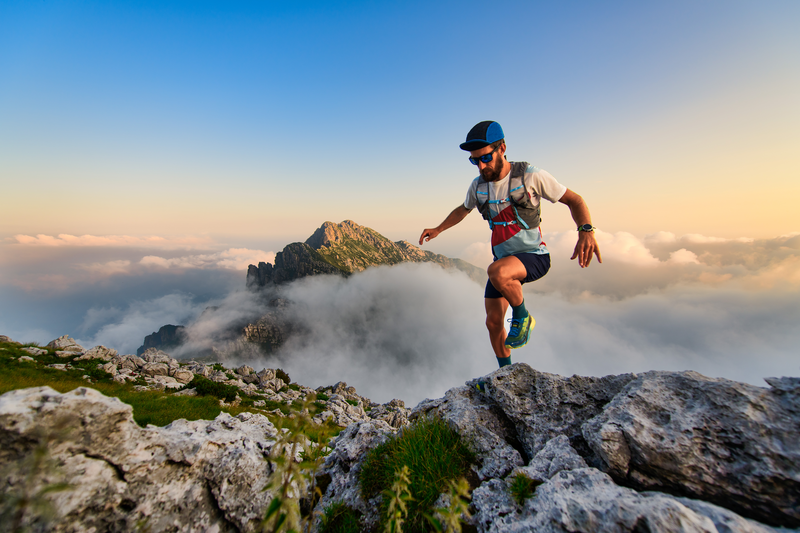
94% of researchers rate our articles as excellent or good
Learn more about the work of our research integrity team to safeguard the quality of each article we publish.
Find out more
ORIGINAL RESEARCH article
Front. Pediatr.
Sec. Pediatric Cardiology
Volume 13 - 2025 | doi: 10.3389/fped.2025.1525549
The final, formatted version of the article will be published soon.
You have multiple emails registered with Frontiers:
Please enter your email address:
If you already have an account, please login
You don't have a Frontiers account ? You can register here
Congenital heart defects (CHDs) often involve complex anatomical structures that can be challenging for patients and their families to understand. While physicians utilize various imaging techniques such as cardiac echocardiograms, CT scans, and MRIs to comprehend these complexities, the information is typically conveyed to patients and families through twodimensional (2D) images and drawings. Traditional methods often fail to fully capture the intricate nature of CHDs. This study compared the effectiveness of 2D imaging with threedimensional (3D) virtual and 3D printed models in enhancing the understanding of CHDs among patients and their families. Family members of patients with congenital heart disease, as well as patients aged 15 years or older, were recruited for the study. Participants were presented with an echocardiogram of their specific cardiac defect alongside an echocardiogram of a structurally normal heart for comparison. They were then randomly assigned to receive education using a 3D printed model or a 3D virtual model of their heart defect. Participants' knowledge of normal cardiac anatomy and the anatomy of their specific cardiac defect was assessed after viewing the echocardiogram (2D image) and again after reviewing the 3D models. One-hundred-nine subjects participated in the study, comprising 79 family members (72.5%) and 30 patients (27.5%). Subjects showed significant improvement in their understanding of normal cardiac anatomy with both 3D printed and 3D virtual models compared to the 2D image (p=0.022 and p=0.012, respectively). Among the subjects, 70% in the 3D printed group and 84% in the 3D virtual group indicated a preference for the 3D models over the 2D image. Both the 3D printed, and 3D virtual model groups rated themselves as having an increased understanding of normal cardiac anatomy compared to the 2D images (p=0.009 and p<0.001, respectively). These findings suggest that incorporating 3D models into the educational process for patients with congenital heart disease can lead to improved comprehension and greater satisfaction.
Keywords: congenital heart disease, Patient Education, 3D printing, cardiac models, Digital models
Received: 09 Nov 2024; Accepted: 17 Feb 2025.
Copyright: © 2025 Zerwic, Mohan, Riley, Byeman and Ashwath. This is an open-access article distributed under the terms of the Creative Commons Attribution License (CC BY). The use, distribution or reproduction in other forums is permitted, provided the original author(s) or licensor are credited and that the original publication in this journal is cited, in accordance with accepted academic practice. No use, distribution or reproduction is permitted which does not comply with these terms.
* Correspondence:
Ravi C Ashwath, CHRISTUS Children’s Hospital, Baylor College of Medicine, San Antonio, United States
Disclaimer: All claims expressed in this article are solely those of the authors and do not necessarily represent those of their affiliated organizations, or those of the publisher, the editors and the reviewers. Any product that may be evaluated in this article or claim that may be made by its manufacturer is not guaranteed or endorsed by the publisher.
Research integrity at Frontiers
Learn more about the work of our research integrity team to safeguard the quality of each article we publish.