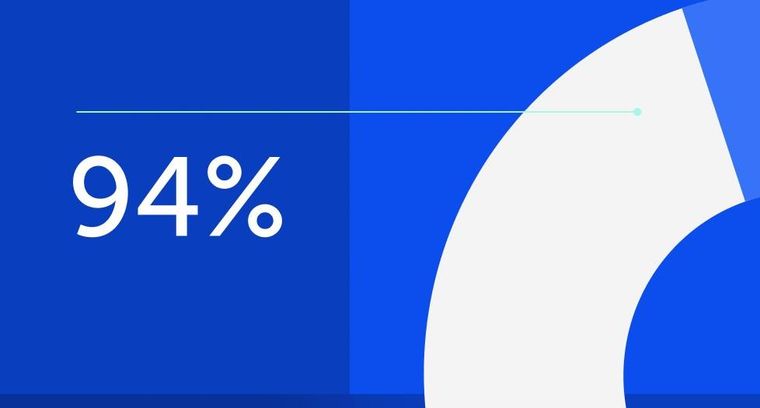
94% of researchers rate our articles as excellent or good
Learn more about the work of our research integrity team to safeguard the quality of each article we publish.
Find out more
ORIGINAL RESEARCH article
Front. Pediatr., 25 March 2025
Sec. Pediatric Critical Care
Volume 13 - 2025 | https://doi.org/10.3389/fped.2025.1494533
Objective: Capillary leak syndrome (CLS) is an urgent problem in postoperative patients, is challenging to diagnose early, and has a poor prognosis. We investigated a quick and convenient diagnostic indicator of secondary CLS in children after cardiopulmonary bypass (CPB).
Methods: We conducted this single-center, observational, prospective study in the Department of Critical Care Medicine at the Children's Hospital of Chongqing Medical University. All the data were collected within 24 h after cardiopulmonary bypass (CPB). The secondary CLS risk factors were determined using univariate and multivariate logistic regression analysis, and the cut-off point of secondary CLS was found by receiver operating characteristic (ROC) analysis.
Results: Our study included two hundred four pediatric patients in the PICU after cardiopulmonary bypass (CPB). 42.65% (87/204) of patients were diagnosed with secondary CLS. The incidence of acute kidney injury (AKI) was 36.76% (75/204), and the mortality was 5.39% (11/204). Logistic analysis indicated that a pulmonary exudation on chest radiograph, a high thoracic fluid content (TFC) and a higher vasoactive inotropic score (VIS) were independent risk factors for secondary CLS [odds ratio [OR] 23.62, 95% confidence interval [CI] 7.20–90.41, p < 0.001; OR 1.08, 95% CI 1.02–1.16, p = 0.010; OR 1.06, 95% CI 1.01–1.14, p = 0.049; respectively]. According to the ROC analysis, the cut-off point for the TFC was 52 (Ω−1).
Conclusions: The TFC plays a key role in the early prediction of secondary CLS in children after CPB, and this novel indicator may help clinicians initiate intensive treatment as early as possible.
China has the most individuals with congenital heart disease (CHD) in the world (1), and the CHD birth incidence has increased from 0.201‰–4.905‰ in the past few decades (2). The proportion of CHD-related deaths in children has risen in recent years (1), and early cardiac surgery may be an opportunity to help these children with CHD survive (3). However, early cardiac surgery may also be associated with increased mortality because of the prolonged inflammation (4). The CLS often occurs in critically ill patients and may lead to multiple organ dysfunction syndrome (5), especially in those children who underwent CPB (4). The CLS has an uncertain incidence and high mortality rate (6). The reported mortality of CLS ranges from 20%–30% (6). Although CLS is common in critically ill patients, uniform diagnostic criteria still need to be established (7), which may inhibit the early recognition of CLS. Wollborn et al. (7) established a scoring system with seven variables to identify CLS patients, but this system may be too cumbersome. A convenient indicator may contribute to the early diagnosis of CLS and provide more intensive treatment for those critical conditions.
TFC has shown promise in predicting CLS (8, 9), a life-threatening condition characterized by fluid shifts and hemodynamic instability (5). Fluid management is key in developing and treating CLS (5). The thoracic cavity can be considered an inhomogeneous electrical conductor (10). Thoracic fluid content (TFC) is derived from the thoracic electrical base impedance (Ω−1). Thoracic electrical base impedance was measured according to the amount of thoracic intravascular and extravascular fluid content. The value of TFC seems to be positively associated with the thoracic fluid volume (11). Capillary leak syndrome (CLS) is a disease characterized by increased capillary permeability, which may lead to fluid leakage into the interstitial space (5). The thoracic fluid content may increase in patients with CLS, and we assumed that TFC may play a crucial role in the early diagnosis of secondary CLS.
TFC was demonstrated to be a potential prognostic indicator in critically ill children (4, 12), but its value in the early diagnosis of secondary CLS was unknown. Our study aimed to evaluate the diagnostic value of the TFC in secondary CLS and determine the optimum cut-off point for the TFC. This study may help clinicians recognize secondary CLS early and provide more intensive therapy.
This prospective, observational, cohort study was conducted in the Department of Critical Care Medicine at the Children's Hospital of Chongqing Medical University, a National Clinical Research Center for Child Health and Disorders in China. The sample size was determined based on the method reported in the literature (13). Patients hospitalized in the pediatric intensive care unit (PICU) after cardiopulmonary bypass were enrolled in the study from December 2020–December 2022. The inclusion criteria were as follows: (i) aged younger than 18 years; (ii) admitted to the PICU after surgery; (iii) underwent cardiopulmonary bypass (CPB). The exclusion criteria were as follows: (i) refused to participate in the study; (ii) had pleural effusion or pericardial effusion before surgery. This study was approved by the Ethics Committee of Children's Hospital of Chongqing Medical University. File No. 2021 (39). Informed consent was obtained from the statutory guardians of the children. All methods were performed according to the relevant guidelines and regulations.
Age, body mass index (BMI), duration of surgery, central venous pressure (CVP), albumin level, intake and output records, chest x-ray results, the incidence of acute kidney injury (AKI), CLS, and mortality were collected from the patient's electronic records. The TFC and stroke volume variation (SVV) were monitored by using a noninvasive AESCULON® device (Osypka Medical, Berlin, Germany. San Diego, California, USA. Website: https://www.osypkamed.com/products/electrical-cardiometry/aesculon/) (14). Four sensors were placed on the left side of the patient's body (Figure 1a). The thorax impedance was measured according to the sensors' low-amplitude, high-frequency electrical current. Based on the impedance, the TFC was determined by using a complex algorithm and detected via the noninvasive hemodynamic monitor (Figure 1b). The risk stratification for congenital heart surgery (RACHS) was used to evaluate the risk of CHD surgery (15). The vasoactive inotropic score (VIS) was used to quantify the degree of hemodynamic support (16). The pediatric sequential organ failure assessment (pSOFA) score was applied to assess the severity of the patients (17). All the data were collected within 24 h after CPB. Secondary CLS was clinically diagnosed by at least two experts with at least 5 years of experience in the intensive care unit and supervised by a senior professor with at least 15 years of experience in the intensive care unit. The discussion among the experts determined the final diagnosis of secondary CLS. They did not know about our study during their clinical diagnosis and treatment. The clinical diagnostic criteria for CLS were as follows (7): (i) positive fluid balance, (ii) hypovolemic shock, (iii) edema with hypoalbuminemia. Furthermore, the AKI was diagnosed according to the Chinese clinical practice guideline for AKI (18). Moreover, hypoalbuminemia was defined as a serum albumin level of less than 3 g/dl (19). The fluid management protocols were refered to Siddall et al. (5).
Continuous variables are medians (quartiles), and categorical variables are frequencies (%). Categorial data were analyzed using the X2 or Fisher's exact test, and continuous data were analyzed using the Student's t-test or the Mann−Whitney U test. Variables with p values ≤ 0.1 were selected from the univariate logistic regression analysis to the multivariate logistic regression analysis. The receiver operating characteristic (ROC) curve was used to determine the optimum cut-off point for predicting secondary CLS. An AUC more significant than 0.9 indicated a high predictive value (20). We performed the statistical analyses in R software (version 4.3.0). A p-level ≤ 0.05 was considered to indicate statistical significance.
From December 2020 to December 2022, 257 children were admitted to the PICU after CPB. In addition, 43 children were excluded at the beginning of the study (30 children had pleural effusion or pericardial effusion before surgery, and 13 children refused to participate). Then, another 10 children were excluded (5 children with missing data and 5 children who gave up the treatment.). Then, another 10 children were excluded (5 children with missing data and five who gave up the treatment). Our study ultimately analyzed 204 children. All the details are shown in Figure 2.
The median age was 4.00 (IQR 1.00–11.25) months. The median duration of aortic cross-clamping time (ACT) and CPB were 76.00 (IQR 56.75–98.00) minutes and 112.00 (IQR 94.00–133.00) minutes, respectively. The median TFC was 42.00 (IQR 29.75–64.25) Ω−1. Moreover, the median pSOFA score was 6.00 (3.00–12.00). 42.65% (87/204) of patients were diagnosed with CLS. In the first 24 h after CPB, one hundred and forty-nine children had a positive fluid balance, and ninety-four had pulmonary exudation according to chest radiography. The incidence of AKI was 36.76% (75/204), and the mortality was 5.39% (11/204). The details are shown in Table 1.
Table 1. Characteristics of 204 pediatric patients in the pediatric intensive care unit (PICU) after cardiopulmonary bypass (CPB).
The children diagnosed with CLS had a significantly greater value of TFC than those not diagnosed with CLS [87.00 (IQR 66.50–95.00) vs. 41.00 (IQR 29.00–62.00), p < 0.001]. The patients with CLS had markedly lower albumin levels than did the patients without CLS [29.00 (IQR 22.50–31.50) vs. 37.00 (IQR 29.00–40.00), p = 0.001]. Compared with those in the non-CLS group, significantly more patients in the CLS group had a positive fluid balance or pulmonary exudation on chest radiography within 24 h after CPB (95.40% vs. 56.41%, p < 0.001; 91.95% vs. 11.97%, p < 0.001; respectively). The values of VIS and pSOFA scores in the CLS group were markedly greater than those in the non-CLS group (24.00 (IQR 20.50–39.00) vs. 15.00 (IQR 8.00–21.00), p = 0.002; 20.00 (IQR 18.50–21.00) vs. 5.00 (IQR 3.00–11.00), p < 0.001; respectively). Patients with CLS had a significantly greater incidence of AKI and mortality than those without CLS (85.06% vs. 0.85%, p < 0.001; 12.64% vs. 0.00%, p < 0.001; respectively). Age, duration of the surgery, SVV, CVP, and the risk stratification for congenital heart surgery were not significantly different between the CLS and the non-CLS groups. The results are presented in Table 2.
Table 2. Comparisons of clinical characteristics in CLS and non-CLS group in 204 pediatric patients in the PICU after cardiopulmonary bypass.
Table 3 shows the results of univariate and multivariate logistic regression analyses of risk factors for CLS. According to univariate logistic analysis, pulmonary exudation on chest radiography within 24 h after CPB and a higher value of VIS or pSOFA score, a lower albumin level, and a greater TFC were positively correlated with the incidence of secondary CLS (p < 0.001). According to our multivariate logistic analysis, pulmonary exudation on chest radiography within 24 h after CPB, and a higher value of TFC or VIS were found to be independent risk factors for secondary CLS (OR 23.62, 95% CI 7.20–90.41, p < 0.001; OR 1.08, 95% CI 1.02–1.16, p = 0.010; OR 1.06, 95% CI 1.01–1.14, p = 0.049).
Table 3. Logistic regression analysis of risk factors for CLS among 204 pediatric patients in the PICU after cardiopulmonary bypass.
The ROC curve of the TFC was plotted according to the secondary CLS in Figure 3. The area under the ROC curve (AUC) was 0.92 (95% CI 0.88–0.96), indicating good predictive efficacy. The optimal cut-off point for the TFC was 52.00 Ω−1 according to Youden's index methodology, with 80.46% sensitivity and 91.45% specificity. After CPB, patients with a TFC > 52.00 Ω−1 were at high risk for CLS.
Figure 3. Receiver operating characteristic (ROC) curves of thoracic fluid content (TFC) to predict capillary leak syndrome (CLS).
The secondary CLS can be understood as a result of fluid imbalance and inflammatory responses triggered by surgery. The pathophysiological mechanisms may be as follows (21). First, the surgical trauma and CPB trigger a systemic inflammatory response, releasing cytokines (e.g., IL-6, TNF-α) that disrupt endothelial integrity, increasing capillary permeability. Second, CPB-induced ischemia-reperfusion injury and oxidative stress damage endothelial cells, further exacerbating capillary leakage. Third, postoperative fluid management challenges, such as excessive fluid administration, can worsen interstitial edema and organ dysfunction. Last, altered hemodynamics post-CHD surgery, including low cardiac output syndrome, contributes to hypoperfusion and endothelial injury, perpetuating CLS.
Thoracic Fluid Content (TFC) measured via electrical bioimpedance is a non-invasive, cost-effective tool for monitoring fluid status in critically ill children (12). It provides real-time data on thoracic fluid dynamics, aiding in the early detection of conditions like pulmonary edema and fluid overload, which are common in PICU. Its low cost and ease of use make it adaptable to various clinical settings, reducing the need for invasive procedures. Most importantly, it is more convenient than the diagnostic scoring system established by Wollborn et al. (7).
Fathy et al. (22) demonstrated the prognostic value of the TFC for predicting weaning failure in critically ill surgical patients, and Sumbel et al. (12) reported that the TFC can predict outcomes in critically ill children. Our study found that the TFC was an independent risk factor for secondary CLS. As the value of TFC increased, patients had a 1.08-fold more significant risk of CLS. Furthermore, according to the ROC analysis, patients with a value of TFC > 52.00 Ω−1 had a risk of CLS, with high predictive ability (AUC 0.92, 95% CI 0.88–0.96, p < 0.001). The prognostic value of the TFC for secondary CLS may be explained as follows.
The electrical base impedance of the thoracic tract changes with the amount of fluid and will be detected and quantified as the TFC using a noninvasive AESCULON® device (12, 14). Yan et al. (11) previously reported a positive correlation between TFC and fluid content, and Perko et al. (9) also demonstrated the association between TFC and fluid balance during cardiac surgery. The capillary permeability to protein was increased in patients with CLS, which may lead to hypovolemia and tissue edema (5). The TFC value changes with the thoracic fluid volume (23). Therefore, the TFC value could indirectly measure the capillary permeability to proteins and might help predict CLS's occurrence. Elevated TFC levels correlate with increased extravascular lung water (12), a key feature of CLS (5), enabling early intervention. This predictive capability is particularly valuable in managing high-risk pediatric patients, potentially improving outcomes by facilitating timely therapeutic measures.
Inflammatory storms are believed to be the potential cause of CLS (5). For patients who undergo CHD surgery, the anesthesia, medication, vascular injury, and surgical stress might induce an inflammation response (4). The lung is the most significant and complex immune organ in the human body and is maximally exposed to the proinflammatory cascade (8). It has already been demonstrated that exudative pleural effusions are involved in all causes of CLS (5). Similarly, our study found that the CLS group had significantly more patients with pulmonary exudation on chest radiography than did the non-CLS group (91.95% vs. 11.97%, p < 0.001). Furthermore, we also found that patients with pulmonary exudation on chest radiography within 24 h after CPB had an approximately 24-fold risk of CLS (OR 23.62, 95% CI 7.20–90.41, p < 0.001). The high OR for pulmonary infiltration remained consistent, suggesting that it was not an artifact of model instability but reflected a strong association in our study population. The high OR may be attributed to the specific characteristics of our cohort, where pulmonary exudation was a prominent and severe manifestation of CLS (5). This finding emphasizes the key role of pulmonary involvement in the pathophysiology of CLS. However, the generalizability of these results may be limited by the specific characteristics of our study population, such as disease severity and demographic composition. Further validation in larger, more diverse cohorts is essential to confirm these observations and explore potential variations across different clinical settings. The perioperative patients were routinely administered with inotropes or vasopressors. Patients with CLS suffer from hypovolemic shock (7). VIS was used to evaluate the degree of cardiovascular support in clinical trials, and a higher VIS might be correlated with poor prognosis (16). Our study also demonstrated that a higher value of VIS was correlated with a greater risk of CLS (OR 1.06, 95% CI 1.01–1.14, p = 0.049).
Patients with CLS always exhibit a loss of protein-rich fluid from the intravascular space to the interstitial space, which may lead to hypoalbuminemia and hypovolemia (5). Both fluid overload and hypovolemia can cause organ injury. Fluid management is crucial for critical patients (24) and patients with CLS (5). However, in our study, a positive fluid balance within 24 h and an albumin level were not found to be independent risk factors for CLS. We assumed this difference may be attributed to the different treatments between the two groups of children after cardiac surgery, such as balance management and colloid supplementation (such as albumin). The group without CLS received routine monitoring and treatment after surgery, while the group with CLS received diuresis, colloid supplementation (such as albumin), peritoneal dialysis, and even blood purification treatment. Furthermore, there is no consensus on defining fluid overload (24), which may also lead to difficulty evaluating the association between fluid management and prognosis.
Our study still has some limitations. First, this was a single-center study with a relatively small sample size. Sample selection bias, insufficient statistical power, and the differences between different levels of medical institutions may limit the external validity of this study. However, this study was conducted at the National Clinical Research Center for Child Health and Disorders in China, which admitted patients from all over Southwest China, and this may help to improve the persuasiveness of our results. Furthermore, a multicenter study may be needed. Second, our study enrolled only children. Therefore, our results are inapplicable to adults. Third, there are no consensus diagnostic criteria for CLS, which may limit the extrapolation of our data to other centers. In our study, CLS was diagnosed by at least two experienced clinicians and supervised by a senior professor. The diagnostic criteria were established according to the pathophysiology of CLS (7, 12). Uniform diagnostic criteria for CLS are expected to be established in the future.
TFC may serve as a novel diagnostic indicator of secondary CLS in PICU children after cardiopulmonary bypass, and patients with a TFC > 52.00 Ω−1 are at high risk of CLS.
The original contributions presented in the study are included in the article/Supplementary Material, further inquiries can be directed to the corresponding author.
The studies involving humans were approved by Ethics Committee of Children's Hospital of Chongqing Medical University. The studies were conducted in accordance with the local legislation and institutional requirements. Written informed consent for participation was not required from the participants or the participants' legal guardians/next of kin in accordance with the national legislation and institutional requirements.
JH: Conceptualization, Data curation, Formal analysis, Funding acquisition, Investigation, Methodology, Project administration, Resources, Software, Supervision, Validation, Visualization, Writing – original draft, Writing – review & editing. JC: Conceptualization, Data curation, Formal analysis, Funding acquisition, Investigation, Methodology, Project administration, Resources, Software, Supervision, Validation, Visualization, Writing – original draft, Writing – review & editing. CL: Conceptualization, Data curation, Formal analysis, Funding acquisition, Investigation, Methodology, Project administration, Resources, Software, Supervision, Validation, Visualization, Writing – original draft, Writing – review & editing. YF: Conceptualization, Data curation, Formal analysis, Funding acquisition, Investigation, Methodology, Project administration, Resources, Software, Supervision, Validation, Visualization, Writing – original draft, Writing – review & editing. FX: Conceptualization, Data curation, Formal Analysis, Funding acquisition, Investigation, Methodology, Project administration, Resources, Software, Supervision, Validation, Visualization, Writing – original draft, Writing – review & editing. JL: Conceptualization, Data curation, Formal analysis, Funding acquisition, Investigation, Methodology, Project administration, Resources, Software, Supervision, Validation, Visualization, Writing – original draft, Writing – review & editing.
The author(s) declare that no financial support was received for the research and/or publication of this article.
We thank American Journal Experts for their valuable advice on the linguistic revision of this manuscript.
The authors declare that the research was conducted in the absence of any commercial or financial relationships that could be construed as a potential conflict of interest.
All claims expressed in this article are solely those of the authors and do not necessarily represent those of their affiliated organizations, or those of the publisher, the editors and the reviewers. Any product that may be evaluated in this article, or claim that may be made by its manufacturer, is not guaranteed or endorsed by the publisher.
ACT, aortic cross-clamping time; AKI, acute kidney injury; AUC, area under the curve; BMI, body mass index; CHD, congenital heart disease; CI, confidence interval; CLS, capillary leak syndrome; CPB, cardiopulmonary bypass; CVP, central venous pressure; IQR, inter-quartile range; OR, odds ratio; PICU, pediatric intensive care unit; pSOFA, pediatric sequential organ failure assessment; RACHS, risk stratification for congenital heart surgery; ROC, receiver operating characteristic; SVV, stroke volume variation; TFC, thoracic fluid content; VIS, vasoactive inotropic score.
1. Ma K, He Q, Dou Z, Hou X, Li X, Zhao J, et al. Current treatment outcomes of congenital heart disease and future perspectives. Lancet Child Adolesc Health. (2023) 7(7):490–501. doi: 10.1016/s2352-4642(23)00076-7
2. Zhao L, Chen L, Yang T, Wang T, Zhang S, Chen L, et al. Birth prevalence of congenital heart disease in China, 1980–2019: a systematic review and meta-analysis of 617 studies. Eur J Epidemiol. (2020) 35(7):631–42. doi: 10.1007/s10654-020-00653-0
3. Lacour-Gayet F, Gouton M, Bical O, Lucet V, Roussin R, Leca F. Surgery for severe congenital heart diseases in children from developing nations. J Thorac Cardiovasc Surg. (2022) 163(2):413–23. doi: 10.1016/j.jtcvs.2021.04.088
4. Kubicki R, Grohmann J, Siepe M, Benk C, Humburger F, Rensing-Ehl A, et al. Early prediction of capillary leak syndrome in infants after cardiopulmonary bypass. Eur J Cardiothorac Surg. (2013) 44(2):275–81. doi: 10.1093/ejcts/ezt028
5. Siddall E, Khatri M, Radhakrishnan J. Capillary leak syndrome: etiologies, pathophysiology, and management. Kidney Int. (2017) 92(1):37–46. doi: 10.1016/j.kint.2016.11.029
6. Bichon A, Bourenne J, Gainnier M, Carvelli J. Capillary leak syndrome: state of the art in 2021. Rev Méd Interne. (2021) 42(11):789–96. doi: 10.1016/j.revmed.2021.05.012
7. Wollborn J, Hassenzahl LO, Reker D, Staehle HF, Omlor AM, Baar W, et al. Diagnosing capillary leak in critically ill patients: development of an innovative scoring instrument for non-invasive detection. Ann Intensive Care. (2021) 11(1):175. doi: 10.1186/s13613-021-00965-8
8. Cordemans C, De Laet I, Van Regenmortel N, Schoonheydt K, Dits H, Huber W, et al. Fluid management in critically ill patients: the role of extravascular lung water, abdominal hypertension, capillary leak, and fluid balance. Ann Intensive Care. (2012) 2:S1. doi: 10.1186/2110-5820-2-s1-s1
9. Perko MJ, Jarnvig IL, Højgaard-Rasmussen N, Eliasen K, Arendrup H. Electric impedance for evaluation of body fluid balance in cardiac surgical patients. J Cardiothorac Vasc Anesth. (2001) 15(1):44–8. doi: 10.1053/jcan.2001.20272
10. Peacock W F, Soto KM. Current technique of fluid status assessment. Congestive Heart Failure. (2010) 16(Suppl 1):S45–51. doi: 10.1111/j.1751-7133.2010.00166.x
11. Yan L, Qiu Y, Liu J, Wu J, Yang J, He W. Increased thoracic fluid content is associated with higher risk for pneumonia in patients undergoing maintenance hemodialysis. Renal Fail. (2023) 45(1):2207666. doi: 10.1080/0886022x.2023.2207666
12. Sumbel L, Wats A, Salameh M, Appachi E, Bhalala U. Thoracic fluid content (TFC) measurement using impedance cardiography predicts outcomes in critically ill children. Front Pediatr. (2020) 8:564902. doi: 10.3389/fped.2020.564902
13. Wang X, Ji X. Sample size estimation in clinical research: from randomized controlled trials to observational studies. Chest. (2020) 158(1s):S12–s20. doi: 10.1016/j.chest.2020.03.010
14. Schwarz CE, O'Toole JM, Healy DB, Panaviene J, Livingstone V, Dempsey EM. Electrical cardiometry during transition and short-term outcome in very preterm infants: a prospective observational study. Eur J Pediatr. (2024) 183(4):1629–36. doi: 10.1007/s00431-023-05387-1
15. Jenkins KJ, Gauvreau K, Newburger JW, Spray TL, Moller JH, Iezzoni LI. Consensus-based method for risk adjustment for surgery for congenital heart disease. J Thorac Cardiovasc Surg. (2002) 123(1):110–8. doi: 10.1067/mtc.2002.119064
16. Belletti A, Lerose CC, Zangrillo A, Landoni G. Vasoactive-Inotropic score: evolution, clinical utility, and pitfalls. J Cardiothorac Vasc Anesth. (2021) 35(10):3067–77. doi: 10.1053/j.jvca.2020.09.117
17. Balamuth F, Scott HF, Weiss SL, Webb M, Chamberlain JM, Bajaj L, et al. Validation of the pediatric sequential organ failure assessment score and evaluation of third international consensus definitions for sepsis and septic shock definitions in the pediatric emergency department. JAMA Pediatr. (2022) 176(7):672–8. doi: 10.1001/jamapediatrics.2022.1301
18. National Clinical Research Center for Kidney Diseases, Chinese Nephrologist Association, Expert Group on AKI Guidelines. Chinese Clinical practice guideline for acute kidney injury. Nat Med J Chin. (2023) 103(42):3332–66. doi: 10.3760/cma.j.cn112137-20230802-00133
19. Macedo E, Karl B, Lee E, Mehta RL. A randomized trial of albumin infusion to prevent intradialytic hypotension in hospitalized hypoalbuminemic patients. Crit Care. (2021) 25(1):18. doi: 10.1186/s13054-020-03441-0
20. Faraggi D, Reiser B. Estimation of the area under the ROC curve. Stat Med. (2002) 21(20):3093–106. doi: 10.1002/sim.1228
21. Yoon TG, Jang K, Oh CS, Kim SH, Kang WS. The correlation between the change in thoracic fluid content and the change in patient body weight in fontan procedure. BioMed Res Int. (2018) 2018:3635708. doi: 10.1155/2018/3635708
22. Fathy S, Hasanin AM, Raafat M, Mostafa MMA, Fetouh AM, Elsayed M, et al. Thoracic fluid content: a novel parameter for predicting failed weaning from mechanical ventilation. J Intensive Care. (2020) 8:20. doi: 10.1186/s40560-020-00439-2
23. Van De Water JM, Mount BE, Chandra KMD, Mitchell BP, Woodruff TA, Dalton ML. TFC (Thoracic fluid content): a new parameter for assessment of changes in chest fluid volume. Am Surg. (2005) 71(1):81–6. doi: 10.1177/000313480507100116
Keywords: thoracic fluid content (TFC), capillary leak syndrome (CLS), indicator, cardiopulmonary bypass (CPB), children
Citation: Huo J, Cheng J, Liu C, Fu Y, Xu F and Li J (2025) Thoracic fluid content as a novel and rapid diagnostic indicator of secondary capillary leak syndrome in pediatric patients post-cardiopulmonary bypass. Front. Pediatr. 13:1494533. doi: 10.3389/fped.2025.1494533
Received: 11 September 2024; Accepted: 14 March 2025;
Published: 25 March 2025.
Edited by:
Silvia Spoto, Fondazione Policlinico Universitario Campus Bio-Medico, ItalyReviewed by:
Veena Raghunathan, Amrita Institute of Medical Sciences, IndiaCopyright: © 2025 Huo, Cheng, Liu, Fu, Xu and Li. This is an open-access article distributed under the terms of the Creative Commons Attribution License (CC BY). The use, distribution or reproduction in other forums is permitted, provided the original author(s) and the copyright owner(s) are credited and that the original publication in this journal is cited, in accordance with accepted academic practice. No use, distribution or reproduction is permitted which does not comply with these terms.
*Correspondence: Jing Li, bGlqaW5nd2FuZ3lpQDEyNi5jb20=
Disclaimer: All claims expressed in this article are solely those of the authors and do not necessarily represent those of their affiliated organizations, or those of the publisher, the editors and the reviewers. Any product that may be evaluated in this article or claim that may be made by its manufacturer is not guaranteed or endorsed by the publisher.
Research integrity at Frontiers
Learn more about the work of our research integrity team to safeguard the quality of each article we publish.