- 1Pediatric Intensive Care Unit, Department of Pediatrics CHU Sainte-Justine, Université de Montréal, Montreal, QC, Canada
- 2Faculty of Medicine of Nancy, University of Lorraine, INSERM UMR_S 1256, Nutrition, Genetics, and Environmental Risk Exposure (NGERE), Nancy, France
- 3Biochemical and Molecular Medicine Department CHU Sainte-Justine, Université de Montréal, Montreal, QC, Canada
- 4Department of Pediatrics CHU Sainte-Justine, Université de Montréal, Montreal, QC, Canada
- 5Hepato-gastro-enterology and Nutrition Unit, Department of Pediatrics CHU Sainte-Justine, Université de Montréal, Montreal, QC, Canada
Purpose: Renal replacement therapy (RRT) is used in hyperammonemia to reduce the concentration of ammonia in the blood. In the case of plasma hyperosmolarity, RRT can also rapidly decrease plasma osmolarity, which may increase cerebral edema in these patients and favor the occurrence of brain herniation.
Methods: We conducted a retrospective clinical study in a tertiary care university-affiliated hospital. All patients admitted in a Pediatric Intensive Care Unit (PICU), less than 18 years old with ammonemia >150 µmol/L and who underwent RRT between January 2015 and June 2023 were included. We collected data on plasma osmolarity levels, osmolar gap and blood ammonia levels before and during RRT.
Results: Eleven patients were included (10 with acute liver failure and 1 with a urea cycle disorders). Their mean age was 36.2 months. Before RRT, the median highest measured osmolarity was 320 (305–324) mOsm/L, whereas the median calculated osmolarity was 303 (293–314) mOsm/L, corresponding to an osmolar gap of 14 mOsm/L. Ammonia blood level over 400 µmol/L are significantly associated with higher plasma osmolarity (P-Value <0.001). In one case, a patient had a brain herniation episode after a quick osmolar drop. This episode was reversed by the administration of hyperosmolar agents and the temporary suspension of RRT.
Conclusion: This study highlights the hyperosmolarity and high osmolar gap that occur in children with hyperammonemia. A careful monitoring and control of plasma osmolarity evolution may alert clinician on the risk of occurrence of neurological complication such as brain herniation.
Introduction
Ammonia (NH3) is a metabolic by-product resulting from the deamination of amino acids, a process catalyzed by enzymes that yield organic acids essential for synthesizing neurotransmitters and hormones. However, high concentrations of ammonia can be toxic (1). The liver converts ammonia into urea via the urea cycle (2), which is the main component of urine. Hyperammonemia is defined as an elevated blood ammonia concentration exceeding 80 µmol/L in neonates and above 50 µmol/L in infants, adolescents, and adults (3). This condition may manifest in cases of acute liver failure (ALF) as well as chronic liver failure (CLF) (4). Inherited metabolic diseases are caused by genetic defects affecting enzymes involved in human metabolism. Hyperammonemia occurs in cases of enzyme deficiencies within the urea cycle (3), as well as in organic acidurias and defects of mitochondrial fatty acid oxidation (5) due to secondary deficiencies in urea cycle metabolism (6).
Ammonia exhibits specific neurotoxic effects, manifesting with symptoms ranging from coma to seizures, and in more severe cases, can lead to death (1). This neurotoxicity is partially explained by the metabolism of glutamine by astrocytes, which result in cerebral edema (7). Treatment modalities primarily focus on reducing protein intake to limit nitrogen intake and utilizing ammonia scavengers to facilitating the elimination of excess ammonia (8, 9). Additionally, interventions targeting the intestinal microbiota, achieved through the administration of antibiotics or lactulose (10), also play a role. In cases of severe encephalopathy, or when the blood ammonia level exceeds 150 µmol/L, or if hyperammonemia proves refractory to medication, prompt initiation of renal replacement therapy (RRT) is strongly recommended to efficiently clear excessive ammonia (11).
All continuous RRT therapeutic modalities are safe and efficacious methods used for inborn errors of metabolism for more than thirty years (11–13). These treatments yield acceptable biochemical levels of ammonia in a couple of hours. Nonetheless, a notable mortality rate is still observed, often correlated with a higher concentration of ammonia in the blood (3). Hyperammonemia cases have been associated with an elevation in plasma osmolarity, resulting in an osmolar gap in afflicted patients (14, 15). But ammonia is not the only contributor to elevated osmolarity, unrecognized osmotically active molecules that accumulate in liver failure may contribute to this osmolar gap (15). Significant fluctuations in cerebral edema are documented in this population, accompanied by an increased vulnerability to neurologic complications such as brain herniation (16).
Dialysis disequilibrium syndrome (DDS), akin to that observed in patients with renal failure, may also ensue in the context of hyperammonemia. The acute reduction of blood urea through dialysis engenders osmotic shifts and consequent cerebral edema (17). Similar observations may occur in case of hyperammonemia. Thus, this study aims to document the osmolarity level at admission in children with hyperammonemia, and the evolution of plasma osmolarity during RRT.
Material and methods
Population
We performed a retrospective longitudinal study in our 32-bed Pediatric Intensive Care Unit (PICU) of a tertiary care university-affiliated hospital in Montréal, Québec, Canada. The unit is a medical-surgical PICU that admits all critically ill children including cardiac surgery and organ transplantation patients. We included all patients under 18 years old who underwent Renal Replacement Therapy (RRT) with an ammonemia blood level exceeding 150 µmol/L between January 2015 and June 2023, and for whom a plasma osmolarity measurement were available. We chose 150 µmol/L as the threshold for hyperammonemia based on recommendations for initiating RRT in cases with neurological manifestations (11). Blood samples for ammonia level evaluation were collected in heparin tubes and immediately placed on ice. Ammonia concentrations were measured using a Beckman Coulter LX-20 automat analyzer with spectrophotometric enzymatic method and Beckman reagents (18). Exclusion criteria included brain death and decision to withdraw or withhold therapy at PICU admission.
This study was approved by the research ethics board of CHU Sainte-Justine, protocol number 2024-6483.
Data sources and study variables
Retrospective identification of patients was done by cross-referencing the laboratory database with the PICU database. Time zero for inclusion was defined as the time of initiation of RRT. Data were extracted from medical charts and collected with a standardized form after codification.
Data collected included sex, age, weight, patients’ underlying condition and etiology of hyperammonemia, neurologic manifestations, biological characteristics at admission, and hyperammonemia treatments (medication and/or extracorporeal removal therapies). Serum ammonia, osmolarity, and blood osmolar gap levels were noted at the time of initiation of RRT, at 3 and 6 h before RRT initiation and at 3, 6, 9, 12, 15, 18, 21, 24, and 27 h after (when data was available). When biological data were available, we calculated osmolarity according to the following formula: [2 × (Na+)] + [glucose] + [urea]). The osmolar gap was calculated as the difference between measured osmolarity and calculated one.
Statistical analysis
Ammonia blood level, blood osmolarity and osmolar gap evolution were recorded for each patient. Biological data ad admission and before CRRT initiation are quoted as median [interquartile range (IQR)] and n values as percentages. Evolution of blood osmolarity according to time is presented as mean (+/-SD). Comparisons between patients with ammonemia blood level over 400 µmol/L and those who have ammonemia blood level under 400 µmol/L were conducted using Wilcoxon tests for continuous variables. A Spearman test was to look for a link between plasma ammonia concentration and plasma osmolarity. A P-Value below 0.05 was considered as statistically significant. Analyses were performed using R, version 4.3.1 (2023-06-16) (R Foundation for Statistical Computing, Vienna, Austria).
Results
Eleven patients were included in this study. 64% are female, with a median age of 36.2 months. Their clinical and biochemical characteristics are presented in Table 1. Most patients (91%) had hyperammonemia caused by an acute liver failure (ALF), resulting in increased INR and liver enzymes (Table 2).
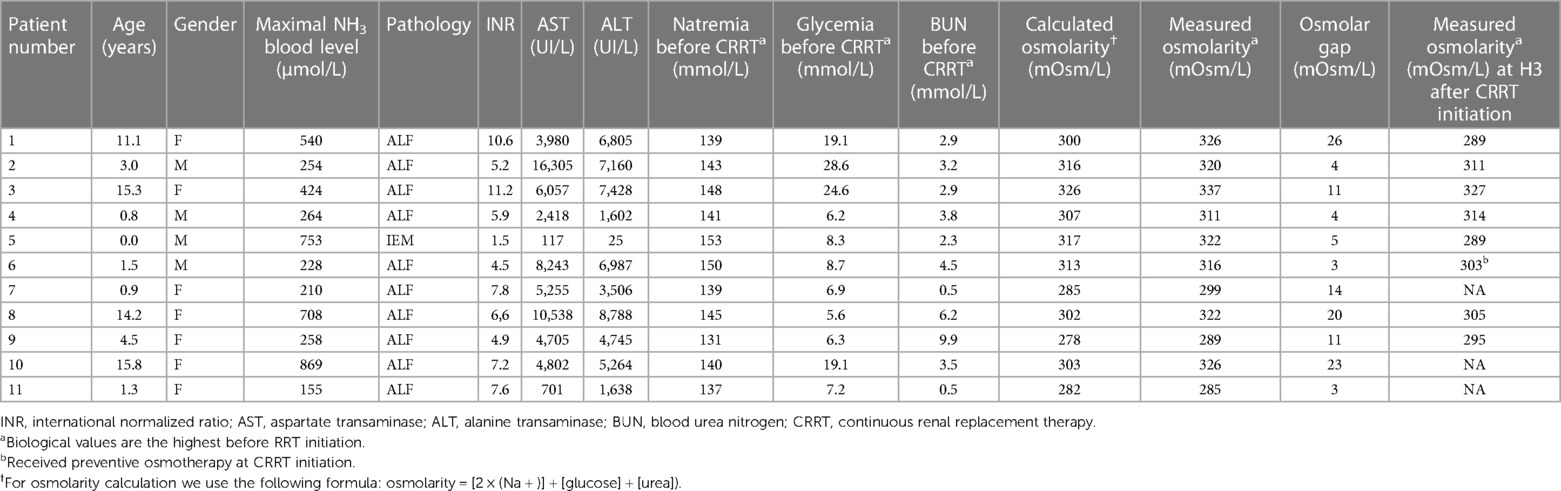
Table 2 Individually clinical and biological (measured and calculated osmolarity) characteristics before CKKT initiation.
Ammonia blood level, measured plasma osmolarity, calculated plasma osmolarity, and plasma osmolar gap before the start of RRT were increased (Table 2). Median highest measured osmolarity within 6 h before CRRT initiation was 320 (305–324) mOsm/L whereas the median calculated osmolarity was 303 (293–314) mOsm/L. The four patients with ammonia blood level above 400 µmol/L had significant higher plasma osmolarity than those under 400 µmol/L: 326 mOsm/L vs. 305 mOsm/L (P-Value <0.001). The spearman correlation between ammonia blood level et plasma osmolarity is 0.77 (P-Value = 0.006). Patients with ALF also had a significant greater osmolar gap than the others: 21.5 mOsm/L vs. 4 mOsm/L (P-Value = 0.031).
Plasma osmolarity and ammonia concentration within the first 24 h following RRT initiation are shown in Figure 1A. As expected, ammonia concentration decreases as the RRT progresses.
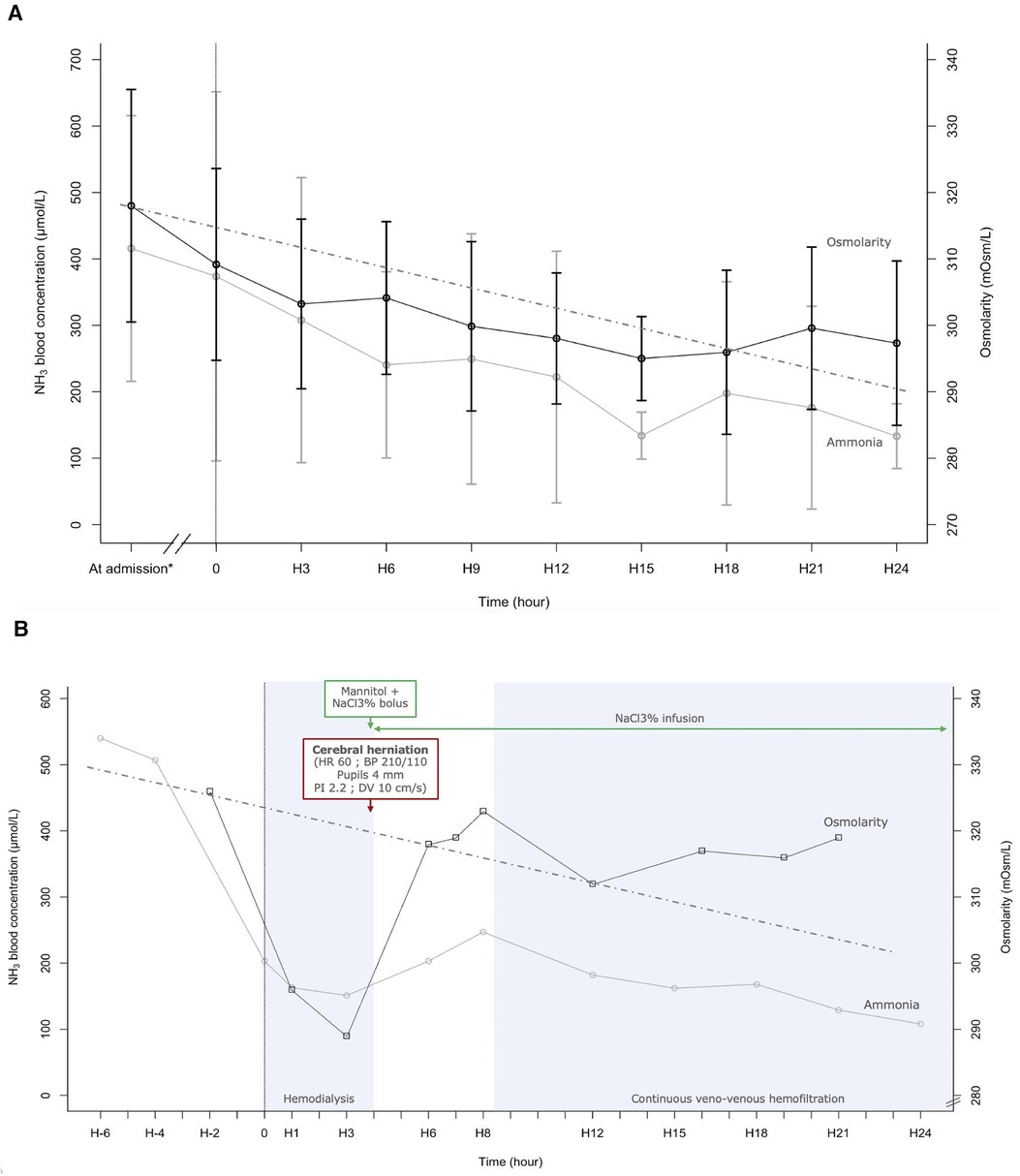
Figure 1 (A) Ammoniac blood concentration and plasma osmolarity during the 24 first hours after renal replacement therapy (RRT) initiation. Each point at “H” is an average of all measurements done 1.5 h before and after. The value at admission is an average of all values measured between 7.5 h and 1.5 h before RRT initiation. The dotted line indicates a reduction of 1 mOsm/L per hour (41). (B) Evolution of ammonia blood concentration and plasma osmolarity according to time and therapy, in a case of fulminant hepatitis (Patient 1). NaCl3%: 3% saline; HR, heart rate; BP, blood pressure; PI, pulsatility index; DV, diastolic velocity. This 11-year-old girl presented with fulminant hepatitis. Four hours after intermittent hemodialysis initiation (H4), she presented cushing reflex, bilateral mydriasis, and elevated intracranial pressure on transcranial doppler. cerebral herniation was reversed with cessation of hemodialysis, a bolus of mannitol and 3% saline, and with continuous infusion of 3% saline. Continuous veno-venous hemofiltration began at H8. Outcomes were good, with a complete recovery.
Plasma osmolarity also decreases, with the highest reduction during the first 3 h of RRT, dropping by 1.98 mOsm/L per hour. Mean osmolarity was 309 ± 14 mOsm/L at the beginning of RRT (H0), 303 ± 13 mOsm/L after 3 h of RRT (H3), and 301 ± 11 mOsm/L after 24 h of RRT (H24).
One of the patients in this study was an 11-year-old girl with an ammonia concentration at admission of 540 µmol/L (Figure 1B). Evaluation of plasma amino acid chromatography observed an nonspecific increase of amino acids concentration equivalent to 8 and 10 mOsm/L. Lactulose, intravenous sodium benzoate and N-acetylcysteine were begun. Because of an altered state of consciousness and life-threatening hyperammonemia, intermittent hemodialysis (IHD) was initiated. 2 h before starting IHD, plasma osmolarity was 326 mOsm/L. Once IHD started, the osmolarity dropped to 296 mOsm/L after 1.2 h and to 289 mOsm/L after 2.8 h. On average, there was a decrease of 8 mOsm/L per hour in the 3 first hours of IHD. After 4 h of IHD, the patient experienced the onset of cerebral herniation. The patient had bradycardia, bilaterally dilated pupils, a hypertensive episode, and a drop in cerebral blood flow seen on transcranial Doppler (Pulsatility index in the middle cerebral artery 2.2 and diastolic flow velocity at 10 cm.s−1 (see Supplementary Figure S1). Two boluses of 3 ml/kg of 3% saline and one bolus of 1 g/kg of mannitol were administered, and IHD was stopped. Hemodynamic parameters and pupillary diameter returned to normal after a few minutes. A continuous infusion of 3% saline was started to maintain plasma osmolarity above 300 mOsm/L. Because of persistent severe hepatic encephalopathy, continuous veno-venous hemofiltration was initiated 4 h after IHD cessation.
10 patients discharge from PICU alive after a median length of hospitalisation of 9 days (8–10.5). 1 patient required liver transplantation (Patient 6). At hospital discharge, Patient 1 had a full liver and neurological function recovery.
Discussion
In our cohort of children with hyperammonemia, predominantly due to ALF, we observed a notable elevation in plasma osmolarity. Similar findings have been documented in critical care within the adult population affected with acute or acute-on-chronic liver failure and severe hepatic encephalopathy (14). RRT was successfully used in cases of PALF (19, 20), allowing children to be stabilized before a liver transplant or recovery. Inability to reduce ammonia by 48 h is associated with poor prognosis and death (20), which is particularly in favor of early treatment in these patients. In our study, a high level of ammonia in the blood is associated with an increase in osmolarity, partly due to many unmeasured amino acids. Consequently, rapid correction of hyperosmolarity within a few hours can have neurological consequences, as was the case for patient 1. Clinicians should be vigilant about this aspect to proactively avoid such complications.
Hepatic encephalopathy (HE) represents a significant complication not only in cases of ALF, but also in chronic liver failure (CLF) and acute-on-chronic liver failure (ACLF) (4). Pathophysiology of HE may diverge according to the underlying etiology of ALF (4). HE is a neuropsychiatric syndrome stemming from liver dysfunction, and it can manifest a wide spectrum of symptoms ranging from subtle neurologic impairment to coma and, ultimately, death because of brain herniation (21). Cerebral edema is one of the main mechanisms leading to HE. This phenomenon has been documented in both ALF and CLF (14, 22–24), and in rare congenital disorders associated with hyperammonemia (25). In case of liver failure, ammonia is not metabolized and is partially detoxified into glutamine. In the brain glutamine is osmotically active and is thought to be responsible for astrocyte swelling and cytotoxic edema seen in PALF (26, 27). Other amino acids increase are observed in case of liver failure (aromatic amino acids, sulfurated amino acids and dicarboxylic amino acids) and may have an osmolar activity (28). Ammonia increase and related amino acids disturbances, could lead to the cerebral herniation (29).
Acute hyperammonemia is a medical emergency which requires early treatment to maximize the patient's prognosis and to prevent irreversible brain injury. Typically, treatment is based on a multifaceted approach combining drugs and nutritional support in accordance with specific guidelines (3, 11, 30). Extracorporeal removal therapies, especially continuous RRT, are recommended in case of rapidly deteriorating neurological condition (coma or cerebral edema) accompanied by blood ammonia level exceeding 150 μmol/L, or in cases of severe encephalopathy, or in cases of high blood ammonia levels surpassing 400 μmol/L refractory to medical interventions (11).
Neurological manifestations are usually linked with hyperammonemia, exhibiting a dose-dependent relationship. Initially, patients may present with hypotony and lethargy, followed by seizures and coma. Pathology specimens have revealed the presence of cerebral edema with blooming astrocytes (7). Hyperammonemia leads to alterations in brain structure and its homeostasis (31), although the precise pathophysiological mechanisms remain not fully elucidated (32). Under normal physiologic conditions, astrocytes transform ammonia into glutamine by the glutamine synthetase, and release glutamine by the sodium-coupled neutral amino acid transporter (SNAT5) into the extracellular space. Accumulation of glutamine into brain leads to many pathological pathway, such as apoptosis of astrocyte, with a release of inflammatory cytokines (33), inhibit of alpha-ketoglutarate dehydrogenase, and so dysfunction of the Krebs cycle (34), decreased expression of glutamate receptors and activation of NMDA receptors by glutamate associated with calcium release (35). Hyperammonemia therefore causes cerebral suffering through different mechanisms, making neurons more sensitive to external attacks. In our cohort, we observed a significant increase in blood osmolarity co-occurring with hyperammonemia. Too rapid restoration of these imbalances, leading to a reduction in serum osmolarity, could exacerbate cerebral edema (14) like for example in situations of acute brain injury (36).
Dialysis disequilibrium syndrome (DDS) is a more common clinical situation in critical care. It is the clinical entity in which the effect of acute osmolarity change has been most studied. It manifests as a neurological complication, typically observed in patients undergoing their first hemodialysis session, resulting from a swift reduction in serum blood urea level (37). This leads to the establishment of an osmolar gradient between the brain and serum (38). Given the limited permeability of the blood-brain barrier to small molecules, water follows this osmolar gradient into the brain (36). These molecular alterations, in the presence of an osmolar gradient, facilitate the formation of cerebral edema (14). We could draw a parallel between this situation and cerebral edema due to hyperammonemia and the increase in other amino acids including glutamine.
To prevent the risk of DDS, it is widely recommended to slowly decrease the blood urea concentration. A goal of reducing urea concentration by 40% over a 2-hours span for the initial treatment is reasonable (39). Special attention should be given to children who are more susceptible to DDS (38). In cases of children with hypernatremia children, increase of cerebral edema is observed when osmolarity is reduced by 2 mOsm/L/h, and not observed when reduced by 1 mOsm/L/h (40). Current recommendations advocate for correcting chronic hypernatremia at a maximum rate of 0.5 mmol/L per hour (41), equivalent to reduction in plasma osmolarity by a maximum of 1 mOsm/L/h (40). Similarly, in hyperammonia, an osmolarity decrease of 1 mOsm/L/h could limit cerebral aggression. This rate of decrease was partially observed in our case series (Figure 1). This was not the case for patient 1, with a decrease of 37 osmol/L in 5 h (7.4 mOsm/L/h).
In cases of hyperammonemia, various RRT modalities are available. However, continuous kidney replacement therapy (CKRT), specifically high-dose CVVHD, is the recommended first-line treatment whenever feasible (11). Intermittent hemodialysis (IDH) is recommended in case of rapid deterioration in neurological status. IDH and high-dose CKRT are both considered when ammonia levels exceed 1,000 µmol/L (11). The challenge lies in the fact that clinicians aim to rapidly lower ammonia concentration but, as indicated in this study, overly swift correction may lead to severe neurological effects and could increase cerebral edema. Furthermore, the choice of RRT modality, dose, and filter membrane properties will influence the loss amino of acids and proteins in patients without protein intake (42). The loss of amino acids is directly linked to plasma amino acid concentration, CVVHD effluent volume, and filtration efficiency, assessed by the ratio of filtered urea nitrogen to blood urea nitrogen (43).
Continuous techniques appear to reduce amino acids effectively, which play a role in the genesis of the osmolar gap. Conversely, IHD demonstrates great efficacy in decreasing small molecules like ammonia, which may be responsible for DDS-like effects. In these patients, it is imperative to exercise caution and limit the decrease in osmolarity to a maximum of 1 mOsm/L/h. To limit changes in plasma osmolarity resulting from CKRT and/or IHD, the consideration of a preemptive infusion of 3% NaCl may be carefully discuss in cases of ALF with early neurocritical involvement (44).
Conclusion
Children suffering from hyperammonemia are exposed to various neurologic complications. In both cases of AFL and rare inborn metabolic diseases, an osmolar gap link to numerous amino acids and organic acids. Close monitoring of osmolarity seems useful to adapt therapeutic management if necessary and limit neurological damage. Further studies are needed to observe the impact of different CRRT modalities on ammonia clearance and osmolar gap correction, but also impact on cerebral edema.
Data availability statement
The raw data supporting the conclusions of this article will be made available by the authors, without undue reservation.
Ethics statement
The studies involving humans were approved by research ethics board of CHU Sainte-Justine, protocol number 2024-6483. The studies were conducted in accordance with the local legislation and institutional requirements. The Ethics Committee/institutional review board waived the requirement of written informed consent for participation from the participants or the participants’ legal guardians/next of kin because we conducted an observation retrospective study on medical datas.
Author contributions
YM: Formal Analysis, Investigation, Writing – original draft, Writing – review & editing. AW: Formal Analysis, Methodology, Supervision, Writing – original draft, Writing – review & editing. LB: Conceptualization, Investigation, Writing – review & editing. FP: Data curation, Formal Analysis, Investigation, Validation, Writing – review & editing. PA: Formal Analysis, Investigation, Methodology, Writing – review & editing. FA: Validation, Writing – review & editing. PJ: Conceptualization, Investigation, Methodology, Resources, Supervision, Validation, Writing – original draft, Writing – review & editing.
Funding
The author(s) declare that no financial support was received for the research, authorship, and/or publication of this article.
Acknowledgments
The authors would like to thank the caregivers that look after the critically ill children with hyperammonemia and the families and children who went through this stressful situation.
Conflict of interest
The authors declare that the research was conducted in the absence of any commercial or financial relationships that could be construed as a potential conflict of interest.
The author(s) declared that they were an editorial board member of Frontiers, at the time of submission. This had no impact on the peer review process and the final decision.
Publisher's note
All claims expressed in this article are solely those of the authors and do not necessarily represent those of their affiliated organizations, or those of the publisher, the editors and the reviewers. Any product that may be evaluated in this article, or claim that may be made by its manufacturer, is not guaranteed or endorsed by the publisher.
Supplementary material
The Supplementary Material for this article can be found online at: https://www.frontiersin.org/articles/10.3389/fped.2024.1431008/full#supplementary-material
Supplementary Figure S1
Middle cerebral artery transcranial doppler of the 11-year-old girl presented with fulminant hepatitis, before the beginning of the dialysis (A - left and B - right), during the herniation episode (C - left and D - right, see Fig 1B), and after the osmotherapy and stopping the dialysis (E - left).
References
1. Dasarathy S, Mookerjee RP, Rackayova V, Rangroo Thrane V, Vairappan B, Ott P, et al. Ammonia toxicity: from head to toe? Metab Brain Dis. (2017) 32(2):529–38. doi: 10.1007/s11011-016-9938-3
2. Matsumoto S, Haberle J, Kido J, Mitsubuchi H, Endo F, Nakamura K. Urea cycle disorders-update. J Hum Genet. (2019) 64(9):833–47. doi: 10.1038/s10038-019-0614-4
3. Haberle J, Burlina A, Chakrapani A, Dixon M, Karall D, Lindner M, et al. Suggested guidelines for the diagnosis and management of urea cycle disorders: first revision. J Inherit Metab Dis. (2019) 42(6):1192–230. doi: 10.1002/jimd.12100
4. Liotta EM, Kimberly WT. Cerebral edema and liver disease: classic perspectives and contemporary hypotheses on mechanism. Neurosci Lett. (2020) 721:134818. doi: 10.1016/j.neulet.2020.134818
5. Ribas GS, Lopes FF, Deon M, Vargas CR. Hyperammonemia in inherited metabolic diseases. Cell Mol Neurobiol. (2022) 42(8):2593–610. doi: 10.1007/s10571-021-01156-6
6. Valayannopoulos V, Baruteau J, Delgado MB, Cano A, Couce ML, Del Toro M, et al. Carglumic acid enhances rapid ammonia detoxification in classical organic acidurias with a favourable risk-benefit profile: a retrospective observational study. Orphanet J Rare Dis. (2016) 11(32). doi: 10.1186/s13023-016-0406-2
7. Cooper AJ, Plum F. Biochemistry and physiology of brain ammonia. Physiol Rev. (1987) 67(2):440–519. doi: 10.1152/physrev.1987.67.2.440
8. Brossier D, Goyer I, Ziani L, Marquis C, Mitchell G, Ozanne B, et al. Influence of implementing a protocol for an intravenously administered ammonia scavenger on the management of acute hyperammonemia in a pediatric intensive care unit. J Inherit Metab Dis. (2019) 42(1):77–85. doi: 10.1002/jimd.12029
9. Savy N, Brossier D, Brunel-Guitton C, Ducharme-Crevier L, Du Pont-Thibodeau G, Jouvet P. Acute pediatric hyperammonemia: current diagnosis and management strategies. Hepat Med. (2018) 10:105–15. doi: 10.2147/HMER.S140711
10. Liu J, Lkhagva E, Chung HJ, Kim HJ, Hong ST. The pharmabiotic approach to treat hyperammonemia. Nutrients. (2018) 10(2). doi: 10.3390/nu10020140
11. Raina R, Bedoyan JK, Lichter-Konecki U, Jouvet P, Picca S, Mew NA, et al. Consensus guidelines for management of hyperammonaemia in paediatric patients receiving continuous kidney replacement therapy. Nat Rev Nephrol. (2020) 16(8):471–82. doi: 10.1038/s41581-020-0267-8
12. Thompson GN, Butt WW, Shann FA, Kirby DM, Henning RD, Howells DW, et al. Continuous venovenous hemofiltration in the management of acute decompensation in inborn errors of metabolism. J Pediatr. (1991) 118(6):879–84. doi: 10.1016/S0022-3476(05)82198-5
13. Abily-Donval L, Dupic L, Joffre C, Brassier A, Arnoux JB, Grimaud M, et al. Management of 35 critically ill hyperammonemic neonates: role of early administration of metabolite scavengers and continuous hemodialysis. Arch Pediatr. (2020) 27(5):250–6. doi: 10.1016/j.arcped.2020.05.002
14. Liotta EM, Romanova AL, Lizza BD, Rasmussen-Torvik LJ, Kim M, Francis B, et al. Osmotic shifts, cerebral edema, and neurologic deterioration in severe hepatic encephalopathy. Crit Care Med. (2018) 46(2):280–9. doi: 10.1097/CCM.0000000000002831
15. Liotta EM, Karvellas CJ, Kim M, Batra A, Naidech A, Prabhakaran S, et al. Serum osmolality, cerebrospinal fluid specific gravity and overt hepatic encephalopathy severity in patients with liver failure. Liver Int. (2020) 40(8):1977–86. doi: 10.1111/liv.14400
16. Kok B, Karvellas CJ. Management of cerebral edema in acute liver failure. Semin Respir Crit Care Med. (2017) 38(6):821–9. doi: 10.1055/s-0037-1608772
17. Murphy N, Auzinger G, Bernel W, Wendon J. The effect of hypertonic sodium chloride on intracranial pressure in patients with acute liver failure. Hepatology. (2004) 39(2):464–70. doi: 10.1002/hep.20056
18. Ozanne B, Nelson J, Cousineau J, Lambert M, Phan V, Mitchell G, et al. Threshold for toxicity from hyperammonemia in critically ill children. J Hepatol. (2012) 56(1):123–8. doi: 10.1016/j.jhep.2011.03.021
19. Starr MC, Cater DT, Wilson AC, Wallace S, Bennett WE Jr., Hains DS. Association between continuous kidney replacement therapy clearance and outcome in pediatric patients with hyperammonemia not due to inborn error of metabolism. Pediatr Crit Care Med. (2022) 23(7):e356–e60. doi: 10.1097/PCC.0000000000002949
20. Deep A, Stewart CE, Dhawan A, Douiri A. Effect of continuous renal replacement therapy on outcome in pediatric acute liver failure. Crit Care Med. (2016) 44(10):1910–9. doi: 10.1097/CCM.0000000000001826
21. Vilstrup H, Amodio P, Bajaj J, Cordoba J, Ferenci P, Mullen KD, et al. Hepatic encephalopathy in chronic liver disease: 2014 practice guideline by the American association for the study of liver diseases and the European association for the study of the liver. Hepatology. (2014) 60(2):715–35. doi: 10.1002/hep.27210
22. Haussinger D. Low grade cerebral edema and the pathogenesis of hepatic encephalopathy in cirrhosis. Hepatology. (2006) 43(6):1187–90. doi: 10.1002/hep.21235
23. Rovira A, Cordoba J, Sanpedro F, Grive E, Rovira-Gols A, Alonso J. Normalization of T2 signal abnormalities in hemispheric white matter with liver transplant. Neurology. (2002) 59(3):335–41. doi: 10.1212/WNL.59.3.335
24. Liotta EM, Bauer RM, Berman MD, Guth JC, Maas MB, Naidech AM, et al. Acute changes in ventricular volume during treatment for hepatic and renal failure. Neurol Clin Pract. (2014) 4(6):478–81. doi: 10.1212/CPJ.0000000000000015
25. Sen K, Whitehead M, Castillo Pinto C, Caldovic L, Gropman A. Fifteen years of urea cycle disorders brain research: looking back, looking forward. Anal Biochem. (2022) 636:114343. doi: 10.1016/j.ab.2021.114343
26. Desjardins P, Du T, Jiang W, Peng L, Butterworth RF. Pathogenesis of hepatic encephalopathy and brain edema in acute liver failure: role of glutamine redefined. Neurochem Int. (2012) 60(7):690–6. doi: 10.1016/j.neuint.2012.02.001
27. Scott TR, Kronsten VT, Hughes RD, Shawcross DL. Pathophysiology of cerebral oedema in acute liver failure. World J Gastroenterol. (2013) 19(48):9240–55. doi: 10.3748/wjg.v19.i48.9240
28. Holecek M. Ammonia and amino acid profiles in liver cirrhosis: effects of variables leading to hepatic encephalopathy. Nutrition. (2015) 31(1):14–20. doi: 10.1016/j.nut.2014.03.016
29. Clemmesen JO, Larsen FS, Kondrup J, Hansen BA, Ott P. Cerebral herniation in patients with acute liver failure is correlated with arterial ammonia concentration. Hepatology. (1999) 29(3):648–53. doi: 10.1002/hep.510290309
30. Posset R, Garbade SF, Gleich F, Gropman AL, de Lonlay P, Hoffmann GF, et al. Long-term effects of medical management on growth and weight in individuals with urea cycle disorders. Sci Rep. (2020) 10(1):11948. doi: 10.1038/s41598-020-67496-3
31. Felipo V, Butterworth RF. Neurobiology of ammonia. Prog Neurobiol. (2002) 67(4):259–79. doi: 10.1016/S0301-0082(02)00019-9
32. Wijdicks EF. Hepatic encephalopathy. N Engl J Med. (2016) 375(17):1660–70. doi: 10.1056/NEJMra1600561
33. Butterworth RF. Effects of hyperammonaemia on brain function. J Inherit Metab Dis. (1998) 21(Suppl 1):6–20. doi: 10.1023/A:1005393104494
34. Clay AS, Hainline BE. Hyperammonemia in the ICU. Chest. (2007) 132(4):1368–78. doi: 10.1378/chest.06-2940
35. Paprocka J, Jamroz E. Hyperammonemia in children: on the crossroad of different disorders. Neurologist. (2012) 18(5):261–5. doi: 10.1097/NRL.0b013e318266f58a
36. Osgood M, Compton R, Carandang R, Hall W, Kershaw G, Muehlschlegel S. Rapid unexpected brain herniation in association with renal replacement therapy in acute brain injury: caution in the neurocritical care unit. Neurocrit Care. (2015) 22(2):176–83. doi: 10.1007/s12028-014-0064-y
37. Bellamkonda A, Chowdhury T, Shankar M, Gousy N. Dialysis disequilibrium syndrome: a red flag to check post hemodialysis. Cureus. (2022) 14(4):e24619. doi: 10.7759/cureus.24619
38. Raina R, Davenport A, Warady B, Vasistha P, Sethi SK, Chakraborty R, et al. Dialysis disequilibrium syndrome (DDS) in pediatric patients on dialysis: systematic review and clinical practice recommendations. Pediatr Nephrol. (2022) 37(2):263–74. doi: 10.1007/s00467-021-05242-1
39. Zepeda-Orozco D, Quigley R. Dialysis disequilibrium syndrome. Pediatr Nephrol. (2012) 27(12):2205–11. doi: 10.1007/s00467-012-2199-4
40. Mistry K. Dialysis disequilibrium syndrome prevention and management. Int J Nephrol Renovasc Dis. (2019) 12:69–77. doi: 10.2147/IJNRD.S165925
41. Didsbury M, See EJ, Cheng DR, Kausman J, Quinlan C. Correcting hypernatremia in children. Clin J Am Soc Nephrol. (2023) 18(3):306–14. doi: 10.2215/CJN.0000000000000077
42. Nystrom EM, Nei AM. Metabolic support of the patient on continuous renal replacement therapy. Nutr Clin Pract. (2018) 33(6):754–66. doi: 10.1002/ncp.10208
43. Frankenfield DC, Badellino MM, Reynolds HN, Wiles CE 3rd, Siegel JH, Goodarzi S. Amino acid loss and plasma concentration during continuous hemodiafiltration. JPEN J Parenter Enteral Nutr. (1993) 17(6):551–61. doi: 10.1177/0148607193017006551
Keywords: hyperosmolarity, hyperammonemia, liver failure, children, brain herniation
Citation: Maghmoul Y, Wiedemann A, Barcat L, Parente F, Allard P, Alvarez F and Jouvet P (2024) Hyperosmolarity in children with hyperammonemia: a risk of brain herniation at the start of renal replacement therapy. Front. Pediatr. 12:1431008. doi: 10.3389/fped.2024.1431008
Received: 11 May 2024; Accepted: 24 June 2024;
Published: 8 July 2024.
Edited by:
Adnan Bhutta, Riley Hospital for Children, United StatesReviewed by:
Riad Lutfi, Riley Children’s Health, United StatesDaniel Cater, Riley Hospital for Children, United States
© 2024 Maghmoul, Wiedemann, Barcat, Parente, Allard, Alvarez and Jouvet. This is an open-access article distributed under the terms of the Creative Commons Attribution License (CC BY). The use, distribution or reproduction in other forums is permitted, provided the original author(s) and the copyright owner(s) are credited and that the original publication in this journal is cited, in accordance with accepted academic practice. No use, distribution or reproduction is permitted which does not comply with these terms.
*Correspondence: Arnaud Wiedemann, YXJuYXVkLndpZWRlbWFubi5oc2pAc3Nzcy5nb3V2LnFjLmNh