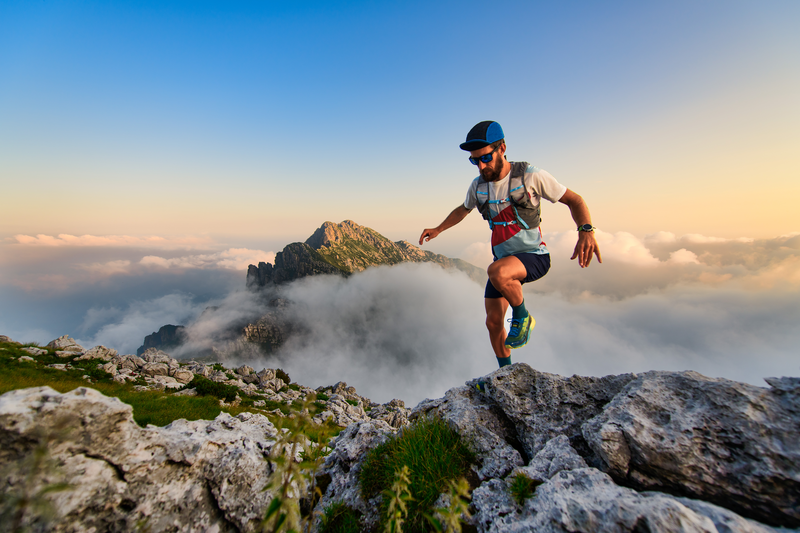
94% of researchers rate our articles as excellent or good
Learn more about the work of our research integrity team to safeguard the quality of each article we publish.
Find out more
REVIEW article
Front. Pediatr. , 16 December 2024
Sec. Pediatric Neurology
Volume 12 - 2024 | https://doi.org/10.3389/fped.2024.1448793
RHOBTB2 is a member of the Rho GTPases subfamily of signaling proteins, known tumor suppressors whose loss of function and decreased expression is associated with cancer onset. Beyond its cancer-related role, RHOBTB2 is implicated in rare neurodevelopmental disorders, specifically RHOBTB2-related disorders, recognized in 2018 as a subtype of developmental and epileptic encephalopathies (DEE). Common symptoms of these disorders include early-onset epilepsy, severe intellectual disability, microcephaly, and movement disorders. Few studies have investigated patient variants associated with RHOBTB2-related disorders, and the impact of these variants on protein function remains unclear. Limited research suggests that the accumulation of RHOBTB2 in neural tissues contributes to the development of DEE. Similarly, preclinical studies indicate that missense variants near or in the BTB domain of RHOBTB2 result in decreased degradation of RHOBTB2 and the onset of DEE, whereas variants in the GTPase domain cause more variable neurodevelopmental symptoms, but do not impair proteasomal degradation of RHOBTB2. However, the exact pathophysiological mechanisms are unclear and may differ across variants. Current treatment approaches for individuals with RHOBTB2-related DEE involve the use of antiseizure medications to decrease seizures; however, no treatments have been identified that address the other symptoms or the underlying pathophysiological mechanisms associated with these disorders. Overall, RHOBTB2 remains an understudied protein with limited information on its function and how it contributes to disease mechanisms. This review provides an overview of the current knowledge of RHOBTB2 function, with an emphasis on its association with neurodevelopmental disorders through an analysis of preclinical studies and case reports that link individual variants with clinical features.
Neurodevelopmental disorders (NDDs) are heterogeneous conditions that arise from impairments in the growth, development, and function of the central nervous system (1). These disorders manifest early during a child's development and can affect various domains including cognition, behavior, motor function, and social interactions. An increasing number of de novo variants occurring in oncogenes and tumor suppressor genes are associated with rare disorders, and in particular, rare neurodevelopmental disorders (NDDs). The reverse also holds true, as individuals with rare NDDs may be at higher risk of developing certain cancers (2). For instance, de novo variants in DDX3X are associated with severe NDD, structural brain abnormalities, and intellectual disability, while somatic DDX3X variants are associated with aggressive cancers (3). Similarly, germline variants in FBXW7, a tumor suppressor, cause impaired ubiquitination and NDD (1). This overlap between NDD and cancer occurrence could be partly due to genes that are involved in signaling pathways shared between the two pathologies, such as MAPK and mTOR, which are crucial for cell proliferation and differentiation (4).
RHOBTB2, first identified as a tumor suppressor gene, has recently been implicated in a neurodevelopmental disorder. While there are some hypotheses surrounding how the RHOBTB2 protein is involved in cancer and NDD related to variant-specific consequences, the mechanism by which it contributes to these pathologies remains unknown. This review will cover an overview of the current understanding of the structure and function of RHOBTB2, followed by an analysis of recent studies highlighting its hypothesized role in cancer and NDD, clinical characteristics, treatment approaches, and current gaps in knowledge. Lastly, future research directions will be discussed, emphasizing the importance of further in vivo studies and the potential for exploring new treatment avenues targeting RHOBTB2-related pathologies. Focusing on understudied genes such as RHOBTB2 exemplifies how deeper insights into monogenic disorders could provide answers for individuals affected by rare neurodevelopmental disorders and for those exploring novel precision oncology treatments.
Rho-related BTB domain-containing protein 2 (RHOBTB2), encoded by the gene RHOBTB2, is a known tumor suppressor and member of the Rho GTPases subfamily of signaling proteins, consisting of RHOBTB1, RHOBTB2, and RHOBTB3 (5, 6). Rho GTPases act as molecular switches and can revert back and forth between an active, GTP-bound, and an inactive, GDP-bound state (7). Rho proteins become activated through interactions with guanine exchange factors (GEFs) that catalyze the release of bound GDP, subsequently leading to the binding of GTP (7). Termination of Rho signaling is achieved through the hydrolysis of GTP by GTPase-activating proteins (GAPs) (Figure 1) (7). When activated, Rho proteins interact with downstream effectors regulating a variety of cellular processes, including apoptosis, cellular growth, cytoskeletal organization, vesicular transport, and transcription (8, 9). There is evidence suggesting that RHOBTB proteins are tissue-specific, with RHOBTB2 primarily being expressed in neural tissues. However, it is also expressed to a lesser extent in fetal tissues, such as the lungs, heart, and brain (10, 11). Pathological variants in Rho family GTPases, such as RAC1, RAC3, and CDC42, as well as GEFs and GAPs, which affect key developmental processes, are also implicated in a variety of NDDs (12–16).
Figure 1. Activation and deactivation of Rho proteins. Guanine exchange factors (GEFs) catalyze the release of bound GDP, resulting in an active, GTP-bound Rho protein (7). GTPase-activating proteins (GAPs) hydrolyze GTP, releasing an inorganic phosphate (Pi), to convert Rho proteins into the inactive form (7). Created with BioRender.com.
RHOBTB2 is considered an atypical Rho protein due to its modular, large size, and overall composition (8, 17, 18). While most Rho proteins exhibit only one Broad-complex, Tramtrack, and Bric-à-brac (BTB) domain, RHOBTB2 has two BTB domains (11). The structure of RHOBTB2 consists of a Rho-like GTPase region at the N-terminus and two BTB domains (Figure 2) (10).
Figure 2. RHOBTB2 gene and protein structure with locations of patient variants associated with neurodevelopmental disorder (NDD) depicted. RHOBTB2 consists of 12 exons (NM_001160036.2, ENST00000519685.5). The non-coding exons are colored gray, and the coding exons are white (boxes). Multiple patient variants are associated with RHOBTB2-related NDD and located in intronic and exonic regions between exon 4 and 12. Intronic or splice variants are labeled in blue and triangles indicate approximate variant locations in relation to exons. Solid triangles indicate multiple individuals reported in the literature with that variant. Missense variants (black) and truncating variants (red) are labeled with triangles in proximity to protein domains (NP_001153508.1). Stacked triangles indicate multiple variants at a specific amino acid residue and slashes delineate all reported amino acid substitutions. The RHOBTB2 protein consists of a Rho-like GTPase region, a BTB1 domain, and a BTB2 domain, based on Pfam (IPR000210) and PANTHER (IPR003578). Variant information was obtained from published reports in the literature (19–27).
There is some speculation that a proline-rich region after the Rho-like GTPase domain might be a Src homology 3 (SH3) domain-binding site, as SH3 domains are found in proteins involved in signal transduction; however, this has yet to be verified experimentally (28, 29). This could indicate the potential of RHOBTB2 to regulate proteins with SH3 domains if confirmed. Another site of protein-protein interactions is the BTB domains (18). Most notably, BTB domains are involved in post-translational modifications, such as ubiquitination and degradation (29). The two BTB domains present in RHOBTB2 enable the formation of both homodimers and heterodimers (29). Generally, most proteins that dimerize are involved in cellular processes such as enzyme activation, transcriptional cofactor recruitment, and signal transduction; therefore, any disruption in dimer formation could have detrimental effects (19, 30). Lastly, the GTPase and C-terminal regions, along with the second BTB domain, serve as substrate recognition sites (28).
RHOBTB2 is responsible for the recruitment of proteins associated with tumor growth for ubiquitination and subsequent degradation by the 26S proteasome (28). It does so through its association with the cullin 3-dependent ubiquitin ligase complex (Cul3) via its first BTB domain. First, RHOBTB2 binds to specific substrates, facilitating their recognition and interaction with the Cul3-Rbx1 complex (31). Then, the ring-box 1 protein (Rbx1) interacts with ubiquitin-conjugating enzymes (E2) to promote the transfer of ubiquitin from the E2 enzyme to the substrate protein bound to Cul3 and RHOBTB2. This process repeats, resulting in a polyubiquitin chain (18). The resulting polyubiquitin chain marks the substrate, as well as RHOBTB2 itself, for degradation in the 26S proteasome. Dysregulation of the Cul3-RHOBTB2 interaction results in RHOBTB2 instability and inactivation, suggesting that Cul3 serves as a regulatory mechanism for controlling the levels of RHOBTB2 in the cell (29, 32).
The RHOBTB family of proteins are tumor suppressors, with diminished or absent expression observed across various cancer types. Specifically, decreased levels of RHOBTB2 have been associated with breast, lung, bladder, and stomach cancers as well as osteosarcomas (5, 33–37). Reduced expression of RHOBTB2 can be caused by epigenetic modification or alternative silencing mechanisms such as the hypermethylation of CpG islands in the promoter region (35). Moreover, deletions and loss-of-function variants in RHOBTB2 were detected in nearly 10% of breast cancer samples, suggesting that these variants may lead to the loss of tumor-suppressor activity and the initiation of cancerous tumor formation (Figure 3) (33).
Figure 3. Lack of RHOBTB2-assisted degradation of tumor-growth substrates could lead to increased tumor growth in the absence of RHOBTB2. The exact mechanism as to how deletions and decreased expression of RHOBTB2 lead to carcinogenesis is unclear, however, one hypothesis is that RHOBTB2 acts as a tumor suppressor by recruiting target proteins, such as CCND1, associated with tumor growth for ubiquitylation and degradation (28, 38, 39). Under normal expression levels, wild-type RHOBTB2 protein acts as a tumor suppressor by recruiting ubiquitination proteins and degradative substrates to a Cul3 ubiquitin ligase complex for degradation (gray box) (28). Deletion variants that lead to a loss of function and/or decreased expression of RHOBTB2 lead to a lack of sequestration and degradation of cancer-related substrates and therefore unchecked cell cycle growth and cancer (33). Created with BioRender.com.
The exact mechanism by which RHOBTB2 functions as a tumor suppressor is unclear. One study examining the impact of missense variants on protein function reported that one missense variant associated with cancer (p.Tyr306Asn) resulted in decreased binding to Cul3 and subsequent impaired proteasomal degradation of RHOBTB2 (20). Impaired degradation of RHOBTB2 was not reported in other cancer cell line studies and could indicate alternative cell or tissue-specific mechanisms. Another hypothesized mechanism involves Cyclin D1 (CCND1). CCND1 is another RHOBTB2 interactor that binds within the first BTB domain (38). CCND1 functions in regulating cell cycle progression and transcription of genes involved in cell proliferation (39). It is considered an oncogenic protein as its hyperactivation results in uncontrolled cell proliferation (40). While RHOBTB2 has been observed to arrest growth in breast cancer cells, potentially by downregulating CCND1, some tumor cells proliferate even in the presence of RHOBTB2 (33, 38, 41). One possible explanation is the activation of alternative pathways, such as CCND1-independent mechanisms, that bypass the tumor-suppressing function of RHOBTB2 (33).
Neurodevelopmental disorders (NDD) encompass a range of conditions often characterized by seizures, intellectual disability, abnormal brain development, movement disorders, and other neurological abnormalities (42). In 2013, NDDs were introduced as an all-encompassing disorder category in The Diagnostic and Statistical Manual of Mental Illnesses (DSM-5) (43). While epilepsy itself is not classified as an NDD, it is a common comorbidity of NDD that is often severely debilitating and can impact intellectual development and lead to developmental regression (44, 45). RHOBTB2-related disorders were first characterized as a severe subtype type of NDD, known as developmental and epileptic encephalopathy (DEE) (19). DEE is characterized by frequent seizures that result in debilitating intellectual and behavioral impairments (46). Before 2018, the role of RHOBTB2 in neurodevelopment had not been studied in detail, however since then, small case studies with one (21), two (22–25), or three patients (26), as well as larger case studies with ten or more patients (19, 20, 27) have been published on NDD related to variants in RHOBTB2. Twenty-eight different variants have been identified to cause RHOBTB2-related NDD (Figure 2) (19–27). Recurrent variants associated with RHOBTB2-related DEE are predominantly de novo heterozygous missense variants found within the BTB domain region, such as p.(Arg483His), p.(Arg507Cys), p.(Arg511Gly), p. (Arg511Trp), and p.(Arg511Gln) (19, 20). Notably, three heterozygous variants linked to DEE, p.(Ala471Val), p.(Ala474Gly), and p.(Arg483His), are located in the BTB1 domain, and one homozygous variant, p.(Ser543Alafs*52), is located within the BTB2 domain; the remaining five heterozygous variants are clustered between the two BTB domains (20).
Genotype-phenotype correlations in RHOBTB2-related NDDs are exemplified by shared clinical features linked to specific variant clusters, such as early seizure onset in individuals with missense RHOBTB2 variants clustering in the BTB domain region. Notably, two children with the p.(Arg483His) variant developed seizures as early as 4 days after birth (19, 23). Early seizure onset is associated with severe intellectual disability, developmental delay, and motor function issues. Various types of seizures have been reported in different individuals, including febrile seizures, bilateral tonic-clonic, generalized tonic-clonic, and status epilepticus. Another condition commonly reported is microcephaly, which has been linked to seizures, aphasia, and developmental delay (47). Developmental regression has occurred in some cases, including a child with a p.(Arg511Gln) variant, who did not develop symptoms of encephalopathy until the age of 14 (23). Cognitive impairment appears milder in those with a later onset of epilepsy, but significant intellectual regression or stagnation is still present (19, 21, 23, 27). Motor function in these individuals is severely limited and delayed compared to healthy individuals, as assessed by the Centers for Disease Control and Prevention (CDC) developmental milestones (48). Many individuals saw a decrease in motor abilities following the onset of status epilepticus. Motor impairments range from total lack of head control and inability to walk to walking with a broad-based or unsteady gait. Some children develop walking abilities after some time, ranging from as early as 1 year or as late as 7 years old (19, 27). An additional study expanded the phenotypic spectrum for RHOBTB2-related disorders to include paroxysmal symptoms similar to those in alternating hemiplegia of childhood (AHC) (27). The study found that 84% of cases were reported to have paroxysmal movement disorders, which were characterized by sporadic involuntary movements, with hemiplegia being one of the most common symptoms (27, 49, 50).
Expanding the genotype-phenotype correlations, individuals with de novo heterozygous missense variants in the GTPase domain exhibit a broader spectrum of neurodevelopmental outcomes distinct from those associated with BTB domain variants, ranging from mild to moderate intellectual disability, learning difficulties, to developmental regression (20). Although seizures have been reported in a few of these individuals, antiseizure medications were effective in obtaining seizure control (20). More thorough clinical summaries of these and other patients can be found in the Supplementary Table 1 of Langhammer et al. (2023). The genotype-phenotype correlations in RHOBTB2-related NDDs highlight the complexity of these conditions, as variants within different protein domains contribute to a spectrum of neurological manifestations. This emphasizes the need for further research elucidating the underlying mechanisms behind these variants to better inform therapeutic interventions.
The molecular mechanisms associated with RHOBTB2 variants have been generally underexplored, leaving a significant gap in our understanding of their impact on patients. A recent study examined in silico structural consequences, as well as Cul3 binding interference and proteasomal degradation of RHOBTB2 using HEK293 cells, to investigate the impact of variants located in the BTB vs. the GTPase domain (20). Their analyses confirmed previous findings that BTB domain variants do not impair Cul3 binding and result in impaired proteasomal degradation of RHOBTB2 (19, 20). For variants located within the GTPase domain, specifically p.(Asp114His), p.(Arg116Cys), p.(Arg154Gln), and p.(Arg154Leu), structural consequences predicted by in silico methods suggest that these variants disrupt the electrostatic complementarity of potential protein-protein interactions (20). Additionally, in vitro methods revealed these GTPase variants, like the BTB variants, do not impair Cul3 binding (20). However, unlike the BTB variants, GTPase variants did not result in increased RHOBTB2 levels, suggesting an alternative pathogenic effect on RHOBTB2 function (20). In a separate study, mice neuroblastoma cells (Neuro-2a cells) were used to investigate the impaired degradation of mutant RHOBTB2 (26). The variants, p.(Arg483His), p.(Arg507Cys), and p.(Arg511Gln), were transfected into the Neuro-2a cells, and the cells were treated with the proteasome inhibitor MG132. The three RHOBTB2 mutants were found to be more abundant than wild-type RHOBTB2, although the difference was only significant for the p.(Arg507Cys) variant (26). Additionally, Cul3 was coexpressed in Neuro-2a cells to test whether mutant RHOBTB2 protein degradation would increase. The level of the wild-type RHOBTB2 protein decreased with the coexpression of Cul3, but there was little or no change in the level of the variant RHOBTB2 protein (26). These results reinforce the previous findings that RHOBTB2 with variants in the BTB domain can bind to Cul3 but is inadequately degraded by proteasomes. These studies support the hypothesis that increased levels of RHOBTB2 are likely caused by an unstable protein structure resulting from missense variants in the BTB region that affect ubiquitination and degradation, rather than affecting the binding of Cul3 (Figure 4) (19, 26).
Figure 4. RHOBTB2 containing missense variants in the BTB region leads to improper ubiquitination by Cul3 and increased levels of RHOBTB2 in neural tissues, causing impaired neurodevelopment. (A) RHOBTB2 binds to Cul3, a ubiquitin ligase scaffolding protein (31). Cul3 forms a complex with E2 and Rbx1. E2 carries the ubiquitin while Rbx1 transfers the ubiquitin to RHOBTB2 (18). The polyubiquitin chain marks RHOBTB2 for degradation by the 26S proteasome. (B) Evidence supports the hypothesis that missense variants in the BTB domains of RHOBTB2 destabilize the protein, thus impairing proper ubiquitination by CUL3 and degradation of RHOBTB2 (19). However, the exact mechanism of action remains unclear. Created with BioRender.com.
To date, no patient-variant animal models have been generated to investigate the neurodevelopmental effects in RHOBTB2-related disorders. However, other animal studies support the role of RHOBTB2 in neuronal processes (Table 1) (51). Drosophila sp. RhoBTB knockout model flies lacking RhoBTB exhibited seizures and motor degression (19). Conversely, flies overexpressing RhoBTB in neurons exhibited more spasms, hyperactivity, and paralysis compared to control flies, supporting the involvement of RHOBTB2 in neuronal function (19). In a study involving prenatal malnourished rats, RHOBTB2 was identified as a gene that modulates neurodevelopment (51). The primary objective of the study was to investigate the impact of maternal prenatal malnutrition on neurodevelopment and identify genes involved in regulating neurodevelopment. The researchers analyzed the growth of rat hippocampus and prefrontal cortex tissue to determine deficiencies. Using a weighted gene co-expression network analysis, this research confirmed the role of RHOBTB2 in synaptic development, neuronal projection, and cognitive functions (51). The positive correlation between RHOBTB2 expression and the development of hippocampal and prefrontal tissue supported its role in these processes. Additionally, the study found a potential regulatory link between RHOBTB2 and the transcription factor gene CEBPA (CCAAT/enhancer-binding protein-alpha), which is involved in cell proliferation and termination (51). The CEBPA protein may promote the transcription of RHOBTB2, however, further studies need to be done to validate and fully understand the potential of upregulating RHOBTB2 through CEBPA modulation (51, 53).
In vitro data suggest that certain RHOBTB2 variants contribute to impaired RHOBTB2 degradation, leading to its accumulation, while animal model data supports the role of RHOBTB2 in proper neuronal development and function (19, 51). However, it remains unknown how increased levels of RHOBTB2 in neurons lead to developmental epileptic encephalopathy (DEE). Epilepsy is the result of abnormal neuronal activity in the brain, and the apoptosis pathway is one of many mechanisms that can contribute to the development of epilepsy and seizure susceptibility (54). RHOBTB2 is implicated in apoptosis as it serves as a molecular target for eukaryotic transcription factor 1 (E2F1) (55). E2F1 has a unique role among the E2F transcription factors in inducing pro-apoptotic genes and repressing pro-apoptotic genes. Moreover, there is a direct correlation between levels of E2F1 and RHOBTB2; overexpression of E2F1 leads to increased RHOBTB2, whereas reducing E2F1 leads to decreased RHOBTB2 levels (55). Given this relationship between E2F1 and RHOBTB2, it is not surprising that cells overexpressing RHOBTB2 exhibit higher levels of pro-apoptotic proteins, such as Bax and Bak (56). Notably, alterations in the expression of pro-apoptotic genes have been reported in hippocampal and neocortical tissue taken from patients with pharmacoresistant epilepsy, although apoptotic cells are rarely found, suggesting concurrent anti-apoptotic signaling changes (54). Therefore, overexpression of RHOBTB2 could result in altered cellular apoptosis, presenting a potential pathological mechanism for RHOBTB2-related disorders (26). Additional studies are needed to test this hypothesis and gain insights into underlying DEE-related mechanisms.
Modulation of RHOBTB2 levels could potentially be a promising treatment for certain cancers and RHOBTB2-related disorders. For example, ectopic expression of RHOBTB2 was shown to inhibit migration and metastasis in breast cancer cell lines (57). However, no RHOBTB2-targeted therapies have been developed to treat cancer.
The current treatment approaches for RHOBTB2-related DEE primarily involve the administration of antiseizure medications, with the most frequently prescribed drugs including valproic acid, levetiracetam, topiramate, and oxcarbazepine to manage seizures (19, 21–23, 26, 27). There remains a lack of effective medications targeting symptoms related to motor function and movement disorders. In vitro functional studies suggest that BTB domain variants linked to RHOBTB2-related disorders lead to RHOBTB2 accumulation, suggesting a potential therapeutic avenue could involve the downregulation of RHOBTB2 expression (19, 20).
The use of artificial intelligence (AI) to investigate molecular-level therapies shows promise in identifying drug candidates capable of binding to and modulating proteins involved in RHOBTB2 signaling. AI systems offer a computational approach to identifying novel gene-related therapies, especially those suitable for drug repurposing (58). For instance, the AI program mediKanren was used to identify drug modulators of RHOBTB2, which could serve as treatments for patients with gain-of-function variants (59). By focusing on E2F1's ability to downregulate RHOBTB2, the researchers identified drugs that downregulate E2F1, thereby indirectly inhibiting RHOBTB2 expression. One of these drugs was Celecoxib (Celebrex), an FDA-approved nonsteroidal anti-inflammatory drug (NSAID) capable of crossing the blood-brain barrier (60). Celecoxib, commonly used to treat symptoms of arthritis and pain, was found to downregulate E2F1 and cyclin D1 (60, 61). This downregulation of E2F1 could potentially reduce the overexpression of RHOBTB2, while the downregulation of cyclin D1 could enhance the tumor suppression function of RHOBTB2 (55, 61). Other NSAIDs, such as diclofenac and indomethacin, have also been linked to the downregulation of E2F1 target genes, and therefore may also alleviate the effects of gain-of-function variants in RHOBTB2 (55, 61, 62). Furthermore, the use of NSAIDs as a therapeutic approach avoids compromising the tumor suppressor function of RHOBTB2, as evidenced by studies in mice demonstrating the antiproliferative effects of NSAIDs in ovarian cancer (55, 61, 62). However, these studies were tissue-specific and additional preclinical studies are needed to assess their applicability to different cancer types.
Gene therapies that specifically target the variant allele in RHOBTB2-related disorders offer future promise and unique challenges. A recent study that is still undergoing development discussed the development of a new antisense oligonucleotide (ASO) therapy that targets RHOBTB2, with the goal of reversing the accumulation of RHOBTB2 to benefit patients with DEE (63). ASOs are short, synthetic strands of nucleic acids designed to bind to specific mRNA sequences, potentially modulating gene expression. However, the rarity of these disorders and the complexity of RHOBTB2's involvement in neural development complicate the development of targeted therapies.
Precise RHOBTB2 expression levels are key to preventing tumor growth and promoting proper nervous system development and function, however, the role of RHOBTB2 in these processes requires further investigation. Studies examining RHOBTB2 as a tumor suppressor show that decreased levels of RHOBTB2, through variants, epigenetic modifications, or silencing mechanisms, are associated with multiple cancers (28, 29, 31, 33, 36). However, the extent of tumor growth induced by RHOBTB2 downregulation and whether RHOBTB2 acts in a tissue-specific manner remains unknown (33). Furthermore, although a few proteins associated with tumor growth have been identified as substrates of the RHOBTB2-Cul3/Rbx1 complex, the exact mechanisms by which RHOBTB2 functions as a tumor suppressor remain unclear.
Alternatively, variants in RHOBTB2 that lead to elevated RHOBTB2 levels are associated with developmental and epileptic encephalopathies (DEE) (19, 20). However, the exact mechanism by which these variants lead to DEE is understudied. One hypothesis from in vitro studies suggests that certain RHOBTB2 variants may destabilize the RHOBTB2 protein, impairing its degradation and interactions with other proteins (19, 26). Overall, there is a lack of preclinical and animal model studies investigating variant impact and therapeutic options for RHOBTB2-related disorders (Table 1). Currently, only a few missense variants of RHOBTB2 have been studied in animal models, and further research is needed to understand the impact of other variants on RHOBTB2 ubiquitination and degradation, particularly those located outside the BTB region and in the Rho-like region of the RHOBTB2 protein (Figure 2).
In both cancer and NDD, RHOBTB2 appears to play a role in the targeted degradation of key proteins related to tumor growth and/or neural development. However, the identification of these proteins and whether other mechanisms are involved is still under investigation. Therefore, further research is warranted to delve into the interactions of RHOBTB2 and degradative pathways.
Research into the pathophysiological disease mechanisms associated with expression levels of RHOBTB2 requires further investigation. While RHOBTB2 is linked to cancer onset in cases of decreased expression and neurodevelopmental disorders in potential gain-of-function and loss-of-function scenarios, it remains relatively understudied, with limited information available on its function and disease mechanisms. There is a consistent association between decreased levels of RHOBTB2 leading to tumor growth in specific tissues, but the mechanisms of tumor suppression are unclear. Increased levels of RHOBTB2 have been linked to DEE, but the role of RHOBTB2 in nervous system development is understudied. Future studies exploring the impact of individual patient variants on RHOBTB2 stability and function will contribute valuable insights to our understanding of its role in health and disease. Lastly, there is a need for further in vivo studies to identify new treatment avenues for both cancer and DEE, as well as paroxysmal movement disorders associated with RHOBTB2-related disorders.
ES: Conceptualization, Formal Analysis, Visualization, Writing – original draft, Writing – review & editing. AF: Formal Analysis, Visualization, Writing – original draft, Writing – review & editing. CC: Conceptualization, Formal Analysis, Funding acquisition, Supervision, Writing – original draft, Writing – review & editing.
The author(s) declare financial support was received for the research, authorship, and/or publication of this article. CMC received support for this work through the University of Alabama at Birmingham (UAB), Undergraduate Neurosciences Program (UNP) and the UAB Office of Service Learning and Undergraduate Research.
We sincerely thank Dr. Cristin Gavin, Co-Director of the UAB Undergraduate Neuroscience Program, and Mr. Gareth Jones, Director of UAB Service Learning and Undergraduate Research, for their support of this work and undergraduate research.
The authors declare that the research was conducted in the absence of any commercial or financial relationships that could be construed as a potential conflict of interest.
All claims expressed in this article are solely those of the authors and do not necessarily represent those of their affiliated organizations, or those of the publisher, the editors and the reviewers. Any product that may be evaluated in this article, or claim that may be made by its manufacturer, is not guaranteed or endorsed by the publisher.
1. Stephenson SEM, Costain G, Blok LER, Silk MA, Nguyen TB, Dong X, et al. Germline variants in tumor suppressor FBXW7 lead to impaired ubiquitination and a neurodevelopmental syndrome. Am J Hum Genet. 022) 109(4):601–17. doi: 10.1016/j.ajhg.2022.03.002
2. Yavuz BR, Arici MK, Demirel HC, Tsai C-J, Jang H, Nussinov R, et al. Neurodevelopmental disorders and cancer networks share pathways, but differ in mechanisms, signaling strength, and outcome. NPJ Genom Med. (2023) 8(1):37. doi: 10.1038/s41525-023-00377-6
3. Scala M, Torella A, Severino M, Morana G, Castello R, Accogli A, et al. Three de novo DDX3X variants associated with distinctive brain developmental abnormalities and brain tumor in intellectually disabled females. Eur J Hum Genet. (2019) 27(8):1254–9. doi: 10.1038/s41431-019-0392-7
4. Nussinov R, Yavuz BR, Arici MK, Demirel HC, Zhang M, Liu Y, et al. Neurodevelopmental disorders, like cancer, are connected to impaired chromatin remodelers, PI3K/mTOR, and PAK1-regulated MAPK. Biophys Rev. (2023) 15(2):163–81. doi: 10.1007/s12551-023-01054-9
5. Mao H, Zhang L, Yang Y, Sun J, Deng B, Feng J, et al. RhoBTB2 (DBC2) functions as tumor suppressor via inhibiting proliferation, preventing colony formation and inducing apoptosis in breast cancer cells. Gene. (2011) 486(1–2):74–80. doi: 10.1016/j.gene.2011.07.018
6. Rivero F, Dislich H, Glöckner G, Noegel AA. The dictyostelium discoideum family of rho-related proteins. Nucleic Acids Res. (2001) 29(5):1068–79. doi: 10.1093/nar/29.5.1068
7. Bos JL, Rehmann H, Wittinghofer A. GEFs and GAPs: critical elements in the control of small G proteins. Cell. (2007) 129(5):865–77. doi: 10.1016/j.cell.2007.05.018
8. Van Aelst L, D'Souza-Schorey C. Rho GTPases and signaling networks. Genes Dev. (1997) 11(18):2295–322. doi: 10.1101/gad.11.18.2295
9. Ridley AJ. Rho GTPases and cell migration. J Cell Sci. (2001) 114(Pt 15):2713–22. doi: 10.1242/jcs.114.15.2713
10. Aspenström P, Fransson A, Saras J. Rho GTPases have diverse effects on the organization of the actin filament system. Biochem J. 2004 377(Pt 2):327–37. doi: 10.1042/bj20031041
11. Ramos S, Khademi F, Somesh BP, Rivero F. Genomic organization and expression profile of the small GTPases of the RhoBTB family in human and mouse. Gene. (2002) 298(2):147–57. doi: 10.1016/S0378-1119(02)00980-0
12. Scala M, Nishikawa M, Nagata K-I, Striano P. Pathophysiological mechanisms in neurodevelopmental disorders caused by rac GTPases dysregulation: what's behind neuro-RACopathies. Cells. (2021) 10(12):3395. doi: 10.3390/cells10123395
13. Barbosa S, Greville-Heygate S, Bonnet M, Godwin A, Fagotto-Kaufmann C, Kajava AV, et al. Opposite modulation of RAC1 by mutations in TRIO is associated with distinct, domain-specific neurodevelopmental disorders. Am J Hum Genet. (2020) 106(3):338–55. doi: 10.1016/j.ajhg.2020.01.018
14. Scala M, Nishikawa M, Ito H, Tabata H, Khan T, Accogli A, et al. Variant-specific changes in RAC3 function disrupt corticogenesis in neurodevelopmental phenotypes. Brain. (2022) 145(9):3308–27. doi: 10.1093/brain/awac106
15. Nishikawa M, Scala M, Umair M, Ito H, Waqas A, Striano P, et al. Gain-of-function p.F28S variant in RAC3 disrupts neuronal differentiation, migration and axonogenesis during cortical development, leading to neurodevelopmental disorder. J Med Genet. (2023) 60(3):223–32. doi: 10.1136/jmedgenet-2022-108483
16. Martinelli S, Krumbach OHF, Pantaleoni F, Coppola S, Amin E, Pannone L, et al. Functional dysregulation of CDC42 causes diverse developmental phenotypes. Am J Hum Genet. (2018) 102(2):309–20. doi: 10.1016/j.ajhg.2017.12.015
17. Boureux A, Vignal E, Faure S, Fort P. Evolution of the rho family of ras-like GTPases in eukaryotes. Mol Biol Evol. (2007) 24(1):203–16. doi: 10.1093/molbev/msl145
18. Wilkins A, Carpenter CL. Regulation of RhoBTB2 by the Cul3 ubiquitin ligase complex. Meth Enzymol. (2008) 439:103–9. doi: 10.1016/S0076-6879(07)00408-9
19. Straub J, Konrad EDH, Grüner J, Toutain A, Bok LA, Cho MT, et al. Missense variants in RHOBTB2 cause a developmental and epileptic encephalopathy in humans, and altered levels cause neurological defects in Drosophila. Am J Hum Genet. (2018) 102(1):44–57. doi: 10.1016/j.ajhg.2017.11.008
20. Langhammer F, Maroofian R, Badar R, Gregor A, Rochman M, Ratliff JB, et al. Genotype-phenotype correlations in RHOBTB2-associated neurodevelopmental disorders. Genet Med. (2023) 25(8):100885. doi: 10.1016/j.gim.2023.100885
21. Defo A, Verloes A, Elenga N. Developmental and epileptic encephalopathy related to a heterozygous variant of the RHOBTB2 gene: a case report from French Guiana. Mol Genet Genomic Med. (2022) 10(6):e1929. doi: 10.1002/mgg3.1929
22. Niu X, Sun Y, Yang Y, Cheng M, Tan Q, Zhang J, et al. RHOBTB2 Gene associated epilepsy and paroxysmal movement disorder: two cases report and literature review. Acta Epileptologica. (2021) 3(1):20. doi: 10.1186/s42494-021-00056-y
23. Knijnenburg ACS, Nicolai J, Bok LA, Bay A, Stegmann APA, Sinnema M, et al. Acute encephalopathy after head trauma in a patient with a RHOBTB2 mutation. Neurol Genet. (2020) 6(3):e418. doi: 10.1212/NXG.0000000000000418
24. Rochtus A, Olson HE, Smith L, Keith LG, El Achkar C, Taylor A, et al. Genetic diagnoses in epilepsy: the impact of dynamic exome analysis in a pediatric cohort. Epilepsia. (2020) 61(2):249–58. doi: 10.1111/epi.16427
25. Spagnoli C, Soliani L, Caraffi SG, Baga M, Rizzi S, Salerno GG, et al. Paroxysmal movement disorder with response to carbamazepine in a patient with RHOBTB2 developmental and epileptic encephalopathy. Parkinsonism Relat Disord. (2020) 76:54–5. doi: 10.1016/j.parkreldis.2020.05.031
26. Belal H, Nakashima M, Matsumoto H, Yokochi K, Taniguchi-Ikeda M, Aoto K, et al. de novo variants in RHOBTB2, an atypical rho GTPase gene, cause epileptic encephalopathy. Hum Mutat. (2018) 39(8):1070–5. doi: 10.1002/humu.23550
27. Zagaglia S, Steel D, Krithika S, Hernandez-Hernandez L, Custodio HM, Gorman KM, et al. RHOBTB2 mutations expand the phenotypic spectrum of alternating hemiplegia of childhood. Neurology. (2021) 96(11):e1539–50. doi: 10.1212/WNL.0000000000011543
28. Berthold J, Schenkova K, Rivero F. Rho GTPases of the RhoBTB subfamily and tumorigenesis. Acta Pharmacol Sin. (2008) 29(3):285–95. doi: 10.1111/j.1745-7254.2008.00773.x
29. Berthold J, Schenková K, Ramos S, Miura Y, Furukawa M, Aspenström P, et al. Characterization of RhoBTB-dependent Cul3 ubiquitin ligase complexes–evidence for an autoregulatory mechanism. Exp Cell Res. (2008) 314(19):3453–65. doi: 10.1016/j.yexcr.2008.09.005
30. Singh SS, Jois SD. Homo- and heterodimerization of proteins in cell signaling: inhibition and drug design. Adv Protein Chem Struct Biol. (2018) 111:1–59. doi: 10.1016/bs.apcsb.2017.08.003
31. Wilkins A, Ping Q, Carpenter CL. RhoBTB2 is a substrate of the mammalian Cul3 ubiquitin ligase complex. Genes Dev. (2004) 18(8):856–61. doi: 10.1101/gad.1177904
32. Zhang H-G, Wang J, Yang X, Hsu H-C, Mountz JD. Regulation of apoptosis proteins in cancer cells by ubiquitin. Oncogene. (2004) 23(11):2009–15. doi: 10.1038/sj.onc.1207373
33. Hamaguchi M, Meth JL, von Klitzing C, Wei W, Esposito D, Rodgers L, et al. DBC2, A candidate for a tumor suppressor gene involved in breast cancer. Proc Natl Acad Sci U S A. (2002) 99(21):13647–52. doi: 10.1073/pnas.212516099
34. Dong W, Meng L, Shen H-C, Du J-J. Loss of DBC2 expression is an early and progressive event in the development of lung adenocarcinoma. Asian Pac J Cancer Prev. (2012) 13(5):2021–3. doi: 10.7314/APJCP.2012.13.5.2021
35. Shi Y, Chen J-Y, Yang J, Li B, Chen Z-H, Xiao C-G. DBC2 gene is silenced by promoter methylation in bladder cancer. Urol Oncol. (2008) 26(5):465–9. doi: 10.1016/j.urolonc.2007.08.009
36. Cho YG, Choi BJ, Kim CJ, Song JH, Zhang C, Nam SW, et al. Genetic analysis of the DBC2 gene in gastric cancer. Acta Oncol. (2008) 47(3):366–71. doi: 10.1080/02841860701644094
37. Jin Z, Han Y-X, Han X-R. Downregulated RhoBTB2 expression contributes to poor outcome in osteosarcoma patients. Cancer Biother Radiopharm. (2013) 28(10):709–16. doi: 10.1089/cbr.2012.1386
38. Yoshihara T, Collado D, Hamaguchi M. Cyclin D1 down-regulation is essential for DBC2's Tumor suppressor function. Biochem Biophys Res Commun. (2007) 358(4):1076–9. doi: 10.1016/j.bbrc.2007.05.037
39. Montalto FI, De Amicis F. Cyclin D1 in cancer: a molecular connection for cell cycle control, adhesion and invasion in tumor and stroma. Cells. (2020) 9(12):2648. doi: 10.3390/cells9122648
40. Tchakarska G, Sola B. The double dealing of cyclin D1. Cell Cycle. (2020) 19(2):163–78. doi: 10.1080/15384101.2019.1706903
41. Collado D, Yoshihara T, Hamaguchi M. DBC2 resistance is achieved by enhancing 26S proteasome-mediated protein degradation. Biochem Biophys Res Commun. (2007) 360(3):600–3. doi: 10.1016/j.bbrc.2007.06.127
42. Morris-Rosendahl DJ, Crocq MA. Neurodevelopmental disorders—the history and future of a diagnostic concept. Dialogues Clin Neurosci. (2020) 22(1):65–72. doi: 10.31887/DCNS.2020.22.1/macrocq
43. American Psychiatric Association. Diagnostic and Statistical Manual of Mental Disorders. 5th ed. Washington, DC: American Psychiatric Association (2013). doi: 10.1176/appi.books.9780890425596
44. Shimizu H, Morimoto Y, Yamamoto N, Tayama T, Ozawa H, Imamura A. Overlap between epilepsy and neurodevelopmental disorders: insights from clinical and genetic studies. In: Czuczwar SJ, editor. Epilepsy. Brisbane (AU): Exon Publications (2022). p. 41–54. doi: 10.36255/exon-publications-epilepsy-neurodevelopmental-disorders
45. Shankar R, Perera B, Thomas RH. Epilepsy, an orphan disorder within the neurodevelopmental family. J Neurol Neurosurg Psychiatry. (2020) 91(12):1245–7. doi: 10.1136/jnnp-2020-324660
46. Scheffer IE, Berkovic S, Capovilla G, Connolly MB, French J, Guilhoto L, et al. ILAE classification of the epilepsies: position paper of the ILAE commission for classification and terminology. Epilepsia. (2017) 58(4):512–21. doi: 10.1111/epi.13709
47. Woods CG, Parker A. Investigating microcephaly. Arch Dis Child. (2013) 98(9):707–13. doi: 10.1136/archdischild-2012-302882
48. Zubler JM, Wiggins LD, Macias MM, Whitaker TM, Shaw JS, Squires JK, et al. Evidence-informed milestones for developmental surveillance tools. Pediatrics. (2022) 149(3):e2021052138. doi: 10.1542/peds.2021-052138
49. Harvey S, King MD, Gorman KM. Paroxysmal movement disorders. Front Neurol. (2021) 12:659064. doi: 10.3389/fneur.2021.659064
50. Bourgeois M, Aicardi J, Goutières F. Alternating hemiplegia of childhood. J Pediatr. (1993) 122(5 Pt 1):673–9. doi: 10.1016/S0022-3476(06)80003-X
51. Chen J, Zhao X, Cui L, He G, Wang X, Wang F, et al. Genetic regulatory subnetworks and key regulating genes in rat hippocampus perturbed by prenatal malnutrition: implications for major brain disorders. Aging (Albany NY). (2020) 12(9):8434–58. doi: 10.18632/aging.103150
52. LePage KT, Dickey RW, Gerwick WH, Jester EL, Murray TF. On the use of neuro-2a neuroblastoma cells versus intact neurons in primary culture for neurotoxicity studies. Crit Rev Neurobiol. (2005) 17(1):27–50. doi: 10.1615/CritRevNeurobiol.v17.i1.20
53. Leroy H, Roumier C, Huyghe P, Biggio V, Fenaux P, Preudhomme C. CEBPA point mutations in hematological malignancies. Leukemia. (2005) 19(3):329–34. doi: 10.1038/sj.leu.2403614
54. Engel T, Henshall DC. Apoptosis, bcl-2 family proteins and caspases: the ABCs of seizure-damage and epileptogenesis? Int J Physiol Pathophysiol Pharmacol. (2009) 1(2):97–115.21383882
55. Freeman SN, Ma Y, Cress WD. RhoBTB2 (DBC2) is a mitotic E2F1 target gene with a novel role in apoptosis. J Biol Chem. (2008) 283(4):2353–62. doi: 10.1074/jbc.M705986200
56. Choi YM, Kim KB, Lee JH, Chun YK, An IS, An S, et al. DBC2/RhoBTB2 functions as a tumor suppressor protein via musashi-2 ubiquitination in breast cancer. Oncogene. (2017) 36(20):2802–12. doi: 10.1038/onc.2016.441
57. Ling L-J, Lu C, Zhou G-P, Wang S. Ectopic expression of RhoBTB2 inhibits migration and invasion of human breast cancer cells. Cancer Biol Ther. (2010) 10(11):1115–22. doi: 10.4161/cbt.10.11.13431
58. Tanoli Z, Vähä-Koskela M, Aittokallio T. Artificial intelligence, machine learning, and drug repurposing in cancer. Expert Opin Drug Discov. (2021) 16(9):977–89. doi: 10.1080/17460441.2021.1883585
59. Foksinska A, Crowder CM, Crouse AB, Henrikson J, Byrd WE, Rosenblatt G, et al. The precision medicine process for treating rare disease using the artificial intelligence tool mediKanren. Front Artif Intell. (2022) 5:910216. doi: 10.3389/frai.2022.910216
60. Gong L, Thorn CF, Bertagnolli MM, Grosser T, Altman RB, Klein TE. Celecoxib pathways: pharmacokinetics and pharmacodynamics. Pharmacogenet Genomics. (2012) 22(4):310–8. doi: 10.1097/FPC.0b013e32834f94cb
61. Guruswamy S, Rao CV. Synergistic effects of lovastatin and celecoxib on caveolin-1 and its down-stream signaling molecules: implications for colon cancer prevention. Int J Oncol. (2009) 35(5):1037–43. doi: 10.3892/ijo_00000418
62. Valle BL, D'Souza T, Becker KG, Wood WH, Zhang Y, Wersto RP, et al. Non-steroidal anti-inflammatory drugs decrease E2F1 expression and inhibit cell growth in ovarian cancer cells. PLoS One. (2013) 8(4):e61836. doi: 10.1371/journal.pone.0061836
Keywords: neurodevelopmental disorder, developmental and epileptic encephalopathy, RHOBTB2-related disorders, cancer, tumor suppressor, precision medicine, genetic variants
Citation: Solano E, Foksinska A and Crowder CM (2024) Variants in RHOBTB2 associated with cancer and rare developmental and epileptic encephalopathy. Front. Pediatr. 12:1448793. doi: 10.3389/fped.2024.1448793
Received: 14 June 2024; Accepted: 29 November 2024;
Published: 16 December 2024.
Edited by:
Bruria Ben-Zeev, Sheba Medical Center, IsraelCopyright: © 2024 Solano, Foksinska and Crowder. This is an open-access article distributed under the terms of the Creative Commons Attribution License (CC BY). The use, distribution or reproduction in other forums is permitted, provided the original author(s) and the copyright owner(s) are credited and that the original publication in this journal is cited, in accordance with accepted academic practice. No use, distribution or reproduction is permitted which does not comply with these terms.
*Correspondence: Camerron M. Crowder, Y2FtZXJyb25AdWFiLmVkdQ==
Disclaimer: All claims expressed in this article are solely those of the authors and do not necessarily represent those of their affiliated organizations, or those of the publisher, the editors and the reviewers. Any product that may be evaluated in this article or claim that may be made by its manufacturer is not guaranteed or endorsed by the publisher.
Research integrity at Frontiers
Learn more about the work of our research integrity team to safeguard the quality of each article we publish.