the ground up: esophageal atresia types, disease severity stratification and survival rates at a single institution. Front Surg. (2022) 9:799052. doi: 10.3389/fsurg.2022.799052
11. van Hoorn CE, Ten Kate CA, Rietman AB, Toussaint-Duyster LCC, Stolker RJ, Wijnen RMH, et al. Long-Term neurodevelopment in children born with esophageal atresia: a systematic review. Dis Esophagus. (2021) 34(11):doab054. doi: 10.1093/dote/doab054
12. Aalten M, Tataranno ML, Dudink J, Lemmers PMA, Lindeboom MYA, Benders M. Brain injury and long-term outcome after neonatal surgery for non-cardiac congenital anomalies. Pediatr Res. (2023) 94(4):1265–72. doi: 10.1038/s41390-023-02629-8
13. Stolwijk LJ, Lemmers PM, Harmsen M, Groenendaal F, de Vries LS, van der Zee DC, et al. Neurodevelopmental outcomes after neonatal surgery for major noncardiac anomalies. Pediatrics. (2016) 137(2):e20151728. doi: 10.1542/peds.2015-1728
14. Stolwijk LJ, Keunen K, de Vries LS, Groenendaal F, van der Zee DC, van Herwaarden MYA, et al. Neonatal surgery for noncardiac congenital anomalies: neonates at risk of brain injury. J Pediatr. (2017) 182:335–41.e1. doi: 10.1016/j.jpeds.2016.11.080
15. Mongerson CRL, Wilcox SL, Goins SM, Pier DB, Zurakowski D, Jennings RW, et al. Infant brain structural mri analysis in the context of thoracic non-cardiac surgery and critical care. Front Pediatr. (2019) 7:315. doi: 10.3389/fped.2019.00315
16. Rudisill SS, Wang JT, Jaimes C, Mongerson CRL, Hansen AR, Jennings RW, et al. Neurologic injury and brain growth in the setting of long-gap esophageal atresia perioperative critical care: a pilot study. Brain Sci. (2019) 9(12):383. doi: 10.3390/brainsci9120383
17. Quinn JA, Munoz FM, Gonik B, Frau L, Cutland C, Mallett-Moore T, et al. Preterm birth: case definition & guidelines for data collection, analysis, and presentation of immunisation safety data. Vaccine. (2016) 34(49):6047–56. doi: 10.1016/j.vaccine.2016.03.045
18. Allin M, Rooney M, Griffiths T, Cuddy M, Wyatt J, Rifkin L, et al. Neurological abnormalities in young adults born preterm. J Neurol Neurosurg Psychiatry. (2006) 77(4):495–9. doi: 10.1136/jnnp.2005.075465
19. Rollins CK, Newburger JW. Cardiology patient page. Neurodevelopmental outcomes in congenital heart disease. Circulation. (2014) 130(14):e124–6. doi: 10.1161/CIRCULATIONAHA.114.008556
20. Sadhwani A, Cheng H, Stopp C, Rollins CK, Jolley MA, Dunbar-Masterson C, et al. Early neurodevelopmental outcomes in children supported with ecmo for cardiac indications. Pediatr Cardiol. (2019) 40(5):1072–83. doi: 10.1007/s00246-019-02115-1
21. McMahon MJ, Evanovich DM, Pier DB, Kagan MS, Wang JT, Zendejas B, et al. Retrospective analysis of neurological findings in esophageal atresia: allostatic load of disease complexity, cumulative sedation, and anesthesia exposure. Birth Defects Res. (2024) 116(1):e2269. doi: 10.1002/bdr2.2269
22. Belanger SA, Caron J. Evaluation of the child with global developmental delay and intellectual disability. Paediatr Child Health. (2018) 23(6):403–19. doi: 10.1093/pch/pxy093
23. Engle WA, American Academy of Pediatrics Committee on F, Newborn. Age terminology during the perinatal period. Pediatrics. (2004) 114(5):1362–4. doi: 10.1542/peds.2004-1915
24. Novak I, Morgan C. High-risk follow-up: early intervention and rehabilitation. Handb Clin Neurol. (2019) 162:483–510. doi: 10.1016/B978-0-444-64029-1.00023-0
25. Francesca B, Benedetta R, Andrea C, Annabella B, Simonetta G, Antonio Z, et al. Neurodevelopmental outcome in infants with esophageal atresia: risk factors in the first year of life. Dis Esophagus. (2021) 34(5):doaa102. doi: 10.1093/dote/doaa102
26. Walker K, Halliday R, Badawi N, Stewart J, Holland AJ. Early developmental outcome following surgery for oesophageal atresia. J Paediatr Child Health. (2013) 49(6):467–70. doi: 10.1111/jpc.12206
27. Mawlana W, Zamiara P, Lane H, Marcon M, Lapidus-Krol E, Chiu PP, et al. Neurodevelopmental outcomes of infants with esophageal atresia and tracheoesophageal Fistula. J Pediatr Surg. (2018) 53(9):1651–4. doi: 10.1016/j.jpedsurg.2017.12.024
28. Gischler SJ, Mazer P, Duivenvoorden HJ, van Dijk M, Bax NM, Hazebroek FW, et al. Interdisciplinary structural follow-up of surgical newborns: a prospective evaluation. J Pediatr Surg. (2009) 44(7):1382–9. doi: 10.1016/j.jpedsurg.2008.12.034
29. Zubler JM, Wiggins LD, Macias MM, Whitaker TM, Shaw JS, Squires JK, et al. Evidence-informed milestones for developmental surveillance tools. Pediatrics. (2022) 149(3):e2021052138. doi: 10.1542/peds.2021-052138
30. Hee Chung E, Chou J, Brown KA. Neurodevelopmental outcomes of preterm infants: a recent literature review. Transl Pediatr. (2020) 9(1):S3–8. doi: 10.21037/tp.2019.09.10
31. Mongerson CR L, Jennings RW, Zurakowski D, Bajic D. Quantitative mri study of infant regional brain size following surgery for long-gap esophageal atresia requiring prolonged critical care. Int J Dev Neurosci. (2019) 79:11–20. doi: 10.1016/j.ijdevneu.2019.09.005
32. Bajic D, Rudisill SS, Jennings RW. Head circumference in infants undergoing foker process for long-gap esophageal atresia repair: call for attention. J Pediatr Surg. (2021) 56(9):1564–9. doi: 10.1016/j.jpedsurg.2021.01.030
Keywords: critical care, developmental delay, Foker process, neurology, prolonged sedation, pediatrics
Citation: Lowdermilk MM, Evanovich DM, Wang JT, Pier DB, Sadhwani A, Zendejas B and Bajic D (2025) Retrospective analysis of early neurodevelopmental outcomes after esophageal atresia repair at a single institution: short-gap vs. long-gap defect. Front. Pediatr. 13:1527880. doi: 10.3389/fped.2025.1527880
Received: 14 November 2024; Accepted: 3 February 2025;
Published: 28 February 2025.
Edited by:
Simonetta Costa, Agostino Gemelli University Polyclinic (IRCCS), ItalyReviewed by:
Danielle S. Wendling-Keim, Ludwig Maximilian University of Munich, GermanyCopyright: © 2025 Lowdermilk, Evanovich, Wang, Pier, Sadhwani, Zendejas and Bajic. This is an open-access article distributed under the terms of the Creative Commons Attribution License (CC BY). The use, distribution or reproduction in other forums is permitted, provided the original author(s) and the copyright owner(s) are credited and that the original publication in this journal is cited, in accordance with accepted academic practice. No use, distribution or reproduction is permitted which does not comply with these terms.
*Correspondence: Dusica Bajic, ZHVzaWNhLmJhamljQGNoaWxkcmVucy5oYXJ2YXJkLmVkdQ==
Disclaimer: All claims expressed in this article are solely those of the authors and do not necessarily represent those of their affiliated organizations, or those of the publisher, the editors and the reviewers. Any product that may be evaluated in this article or claim that may be made by its manufacturer is not guaranteed or endorsed by the publisher.
Research integrity at Frontiers
Learn more about the work of our research integrity team to safeguard the quality of each article we publish.