- Neonatal Intensive Care Unit, Santa Maria Hospital, Terni, Italy
Bronchopulmonary dysplasia (BPD) is a common morbidity affecting preterm infants and is associated with substantial long-term disabilities. The pathogenesis of BPD is multifactorial, and the clinical phenotype is variable. Extensive research has improved the current understanding of the factors contributing to BPD pathogenesis. However, effectively preventing and managing BPD remains a challenge. This review aims to provide an overview of the current evidence regarding the prevention of BPD in preterm infants, offering practical insights for clinicians.
Introduction
Bronchopulmonary dysplasia (BPD) is the result of a complex process in which several prenatal and/or postnatal factors interfere with lower respiratory tract development, leading to a severe, lifelong disease (1). BPD was first described by Northway et al. (2) based on radiographic and histological evidence of pulmonary disease.
BPD used to be a significant concern for all preterm infants requiring prolonged invasive mechanical ventilation. However, with the widespread adoption of antenatal corticosteroids, surfactant therapy, and gentle ventilation strategies, BPD has become rare among preterm infants with birth weights greater than 1,500 g (3). Despite these advancements, recent data suggest that BPD rates have not improved and may even be increasing among extremely preterm infants (4). In this review, we summarize the available evidence for the different pharmacological agents currently in use to prevent BPD, primarily focusing on findings from randomized controlled trials (RCTs). Table 1 provides a summary of strategies commonly used for the prevention of BPD.
Definitions of BPD across the ages
BPD was first described in moderately preterm infants during a time when supplemental oxygen was the primary treatment for severe respiratory distress syndrome (RDS) and mortality rates exceeded 50%. Since then, the definition of BPD has evolved (Figure 1), largely due to the improved survival rates of extremely preterm infants and the development of new support modalities.
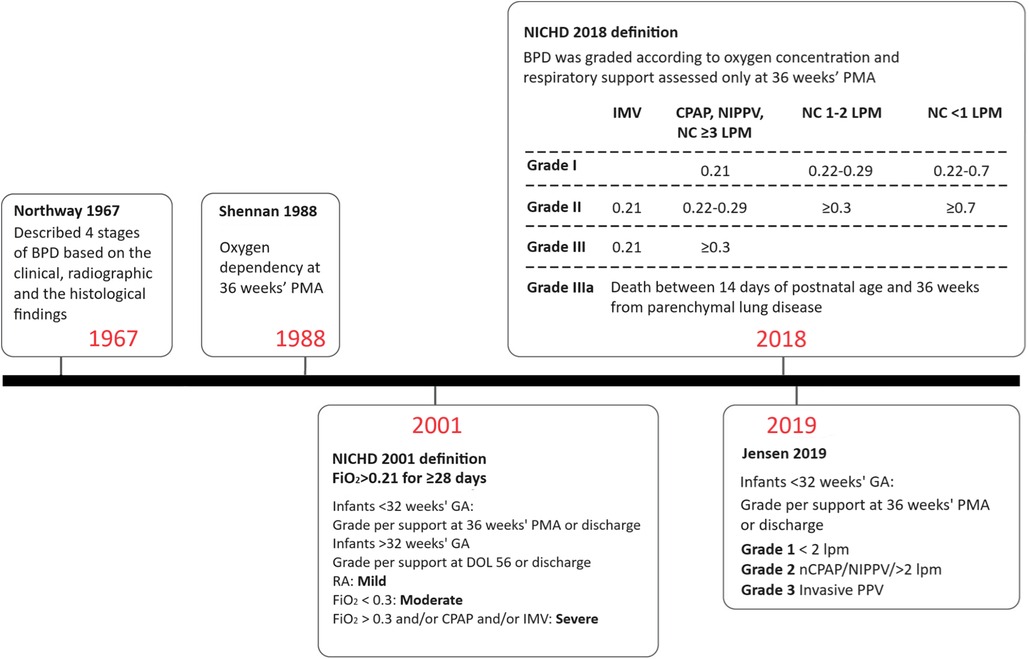
Figure 1 Definitions of BPD over time. BPD, bronchopulmonary dysplasia; CPAP, continuous positive airway pressure; DOL, day of life; FiO2, fraction of inspired oxygen; GA, gestational age; IMV, invasive mechanical ventilation; LPM, liters per minute; NC, nasal cannula; NIPPV, nasal intermittent positive pressure ventilation; PMA, postmenstrual age; RA, room air.
The original definition of BPD was published by Northway in 1967. Affected infants were born during the late saccular or alveolar stages of lung development and were exposed to high oxygen concentrations and high peak airway pressures during mechanical ventilation (2, 5). Northway described four stages of BPD based on clinical, radiographic, and histopathological findings (6). Preterm infants with BPD initially presented with acute respiratory distress syndrome, which evolved into a chronic disease after day 28 of life. Chest radiograph findings correlated with histopathological changes, showing an initial acute inflammatory stage followed by chronic inflammation resulting in fibrosis.
The 2001 National Heart, Lung, and Blood Institute (NHLBI) workshop definition took the important step of including a BPD severity scale (7), and infants with severe BPD were subsequently found to have higher mortality and rates of adverse outcomes after discharge than those with mild or moderate disease (8). However, the definition of severe BPD was overly broad, and combined infants receiving 31% oxygen by low flow nasal cannula with infants on high ventilator settings and receiving drugs for pulmonary hypertension. This issue was partly addressed by the NHLBI 2018 revision, which reserved grade 3 BPD for infants receiving positive pressure or nasal cannula flow >3 L/min in addition to oxygen (9).
Most recently, Jensen et al. analyzed 18 prespecified BPD definitions based on the respiratory support level and supplemental oxygen at 36 weeks postmenstrual age (PMA) to determine which definition best correlated with early childhood morbidity. The study demonstrated that a grading system based on respiratory support, regardless of oxygen level, best predicted late death or serious respiratory morbidity at 18–26 months corrected age (10). The Jensen definition classifies BPD severity in infants at 36 weeks PMA into grade 1 for those requiring 2 L/min nasal cannula or less, grade 2 for those requiring more than 2 L/min nasal cannula or other forms of non-invasive ventilation support, and grade 3 for those requiring invasive mechanical ventilation (10).
Several limitations remain with the revised definition (11). Firstly, the decision for respiratory support still lies with the clinician. These decisions are often based on subjective assessments of the infant's work of breathing, frequency of apnea/bradycardic events, ability to take adequate oral feeds or maintenance of an optimal growth trajectory. At 36 weeks’ PMA, preterm infants may still exhibit immature respiratory control, manifesting as periodic breathing or apneic events. The use of diuretics (12) and bronchodilators (13), which can influence the need for respiratory support, varies markedly between centers (14) and may affect the reporting of BPD. Additionally, the revised definition does not differentiate infants with BPD-associated pulmonary hypertension (BPD-PH).
Epidemiology
Prevalence
Premature birth (<37 weeks gestational age) is common and affects 6%–14% of pregnancies, depending on the country (15, 16). Premature infants are generally classified based on gestational age [extremely preterm infants or extremely low gestational age newborns (ELGANs) are <28 weeks gestation; very preterm infants are 28 to <32 weeks gestation, moderate preterm infants are 32 to <34 weeks and late preterm infants are 34 to <37 weeks] or birth weight (ELBW infants are <1,000 g, very-low-birth-weight (VLBW) infants are <1,500 g, and low-birth-weight (LBW) infants are <2,500 g). BPD incidence increases as gestational age and weight at birth decreases. BPD remains the most common complication associated with prematurity and is increasing in prevalence, most likely due to the increased survival of ELGANs (17, 18). Data from major cohort studies (such as ELGAN, Canadian Neonatal Network, Korean Neonatal Network, Vermont–Oxford Network, and Swiss Neonatal Network, as well as studies in China, Taiwan, and India) demonstrate a BPD prevalence of 11%–50%, a wide range that is due to differences in gestational age or birth weight criteria for a BPD diagnosis (19–25).
Risk factors
The strongest risk factors for BPD are prematurity and low birth weight (26–29). Nearly 80% of infants born at 22–24 weeks of gestation are diagnosed with BPD (30), while only 20% of those born at 28 weeks develop the condition. Among infants with BPD, 95% are VLBW (31). Other perinatal risk factors include intrauterine growth restriction (IUGR) (21), male sex (21, 29), and, inconsistently, chorioamnionitis (32), race or ethnicity (21, 27, 29), and maternal smoking (33, 34). Genetic factors may also contribute to BPD development, as suggested by twin studies (35, 36), and research is ongoing to identify specific genetic markers associated with BPD (37, 38).
Evaluation
The evaluation of BPD involves assessing blood gases, chest x-rays, and the nutritional status of the patient (39). An arterial blood gas analysis may reveal hypoxia, hypercarbia, or acidosis. Patients with BPD are monitored with continuous pulse oximetry to maintain adequate oxygen saturation levels. Many centers also use transcutaneous carbon dioxide monitoring to assess the infant's ventilation.
Among imaging modalities, plain chest radiography and computed tomography scanning remain the most extensively studied in BPD (40). Chest radiographic features of established BPD include interstitial thickening, focal or generalized hyperexpansion, and atelectasis (Figure 2) (41). High-resolution CT scans can reveal abnormalities not easily seen with routine chest radiography. Although computed tomography findings were more specific for BPD than those of plain radiographs (42, 43), the considerable exposure to radiation, increased cost of the procedure, and need for either patient cooperation or deep sedation have limited the use of computed tomography. The European Respiratory Society (ERS) guidelines recommend follow-up imaging with ionizing radiation only in the most severely affected patients (44).
In contrast, ultrasound does not require exposure to ionizing radiation and can be performed at the patient's bedside. An ultrasound scoring system involving the evaluation of three different areas of the lung with a semi-quantitative score has shown some potential for predicting moderate to severe BPD (45).
Infants with moderate or severe BPD should be screened for pulmonary hypertension (PH) at 36 weeks PMA using an echocardiogram (46, 47). Some centers choose to screen all patients with BPD for PH due to the high morbidity and mortality associated with this condition. There are known limitations in the sensitivity and specificity of echocardiography to identify PH, and cardiac catheterization is recommended when there are uncertain findings, concerns for inadequate response to pulmonary vasodilators, or more precise measures of pulmonary pressures and vasoreactivity are needed (48).
Lung protective ventilation strategies
Successful transition to postnatal breathing requires the clearance of fetal lung fluid and adequate lung aeration. However, several factors in very preterm infants can hinder this process, including high chest wall compliance, weak respiratory muscles, incomplete surfactant production, and underexpression of transepithelial sodium channels (49–51). As a result, many very preterm infants require positive airway pressure and supplemental oxygen shortly after birth to maintain physiological stability. While invasive mechanical ventilation can be life-saving in these cases, it also poses a risk of lung injury. Animal studies have demonstrated a clear connection between baro- and volutrauma induced by mechanical ventilation and pathological changes in the lung resembling BPD (52, 53). Additionally, observational studies have suggested a link between invasive mechanical ventilation and an increased risk of BPD (26, 54). To mitigate lung injury and prevent BPD, researchers have investigated various noninvasive and gentler invasive ventilation strategies. Salient data from randomized controlled trials on the effects of various respiratory support strategies for prevention are summarized in Table 2.
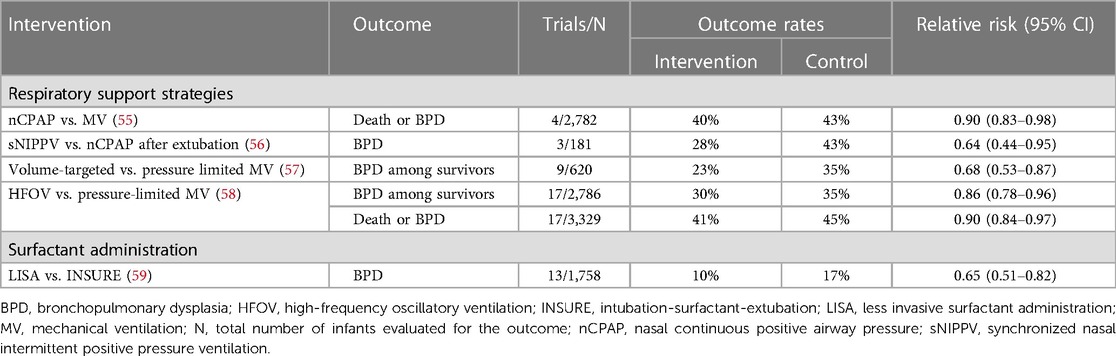
Table 2 Summary of randomized, controlled trial data on the effects of various respiratory support strategies for preventing death and/or bronchopulmonary dysplasia.
Noninvasive positive airway pressure
One strategy to prevent ventilator-induced lung injury is to avoid mechanical ventilation altogether. Three large RCTs have compared early noninvasive continuous positive airway pressure (CPAP) with immediate intubation and surfactant administration. Despite variations in design elements, including the gestational ages of enrolled infants and initial CPAP settings (ranging from 5 to 8 cm H2O), each study demonstrated a nonsignificant reduction in the rate of death or BPD at 36 weeks’ PMA among infants initially treated with CPAP (60–62). Meta-analyses of the available trial data, some of which also included smaller RCTs, demonstrated a small but statistically significant reduction in the risk for death or BPD with CPAP therapy (Table 2). Although one large trial reported higher rates of pneumothorax in CPAP-treated infants, meta-analyses did not show an increased risk for pneumothorax or other adverse events with early CPAP (55, 63, 64). Consequently, the American Academy of Pediatrics Committee on Fetus and Newborn recommends early use of CPAP with subsequent selective surfactant administration in extremely preterm infants as an evidence-based strategy to reduce the risk of death or BPD (65).
Heated and humidified high-flow nasal cannula (HFNC), typically administered with flow rates higher than 1 to 2 L/min, has gained popularity as an alternative to nCPAP. HFNC offers potential advantages such as reduced nasal trauma, simpler device setup, and greater facilitation of oral feeding and skin-to-skin care (66). However, recent trial data suggest that HFNC and nasal CPAP may not be equivalent therapies. Treatment failure is more common among very preterm infants who receive HFNC compared with nasal CPAP as a primary support modality. While HFNC may be an acceptable alternative to nasal CPAP for post-extubation support among infants born at 28 weeks of gestation or more, routine use in less mature infants is not recommended (67).
Nasal intermittent positive pressure ventilation (NIPPV) is a form of non-invasive ventilation that delivers a baseline distending pressure similar to CPAP but with the addition of superimposed peak inspiratory pressures at intervals (68). NIPPV may be synchronized (SNIPPV) or non-synchronized to the infant's breathing efforts. A Cochrane analysis of ten studies including 1,061 infants comparing early NIPPV and early CPAP use determined that even though infants randomized to early NIPPV had reduced risk of requiring intubation (RR 0.78 CI 0.64–0.94) and respiratory failure (RR 0.65 CI 0.51–0.82), there was no reduction in the risk of BPD among infants who received NIPPV (69). Another meta-analysis of the use of NIPPV vs. CPAP in preterm infants after extubation found a reduction in BPD associated with synchronized NIPPV (RR 0.64, 95% CI 0.44–0.95) on subgroup analysis, but in the overall cohort no difference was found in the rates of BPD between the two groups (RR 0.94, 95% CI 0.80–1.10) (56).
Mechanical ventilation
Mechanical ventilation remains an essential tool in the care of critically sick and very preterm infants, despite improvements in perinatal care including increased use of antenatal steroids and non-invasive respiratory support. Studies from CPAP trials suggest that as many as 65% of spontaneously breathing extremely preterm infants may still require intubation and mechanical ventilation despite early CPAP therapy (55). In such cases, or when invasive respiratory support is needed soon after birth, clinicians must carefully choose a mode of mechanical ventilation.
Time-cycled pressure-limited ventilation (PLV) has been traditionally used for newborn infants. This form of ventilation uses a designated volume of gas with a preset peak inspiratory pressure (PIP), over a defined time cycle. Both overexpansion (volutrauma) and under expansion/collapse (atelectrauma) have been previously reported during the use of PLV (70–72). It has also been reported that tidal volume (VT), rather than inflation pressure, is the main determinant of ventilator-induced lung injury (VILI) (73). In volume-targeted ventilation (VTV), automatic adjustments are made to the peak positive pressure and the duration of the ventilator cycle to maintain a target VT. VTV mode has been proposed as a means to reduce VILI caused by ventilation with excessive or insufficient VT during conventional pressure-controlled ventilation. A 2017 Cochrane review provided moderate-quality evidence supporting the use of volume-targeted ventilation over pressure-limited ventilation to reduce the composite outcome of death or BPD, length of mechanical ventilation, and rates of severe intraventricular hemorrhage (57).
High-frequency oscillatory ventilation (HFOV) is another ventilation strategy that may help mitigate lung injury. A 2015 Cochrane review evaluating HFOV as a primary mode of invasive respiratory support found a small reduction in the risk for death or BPD, as well as BPD alone, among infants treated with HFOV compared to pressure-limited conventional ventilation (58). However, pulmonary air leaks, such as pneumothorax or pulmonary interstitial emphysema, were more common in infants treated with HFOV (58).
Surfactant administration
Endogenous pulmonary surfactant plays a crucial role in reducing surface tension at the air/liquid interface within the alveoli, thereby enhancing lung deflation stability (74). In extremely preterm infants, surfactant deficiency is a central aspect of the pathophysiology of neonatal respiratory distress syndrome (RDS) (75). Several older RCTs demonstrated that administration of exogenous surfactant, compared with mechanical ventilation alone, reduces rates of death or the need for supplemental oxygen 28 days after birth (the conventional definition of BPD at that time) (76–78).
To optimize the potential advantages of early surfactant administration without the harmful effects of prolonged invasive mechanical ventilation, Victorin et al. introduced the technique known as intubation, surfactant administration during brief mechanical ventilation, followed by extubation (INSURE) (79). Initial RCTs suggested that INSURE reduces the need for supplemental oxygen at 28 days of age. However, meta-analyses incorporating more recent trials have indicated that compared to CPAP, INSURE does not decrease the risk of death or BPD (RR 0.88, 95% CI 0.76–1.02) (80, 81).
Several techniques have been developed to administer surfactant avoiding traditional endotracheal intubation (82). Among these strategies, surfactant instillation via a thin catheter, commonly known as less invasive surfactant administration (LISA) has been extensively studied. Four RCTs conducted in extremely preterm infants compared LISA with endotracheal tube administration of surfactant (three trials vs. INSURE, one trial vs. continued mechanical ventilation after surfactant therapy), while one trial compared LISA with CPAP therapy alone (83–86). A recent meta-analysis (59) showed that LISA vs. INSURE reduced the risk for BPD (RR 0.65, 95% CI 0.51–0.82) and the rate of mortality (RR 0.76, 95% CI 0.58–1.00).
Oxygen saturation targets
Exposure to supraphysiological oxygen has been associated with BPD, making the definition of optimal oxygen saturation targets a critical area of study. Infants born at less than 30 weeks gestation randomized to a high-saturation target (95%–98%) have a significantly higher risk of needing supplemental oxygen at 36 weeks compared to those randomized to a target of 92%–94% [odds ratio (OR) 1.40, 95% CI 1.15–1.70] (87). An individual patient meta-analysis of the five RCTs of the Neonatal Oxygen Prospective Meta-Analysis (NeOProM) Collaboration examined restricted (85%–89%) vs. liberal (91%–95%) oxygen saturation targets in infants less than 28 weeks gestation. The analysis found no significant difference in the composite outcome of death or major neurodevelopmental outcomes, or severe visual problems at 18–24 months between the two groups (RR 1.04, 95% CI 0.98–1.09) (88). Significantly fewer infants in the restricted oxygen saturation target group received supplemental oxygen at 36 weeks PMA (RR 0.81, 95% CI 0.74–0.90), but there was also an increase in the risk of death (RR 1.17, 95% CI 1.04–1.31) and necrotizing enterocolitis (NEC) (RR 1.33, 95% CI 1.10–1.61) in this group (89). While further studies are needed to make definitive conclusions, some authors suggest maintaining oxygen saturation targets between 88% and 92%, with a higher alarm limit of 96% (90).
Pharmacologic therapies
Despite the physiological and observational evidence linking invasive mechanical ventilation to the development of BPD, the beneficial effects of the respiratory support strategies described above are modest. Longitudinal data also suggest that the increased use of noninvasive respiratory support over time has not been accompanied by substantial improvements in BPD rates among surviving extremely preterm infants (91). Given the limited benefit of gentle ventilation techniques, pharmacologic therapies are becoming an essential component in ongoing efforts to reduce BPD rates (92). Drug therapies demonstrated in randomized controlled trials to reduce BPD are summarized below and in Table 3.
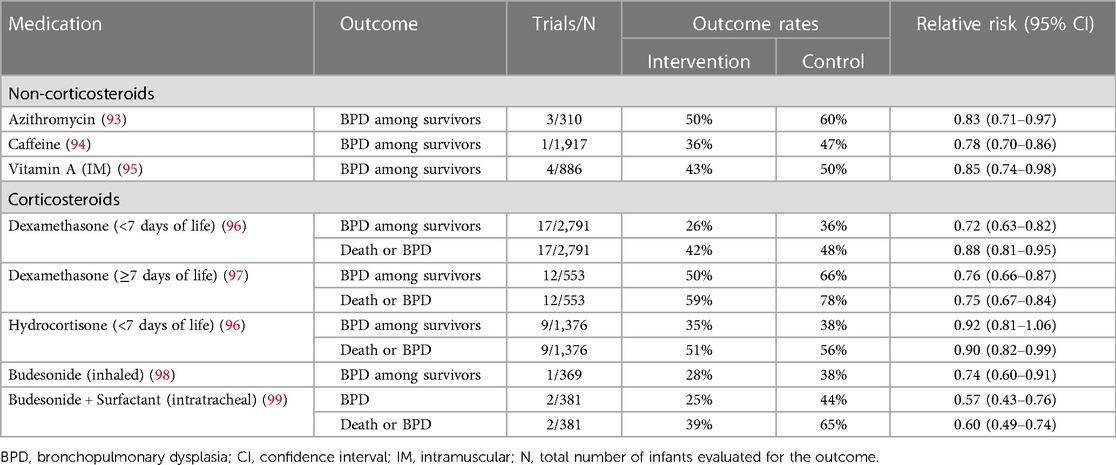
Table 3 Summary of randomized, controlled trial data on the effects of various medications for preventing death and/or bronchopulmonary dysplasia.
Noncorticosteroid agents
Azithromycin
Azithromycin is a macrolide antibiotic that possesses both antimicrobial and anti-inflammatory properties (100, 101), making it a potentially attractive option for preventing BPD. In very preterm infants, Ureaplasma infection is linked to BPD development (102–104). Additionally, lung and systemic inflammation contribute to BPD pathophysiology (105, 106). Three small trials assessed azithromycin's efficacy in preventing BPD (93). A meta-analysis of these studies revealed a reduction in the risk for BPD and BPD/death among infants treated with azithromycin, irrespective of known Ureaplasma colonization or infection. However, the quality of evidence was low (93, 107). Furthermore, trials evaluating other macrolides have not shown benefits for preventing BPD (107, 108). Larger trials are necessary to establish the safety and efficacy of prophylactic azithromycin before recommending this therapy.
Caffeine
Caffeine is a central nervous system (CNS) stimulant of the methylxanthine class that primarily acts by inhibiting the adenosine receptors A1 and A2A in the brain (109, 110). In addition, caffeine improves diaphragmatic contractility and prevents diaphragmatic fatigue by increasing intracellular Ca2+ and responsiveness of the central and peripheral chemoreceptors to CO2, resulting in increased minute ventilation (111).
The Caffeine for Apnea of Prematurity (CAP) trial was a pivotal study in the field of neonatology (94). This multicenter RCT investigated the long-term impacts of caffeine administration, initiated before ten days of age (median: 3 days), on infants <1,500 g. The trial examined a combined outcome of death and neurodevelopmental progress at 18–21 months of corrected age. The findings confirmed the safety of prolonged caffeine use in premature infants and demonstrated a significant reduction in the incidence of BPD with early caffeine administration (p < 0.001). By preventing apnea of prematurity, caffeine reduces the need for intubation and promotes early, successful extubation of ventilated infants, thereby reducing mechanical ventilation-associated lung injury, a critical factor in the development of BPD. Additionally, the CAP trial revealed that only 33.8% of infants treated with caffeine required medical or surgical closure of patent ductus arteriosus (PDA), compared to 50.7% in the placebo group (p < 0.001). Follow-up data collected through age 11 years indicated sustained, long-term improvement in motor function with caffeine therapy (112).
The benefits of caffeine in BPD prevention also depend on the timing of initiation of therapy. Since the CAP trial, the definition of early caffeine administration has evolved and is now defined as caffeine administration before three days of age. A retrospective study found that initiating caffeine treatment within the first three days of life was significantly associated with lower rates of BPD and the combined outcome of death or BPD compared to late caffeine administration (≥3 days) (113). Several RCTs and meta-analyses have confirmed this finding (114–117). The National Institute for Health and Care Excellence (NICE) recommends routine caffeine use for all premature infants ≤30 weeks’ GA soon after birth (118).
Vitamin A
Vitamin A plays a crucial role in the growth and maturation of epithelial cells lining the respiratory tract (119, 120). Previous studies have indicated that preterm infants who develop BPD tend to have lower plasma vitamin A levels (121–123). Subsequent large-scale trials, such as a multicenter trial published in 1999, found that intramuscular injections of vitamin A during the first 4 weeks of age reduced rates of death or BPD and BPD alone among surviving extremely low-birthweight infants (124). Meta-analyses of these trial data have confirmed a small benefit for reducing BPD among survivors (95). However, recent observational studies have raised questions about the effectiveness of vitamin A in the current era, with some studies showing similar rates of BPD among infants who received vitamin A and untreated controls (125, 126). Ongoing research, including randomized controlled trials investigating enteral vitamin A, may help resolve these conflicting findings. However, until more definitive results are available, intramuscular vitamin A administration, if commercially available, remains a recommended evidence-based strategy to prevent BPD in extremely preterm infants.
Corticosteroids
The potent anti-inflammatory properties of corticosteroids make them a logical therapeutic agent for BPD prevention.
Dexamethasone (systemic)
Dexamethasone has been extensively studied for preventing BPD, with trials categorizing its use into early initiation within the first 8 days of age and late initiation thereafter. A recent Cochrane review highlighted that early dexamethasone therapy reduces BPD risk but elevates the risks for gastrointestinal perforation, hypertrophic cardiomyopathy, cerebral palsy (CP), and major neurosensory disability (96). Due to these adverse effects, early dexamethasone for BPD prevention is not recommended.
The risks and benefits of “late” dexamethasone are not as well-defined. Meta-analysis of available trial data indicates that initiating dexamethasone after the first week of age reduces the risk of BPD but is associated with short-term side effects like hyperglycemia, glycosuria, and hypertension (96). Unlike early use, recent meta-analyses have not shown clear evidence of increased risk of CP among surviving infants treated with late dexamethasone (96). However, long-term outcome evaluations in follow-up studies lacked adequate power, and the high rates of open-label dexamethasone use in these studies may obscure actual treatment effects (97, 127).
If a clinician opts to administer dexamethasone, they must then determine the dose and treatment duration. While there is a general consensus favoring the use of low, tapering doses administered for short periods (typically 1–2 weeks at most), there is limited robust data to guide these specific choices (128). One dosing regimen used in the discontinued Dexamethasone: A Randomized Trial (DART) study involved administering 0.89 mg/kg over 10 days (129). In this trial involving 70 very preterm infants receiving invasive mechanical ventilation, dexamethasone significantly improved rates of successful extubation (60% in the dexamethasone group vs. 12% in the placebo group) without evidence of long-term harm (129, 130). However, the risk for BPD was not significantly reduced in the dexamethasone-treated infants (odds ratio 0.58, 95% CI 0.13–2.66) (129).
Hydrocortisone (systemic)
Randomized trials of hydrocortisone involving human infants are limited. The PREMILOC trial, the largest among them, compared a 10-day course of low-dose hydrocortisone initiated within the first 24 h after birth with placebo in infants born at less than 28 weeks’ gestation (131). Hydrocortisone significantly improved BPD-free survival at 36 weeks’ PMA (60% vs. 51%; p = 0.04) (131). However, a subgroup analysis revealed a nearly twofold increase in the risk of late-onset sepsis among infants born at 24–25 weeks’ gestation treated with early hydrocortisone (131). Furthermore, hydrocortisone did not improve neurodevelopmental outcomes at 2 years despite reducing the incidence of death or BPD (132). Meta-analysis of all available trials initiating hydrocortisone in the first week of age indicated a reduction in the composite outcome of death or BPD with hydrocortisone therapy but no benefit for BPD among survivors (96). Gastrointestinal perforation was more common in the hydrocortisone-treated infants (96).
The STOP-BPD trial was a double-blinded RCT in which 372 infants <30 weeks and/or 1,250 g birthweight were randomly assigned to receive systemic hydrocortisone (72.5 mg/kg over 22 days) or a placebo. Infants were enrolled if they were dependent on mechanical ventilation (MV) in the 2nd week of life. There was no significant difference in the BPD incidence at 36 weeks’ PMA or the composite outcome of death or BPD at 36 weeks’ PMA with hydrocortisone use. However, death at 36 weeks’ PMA was higher in the placebo group. Despite an initial decrease in extubation failure with hydrocortisone, this difference was not seen 21 days after starting the treatment (133).
Budesonide (inhaled)
Inhaled corticosteroids offer the potential benefit of reducing inflammation in the lung without the adverse effects associated with systemic corticosteroids administration. Research has investigated the efficacy of four different inhaled steroids (budesonide, beclomethasone, fluticasone, and flunisolide) in preventing BPD through RCTs (134, 135). A meta-analysis of all trial data, incorporating all four steroids, revealed a reduced risk of BPD among surviving infants as well as the composite outcome of death or BPD in infants treated with inhaled corticosteroids (134). However, these favorable results are primarily driven by the NEUROSIS trial, which found that inhaled budesonide decreased BPD rates among survivors (98, 136). It's important to note that this benefit was accompanied by higher mortality among infants treated with budesonide, with similar rates of neurodevelopmental impairment observed between the two study groups (136). Although the cause of the increased mortality in the budesonide group remains unidentified, this concerning finding outweighs the observed benefit for BPD.
Two RCTs evaluated the usefulness of intratracheal budesonide combined with surfactant relative to surfactant therapy alone among very low birth weight infants with severe RDS (99). The combined therapy reduced the risk of death or BPD. Follow-up performed up to 3 years of age found no difference in motor or cognitive function between the groups (99). This promising finding awaits confirmation in larger trials before widespread use is recommended.
Nutritional strategies
The ability to maintain lung growth and repair is dependent on adequate postnatal nutrition. A retrospective cohort study revealed that both lower energy intake during the first four weeks of life and increased fluid intake were significantly associated with BPD (137).
Breast milk is well known for its protective effect against NEC, and has also been studied for its role in preventing BPD (138–140). In a multicenter cohort study of 1,587 preterm infants who received an exclusively human breast milk-based diet, the incidence of BPD was significantly lower compared to infants who received either preterm formula or maternal breast milk with bovine fortifier (56.3% vs. 47.7%, p = 0.0015) (140). A pooled meta-analysis of eight observational studies showed a BPD protective effect of donor human milk compared to formula when used as a supplement to the mother's own milk (RR 0.78, 95% CI 0.67–0.90) (140). However, a meta-analysis of three RCTs found no statistically significant difference in BPD between infants receiving donor human milk compared to preterm formula when the mother's own milk was unavailable (RR 0.89, 95% CI 0.60–1.32) (139).
Unproven interventions
Certainly, while many medications and care strategies hold promise for preventing BPD, several have ultimately been found ineffective in reducing its risk through RCTs. Although delving into each of these therapies is beyond the scope of this article, it's worth mentioning a few of the more commonly considered strategies.
Antenatal corticosteroids
In 1972, Liggins et al. demonstrated that antenatal corticosteroids (ACS) promote lung maturation and prevent RDS in preterm infants (141). Approximately two decades later, the National Institute of Health (NIH) consensus panel recommended the use of ACS for all impending preterm births between 24 and 34 weeks of gestation (142). ACS promote lung maturation through several mechanisms. They induce the differentiation of alveolar epithelial cells into type II pneumocytes and increase the expression of surfactant proteins (SP)-A and SP-B, which enhances overall surfactant production. Additionally, ACS improve pulmonary blood flow by activating endothelial nitric oxide synthase and increase the activity of epithelial sodium channels, thereby improving respiratory function (143–146).
The American College of Obstetrics and Gynecology (ACOG) recommends specific regimens for ACS administration. For pregnant women between 24 0/7 and 33 6/7 weeks of gestation at risk of delivery within seven days, the recommended regimen is either two doses of intramuscular (IM) betamethasone or four doses of IM dexamethasone (147). In cases of pregnancies between 23 0/7 and 23 6/7 weeks of gestation, ACS should be administered if resuscitation is desired. Additionally, for women between 34 0/7 and 36 6/7 weeks of gestation who are at risk of preterm delivery within seven days and have not previously received ACS, administration is also recommended (147). Despite a clear improvement in mortality and RDS, the 2020 Cochrane review comparing ACS with placebo noted no significant difference in the incidence of BPD between the two groups (148).
Treatment of a patent ductus arteriosus
Observational data strongly suggest a link between the presence of a PDA and the development of BPD in preterm infants (149, 150). However, despite this association, no medication specifically targeting ductal closure (indomethacin, ibuprofen, or acetaminophen) administered either prophylactically or after identifying a “hemodynamically significant” PDA, has been shown to reduce the risk of BPD (151–155). Surgical ligation is effective in achieving closure of the PDA but may increase the risk for BPD and long-term neurodevelopmental impairment (156, 157). Given the lack of conclusive evidence surrounding PDA closure to prevent BPD and the adverse effects of all available management strategies, there is an increasing trend towards a more conservative approach of “watchful waiting” across centers (158).
Fluid restriction and diuretics
Excessive fluid intake in extremely low-birthweight infants can lead to complications such as pulmonary edema, necessitating greater respiratory support and potentially contributing to the development of BPD. Observational data have suggested that infants receiving higher fluid intake, particularly those with less weight loss in the first 1–2 weeks of life, are at an increased risk of developing BPD (150). However, studies comparing restrictive fluid administration with more liberal approaches have not consistently shown clear benefits in reducing the incidence of BPD (159). While restrictive fluid strategies may help mitigate the risk of pulmonary edema, their impact on BPD risk remains uncertain.
Diuretics are sometimes used to manage pulmonary edema in preterm infants, offering short-term improvement in respiratory mechanics. However, there is a lack of data indicating that regular diuretic use reduces the risk of developing BPD (160).
Inhaled nitric oxide
Inhaled nitric oxide (iNO) is a potent pulmonary vasodilator and an effective treatment for persistent pulmonary hypertension in near-term and full-term newborns (161). Despite these benefits, iNO does not prevent BPD when used as an early routine strategy or as a rescue therapy in very preterm infants (162, 163). A Cochrane review examining the efficacy of iNO in preterm infants included seventeen RCTs (163). The review found no significant difference in the overall incidence of death and/or BPD at 36 weeks’ PMA between preterm infants who received routine iNO and those in the control group (163–167). Furthermore, the recently published NEWNO trial, a large RCT involving 451 preterm infants less than 30 weeks’ GA and less than 1,250 grams birth weight, receiving mechanical ventilation between postnatal days 5 and 14, showed no significant differences in BPD at 36 weeks’ PMA or in neurodevelopmental and respiratory outcomes at 18–24 months between the treatment and placebo groups (168). Data from studies published to date are not sufficient to recommend the routine use of iNO for the prevention of BPD.
Bronchodilators
Data on the use of bronchodilators are limited. In a systematic review, only one randomized trial had usable outcome data. In this trial of 173 preterm infants (gestational age less than 31 weeks), salbutamol did not reduce the risk of BPD at 28 days when compared to no intervention/placebo (RR 1.03, 95% CI 0.78–1.37) (169).
Experimental drugs
Stem cell therapy
Mesenchymal stromal cell (MSC) therapy is a relatively new treatment that may prove to be an essential weapon in the prevention of BPD. Multiple preclinical studies have demonstrated the promising reparative potential of stem/progenitor cells in promoting lung growth and preventing lung injury (170). In animal models, various types of progenitor cells have shown protective effects against neonatal lung injuries induced by factors such as lipopolysaccharide and hyperoxia (171). Importantly, these cell-based therapies primarily exert their effects through paracrine mechanisms rather than direct integration into the repaired tissue (172). Stem cells can modulate innate and adaptive immune responses, reduce inflammation, enhance injury repair, and exert anti-apoptotic effects by secreting paracrine factors (173).
A phase I dose-escalation study conducted in 2014 investigated the safety and feasibility of a single intratracheal transplantation of allogeneic human umbilical cord blood (hUCB)-derived mesenchymal stem cells in extremely low birth weight infants (ELBWI) at high risk for BPD (174). The study reported a decrease in the severity of BPD in the transplanted group compared to the control group, with no adverse outcomes observed. While this study suggested that hUCB-MSC therapy is safe and feasible in preterm infants, further research is necessary to fully elucidate the safety profile and efficacy of stem cell therapy or their conditioned medium before they can be utilized in clinical settings.
Erythropoietin (EPO)
Recombinant human erythropoietin (rhEPO) treatment has shown promise in neonatal rats exposed to hyperoxia, where it upregulated epidermal growth factor-line domain 7 and was associated with decreased alveolar simplification, improved angiogenesis, and decreased fibrosis (175, 176). However, in preterm lambs, EPO administration increased alveolar inflammation and exacerbated ventilator-associated lung injury (177). In a multi-center prospective cohort study involving 867 neonates born before 28 weeks of GA, those with higher blood EPO concentrations on day 14 of life had a higher incidence of moderate but not severe BPD (178).
While retrospective studies have suggested a potential benefit of erythropoietin in reducing BPD in preterm infants, a meta-analysis of 17 RCTs found no difference in the incidence of BPD between infants receiving EPO and those receiving placebo (179–181). The PENUT trial (Preterm Erythropoietin Neuroprotection trial) also showed no reduction in the incidence of BPD after high-dose EPO supplementation (182). Importantly, EPO use did not increase the incidence of retinopathy of prematurity (ROP), sepsis, or NEC (181, 182). Combining EPO with mesenchymal stem cells has shown potential in attenuating lung injury by promoting angiogenesis and decreasing fibrosis in murine hyperoxia-induced lung injury models (183, 184). However, this combination therapy has not yet been studied in humans.
Recombinant human Clara cell 10 protein (rhCC10)
Clara Cell 10 Protein (CC10) is a 10-kilodalton protein secreted by non-ciliated bronchiolar epithelial cells (club cells) and is one of the most abundant proteins within the fluid lining the lung epithelium (185). CC10 has extensive anti-inflammatory properties and has been shown to be significantly lower in tracheal aspirates of premature infants who subsequently died or developed BPD (186, 187). Animal studies have demonstrated that the administration of recombinant human CC10 (rhCC10) upregulates SP and vascular endothelial growth factor (VEGF) expression while improving respiratory mechanics (188, 189). A pilot trial conducted in 22 VLBW infants demonstrated that intratracheal administration of rhCC10 was well-tolerated and had significant anti-inflammatory effects in the lung (190). In another multicenter RCT consisting of 88 infants 24 0/7 to 29 0/7 weeks’ GA, a single dose of intratracheal rhCC10 (1.5 mg/kg or 5 mg/kg) was found to be ineffective in changing long-term respiratory outcomes at 12 months of age (191).
Superoxyde dismutase (SOD)
Superoxide dismutases (SODs) are antioxidant enzymes that are reduced in various animal models of BPD (192, 193). Studies have shown that increased expression of extracellular SOD in transgenic mice preserved pulmonary angiogenesis following exposure to hyperoxia (194). Initial findings by Rosenfeld et al. suggested a decrease in both radiologic and clinical features of BPD after the subcutaneous administration of multiple doses of bovine SOD to infants with severe RDS (195). However, a randomized controlled trial by Davis et al. did not find a difference in the rate of death or BPD at 28 days or 36 weeks of PMA in infants receiving recombinant human CuZn-SOD (rh-CuZn-SOD) compared to placebo. Notably, infants treated with rhCuZn-SOD experienced fewer episodes of wheezing, required asthma medications less frequently, and had lower hospitalization rates before one year of corrected age (196). A recent study found no correlation between extracellular SOD in serum and the risk of BPD in infants born at less than 32 weeks’ gestational age (197). While SODs may hold promise for improving the long-term pulmonary outcomes of infants with BPD, further research is needed to determine their efficacy, safety, and optimal dosing before they can be implemented in clinical practice.
Inositol
Inositol is a nutrient that promotes the production of phosphatidylcholine and phosphatidylinositol, and preterm infants with a premature decline in myoinositol have a more severe course of RDS (198). Multiple RCTs have been conducted to evaluate the efficacy of inositol supplementation in preventing RDS and BPD. While no acute adverse effects were reported with the use of inositol, a recent Cochrane review concluded that inositol supplementation did not decrease the rates of BPD, death, or the composite outcome of BPD or death in preterm infants. Based on these findings, the review recommended against further clinical trials in neonates regarding the use of inositol for this purpose (199, 200).
Docosahexaenoic acid (DHA)
DHA is a long-chain polyunsaturated fatty acid known for its anti-inflammatory and antioxidant properties (201). In the DINO trial, which involved over 600 infants born before 33 weeks of GA, Manley et al. observed a reduced incidence of BPD in male infants and those weighing less than 1.25 kg whose mothers consumed tuna oil capsules containing a high amount of DHA. However, no significant difference was observed in female infants or those weighing more than 1.25 kg (202). However, the N3RO trial, which included over a thousand infants born before 29 weeks’ GA, reported a higher rate of BPD at 36 weeks of PMA and of BPD or death before 36 weeks’ PMA in infants receiving enteral DHA supplementation at a dose of 60 mg/kg/day (203). Similarly, the MOBYDIck trial, which involved mothers delivering infants at less than 29 weeks’ GA, found no significant improvement in BPD-free survival rates in infants whose mothers received DHA capsules (204). Due to insufficient data and conflicting results, DHA should only be used in research settings. Larger clinical studies are necessary to determine the appropriate dose and assess the efficacy of DHA in preventing BPD in extremely preterm infants.
Other potential treatments
Various potential targets including growth factors, anti-inflammatory agents, and antioxidants are currently being evaluated for the prevention of BPD in extremely premature infants. In a phase II RCT that included infants with a gestational age of 23 weeks and 0 days to 27 weeks and 6 days, Ley et al. demonstrated a significant decrease in the incidence of severe BPD following treatment with recombinant human insulin-like growth factor 1 (rhIGF-1) complexed with recombinant human insulin-like growth factor-binding protein 3 (rhIGFBP3) (205). Additionally, other factors showing promise in the pre-clinical phase include growth factors such as hypoxia-inducible factor 1-alpha (HIF-1α) and vascular endothelial growth factor (VEGF), anti-inflammatory agents like interleukin-1 receptor antagonist (ILR1A), and various long non-coding and micro RNAs (lncRNA and miRNA) (206–210).
Conclusions
BPD requires comprehensive strategies for prevention throughout the neonatal period. Initial respiratory management of very preterm infants should prioritize noninvasive support like nasal CPAP, reserving endotracheal intubation and surfactant administration for those who fail noninvasive support or do not demonstrate spontaneous respiratory effort after resuscitation. Utilizing a volume-targeted ventilation approach for those requiring mechanical ventilation may reduce the risk of BPD. Caffeine and vitamin A are supported by strong evidence for BPD prevention. Dexamethasone may be considered in high-risk cases initiated after the first week, although its risks should be carefully weighed. Hydrocortisone is an alternative with benefits but potential adverse effects. Newer experimental therapies appear to be promising for BPD prevention in extremely preterm infants; however, further research is necessary before their safety and efficacy in clinical practice can be established.
Author contributions
GD: Conceptualization, Data curation, Formal Analysis, Investigation, Writing – original draft, Methodology, Validation. SC: Conceptualization, Supervision, Writing – review & editing. FC: Conceptualization, Resources, Supervision, Writing – review & editing.
Funding
The author(s) declare that no financial support was received for the research, authorship, and/or publication of this article.
Conflict of interest
The authors declare that the research was conducted in the absence of any commercial or financial relationships that could be construed as a potential conflict of interest.
Publisher's note
All claims expressed in this article are solely those of the authors and do not necessarily represent those of their affiliated organizations, or those of the publisher, the editors and the reviewers. Any product that may be evaluated in this article, or claim that may be made by its manufacturer, is not guaranteed or endorsed by the publisher.
References
1. Bhandari A, Bhandari V. Pitfalls, problems, and progress in bronchopulmonary dysplasia. Pediatrics. (2009) 123(6):1562–73. doi: 10.1542/peds.2008-1962
2. Northway WH Jr, Rosan RC, Porter DY. Pulmonary disease following respirator therapy of hyaline-membrane disease. Bronchopulmonary dysplasia. N Engl J Med. (1967) 276(7):357–68. doi: 10.1056/NEJM196702162760701
3. Bancalari E, Claure N, Sosenko IR. Bronchopulmonary dysplasia: changes in pathogenesis, epidemiology and definition. Semin Neonatol. (2003) 8(1):63–71. doi: 10.1016/s1084-2756(02)00192-6
4. Stoll BJ, Hansen NI, Bell EF, Walsh MC, Carlo WA, Shankaran S, et al. Trends in care practices, morbidity, and mortality of extremely preterm neonates, 1993–2012. JAMA. (2015) 314(10):1039–51. doi: 10.1001/jama.2015.10244
5. Taghizadeh A, Reynolds EO. Pathogenesis of bronchopulmonary dysplasia following hyaline membrane disease. Am J Pathol. (1976) 82(2):241–64.175660
6. McEvoy CT, Aschner JL. The natural history of bronchopulmonary dysplasia: the case for primary prevention. Clin Perinatol. (2015) 42(4):911–31. doi: 10.1016/j.clp.2015.08.014
7. Jobe AH, Bancalari E. Bronchopulmonary dysplasia. Am J Respir Crit Care Med. (2001) 163(7):1723–9. doi: 10.1164/ajrccm.163.7.2011060
8. Ehrenkranz RA, Walsh MC, Vohr BR, Jobe AH, Wright LL, Fanaroff AA, et al. Validation of the national institutes of health consensus definition of bronchopulmonary dysplasia. Pediatrics. (2005) 116(6):1353–60. doi: 10.1542/peds.2005-0249
9. Higgins RD, Jobe AH, Koso-Thomas M, Bancalari E, Viscardi RM, Hartert TV, et al. Bronchopulmonary dysplasia: executive summary of a workshop. J Pediatr. (2018) 197:300–8. doi: 10.1016/j.jpeds.2018.01.043
10. Jensen EA, Dysart K, Gantz MG, McDonald S, Bamat NA, Keszler M, et al. The diagnosis of bronchopulmonary dysplasia in very preterm infants. An evidence-based approach. Am J Respir Crit Care Med. (2019) 200(6):751–9. doi: 10.1164/rccm.201812-2348OC
11. Stoecklin B, Simpson SJ, Pillow JJ. Bronchopulmonary dysplasia: rationale for a pathophysiological rather than treatment based approach to diagnosis. Paediatr Respir Rev. (2019) 32:91–7. doi: 10.1016/j.prrv.2018.12.002
12. Stewart A, Brion LP, Ambrosio-Perez I. Diuretics acting on the distal renal tubule for preterm infants with (or developing) chronic lung disease. Cochrane Database Syst Rev. (2011) 2011(9):CD001817. doi: 10.1002/14651858.CD001817.pub2
13. Khalaf MN, Hurley JF, Bhandari V. A prospective controlled trial of albuterol aerosol delivered via metered dose inhaler-spacer device (MDI) versus jet nebulizer in ventilated preterm neonates. Am J Perinatol. (2001) 18(3):169–74. doi: 10.1055/s-2001-14526
14. Slaughter JL, Stenger MR, Reagan PB. Variation in the use of diuretic therapy for infants with bronchopulmonary dysplasia. Pediatrics. (2013) 131(4):716–23. doi: 10.1542/peds.2012-1835
15. Zeitlin J, Szamotulska K, Drewniak N, Mohangoo AD, Chalmers J, Sakkeus L, et al. Preterm birth time trends in Europe: a study of 19 countries. BJOG. (2013) 120(11):1356–65. doi: 10.1111/1471-0528.12281
16. Ferré C, Callaghan W, Olson C, Sharma A, Barfield W. Effects of maternal age and age-specific preterm birth rates on overall preterm birth rates—United States, 2007 and 2014. MMWR Morb Mortal Wkly Rep. (2016) 65(43):1181–4. doi: 10.15585/mmwr.mm6543a1
17. Stoll BJ, Hansen NI, Bell EF, Shankaran S, Laptook AR, Walsh MC, et al. Neonatal outcomes of extremely preterm infants from the NICHD neonatal research network. Pediatrics. (2010) 126(3):443–56. doi: 10.1542/peds.2009-2959
18. Wadhawan R, Vohr BR, Fanaroff AA, Perritt RL, Duara S, Stoll BJ, et al. Does labor influence neonatal and neurodevelopmental outcomes of extremely-low-birth-weight infants who are born by cesarean delivery? Am J Obstet Gynecol. (2003) 189(2):501–6. doi: 10.1067/s0002-9378(03)00360-0
19. Adams M, Bassler D, Bucher HU, Roth-Kleiner M, Berger TM, Braun J, et al. Variability of very low birth weight infant outcome and practice in Swiss and US neonatal units. Pediatrics. (2018) 141(5):e20173436. doi: 10.1542/peds.2017-3436
20. Bhunwal S, Mukhopadhyay K, Bhattacharya S, Dey P, Dhaliwal LK. Bronchopulmonary dysplasia in preterm neonates in a level III neonatal unit in India. Indian Pediatr. (2018) 55(3):211–5. doi: 10.1007/s13312-018-1319-z
21. Bose C, Van Marter LJ, Laughon M, O’Shea TM, Allred EN, Karna P, et al. Fetal growth restriction and chronic lung disease among infants born before the 28th week of gestation. Pediatrics. (2009) 124(3):e450–8. doi: 10.1542/peds.2008-3249
22. Isayama T, Lee SK, Yang J, Lee D, Daspal S, Dunn M, et al. Revisiting the definition of bronchopulmonary dysplasia: effect of changing panoply of respiratory support for preterm neonates. JAMA Pediatr. (2017) 171(3):271–9. doi: 10.1001/jamapediatrics.2016.4141
23. Lee JH, Noh OK, Chang YS, Korean Neonatal Network. Neonatal outcomes of very low birth weight infants in Korean neonatal network from 2013 to 2016. J Korean Med Sci. (2019) 34(5):e40. doi: 10.3346/jkms.2019.34.e40
24. Lin HJ, Du LZ, Ma XL, Shi LP, Pan JH, Tong XM, et al. Mortality and morbidity of extremely low birth weight infants in the mainland of China: a multi-center study. Chin Med J (Engl). (2015) 128(20):2743–50. doi: 10.4103/0366-6999.167312
25. Su BH, Hsieh WS, Hsu CH, Chang JH, Lien R, Lin CH, et al. Neonatal outcomes of extremely preterm infants from Taiwan: comparison with Canada, Japan, and the USA. Pediatr Neonatol. (2015) 56(1):46–52. doi: 10.1016/j.pedneo.2014.05.002
26. Ambalavanan N, Van Meurs KP, Perritt R, Carlo WA, Ehrenkranz RA, Stevenson DK, et al. Predictors of death or bronchopulmonary dysplasia in preterm infants with respiratory failure. J Perinatol. (2008) 28(6):420–6. doi: 10.1038/jp.2008.18
27. Lemons JA, Bauer CR, Oh W, Korones SB, Papile LA, Stoll BJ, et al. Very low birth weight outcomes of the national institute of child health and human development neonatal research network, January 1995 through December 1996. NICHD neonatal research network. Pediatrics. (2001) 107(1):E1. doi: 10.1542/peds.107.1.e1
28. Marshall DD, Kotelchuck M, Young TE, Bose CL, Kruyer L, O’Shea TM. Risk factors for chronic lung disease in the surfactant era: a North Carolina population-based study of very low birth weight infants. North Carolina neonatologists association. Pediatrics. (1999) 104(6):1345–50. doi: 10.1542/peds.104.6.1345
29. Rojas MA, Gonzalez A, Bancalari E, Claure N, Poole C, Silva-Neto G. Changing trends in the epidemiology and pathogenesis of neonatal chronic lung disease. J Pediatr. (1995) 126(4):605–10. doi: 10.1016/s0022-3476(95)70362-4
30. Younge N, Goldstein RF, Bann CM, Hintz SR, Patel RM, Smith PB, et al. Survival and neurodevelopmental outcomes among periviable infants. N Engl J Med. (2017) 376(7):617–28. doi: 10.1056/NEJMoa1605566
31. Walsh MC, Yao Q, Gettner P, Hale E, Collins M, Hensman A, et al. Impact of a physiologic definition on bronchopulmonary dysplasia rates. Pediatrics. (2004) 114(5):1305–11. doi: 10.1542/peds.2004-0204
32. Hartling L, Liang Y, Lacaze-Masmonteil T. Chorioamnionitis as a risk factor for bronchopulmonary dysplasia: a systematic review and meta-analysis. Arch Dis Child Fetal Neonatal Ed. (2012) 97(1):F8–F17. doi: 10.1136/adc.2010.210187
33. McEvoy CT, Spindel ER. Pulmonary effects of maternal smoking on the fetus and child: effects on lung development, respiratory morbidities, and life long lung health. Paediatr Respir Rev. (2017) 21:27–33. doi: 10.1016/j.prrv.2016.08.005
34. Morrow LA, Wagner BD, Ingram DA, Poindexter BB, Schibler K, Cotten CM, et al. Antenatal determinants of bronchopulmonary dysplasia and late respiratory disease in preterm infants. Am J Respir Crit Care Med. (2017) 196(3):364–74. doi: 10.1164/rccm.201612-2414OC
35. Lavoie PM, Pham C, Jang KL. Heritability of bronchopulmonary dysplasia, defined according to the consensus statement of the national institutes of health. Pediatrics. (2008) 122(3):479–85. doi: 10.1542/peds.2007-2313
36. Parker RA, Lindstrom DP, Cotton RB. Evidence from twin study implies possible genetic susceptibility to bronchopulmonary dysplasia. Semin Perinatol. (1996) 20(3):206–9. doi: 10.1016/s0146-0005(96)80049-8
37. Parad RB, Winston AB, Kalish LA, Gupta M, Thompson I, Sheldon Y, et al. Role of genetic susceptibility in the development of bronchopulmonary dysplasia. J Pediatr. (2018) 203:234–241.e2. doi: 10.1016/j.jpeds.2018.07.099
38. Yu KH, Li J, Snyder M, Shaw GM, O'Brodovich HM. The genetic predisposition to bronchopulmonary dysplasia. Curr Opin Pediatr. (2016) 28(3):318–23. doi: 10.1097/MOP.0000000000000344
39. Bamat NA, Zhang H, McKenna KJ, Morris H, Stoller JZ, Gibbs K. The clinical evaluation of severe bronchopulmonary dysplasia. Neoreviews. (2020) 21(7):e442–53. doi: 10.1542/neo.21-7-e442
40. Vanhaverbeke K, Van Eyck A, Van Hoorenbeeck K, De Winter B, Snoeckx A, Mulder T, et al. Lung imaging in bronchopulmonary dysplasia: a systematic review. Respir Med. (2020) 171:106101. doi: 10.1016/j.rmed.2020.106101
41. Griscom NT, Wheeler WB, Sweezey NB, Kim YC, Lindsey JC, Wohl ME. Bronchopulmonary dysplasia: radiographic appearance in middle childhood. Radiology. (1989) 171(3):811–4. doi: 10.1148/radiology.171.3.2717757
42. Ochiai M, Hikino S, Yabuuchi H, Nakayama H, Sato K, Ohga S, et al. A new scoring system for computed tomography of the chest for assessing the clinical status of bronchopulmonary dysplasia. J Pediatr. (2008) 152(1):90–5. 95.e1–3. doi: 10.1016/j.jpeds.2007.05.043
43. Shin SM, Kim WS, Cheon JE, Kim HS, Lee W, Jung AY, et al. Bronchopulmonary dysplasia: new high resolution computed tomography scoring system and correlation between the high resolution computed tomography score and clinical severity. Korean J Radiol. (2013) 14(2):350–60. doi: 10.3348/kjr.2013.14.2.350
44. Duijts L, van Meel ER, Moschino L, Baraldi E, Barnhoorn M, Bramer WM, et al. European respiratory society guideline on long-term management of children with bronchopulmonary dysplasia. Eur Respir J. (2020) 55(1):1900788. doi: 10.1183/13993003.00788-2019
45. Alonso-Ojembarrena A, Lubián-López SP. Lung ultrasound score as early predictor of bronchopulmonary dysplasia in very low birth weight infants. Pediatr Pulmonol. (2019) 54(9):1404–9. doi: 10.1002/ppul.24410
46. Abman SH, Collaco JM, Shepherd EG, Keszler M, Cuevas-Guaman M, Welty SE, et al. Interdisciplinary care of children with severe bronchopulmonary dysplasia. J Pediatr. (2017) 181:12–28.e1. doi: 10.1016/j.jpeds.2016.10.082
47. Allen J, Zwerdling R, Ehrenkranz R, Gaultier C, Geggel R, Greenough A, et al. Statement on the care of the child with chronic lung disease of infancy and childhood. Am J Respir Crit Care Med. (2003) 168(3):356–96. doi: 10.1164/rccm.168.3.356
48. Krishnan U, Feinstein JA, Adatia I, Austin ED, Mullen MP, Hopper RK, et al. Evaluation and management of pulmonary hypertension in children with bronchopulmonary dysplasia. J Pediatr. (2017) 188:24–34.e1. doi: 10.1016/j.jpeds.2017.05.029
49. Heldt GP, McIlroy MB. Dynamics of chest wall in preterm infants. J Appl Physiol (1985). (1987) 62(1):170–4. doi: 10.1152/jappl.1987.62.1.170
50. Barker PM, Gowen CW, Lawson EE, Knowles MR. Decreased sodium ion absorption across nasal epithelium of very premature infants with respiratory distress syndrome. J Pediatr. (1997) 130(3):373–7. doi: 10.1016/s0022-3476(97)70198-7
51. Obladen M. Factors influencing surfactant composition in the newborn infant. Eur J Pediatr. (1978) 128(3):129–43. doi: 10.1007/BF00444298
52. Dreyfuss D, Saumon G. Ventilator-induced lung injury: lessons from experimental studies. Am J Respir Crit Care Med. (1998) 157(1):294–323. doi: 10.1164/ajrccm.157.1.9604014
53. Mokres LM, Parai K, Hilgendorff A, Ertsey R, Alvira CM, Rabinovitch M, et al. Prolonged mechanical ventilation with air induces apoptosis and causes failure of alveolar septation and angiogenesis in lungs of newborn mice. Am J Physiol Lung Cell Mol Physiol. (2010) 298(1):L23–35. doi: 10.1152/ajplung.00251.2009
54. Van Marter LJ, Allred EN, Pagano M, Sanocka U, Parad R, Moore M, et al. Do clinical markers of barotrauma and oxygen toxicity explain interhospital variation in rates of chronic lung disease? The neonatology committee for the developmental network. Pediatrics. (2000) 105(6):1194–201. doi: 10.1542/peds.105.6.1194
55. Schmölzer GM, Kumar M, Pichler G, Aziz K, O'Reilly M, Cheung PY. Non-invasive versus invasive respiratory support in preterm infants at birth: systematic review and meta-analysis. Br Med J. (2013) 347:f5980. doi: 10.1136/bmj.f5980
56. Lemyre B, Davis PG, De Paoli AG, Kirpalani H. Nasal intermittent positive pressure ventilation (NIPPV) versus nasal continuous positive airway pressure (NCPAP) for preterm neonates after extubation. Cochrane Database Syst Rev. (2017) 2(2):CD003212. doi: 10.1002/14651858.CD003212.pub3
57. Klingenberg C, Wheeler KI, McCallion N, Morley CJ, Davis PG. Volume-targeted versus pressure-limited ventilation in neonates. Cochrane Database Syst Rev. (2017) 10(10):CD003666. doi: 10.1002/14651858.CD003666.pub4
58. Cools F, Offringa M, Askie LM. Elective high frequency oscillatory ventilation versus conventional ventilation for acute pulmonary dysfunction in preterm infants. Cochrane Database Syst Rev. (2015) 2015(3):CD000104. doi: 10.1002/14651858.CD000104.pub4
59. Silveira RC, Panceri C, Munõz NP, Carvalho MB, Fraga AC, Procianoy RS. Less invasive surfactant administration versus intubation-surfactant-extubation in the treatment of neonatal respiratory distress syndrome: a systematic review and meta-analyses. J Pediatr (Rio J). (2024) 100(1):8–24. doi: 10.1016/j.jped.2023.05.008
60. Dunn MS, Kaempf J, de Klerk A, de Klerk R, Reilly M, Howard D, et al. Randomized trial comparing 3 approaches to the initial respiratory management of preterm neonates. Pediatrics. (2011) 128(5):e1069–76. doi: 10.1542/peds.2010-3848
61. Morley CJ, Davis PG, Doyle LW, Brion LP, Hascoet JM, Carlin JB. Nasal CPAP or intubation at birth for very preterm infants. N Engl J Med. (2008) 358(7):700–8. doi: 10.1056/NEJMoa072788
62. Finer NN, Carlo WA, Walsh MC, Rich W, Gantz MG, Laptook AR, et al. Early CPAP versus surfactant in extremely preterm infants. N Engl J Med. (2010) 362(21):1970–9. doi: 10.1056/NEJMoa0911783
63. Fischer HS, Bührer C. Avoiding endotracheal ventilation to prevent bronchopulmonary dysplasia: a meta-analysis. Pediatrics. (2013) 132(5):e1351–60. doi: 10.1542/peds.2013-1880
64. Subramaniam P, Ho JJ, Davis PG. Prophylactic nasal continuous positive airway pressure for preventing morbidity and mortality in very preterm infants. Cochrane Database Syst Rev. (2016) 6:CD001243. Update in: Cochrane Database Syst Rev. 2021;10:CD001243. doi: 10.1002/14651858.CD001243.pub4. doi: 10.1002/14651858.CD001243.pub3
65. Committee on Fetus and Newborn; American Academy of Pediatrics. Respiratory support in preterm infants at birth. Pediatrics. (2014) 133(1):171–4. doi: 10.1542/peds.2013-3442
66. Roberts CT, Hodgson KA. Nasal high flow treatment in preterm infants. Matern Health Neonatol Perinatol. (2017) 3:15. doi: 10.1186/s40748-017-0056-y
67. Wilkinson D, Andersen C, O'Donnell CP, De Paoli AG, Manley BJ. High flow nasal cannula for respiratory support in preterm infants. Cochrane Database Syst Rev. (2016) 2(2):CD006405. doi: 10.1002/14651858.CD006405.pub3
68. Courtney SE, Barrington KJ. Continuous positive airway pressure and noninvasive ventilation. Clin Perinatol. (2007) 34(1):73–92. vi. doi: 10.1016/j.clp.2006.12.008
69. Lemyre B, Laughon M, Bose C, Davis PG. Early nasal intermittent positive pressure ventilation (NIPPV) versus early nasal continuous positive airway pressure (NCPAP) for preterm infants. Cochrane Database Syst Rev. (2016) 12(12):CD005384. doi: 10.1002/14651858.CD005384.pub2
70. Attar MA, Donn SM. Mechanisms of ventilator-induced lung injury in premature infants. Semin Neonatol. (2002) 7(5):353–60. doi: 10.1053/siny.2002.0129
71. Clark RH, Slutsky AS, Gerstmann DR. Lung protective strategies of ventilation in the neonate: what are they? Pediatrics. (2000) 105(1 Pt 1):112–4. doi: 10.1542/peds.105.1.112
72. Clark RH, Gerstmann DR, Jobe AH, Moffitt ST, Slutsky AS, Yoder BA. Lung injury in neonates: causes, strategies for prevention, and long-term consequences. J Pediatr. (2001) 139(4):478–86. doi: 10.1067/mpd.2001.118201
73. Dreyfuss D, Soler P, Basset G, Saumon G. High inflation pressure pulmonary edema. Respective effects of high airway pressure, high tidal volume, and positive end-expiratory pressure. Am Rev Respir Dis. (1988) 137(5):1159–64. doi: 10.1164/ajrccm/137.5.1159
74. Nkadi PO, Merritt TA, Pillers DA. An overview of pulmonary surfactant in the neonate: genetics, metabolism, and the role of surfactant in health and disease. Mol Genet Metab. (2009) 97(2):95–101. doi: 10.1016/j.ymgme.2009.01.015
75. Reynolds EO, Roberton NR, Wigglesworth JS. Hyaline membrane disease, respiratory distress, and surfactant deficiency. Pediatrics. (1968) 42(5):758–68. doi: 10.1542/peds.42.5.758
76. Soll RF. Prophylactic natural surfactant extract for preventing morbidity and mortality in preterm infants. Cochrane Database Syst Rev. (2000) 1997(2):CD000511. doi: 10.1002/14651858.CD000511
77. Soll RF. Prophylactic synthetic surfactant for preventing morbidity and mortality in preterm infants. Cochrane Database Syst Rev. (2000) (2):CD001079. doi: 10.1002/14651858.CD001079
78. Soll R, Ozek E. Prophylactic protein free synthetic surfactant for preventing morbidity and mortality in preterm infants. Cochrane Database Syst Rev. (2010) 2010(1):CD001079. doi: 10.1002/14651858.CD001079.pub2
79. Victorin LH, Deverajan LV, Curstedt T, Robertson B. Surfactant replacement in spontaneously breathing babies with hyaline membrane disease–a pilot study. Biol Neonate. (1990) 58(3):121–6. doi: 10.1159/000243250
80. Stevens TP, Harrington EW, Blennow M, Soll RF. Early surfactant administration with brief ventilation vs. selective surfactant and continued mechanical ventilation for preterm infants with or at risk for respiratory distress syndrome. Cochrane Database Syst Rev. (2007) 2007(4):CD003063. doi: 10.1002/14651858.CD003063.pub3
81. Isayama T, Chai-Adisaksopha C, McDonald SD. Noninvasive ventilation with vs without early surfactant to prevent chronic lung disease in preterm infants: a systematic review and meta-analysis. JAMA Pediatr. (2015) 169(8):731–9. doi: 10.1001/jamapediatrics.2015.0510
82. More K, Sakhuja P, Shah PS. Minimally invasive surfactant administration in preterm infants: a meta-narrative review. JAMA Pediatr. (2014) 168(10):901–8. doi: 10.1001/jamapediatrics.2014.1148
83. Bao Y, Zhang G, Wu M, Ma L, Zhu J. A pilot study of less invasive surfactant administration in very preterm infants in a Chinese tertiary center. BMC Pediatr. (2015) 15:21. doi: 10.1186/s12887-015-0342-7
84. Kanmaz HG, Erdeve O, Canpolat FE, Mutlu B, Dilmen U. Surfactant administration via thin catheter during spontaneous breathing: randomized controlled trial. Pediatrics. (2013) 131(2):e502–9. doi: 10.1542/peds.2012-0603
85. Kribs A, Roll C, Göpel W, Wieg C, Groneck P, Laux R, et al. Nonintubated surfactant application vs conventional therapy in extremely preterm infants: a randomized clinical trial. JAMA Pediatr. (2015) 169(8):723–30. doi: 10.1001/jamapediatrics.2015.0504
86. Mirnia K, Heidarzadeh M, Hosseini MB, Sadeghnia A, Balila M, Ghojazadeh M. Comparison outcome of surfactant administration via tracheal catheterization during spontaneous breathing with INSURE. Med J Islamic World Acad Sci. (2013) 21(4):143–8. doi: 10.12816/0002647
87. Askie LM, Henderson-Smart DJ, Irwig L, Simpson JM. Oxygen-saturation targets and outcomes in extremely preterm infants. N Engl J Med. (2003) 349(10):959–67. doi: 10.1056/NEJMoa023080
88. Manja V, Lakshminrusimha S, Cook DJ. Oxygen saturation target range for extremely preterm infants: a systematic review and meta-analysis. JAMA Pediatr. (2015) 169(4):332–40. doi: 10.1001/jamapediatrics.2014.3307
89. Askie LM, Darlow BA, Davis PG, Finer N, Stenson B, Vento M, et al. Effects of targeting lower versus higher arterial oxygen saturations on death or disability in preterm infants. Cochrane Database Syst Rev. (2017) 4(4):CD011190. doi: 10.1002/14651858.CD011190.pub2
90. Nelin LD, Bhandari V. How to decrease bronchopulmonary dysplasia in your neonatal intensive care unit today and “tomorrow”. F1000Res. (2017) 6:539. doi: 10.12688/f1000research.10832.1
91. Doyle LW, Carse E, Adams AM, Ranganathan S, Opie G, Cheong JLY, et al. Ventilation in extremely preterm infants and respiratory function at 8 years. N Engl J Med. (2017) 377(4):329–37. doi: 10.1056/NEJMoa1700827
92. Sakaria RP, Dhanireddy R. Pharmacotherapy in bronchopulmonary dysplasia: what is the evidence? Front Pediatr. (2022) 10:820259. doi: 10.3389/fped.2022.820259
93. Nair V, Loganathan P, Soraisham AS. Azithromycin and other macrolides for prevention of bronchopulmonary dysplasia: a systematic review and meta-analysis. Neonatology. (2014) 106(4):337–47. doi: 10.1159/000363493
94. Schmidt B, Roberts RS, Davis P, Doyle LW, Barrington KJ, Ohlsson A, et al. Caffeine therapy for apnea of prematurity. N Engl J Med. (2006) 354(20):2112–21. doi: 10.1056/NEJMoa054065
95. Darlow BA, Graham PJ, Rojas-Reyes MX. Vitamin A supplementation to prevent mortality and short- and long-term morbidity in very low birth weight infants. Cochrane Database Syst Rev. (2016) 2016(8):CD000501. doi: 10.1002/14651858.CD000501.pub4
96. Doyle LW, Cheong JL, Hay S, Manley BJ, Halliday HL. Early (<7 days) systemic postnatal corticosteroids for prevention of bronchopulmonary dysplasia in preterm infants. Cochrane Database Syst Rev. (2021) 10(10):CD001146. doi: 10.1002/14651858.CD001146.pub6
97. Doyle LW, Cheong JL, Hay S, Manley BJ, Halliday HL. Late (≥7 days) systemic postnatal corticosteroids for prevention of bronchopulmonary dysplasia in preterm infants. Cochrane Database Syst Rev. (2021) 11(11):CD001145. doi: 10.1002/14651858.CD001145.pub5
98. Bassler D, Plavka R, Shinwell ES, Hallman M, Jarreau PH, Carnielli V, et al. Early inhaled budesonide for the prevention of bronchopulmonary dysplasia. N Engl J Med. (2015) 373(16):1497–506. doi: 10.1056/NEJMoa1501917
99. Venkataraman R, Kamaluddeen M, Hasan SU, Robertson HL, Lodha A. Intratracheal administration of budesonide-surfactant in prevention of bronchopulmonary dysplasia in very low birth weight infants: a systematic review and meta-analysis. Pediatr Pulmonol. (2017) 52(7):968–75. doi: 10.1002/ppul.23680
100. Jaffé A, Bush A. Anti-inflammatory effects of macrolides in lung disease. Pediatr Pulmonol. (2001) 31(6):464–73. doi: 10.1002/ppul.1076
101. Aghai ZH, Kode A, Saslow JG, Nakhla T, Farhath S, Stahl GE, et al. Azithromycin suppresses activation of nuclear factor-kappa B and synthesis of pro-inflammatory cytokines in tracheal aspirate cells from premature infants. Pediatr Res. (2007) 62(4):483–8. doi: 10.1203/PDR.0b013e318142582d
102. Wang EE, Ohlsson A, Kellner JD. Association of Ureaplasma urealyticum colonization with chronic lung disease of prematurity: results of a metaanalysis. J Pediatr. (1995) 127(4):640–4. doi: 10.1016/s0022-3476(95)70130-3
103. Schelonka RL, Katz B, Waites KB, Benjamin DK Jr. Critical appraisal of the role of Ureaplasma in the development of bronchopulmonary dysplasia with metaanalytic techniques. Pediatr Infect Dis J. (2005) 24(12):1033–9. doi: 10.1097/01.inf.0000190632.31565.83
104. Viscardi RM, Kallapur SG. Role of Ureaplasma respiratory tract colonization in bronchopulmonary dysplasia pathogenesis: current concepts and update. Clin Perinatol. (2015) 42(4):719–38. doi: 10.1016/j.clp.2015.08.003
105. Bose CL, Dammann CE, Laughon MM. Bronchopulmonary dysplasia and inflammatory biomarkers in the premature neonate. Arch Dis Child Fetal Neonatal Ed. (2008) 93(6):F455–61. doi: 10.1136/adc.2007.121327
106. Speer CP. Pulmonary inflammation and bronchopulmonary dysplasia. J Perinatol. (2006) 26(Suppl 1):S57–62. discussion S63–4. doi: 10.1038/sj.jp.7211476
107. Jensen EA, Foglia EE, Schmidt B. Evidence-based pharmacologic therapies for prevention of bronchopulmonary dysplasia: application of the grading of recommendations assessment, development, and evaluation methodology. Clin Perinatol. (2015) 42(4):755–79. doi: 10.1016/j.clp.2015.08.005
108. Ozdemir R, Erdeve O, Dizdar EA, Oguz SS, Uras N, Saygan S, et al. Clarithromycin in preventing bronchopulmonary dysplasia in Ureaplasma urealyticum-positive preterm infants. Pediatrics. (2011) 128(6):e1496–501. doi: 10.1542/peds.2011-1350
109. Mabanta CG, Pryhuber GS, Weinberg GA, Phelps DL. Erythromycin for the prevention of chronic lung disease in intubated preterm infants at risk for, or colonized or infected with Ureaplasma urealyticum. Cochrane Database Syst Rev. (2003) (4):CD003744. doi: 10.1002/14651858.CD003744
110. Ribeiro JA, Sebastião AM. Caffeine and adenosine. J Alzheimers Dis. (2010) 20(Suppl 1):S3–15. doi: 10.3233/JAD-2010-1379
111. Abu-Shaweesh JM, Martin RJ. Caffeine use in the neonatal intensive care unit. Semin Fetal Neonatal Med. (2017) 22(5):342–7. doi: 10.1016/j.siny.2017.07.011
112. Schmidt B, Roberts RS, Anderson PJ, Asztalos EV, Costantini L, Davis PG, et al. Academic performance, motor function, and behavior 11 years after neonatal caffeine citrate therapy for apnea of prematurity: an 11-year follow-up of the CAP randomized clinical trial. JAMA Pediatr. (2017) 171(6):564–72. doi: 10.1001/jamapediatrics.2017.0238
113. Patel RM, Leong T, Carlton DP, Vyas-Read S. Early caffeine therapy and clinical outcomes in extremely preterm infants. J Perinatol. (2013) 33(2):134–40. doi: 10.1038/jp.2012.52
114. Lodha A, Seshia M, McMillan DD, Barrington K, Yang J, Lee SK, et al. Association of early caffeine administration and neonatal outcomes in very preterm neonates. JAMA Pediatr. (2015) 169(1):33–8. doi: 10.1001/jamapediatrics.2014.2223
115. Taha D, Kirkby S, Nawab U, Dysart KC, Genen L, Greenspan JS, et al. Early caffeine therapy for prevention of bronchopulmonary dysplasia in preterm infants. J Matern Fetal Neonatal Med. (2014) 27(16):1698–702. doi: 10.3109/14767058.2014.885941
116. Kua KP, Lee SW. Systematic review and meta-analysis of clinical outcomes of early caffeine therapy in preterm neonates. Br J Clin Pharmacol. (2017) 83(1):180–91. doi: 10.1111/bcp.13089
117. Dobson NR, Patel RM, Smith PB, Kuehn DR, Clark J, Vyas-Read S, et al. Trends in caffeine use and association between clinical outcomes and timing of therapy in very low birth weight infants. J Pediatr. (2014) 164(5):992–998.e3. doi: 10.1016/j.jpeds.2013.12.025
118. Rodgers A, Singh C. Specialist neonatal respiratory care for babies born preterm (NICE guideline 124): a review. Arch Dis Child Educ Pract Ed. (2020) 105(6):355–7. doi: 10.1136/archdischild-2019-317461
119. Niederreither K, Dollé P. Retinoic acid in development: towards an integrated view. Nat Rev Genet. (2008) 9(7):541–53. doi: 10.1038/nrg2340
120. Biesalski HK, Nohr D. Importance of vitamin-A for lung function and development. Mol Aspects Med. (2003) 24(6):431–40. doi: 10.1016/s0098-2997(03)00039-6
121. Shenai JP, Chytil F, Stahlman MT. Vitamin A status of neonates with bronchopulmonary dysplasia. Pediatr Res. (1985) 19(2):185–8. doi: 10.1203/00006450-198502000-00007
122. Hustead VA, Gutcher GR, Anderson SA, Zachman RD. Relationship of vitamin A (retinol) status to lung disease in the preterm infant. J Pediatr. (1984) 105(4):610–5. doi: 10.1016/s0022-3476(84)80432-1
123. Chytil F. The lungs and vitamin A. Am J Physiol. (1992) 262(5 Pt 1):L517–27. doi: 10.1152/ajplung.1992.262.5.L517
124. Tyson JE, Wright LL, Oh W, Kennedy KA, Mele L, Ehrenkranz RA, et al. Vitamin A supplementation for extremely-low-birth-weight infants. National institute of child health and human development neonatal research network. N Engl J Med. (1999) 340(25):1962–8. doi: 10.1056/NEJM199906243402505
125. Gadhia MM, Cutter GR, Abman SH, Kinsella JP. Effects of early inhaled nitric oxide therapy and vitamin A supplementation on the risk for bronchopulmonary dysplasia in premature newborns with respiratory failure. J Pediatr. (2014) 164(4):744–8. doi: 10.1016/j.jpeds.2013.11.040
126. Meyer S, Gortner L, NeoVitaA Trial Investigators. Early postnatal additional high-dose oral vitamin A supplementation versus placebo for 28 days for preventing bronchopulmonary dysplasia or death in extremely low birth weight infants. Neonatology. (2014) 105(3):182–8. doi: 10.1159/000357212
127. Onland W, van Kaam AH, De Jaegere AP, Offringa M. Open-label glucocorticoids modulate dexamethasone trial results in preterm infants. Pediatrics. (2010) 126(4):e954–64. doi: 10.1542/peds.2010-0597
128. Watterberg KL, American Academy of Pediatrics, Committee on Fetus and Newborn. Policy statement: postnatal corticosteroids to prevent or treat bronchopulmonary dysplasia. Pediatrics. (2010) 126(4):800–8. doi: 10.1542/peds.2010-1534
129. Doyle LW, Davis PG, Morley CJ, McPhee A, Carlin JB, DART Study Investigators. Low-dose dexamethasone facilitates extubation among chronically ventilator-dependent infants: a multicenter, international, randomized, controlled trial. Pediatrics. (2006) 117(1):75–83. doi: 10.1542/peds.2004-2843
130. Doyle LW, Davis PG, Morley CJ, McPhee A, Carlin JB, DART Study Investigators. Outcome at 2 years of age of infants from the DART study: a multicenter, international, randomized, controlled trial of low-dose dexamethasone. Pediatrics. (2007) 119(4):716–21. doi: 10.1542/peds.2006-2806
131. Baud O, Maury L, Lebail F, Ramful D, El Moussawi F, Nicaise C, et al. Effect of early low-dose hydrocortisone on survival without bronchopulmonary dysplasia in extremely preterm infants (PREMILOC): a double-blind, placebo-controlled, multicentre, randomised trial. Lancet. (2016) 387(10030):1827–36. doi: 10.1016/S0140-6736(16)00202-6
132. Baud O, Trousson C, Biran V, Leroy E, Mohamed D, Alberti C, et al. Association between early low-dose hydrocortisone therapy in extremely preterm neonates and neurodevelopmental outcomes at 2 years of age. JAMA. (2017) 317(13):1329–37. doi: 10.1001/jama.2017.2692
133. Onland W, Cools F, Kroon A, Rademaker K, Merkus MP, Dijk PH, et al. Effect of hydrocortisone therapy initiated 7 to 14 days after birth on mortality or bronchopulmonary dysplasia among very preterm infants receiving mechanical ventilation: a randomized clinical trial. JAMA. (2019) 321(4):354–63. doi: 10.1001/jama.2018.21443
134. Shinwell ES, Portnov I, Meerpohl JJ, Karen T, Bassler D. Inhaled corticosteroids for bronchopulmonary dysplasia: a meta-analysis. Pediatrics. (2016) 138(6):e20162511. doi: 10.1542/peds.2016-2511
135. Onland W, Offringa M, van Kaam A. Late (≥ 7 days) inhalation corticosteroids to reduce bronchopulmonary dysplasia in preterm infants. Cochrane Database Syst Rev. (2017) 8(8):CD002311. doi: 10.1002/14651858.CD002311.pub4
136. Bassler D, Shinwell ES, Hallman M, Jarreau PH, Plavka R, Carnielli V, et al. Long-term effects of inhaled budesonide for bronchopulmonary dysplasia. N Engl J Med. (2018) 378(2):148–57. doi: 10.1056/NEJMoa1708831
137. Uberos J, Jimenez-Montilla S, Molina-Oya M, García-Serrano JL. Early energy restriction in premature infants and bronchopulmonary dysplasia: a cohort study. Br J Nutr. (2020) 123(9):1024–31. doi: 10.1017/S0007114520000240
138. Moschino L, Duci M, Fascetti Leon F, Bonadies L, Priante E, Baraldi E, et al. Optimizing nutritional strategies to prevent necrotizing enterocolitis and growth failure after bowel resection. Nutrients. (2021) 13(2):340. doi: 10.3390/nu13020340
139. Villamor-Martínez E, Pierro M, Cavallaro G, Mosca F, Kramer BW, Villamor E. Donor human milk protects against bronchopulmonary dysplasia: a systematic review and meta-analysis. Nutrients. (2018) 10(2):238. doi: 10.3390/nu10020238
140. Hair AB, Peluso AM, Hawthorne KM, Perez J, Smith DP, Khan JY, et al. Beyond necrotizing enterocolitis prevention: improving outcomes with an exclusive human milk-based diet. Breastfeed Med. (2016) 11(2):70–4. doi: 10.1089/bfm.2015.0134
141. Liggins GC, Howie RN. A controlled trial of antepartum glucocorticoid treatment for prevention of the respiratory distress syndrome in premature infants. Pediatrics. (1972) 50(4):515–25. doi: 10.1542/peds.50.4.515
142. Effect of corticosteroids for fetal maturation on perinatal outcomes. NIH consensus development panel on the effect of corticosteroids for fetal maturation on perinatal outcomes. JAMA. (1995) 273(5):413–8. doi: 10.1001/jama.1995.03520290065031
143. Venkatesh VC, Katzberg HD. Glucocorticoid regulation of epithelial sodium channel genes in human fetal lung. Am J Physiol. (1997) 273(1 Pt 1):L227–33. doi: 10.1152/ajplung.1997.273.1.L227
144. Ballard PL. Scientific rationale for the use of antenatal glucocorticoids to promote fetal development. Pediatr Rev. (2000) 1(5):E83–90. doi: 10.1542/neo.1-5-e83
145. Millage AR, Latuga MS, Aschner JL. Effect of perinatal glucocorticoids on vascular health and disease. Pediatr Res. (2017) 81(1-1):4–10. doi: 10.1038/pr.2016.188
146. Haviv HR, Said J, Mol BW. The place of antenatal corticosteroids in late preterm and early term births. Semin Fetal Neonatal Med. (2019) 24(1):37–42. doi: 10.1016/j.siny.2018.10.001
147. Committee on Obstetric Practice. Committee opinion no. 713: antenatal corticosteroid therapy for fetal maturation. Obstet Gynecol. (2017) 130(2):e102–9. doi: 10.1097/AOG.0000000000002237
148. McGoldrick E, Stewart F, Parker R, Dalziel SR. Antenatal corticosteroids for accelerating fetal lung maturation for women at risk of preterm birth. Cochrane Database Syst Rev. (2020) 12(12):CD004454. doi: 10.1002/14651858.CD004454.pub4
149. Palta M, Gabbert D, Weinstein MR, Peters ME. Multivariate assessment of traditional risk factors for chronic lung disease in very low birth weight neonates. The newborn lung project. J Pediatr. (1991) 119(2):285–92. doi: 10.1016/s0022-3476(05)80746-2
150. Oh W, Poindexter BB, Perritt R, Lemons JA, Bauer CR, Ehrenkranz RA, et al. Association between fluid intake and weight loss during the first ten days of life and risk of bronchopulmonary dysplasia in extremely low birth weight infants. J Pediatr. (2005) 147(6):786–90. doi: 10.1016/j.jpeds.2005.06.039
151. Benitz WE. Patent ductus arteriosus: to treat or not to treat? Arch Dis Child Fetal Neonatal Ed. (2012) 97(2):F80–2. doi: 10.1136/archdischild-2011-300381
152. Fowlie PW, Davis PG, McGuire W. Prophylactic intravenous indomethacin for preventing mortality and morbidity in preterm infants. Cochrane Database Syst Rev. (2010) 2010(7):CD000174. doi: 10.1002/14651858.CD000174.pub2
153. Ohlsson A, Walia R, Shah SS. Ibuprofen for the treatment of patent ductus arteriosus in preterm or low birth weight (or both) infants. Cochrane Database Syst Rev. (2018) 9(9):CD003481. doi: 10.1002/14651858.CD003481.pub7
154. Ohlsson A, Shah PS. Paracetamol (Acetaminophen) for patent ductus arteriosus in preterm or low birth weight infants. Cochrane Database Syst Rev. (2020) 1(1):CD010061. doi: 10.1002/14651858.CD010061.pub4
155. Benitz WE; Committee on Fetus and Newborn, American Academy of Pediatrics. Patent ductus arteriosus in preterm infants. Pediatrics. (2016) 137(1):e20153730. doi: 10.1542/peds.2015-3730
156. Clyman R, Cassady G, Kirklin JK, Collins M, Philips JB III. The role of patent ductus arteriosus ligation in bronchopulmonary dysplasia: reexamining a randomized controlled trial. J Pediatr. (2009) 154(6):873–6. doi: 10.1016/j.jpeds.2009.01.005
157. Kabra NS, Schmidt B, Roberts RS, Doyle LW, Papile L, Fanaroff A, et al. Neurosensory impairment after surgical closure of patent ductus arteriosus in extremely low birth weight infants: results from the trial of indomethacin prophylaxis in preterms. J Pediatr. (2007) 150(3):229–34. 234.e1. doi: 10.1016/j.jpeds.2006.11.039
158. Sankar MN, Bhombal S, Benitz WE. PDA: to treat or not to treat. Congenit Heart Dis. (2019) 14(1):46–51. doi: 10.1111/chd.12708
159. Kavvadia V, Greenough A, Dimitriou G, Hooper R. Randomised trial of fluid restriction in ventilated very low birthweight infants. Arch Dis Child Fetal Neonatal Ed. (2000) 83(2):F91–6. doi: 10.1136/fn.83.2.F91
160. Iyengar A, Davis JM. Drug therapy for the prevention and treatment of bronchopulmonary dysplasia. Front Pharmacol. (2015) 6:12. doi: 10.3389/fphar.2015.00012
161. Barrington KJ, Finer N, Pennaforte T, Altit G. Nitric oxide for respiratory failure in infants born at or near term. Cochrane Database Syst Rev. (2017) 1(1):CD000399. doi: 10.1002/14651858.CD000399.pub3
162. Askie LM, Ballard RA, Cutter GR, Dani C, Elbourne D, Field D, et al. Inhaled nitric oxide in preterm infants: an individual-patient data meta-analysis of randomized trials. Pediatrics. (2011) 128(4):729–39. doi: 10.1542/peds.2010-2725
163. Barrington KJ, Finer N, Pennaforte T. Inhaled nitric oxide for respiratory failure in preterm infants. Cochrane Database Syst Rev. (2017) 1(1):CD000509. doi: 10.1002/14651858.CD000509.pub5
164. Schreiber MD, Gin-Mestan K, Marks JD, Huo D, Lee G, Srisuparp P. Inhaled nitric oxide in premature infants with the respiratory distress syndrome. N Engl J Med. (2003) 349(22):2099–107. doi: 10.1056/NEJMoa031154
165. Kinsella JP, Cutter GR, Walsh WF, Gerstmann DR, Bose CL, Hart C, et al. Early inhaled nitric oxide therapy in premature newborns with respiratory failure. N Engl J Med. (2006) 355(4):354–64. doi: 10.1056/NEJMoa060442
166. Mercier JC, Hummler H, Durrmeyer X, Sanchez-Luna M, Carnielli V, Field D, et al. Inhaled nitric oxide for prevention of bronchopulmonary dysplasia in premature babies (EUNO): a randomised controlled trial. Lancet. (2010) 376(9738):346–54. doi: 10.1016/S0140-6736(10)60664-2
167. Kinsella JP, Cutter GR, Steinhorn RH, Nelin LD, Walsh WF, Finer NN, et al. Noninvasive inhaled nitric oxide does not prevent bronchopulmonary dysplasia in premature newborns. J Pediatr. (2014) 165(6):1104–1108.e1. doi: 10.1016/j.jpeds.2014.06.018
168. Hasan SU, Potenziano J, Konduri GG, Perez JA, Van Meurs KP, Walker MW, et al. Effect of inhaled nitric oxide on survival without bronchopulmonary dysplasia in preterm infants: a randomized clinical trial. JAMA Pediatr. (2017) 171(11):1081–9. doi: 10.1001/jamapediatrics.2017.2618
169. Ng G, da Silva O, Ohlsson A. Bronchodilators for the prevention and treatment of chronic lung disease in preterm infants. Cochrane Database Syst Rev. (2016) 12(12):CD003214. Update in: Cochrane Database Syst Rev. 2024 April 9;4:CD003214. doi: 10.1002/14651858.CD003214.pub4. doi: 10.1002/14651858.CD003214.pub3
170. Pierro M, Thébaud B, Soll R. Mesenchymal stem cells for the prevention and treatment of bronchopulmonary dysplasia in preterm infants. Cochrane Database Syst Rev. (2017) 11(11):CD011932. doi: 10.1002/14651858.CD011932.pub2
171. O'Reilly M, Thébaud B. Using cell-based strategies to break the link between bronchopulmonary dysplasia and the development of chronic lung disease in later life. Pulm Med. (2013) 2013:874161. doi: 10.1155/2013/874161
172. Fung ME, Thébaud B. Stem cell-based therapy for neonatal lung disease: it is in the juice. Pediatr Res. (2014) 75(1-1):2–7. doi: 10.1038/pr.2013.176
173. Lee JW, Fang X, Krasnodembskaya A, Howard JP, Matthay MA. Concise review: mesenchymal stem cells for acute lung injury: role of paracrine soluble factors. Stem Cells. (2011) 29(6):913–9. doi: 10.1002/stem.643
174. Chang YS, Ahn SY, Yoo HS, Sung SI, Choi SJ, Oh WI, et al. Mesenchymal stem cells for bronchopulmonary dysplasia: phase 1 dose-escalation clinical trial. J Pediatr. (2014) 164(5):966–972.e6. doi: 10.1016/j.jpeds.2013.12.011
175. Ozer EA, Kumral A, Ozer E, Yilmaz O, Duman N, Ozkal S, et al. Effects of erythropoietin on hyperoxic lung injury in neonatal rats. Pediatr Res. (2005) 58(1):38–41. doi: 10.1203/01.PDR.0000163391.75389.52
176. Cui H, He J, Chen H, Chen J, Qian X, Huang W. Erythropoietin attenuates hyperoxia-induced lung injury by upregulating epidermal growth factor-like domain 7 in newborn rats. Biomed Rep. (2017) 6(1):32–8. doi: 10.3892/br.2016.820
177. Polglase GR, Barton SK, Melville JM, Zahra V, Wallace MJ, Siew ML, et al. Prophylactic erythropoietin exacerbates ventilation-induced lung inflammation and injury in preterm lambs. J Physiol. (2014) 592(9):1993–2002. doi: 10.1113/jphysiol.2013.270348
178. Holm M, Skranes J, Dammann O, Fichorova RN, Allred EN, Leviton A. Systemic endogenous erythropoietin and associated disorders in extremely preterm newborns. Arch Dis Child Fetal Neonatal Ed. (2016) 101(5):F458–63. doi: 10.1136/archdischild-2015-309127
179. Bui KCT, Kim R, Abbasi A, Nguyen M, Villosis MF, Chen Q. Erythropoietin treatment is associated with a reduction in moderate to severe bronchopulmonary dysplasia in preterm infants. A regional retrospective study. Early Hum Dev. (2019) 137:104831. doi: 10.1016/j.earlhumdev.2019.104831
180. Rayjada N, Barton L, Chan LS, Plasencia S, Biniwale M, Bui KC. Decrease in incidence of bronchopulmonary dysplasia with erythropoietin administration in preterm infants: a retrospective study. Neonatology. (2012) 102(4):287–92. doi: 10.1159/000341615
181. Ananthan A, Balasubramanian H, Rao S, Patole S. Clinical outcomes related to the gastrointestinal trophic effects of erythropoietin in preterm neonates: a systematic review and meta-analysis. Adv Nutr. (2018) 9(3):238–46. doi: 10.1093/advances/nmy005
182. Juul SE, Comstock BA, Wadhawan R, Mayock DE, Courtney SE, Robinson T, et al. A randomized trial of erythropoietin for neuroprotection in preterm infants. N Engl J Med. (2020) 382(3):233–43. doi: 10.1056/NEJMoa1907423
183. Sun C, Zhang S, Wang J, Jiang W, Xin Q, Chen X, et al. EPO Enhances the protective effects of MSCs in experimental hyperoxia-induced neonatal mice by promoting angiogenesis. Aging (Albany NY). (2019) 11(8):2477–87. doi: 10.18632/aging.101937
184. Luan Y, Zhang L, Chao S, Liu X, Li K, Wang Y, et al. Mesenchymal stem cells in combination with erythropoietin repair hyperoxia-induced alveoli dysplasia injury in neonatal mice via inhibition of TGF-β1 signaling. Oncotarget. (2016) 7(30):47082–94. doi: 10.18632/oncotarget.9314
185. Greenough A. Clara cell secretory protein and bronchopulmonary dysplasia in prematurely born infants. Eur J Pediatr. (2008) 167(11):1347–8. doi: 10.1007/s00431-008-0746-6
186. Jorens PG, Sibille Y, Goulding NJ, van Overveld FJ, Herman AG, Bossaert L, et al. Potential role of Clara cell protein, an endogenous phospholipase A2 inhibitor, in acute lung injury. Eur Respir J. (1995) 8(10):1647–53. doi: 10.1183/09031936.95.08101647
187. Broeckaert F, Clippe A, Knoops B, Hermans C, Bernard A. Clara cell secretory protein (CC16): features as a peripheral lung biomarker. Ann N Y Acad Sci. (2000) 923:68–77. doi: 10.1111/j.1749-6632.2000.tb05520.x
188. Wolfson MR, Funanage VL, Kirwin SM, Pilon AL, Shashikant BN, Miller TL, et al. Recombinant human Clara cell secretory protein treatment increases lung mRNA expression of surfactant proteins and vascular endothelial growth factor in a premature lamb model of respiratory distress syndrome. Am J Perinatol. (2008) 25(10):637–45. doi: 10.1055/s-0028-1090587
189. Miller TL, Shashikant BN, Melby JM, Pilon AL, Shaffer TH, Wolfson MR. Recombinant human Clara cell secretory protein in acute lung injury of the rabbit: effect of route of administration. Pediatr Crit Care Med. (2005) 6(6):698–706. doi: 10.1097/01.pcc.0000165565.96773.08
190. Levine CR, Gewolb IH, Allen K, Welch RW, Melby JM, Pollack S, et al. The safety, pharmacokinetics, and anti-inflammatory effects of intratracheal recombinant human Clara cell protein in premature infants with respiratory distress syndrome. Pediatr Res. (2005) 58(1):15–21. doi: 10.1203/01.PDR.0000156371.89952.35
191. Davis JM, Pilon AL, Shenberger J, Breeze JL, Terrin N, Mazela J, et al. The role of recombinant human CC10 in the prevention of chronic pulmonary insufficiency of prematurity. Pediatr Res. (2019) 86(2):254–60. doi: 10.1038/s41390-019-0419-3
192. Poonyagariyagorn HK, Metzger S, Dikeman D, Mercado AL, Malinina A, Calvi C, et al. Superoxide dismutase 3 dysregulation in a murine model of neonatal lung injury. Am J Respir Cell Mol Biol. (2014) 51(3):380–90. doi: 10.1165/rcmb.2013-0043OC
193. Delaney C, Wright RH, Tang JR, Woods C, Villegas L, Sherlock L, et al. Lack of EC-SOD worsens alveolar and vascular development in a neonatal mouse model of bleomycin-induced bronchopulmonary dysplasia and pulmonary hypertension. Pediatr Res. (2015) 78(6):634–40. doi: 10.1038/pr.2015.166
194. Perveen S, Patel H, Arif A, Younis S, Codipilly CN, Ahmed M. Role of EC-SOD overexpression in preserving pulmonary angiogenesis inhibited by oxidative stress. PLoS One. (2012) 7(12):e51945. doi: 10.1371/journal.pone.0051945
195. Rosenfeld W, Evans H, Concepcion L, Jhaveri R, Schaeffer H, Friedman A. Prevention of bronchopulmonary dysplasia by administration of bovine superoxide dismutase in preterm infants with respiratory distress syndrome. J Pediatr. (1984) 105(5):781–5. doi: 10.1016/s0022-3476(84)80307-8
196. Davis JM, Parad RB, Michele T, Allred E, Price A, Rosenfeld W, et al. Pulmonary outcome at 1 year corrected age in premature infants treated at birth with recombinant human CuZn superoxide dismutase. Pediatrics. (2003) 111(3):469–76. doi: 10.1542/peds.111.3.469
197. Kicinski P, Malachowska B, Wyka K, Gach A, Jakubowski L, Gulczynska E. The level of extracellular superoxide dismutase in the first week of life in very and extremely low birth weight infants and the risk of developing bronchopulmonary dysplasia. J Perinat Med. (2019) 47(6):671–6. doi: 10.1515/jpm-2018-0418
198. Hallman M, Saugstad OD, Porreco RP, Epstein BL, Gluck L. Role of myoinositol in regulation of surfactant phospholipids in the newborn. Early Hum Dev. (1985) 10(3-4):245–54. doi: 10.1016/0378-3782(85)90055-6
199. Phelps DL, Ward RM, Williams RL, Watterberg KL, Laptook AR, Wrage LA, et al. Pharmacokinetics and safety of a single intravenous dose of myo-inositol in preterm infants of 23–29 wk. Pediatr Res. (2013) 74(6):721–9. doi: 10.1038/pr.2013.162
200. Howlett A, Ohlsson A, Plakkal N. Inositol in preterm infants at risk for or having respiratory distress syndrome. Cochrane Database Syst Rev. (2019) 7(7):CD000366. doi: 10.1002/14651858.CD000366.pub4
201. Valentine CJ. Maternal dietary DHA supplementation to improve inflammatory outcomes in the preterm infant. Adv Nutr. (2012) 3(3):370–6. doi: 10.3945/an.111.001248
202. Manley BJ, Makrides M, Collins CT, McPhee AJ, Gibson RA, Ryan P, et al. High-dose docosahexaenoic acid supplementation of preterm infants: respiratory and allergy outcomes. Pediatrics. (2011) 128(1):e71–7. doi: 10.1542/peds.2010-2405
203. Collins CT, Makrides M, McPhee AJ, Sullivan TR, Davis PG, Thio M, et al. Docosahexaenoic acid and bronchopulmonary dysplasia in preterm infants. N Engl J Med. (2017) 376(13):1245–55. doi: 10.1056/NEJMoa1611942
204. Marc I, Piedboeuf B, Lacaze-Masmonteil T, Fraser W, Mâsse B, Mohamed I, et al. Effect of maternal docosahexaenoic acid supplementation on bronchopulmonary dysplasia-free survival in breastfed preterm infants: a randomized clinical trial. JAMA. (2020) 324(2):157–67. doi: 10.1001/jama.2020.8896
205. Ley D, Hallberg B, Hansen-Pupp I, Dani C, Ramenghi LA, Marlow N, et al. rhIGF-1/rhIGFBP-3 in preterm infants: a phase 2 randomized controlled trial. J Pediatr. (2019) 206:56–65.e8. doi: 10.1016/j.jpeds.2018.10.033
206. Vadivel A, Alphonse RS, Etches N, van Haaften T, Collins JJ, O'Reilly M, et al. Hypoxia-inducible factors promote alveolar development and regeneration. Am J Respir Cell Mol Biol. (2014) 50(1):96–105. doi: 10.1165/rcmb.2012-0250OC
207. Nadeau-Vallée M, Chin PY, Belarbi L, Brien MÈ, Pundir S, Berryer MH, et al. Antenatal suppression of IL-1 protects against inflammation-induced fetal injury and improves neonatal and developmental outcomes in mice. J Immunol. (2017) 198(5):2047–62. doi: 10.4049/jimmunol.1601600
208. Royce SG, Nold MF, Bui C, Donovan C, Lam M, Lamanna E, et al. Airway remodeling and hyperreactivity in a model of bronchopulmonary dysplasia and their modulation by IL-1 receptor antagonist. Am J Respir Cell Mol Biol. (2016) 55(6):858–68. doi: 10.1165/rcmb.2016-0031OC
209. Zhang ZQ, Hong H, Li J, Li XX, Huang XM. MicroRNA-214 promotes alveolarization in neonatal rat models of bronchopulmonary dysplasia via the PlGF-dependent STAT3 pathway. Mol Med. (2021) 27(1):109. doi: 10.1186/s10020-021-00374-4
Keywords: bronchopulmonary dysplasia, neonatal care, postnatal corticosteroids, preterm infants, lung disease
Citation: Dini G, Ceccarelli S and Celi F (2024) Strategies for the prevention of bronchopulmonary dysplasia. Front. Pediatr. 12: 1439265. doi: 10.3389/fped.2024.1439265
Received: 27 May 2024; Accepted: 16 July 2024;
Published: 24 July 2024.
Edited by:
Giuseppe De Bernardo, Ospedale Buon Consiglio Fatebenefratelli, ItalyReviewed by:
Hyun Ho Kim, Jeonbuk National University, Republic of Korea© 2024 Dini, Ceccarelli and Celi. This is an open-access article distributed under the terms of the Creative Commons Attribution License (CC BY). The use, distribution or reproduction in other forums is permitted, provided the original author(s) and the copyright owner(s) are credited and that the original publication in this journal is cited, in accordance with accepted academic practice. No use, distribution or reproduction is permitted which does not comply with these terms.
*Correspondence: Gianluca Dini, gianlucadini90@gmail.com