- Department of Biological and Medical Science, Faculty of Physical Education and Sports, Comenius University in Bratislava, Bratislava, Slovakia
Acute lymphoblastic leukemia (ALL) treatment, involving chemotherapy, radiotherapy, and pharmacotherapy (antibiotics, antineoplastics) perturbs the gut microbiota in pediatric patients, with enduring effects post-treatment. ALL treatments diminish microbial richness and diversity, favoring pathogenic bacteria. Probiotics may offer promise in mitigating these disruptions and associated side effects. This mini-review explores the impact of ALL treatment on the gut microbiota and the potential benefits of probiotics in pediatric oncology. Probiotics have shown promise in restoring gut microbial balance, reducing treatment-associated side effects, and potentially improving quality of life. However, potential adverse effects, particularly in immunocompromised patients, warrant caution. Notably, there's emerging interest in probiotics’ role in bone health and mineral bioaccessibility. Further research is needed to elucidate probiotics’ mechanisms and their broader impact on pediatric health. Integration of probiotics into ALL treatment and post-treatment regimens offers significant potential for improving patient outcomes and reducing treatment-related complications and long-lasting disruptions, although careful monitoring is essential.
Introduction
Acute lymphoblastic leukemia (ALL) is the malignant transformation and proliferation of lymphoid progenitor cells. The disease results from the clonic expansion of abnormal lymphoid B lymphocyte progenitors (BCP-ALL) in 80% of all cases, or T lymphocytes (T-ALL) in 20% of all cases, which attack bone marrow, peripheral blood, and extramedullary sites (1, 2). About 75% of paediatric ALL develops from B-cell lineage precursors, with the remainder comprising malignant T-cell precursors (3). ALL used to be intractable, but now has a survival rate of up to 80%–90% (4). The incidence of ALL is highest in children aged 1–4 years and represents 80% of the most common cancer before the age of 15 years (5–7). The treatment of ALL typically involves chemotherapy, radiotherapy, and immunotherapy (8). While these therapies target cancer cells, they often also affect healthy cells and tissues, leading to significant disruptions in the body. One major impact is the destruction of the gut's microbiology, which weakens the immune system. Additionally, these treatments can cause a range of side effects, including hair loss, fatigue, anemia, and weight changes—particularly with chemotherapy (9). Digestive problems and skin issues such as dryness, redness, or itching are common with radiotherapy (10), while immunotherapy may result in fever and high blood pressure (11). Probiotics, as live microorganisms, could significantly contribute to the recovery of the intestinal microflora and mitigate treatment-related side effects (12).
Acute effects of ALL treatment on gut microbota composition
The human gastrointestinal tract harbors a diverse and ever-changing community of microorganisms, predominantly bacteria (of a number CFU exceeding 1014) that exert a substantial influence on the host. Approximately 90% of the this bacterial population is comprised of two phyla: gram-positive Bacillota synonym Firmicutes (including Bacillus spp., Lactobacillus spp., and Clostridium spp.) and gram-negative Bacteroidetes (13). A harmonious equilibrium among bacterial populations is essential for maintaining the homeostatic conditions of the gastrointestinal tract. Traditional treatments for ALL encompass chemotherapy, radiotherapy, and antimicrobial agents, that significantly disrupt this homeostasis (14). Research on gut microbiome perturbation during childhood treatment ALL has identified chemotherapy, antibiotics, immunosuppression, dietary modifications, and direct toxic effects as key contributors to changes in the gut ecosystem (15, 16). Furthermore, the consistently observed deficiency of short-chain fatty acid-producing bacterial taxa in children with ALL may exacerbate these gut ecosystem changes, leading to dysregulated immune responses and potentially increasing the risk of progression from preleukemic clones to overt leukemia (17, 18). Numerous investigations have delved into the ramifications of systemic cancer therapies on intestinal microflora composition (19, 20). Notably, multi-day regimens of high-dose chemotherapy have been associated with a marked reduction in microbial richness and gastrointestinal diversity (21). Furthermore, a discernible shift from gram-positive to gram-negative bacteria is statistically evident (22). Specifically, there is an escalation in the abundance of Bacteroidetes (including Bacteroides and Prevotella) and Proteobacteria (such as Enterobacteria, e.g., Escherichia coli, and Shigella sp.), alongside an increase in Firmicutes (comprising Lactobacillus sp. and Enterococcus sp.), while Actinobacteria (including Bifidobacterium sp.) exhibit a decrease (23). It was found that the total amount of flora in the stools of children with ALL decreased within 1 week by almost 30% after chemotherapy compared with healthy children (24). In our previous research, we confirmed the decline in bacterial diversity and richness in pediatric hematopoietic stem cell transplantation (HSCT) patients post-cancer treatment. Beneficial bacteria decreased notably from day 0 to day 90 after HSCT. Additionally, we reported a shift in gut microbiome composition, with a dominance of unclassified Enterococcus species and Enterococcaceae family during the 3 month period post-HSCT (25). Furthermore, the overuse of antibiotics in ALL treatment promotes the colonization of Clostridium difficile (26), an opportunistic pathogen responsible for antibiotic-associated diarrhea, while also reducing the presence of protective species like Bifidobacterium spp. (27). Noteworthy, Clostridium difficile infections, in conjunction with bloodstream infections, pose grave complications in pediatric oncology and bone marrow transplant recipients (28). A recent study demonstrated that IL-22, derived from the intestinal microbiota, regulates mucosal glycosylation, promotes the growth of the symbiotic bacterium Phascolarctobacterium, and enables it to compete with Clostridium difficile for succinate, thereby preventing Clostridium difficile infection (29).
Long effects of ALL treatment on gut microbiota composition
Although it is well known that cancer treatment can temporarily alter the composition of normal human microflora, long-term effects have also been demonstrated (30). Data from child and adolescent cancer patients confirmed that intestinal microbial dysfunction persists for months after intensive cancer (31). The microbiological profiles of both patient and sibling control groups are dominated by members of Bacteroides, Prevotella, and Faecalibacterium (31). At the level of the genus, both groups share many common taxa, but the diversity of micro-organisms in the patient group is significantly lower than in the control group. In the control group, higher proportions of Anaerostipes, Coprococcus, Roseburia, and Ruminococcus taxis were observed in pores with oncological patients (32). Further analysis of different bacterial species between ALL and control groups found that the relative abundance of Edwardsiella tarda and Prevotella maculosa was reduced in pediatric ALL patients and was positively correlated with interleukin-10 (IL-10) levels (18). It was reported that certain probiotic strains regulated T helper 17 (Th17) cell polarization, which in turn triggered innate lymphocytes to produce interleukin-22 (IL-22). IL-22, a key immune defense cytokine, played a crucial role in maintaining intestinal homeostasis and promoting tissue healing and regeneration (33). In terms of time to oncological treatment during the first 6 weeks of treatment, specifically in the induction phase, the rates of Streptococcaceae and Enterococcaceae were increasing (31). During the consolidation (9 months) and maintenance phases (2–3 years), the frequency of Lachnospiraceae and Clostridiceae decreases and Bifidobacteriaceae, Streptococcacea, and Enterococcaceae appear (34). Thomas et al. (35) found that the microbial composition at the end of therapy differed significantly from that of healthy children. Compared to healthy controls, the microbial diversity of adult patients who survived pediatric ALL and discontinued treatment for at least 5 years before observation was significantly reduced, with exceptional enrichment by actinobacteria. Therefore, microbial dysregulation due to chemotherapy and antibiotics during treatment may have long-term effects on the development of diseases other than obesity or diabetes in adults who have received ALL as children (36).
Probiotic benefits for post-therapy complications
Probiotic functions that are beneficial promote intestinal restoration and maintenance of homeostasis (37). Probiotics are living microorganisms that confer benefits to the host when administered in suitable dosages (38, 39). At present, probiotic bacteria can be found in a wide range of products, including foods such as yogurt, kefir, and fermented cabbage; dietary supplements, and medicines (40). In human nutrition, probiotic microorganisms are predominantly classified into the following genera: Lactobacillus, Bifidobacterium, Lactococcus, Streptococcus, and Enterococcus (41). Probiotics have demonstrated numerous beneficial effects on clinical outcomes, including treatment of mucositis, respiratory infections, and antibiotic-related digestion (42, 43). It was discovered that peritonitis patients exhibited a restoration of commensal intestinal bacteria, both in quantity and functionality, which had potentially been eradicated after the treatment (44). Treatment-related diarrhea is one of the most frequent and problematic adverse reactions associated with chemotherapy or radiotherapy in people with cancer. Its reported incidence was up to 50%–80% (45). Intestinal mucositis causing severe treatment-related diarrhea can lead to fluid and electrolyte losses and nutritional deficiencies and could adversely affect quality of life (46, 47). Probiotics may be effective in the prevention or treatment of diarrhea caused by chemotherapy or radiotherapy (48). Potentially, probiotic-mediated microbiota modulation could reduce chemotherapy-associated adverse effects and eradicate multidrug-resistant strains in child patients with hematologic malignancies (49). The beneficial effects of probiotics including a decrease in treatment-associated side effects and nausea and vomiting (49–51) are presented in Table 1. Despite limited research on gut microbiota restoration in patients in remission, our recent study confirmed the combined effect of probiotics and physical exercise on the structure of the intestinal microbiota in pediatric children with ALL in remission (52). The principal findings indicate that exercise and probiotics have a substantial positive effect on microbial alpha diversity.

Table 1. Therapeutic effect of probiotics in pediatric oncology patients with acute lymphoblastic leukemia (ALL).
The role of probiotics on quality of life
There are significant associations between intestinal microbes and psychoneurological symptoms (PNS), including fatigue, exercise capacity, anxiety, and depression, according to preclinical studies (53). Intestinal microbial dysfunction has been implicated in the development of anxiety and mood disorders in patients. Probiotics can modify the performance of functional brain activity and affect emotional attention, according to additional clinical studies (54). Probiotics have been linked to notable weight gain in underweight pediatric oncology patients exhibiting reduced appetite. By improving gut health, probiotics may reduce symptoms like bloating, diarrhea, and abdominal pain, which can significantly enhance overall well-being and quality of life (55, 56). Furthermore, alterations in mood and sleep quality were detected. Particularly, the research demonstrates progressive enhancements in diverse dimensions of the affective profile, including melancholy, ire, and lethargy, among individuals undergoing probiotic treatment in childhood leukemia (57). Reportedly, the quality of sleep has been enhanced despite the implementation of probiotics in both cancer patients and survivors (58).
Awareness and adverse effects
Although probiotics have been shown to have considerable advantages, their safety and effectiveness in immunocompromised patients are still unknown; therefore, care should be taken when administering them. It is crucial to take into account potential adverse effects associated with probiotics, such as systemic infections, gastrointestinal disturbances, skin reactions, acquisition of antibiotic resistance genes, detrimental impacts of probiotic metabolites, and atypical immune stimulation (54). Although probiotics are generally regarded as safe for healthy individuals, there is limited evidence to suggest otherwise for certain populations, including oncology patients in pediatrics. Pediatric patients can develop systemic infections, detrimental metabolic processes, heightened immune stimulation in vulnerable individuals, and the transfer of resistance genes (59). An extensive epidemiological study on patients revealed that instances of infectious endocarditis and bacteremia caused by Bifidobacteria and Lactobacillus were exceedingly rare, accounting for a mere 0.05%–0.4% of the total cases (60). Infections that are linked to probiotic strains of lactobacilli are exceedingly uncommon. Land et al. (61) and Avcin et al. (62) described two patients who were administered probiotic lactobacilli and bifidobacterium and subsequently developed bacteremia and sepsis. The risk of infection was found to be correlated not only with individual factors but also with the specific type and dosage of probiotics. Five out of 1,530 patients were found to have probiotic-related bacteremia, according to one study; however, probiotic therapy did reduce the frequency and severity of diarrhea in these cancer patients (63).
Future perspectives
The majority of probiotic research in recent years has focused on their effect on the composition of gut microbiota. However, there is still a fascinating and relatively unexplored area regarding how these microorganisms function through specific molecules, metabolic pathways, and transport carriers within their host, pediatric oncology patients (Figure 1). As mentioned earlier, reduced bone mineral density, a known side effect, is present during and persists a couple of years after the ALL treatment (64–66). Chemotherapy disrupts the balance between bone resorption and formation by affecting osteoclasts, leading to increased bone resorption (67). In children with ALL, ALP levels serve as a marker for monitoring bone health during treatment. Emerging research suggests that probiotics may influence bone metabolism and, as a result, potentially reflect these ALP levels (68). Therefore, it is critical to investigate factors such as mineral bioaccessibility and bioavailability associated with probiotic use, as well as shifts in the gut microbiota composition (69). Future strategies to improve intestinal permeability in pediatric ALL patients may focus on reducing the consequences of compromised gut barrier function, such as elevated zonulin levels (70). Interventions like targeted probiotics and dietary modifications could potentially mitigate intestinal inflammation and restore barrier integrity, thereby addressing the underlying factors that lead to increased zonulin and disrupted gut permeability. We think this area warrants further investigation and could yield valuable insights into the broader effects of probiotics on pediatric bone health.
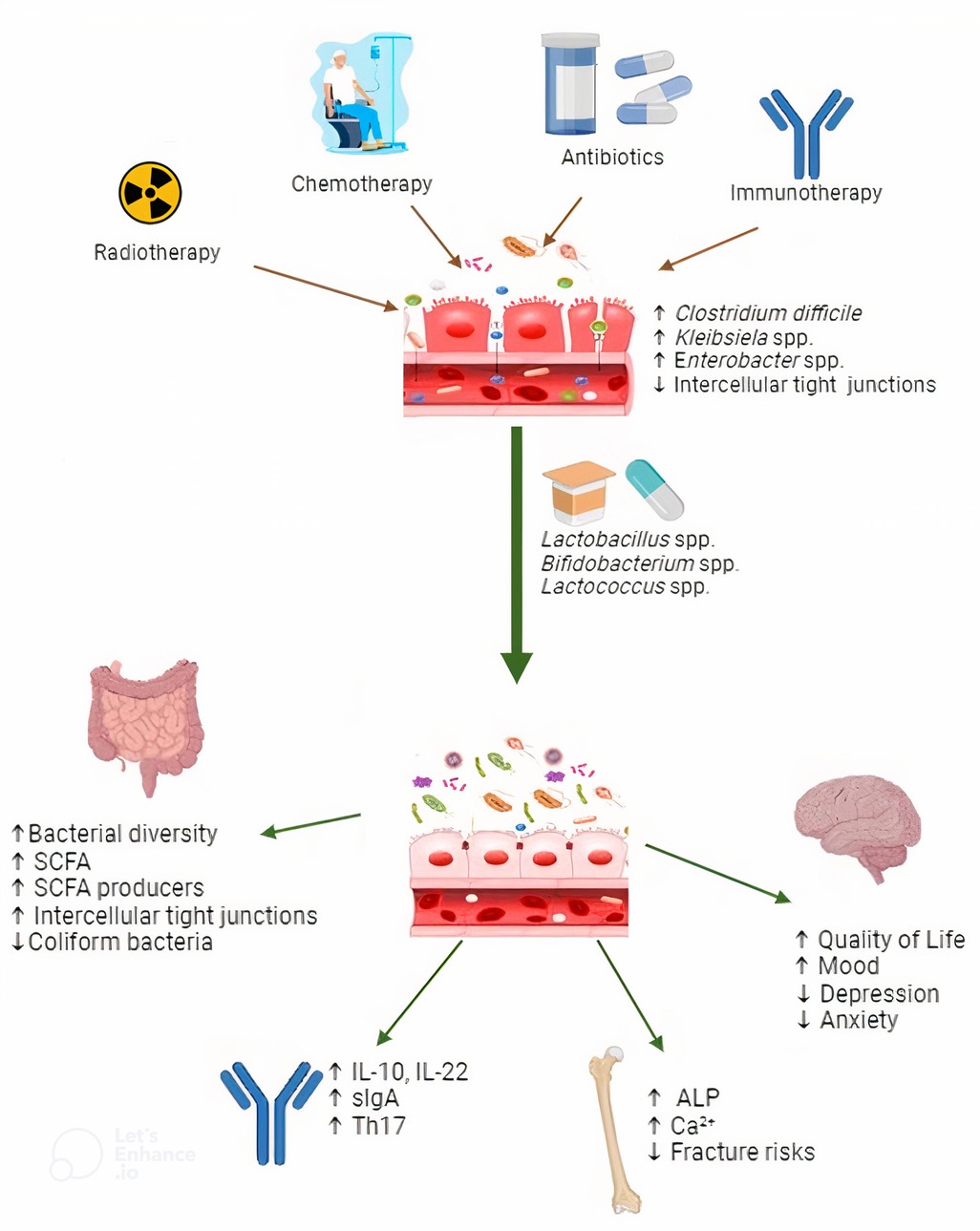
Figure 1. The effects of antibiotics, chemotherapy, radiotherapy, and immunotherapy on the gut microbiota in pediatric oncology patients with acute lymphoblastic leukemia (ALL), and the role of probiotics in mitigating these effects. Probiotics help restore microbial balance by promoting the growth of beneficial bacteria that produce short-chain fatty acids (SCFAs), inhibiting the growth of harmful coliform bacteria, and maintaining intestinal barrier integrity, including the restoration of intercellular tight junctions. They influence immune modulation, reflected in increased levels of IL-10, IL-22, secretory IgA (sIgA), and Th17 cells, which are markers of enhanced immune responses. Additionally, probiotics contribute to bone health by supporting calcium absorption and promoting bone remodeling, as indicated by elevated alkaline phosphatase (ALP) levels, ultimately lowering the risk of fractures. Probiotics also positively impact mental health by reducing symptoms of anxiety and depression, thereby improving the overall quality of life in these patients.
Conclusion
Despite limited research, this mini-review outlines the intricate relationship between acute lymphoblastic leukemia (ALL) treatment, gut microbiota, and the potential benefits of probiotics. It highlights the severe impact of chemotherapy and antibiotics on gut microbial diversity, leading to long-lasting changes even after treatment. Probiotics emerged as a promising solution, offering restoration of beneficial bacteria, reduction of treatment-related side effects, and potential improvements in quality of life for ALL patients. However, we advise caution regarding the risk of adverse reactions, especially in individuals with compromised immune systems. Overall, the integration of probiotics into ALL treatment regimens holds significant potential for enhancing patient outcomes and mitigating treatment-related complications. Based on current scientific evidence, we contend that, with cautious administration, the pros of probiotics for pediatric oncology patients outweigh the potential cons.
Author contributions
MŠ: Writing – original draft, Investigation, Formal Analysis, Data curation. VB: Writing – review & editing, Project administration, Funding acquisition, Conceptualization.
Funding
The author(s) declare financial support was received for the research, authorship, and/or publication of this article. This study was supported by the following granting schemes: APVV-17-0099, APVV-22-0047 and VEGA 1/0260/21.
Conflict of interest
The authors declare that the research was conducted in the absence of any commercial or financial relationships that could be construed as a potential conflict of interest.
Publisher's note
All claims expressed in this article are solely those of the authors and do not necessarily represent those of their affiliated organizations, or those of the publisher, the editors and the reviewers. Any product that may be evaluated in this article, or claim that may be made by its manufacturer, is not guaranteed or endorsed by the publisher.
References
1. Rudant J, Lightfoot T, Urayama KY, Petridou E, Dockerty JD, Magnani C, et al. Childhood acute lymphoblastic leukemia and indicators of early immune stimulation: a childhood leukemia international consortium study. Am J Epidemiol. (2015) 181(8):549–62. doi: 10.1093/aje/kwu298
2. Teachey DT, Pui CH. Comparative features and outcomes between paediatric T-cell and B-cell acute lymphoblastic leukaemia. Lancet Oncol. (2019) 20(3):e142–54. doi: 10.1016/S1470-2045(19)30031-2
3. Terwilliger T, Abdul-Hay M. Acute lymphoblastic leukemia: a comprehensive review and 2017 update. Blood Cancer J. (2017) 7(6):e577. doi: 10.1038/bcj.2017.53
4. Demidowicz E, Pogorzała M, Łęcka M, Żołnowska H, Marjańska A, Kubicka M, et al. Outcome of pediatric acute lymphoblastic leukemia: sixty years of progress. Anticancer Res. (2019) 39(9):5203–7. doi: 10.21873/anticanres.13717
5. Greaves M. Infection, immune responses and the etiology of childhood leukemia. Nat Rev Cancer. (2006) 6:193–203. doi: 10.1038/nrc1816
6. Figueroa ME, Chen SC, Andersson AK, Phillips LA, Li Y, Sotzen J, et al. Integrated genetic and epigenetic analysis of childhood acute lymphoblastic leukemia. J Clin Invest. (2013) 123(7):3099–111. doi: 10.1172/JCI66203
7. Wiemels J. Perspectives on the causes of childhood leukemia. Chem Biol Interact. (2012) 196(3):59–67. doi: 10.1016/j.cbi.2012.01.007
8. Ward E, DeSantis C, Robbins A, Kohler B, Jemal A. Childhood and adolescent cancer statistics, 2014. CA Cancer J Clin. (2014) 64(2):83–103. doi: 10.3322/caac.21219
9. van den Boogaard WMC, Komninos DSJ, Vermeij WP. Chemotherapy side-effects: not all DNA damage is equal. Cancers (Basel). (2022) 14(3):627. doi: 10.3390/cancers14030627
10. Barazzuol L, Coppes RP, van Luijk P. Prevention and treatment of radiotherapy-induced side effects. Mol Oncol. (2020) 14(7):1538–54. doi: 10.1002/1878-0261.12750
11. Zaidi N, Jaffee EM. Immunotherapy transforms cancer treatment. J Clin Invest. (2018) 129(1):46–7. doi: 10.1172/JCI126046
12. Reyna Figueroa J, Barrón-Calvillo E, García-Parra C, Galindo-Delgado P, Contreras-Ochoa C, Lagunas-Martínez A, et al. Probiotic supplementation decreases chemotherapy-induced gastrointestinal side effects in patients with acute leukemia. J Pediatr Hematol Oncol. (2019) 41:1. doi: 10.1097/MPH.0000000000001497
13. Arumugam M, Raes J, Pelletier E, Le Paslier D, Yamada T, Mende D, et al. Enterotypes of the human gut microbiome. Nature. (2011) 473:174–80. doi: 10.1038/nature09944
14. Kato M, Manabe A. Treatment and biology of pediatric acute lymphoblastic leukemia. Pediatr Int. (2018) 60(1):4–12. doi: 10.1111/ped.13457
15. Wen Y, Jin R, Chen H. Interactions between gut Microbiota and acute childhood leukemia. Front Microbiol. (2019) 10:1–30. doi: 10.3389/fmicb.2019.01300
16. Uribe-Herranz M, Klein-González N, Rodríguez-Lobato LG, Juan Otero M, de Larrea C. Gut microbiota influence in hematological malignancies: from genesis to cure. Int J Mol Sci. (2021) 22:1026. doi: 10.3390/ijms22031026
17. Peppas I, Ford AM, Furness CL, Greaves MF. Gut microbiome immaturity and childhood acute lymphoblastic leukaemia. Nat Rev Cancer. (2023) 23(8):565–76. doi: 10.1038/s41568-023-00584-4
18. Liu X, Zou Y, Ruan M, Chang L, Chen X, Wang S, et al. Pediatric acute lymphoblastic leukemia patients exhibit distinctive alterations in the gut microbiota. Front Cell Infect Microbiol. (2020) 10:1–9. doi: 10.3389/fcimb.2020.558799
19. Aarnoutse R, Ziemons J, Penders J, Rensen SS, de Vos-Geelen J, Smidt ML. The clinical link between human intestinal microbiota and systemic cancer therapy. Int J Mol Sci. (2019) 20(17):41–5. doi: 10.3390/ijms20174145
20. Montassier E, Batard E, Sebastien M, Gastinne T, Carton T, Caillon J, et al. 16S rRNA gene pyrosequencing reveals shift in patient faecal Microbiota during high-dose chemotherapy as conditioning regimen for bone marrow transplantation. Microb Ecol. (2014) 67:690–9. doi: 10.1007/s00248-013-0355-4
21. Galloway-Peña JR, Smith DP, Sahasrabhojane P, Ajami NJ, Wadsworth WD, Daver NG, et al. The role of the gastrointestinal microbiome in infectious complications during induction chemotherapy for acute myeloid leukemia. Cancer. (2016) 122(14):2186–96. doi: 10.1002/cncr.30039
22. Zhu Q, Li H, Zheng S, Wang B, Li M, Zeng W, et al. IL-6 and IL-10 are associated with gram-negative and gram-positive bacteria infection in lymphoma. Front Immunol. (2022) 13:1–9. doi: 10.3389/fimmu.2022.856039
23. Masetti R, Muratore E, Leardini D, Zama D, Turroni S, Brigidi P, et al. Gut microbiome in pediatric acute leukemia: from predisposition to cure. Blood Adv. (2021) 5(22):4619–29. doi: 10.1182/bloodadvances.2021005129
24. Wang H, Zhang Y, Zhou Q, Yu L, Fu J, Lin D, et al. Microbial metagenomic shifts in children with acute lymphoblastic leukaemia during induction therapy and predictive biomarkers for infection. Ann Clin Microbiol Antimicrob. (2024) 23(1):52. doi: 10.1186/s12941-024-00717-z
25. Ugrayová S, Švec P, Hric I, Šardzíková S, Kubáňová L, Penesová A, et al. Gut microbiome suffers from hematopoietic stem cell transplantation in childhood and its characteristics are positively associated with intra-hospital physical exercise. Biology (Basel). (2022) 11(5):1–14. doi: 10.3390/biology11050785
26. Pérez-Cobas AE, Artacho A. Differential effects of antibiotic therapy on the structure and function of human gut Microbiota. PLoS One. (2013) 8(11):1–13. doi: 10.1371/journal.pone.0080201
27. Panda S, El khader I. Short-term effect of antibiotics on human gut microbiota. PLoS One. (2014) 9(4):1–7. doi: 10.1371/journal.pone.0095476
28. Nycz Bryan T, Dominguez S. Evaluation of bloodstream infections, clostridium difficile infections, and gut microbiota in pediatric oncology patients. PLoS One. (2018) 13(1):1–16. doi: 10.1371/journal.pone.0191232
29. Nagao-Kitamoto H, Leslie JL, Kitamoto S, Jin C, Thomsson KA, Gillilland MG, et al. Interleukin-22-mediated host glycosylation prevents clostridioides difficile infection by modulating the metabolic activity of the gut microbiota. Nat Med. (2020) 26(4):608–17. doi: 10.1038/s41591-020-0764-0
30. Deleemans JM, Chleilat F, Reimer RA, Baydoun M, Piedalue KA, Lowry DE, et al. The chemo-gut pilot study: associations between gut microbiota, gastrointestinal symptoms, and psychosocial health outcomes in a cross-sectional sample of young adult cancer survivors. Curr Oncol. (2022) 29(5):2973–94. doi: 10.3390/curroncol29050243
31. Rajagopala S, Singh H, Yu Y, Zabokrtsky K, Torralba M, Moncera K, et al. Persistent gut microbial dysbiosis in children with acute lymphoblastic leukemia (ALL) during chemotherapy. Microb Ecol. (2020) 79:1034–43. doi: 10.1007/s00248-019-01448-x
32. Rajagopala S, Yooseph S, Harkins D, Moncera K, Zabokrtsky K, Torralba M, et al. Gastrointestinal microbial populations can distinguish pediatric and adolescent acute lymphoblastic leukemia (ALL) at the time of disease diagnosis. BMC Genomics. (2016) 17:1–10. doi: 10.1186/s12864-016-2965-y
33. Hrdý J, Alard J, Couturier-Maillard A, Boulard O, Boutillier D, Delacre M, et al. Lactobacillus reuteri 5454 and Bifidobacterium animalis ssp. lactis 5764 improve colitis while differentially impacting dendritic cells maturation and antimicrobial responses. Sci Rep. (2020) 10(1):5345. doi: 10.1038/s41598-020-62161-1
34. Hakim H, Dallas R, Wolf J, Tang L, Schultz-Cherry S, Darling V, et al. Gut microbiome composition predicts infection risk during chemotherapy in children with acute lymphoblastic leukemia. Clin Infect Dis. (2018) 67:541–8. doi: 10.1093/cid/ciy153
35. Thomas R, Wong WSW, Saadon R, Vilboux T, Deeken J, Niederhuber J, et al. Gut microbial composition difference between pediatric ALL survivors and siblings. Pediatr Hematol Oncol. (2020) 37(6):475–88. doi: 10.1080/08880018.2020.1759740
36. Chua LL, Rajasuriar R, Lim Y, Woo Y, Loke P, Ariffin H. Temporal changes in gut microbiota profile in children with acute lymphoblastic leukemia prior to commencement-, during-, and post-cessation of chemotherapy. BMC Cancer. (2020) 20:1–11. doi: 10.1186/s12885-020-6654-5
37. Singh A, Alexander SG, Martin S. Gut microbiome homeostasis and the future of probiotics in cancer immunotherapy. Front Immunol. (2023) 14:1–8. doi: 10.3389/fimmu.2023.1114499
38. Gibson GR, Hutkins R, Sanders ME, Prescott SL, Reimer RA, Salminen SJ, et al. Expert consensus document: the international scientific association for probiotics and prebiotics (ISAPP) consensus statement on the definition and scope of prebiotics. Nat Rev Gastroenterol Hepatol. (2017) 14(8):491–502. doi: 10.1038/nrgastro.2017.75
39. Rondanelli M, Faliva MA, Perna S, Castellazzi AM. Using probiotics in clinical practice: where are we now? A review of existing meta-analyses. Gut Microbes. (2017) 8(6):521–43. doi: 10.1080/19490976.2017.1345414
40. McFarland LV, Evans CT, Goldstein EJC. Strain-specificity and disease-specificity of probiotic efficacy: a systematic review and meta-analysis. Front Med (Lausanne). (2018) 5:1–14. doi: 10.3389/fmed.2018.00124
41. Chiu YH, Lin SL, Tsai JJ, Lin MY. Probiotic actions on diseases: implications for therapeutic treatments. Food Funct. (2014) 5(4):625–34. doi: 10.1039/c3fo60600g
42. Eghbali A, Ghaffari K, Rahimi Afzal R, Eghbali A, Ghasemi A. The effects of LactoCare synbiotic administration on chemotherapy-induced nausea, vomiting, diarrhea, and constipation in children with ALL: a double-blind randomized clinical trial. Pediatr Blood Cancer. (2023) 70:30–2. doi: 10.1002/pbc.30328
43. Aronica TS, Carella M, Balistreri CR. Different levels of therapeutic strategies to recover the microbiome to prevent/delay acute lymphoblastic leukemia (ALL) or arrest its progression in children. Int J Mol Sci. (2024) 25(7):1–20. doi: 10.3390/ijms25073928
44. Badgeley A, Anwar H, Modi K, Murphy P, Lakshmikuttyamma A. Effect of probiotics and gut microbiota on anti-cancer drugs: mechanistic perspectives. Biochim Biophys Acta Rev Cancer. (2020) 1875:188494. doi: 10.1016/j.bbcan.2020.188494
45. Wang Y, Xie H, Jiang H, Chen M, Ren L, Dong C. Analyzing the symptom clusters among children with acute lymphoblastic leukemia during chemotherapy based on electronic nursing records. Cancer Nurs. (2023) doi: 10.1097/NCC.0000000000001287. [Epub ahead of print]
46. Zareifar S, Farahmandfar MR, Cohan N, Modarresnia F, Haghpanah S. Evaluation of health related quality of life in 6–18 Years old patients with acute leukemia during chemotherapy. Indian J Pediatr. (2012) 79(2):177–82. doi: 10.1007/s12098-011-0483-0
47. De Pietri S, Ingham AC, Frandsen TL, Rathe M, Krych L, Castro-Mejía JL, et al. Gastrointestinal toxicity during induction treatment for childhood acute lymphoblastic leukemia: the impact of the gut microbiota. Int J Cancer. (2020) 147(7):1953–62. doi: 10.1002/ijc.32942
48. Wei D, Heus P, van de Wetering FT, van Tienhoven G, Verleye L, Scholten RJ. Probiotics for the prevention or treatment of chemotherapy- or radiotherapy-related diarrhoea in people with cancer. Cochrane Database Syst Rev. (2018) 8(8):CD008831. doi: 10.1002/14651858.CD008831.pub3
49. Ekert H, Jurk IH, Waters KD, Tiedemann K. Prophylactic co-trimoxazole and lactobacilli preparation in neutropenic patients. Med Pediatr Oncol. (1980) 8(1):47–51. doi: 10.1002/mpo.2950080108
50. Wada M, Nagata S, Saito M, Shimizu T, Yamashiro Y, Matsuki T, et al. Effects of the enteral administration of bifidobacterium breve on patients undergoing chemotherapy for pediatric malignancies. Support Care Cancer. (2010) 18:751–9. doi: 10.1007/s00520-009-0711-6
51. Reyna-Figueroa J, Barrón-Calvillo EE, García-Parra C, Galindo-Delgado P, Contreras-Ochoa CO, Lagunas-Martínez A, et al. Probiotic supplementation decreases chemotherapy-induced gastrointestinal side effects in patients with acute Leukemia. J Pediatr Hematol Oncol. (2019) 41:468–72. doi: 10.1097/MPH.0000000000001497
52. Bielik V, Hric I, SmahovA S, Tkačiková M, Hlaváčová V, Nechalová L, et al. The effect of physical exercise and dairy probiotics (Lactobacillus casei) on gut microbiome in childhood cancer survivors. Neoplasma. (2023) 70(04):588–96. doi: 10.4149/neo_2023_230526N287
53. Cryan JF, Dinan TG. Mind-altering microorganisms: the impact of the gut microbiota on brain and behaviour. Nat Rev Neurosci. (2012) 13:701–12. doi: 10.1038/nrn3346
54. Sturgeon M, Wetta-Hall R, Hart T, Good M, Dakhil S. Effects of therapeutic massage on the quality of life among patients with breast cancer during treatment. J Altern Complement Med. (2009) 15:373–80. doi: 10.1089/acm.2008.0399
55. Park H, Uhlemann A, Jacobs S, Mowbray C, Jubelirer T, Kelly K, et al. Title: obesogenic microbial signatures and the development of obesity in childhood acute lymphoblastic leukemia. Leuk Res. (2023) 126:107017. doi: 10.1016/j.leukres.2023.107017
56. Walters M, Mowbray C, Jubelirer T, Jacobs S, Kelly KM, Smith K, et al. A bilingual dietary intervention early in treatment is feasible and prevents weight gain in childhood acute lymphoblastic leukemia. Pediatr Blood Cancer. (2021) 68(5). doi: 10.1002/pbc.28910
57. Deleemans J, Gajtani Z, Baydoun M, Reimer R, Piedalue KA, Carlson L. The use of prebiotic and probiotic interventions for treating gastrointestinal and psychosocial health symptoms in cancer patients and survivors: a systematic review. Integr Cancer Ther. (2021) 20:153473542110617. doi: 10.1177/15347354211061733
58. Sung L, Yanofsky R, Klaassen R, Dix D, Pritchard S, Winick N, et al. Quality of life during active treatment for pediatric acute lymphoblastic leukemia. Int J Cancer J Int du Cancer. (2011) 128:1213–20. doi: 10.1002/ijc.25433
59. Sanders M, Akkermans L, Haller D, Hammerman C, Heimbach J, Hörmannsperger G, et al. Safety assessment of probiotics for human use. Gut Microbes. (2010) 1:164–85. doi: 10.4161/gmic.1.3.12127
60. Deleemans JM, Gajtani Z, Baydoun M, Reimer RA, Piedalue KA, Carlson LE. The use of prebiotic and probiotic interventions for treating gastrointestinal and psychosocial health symptoms in cancer patients and survivors: a systematic Review. Integr Cancer Ther. (2021) 20:15347354211061732. doi: 10.1177/15347354211061733
61. Land MH, Rouster-Stevens K, Woods CR, Cannon ML, Cnota J, Shetty AK. Lactobacillus sepsis associated with probiotic therapy. Pediatrics. (2005) 115(1):178–81. doi: 10.1542/peds.2004-2137
62. Avcin SL, Pokorn M, Kitanovski L, Premru MM, Jazbec J. Bifidobacterium breve sepsis in child with high-risk acute lymphoblastic leukemia. Emerg Infect Dis. (2015) 21(9):1674–5. doi: 10.3201/eid2109.150097
63. Chen E, Tecson M, Phan R. Abstracts from the American College of Clinical Pharmacy 2016 Virtual Poster Symposium. Pharmacother J Hum Pharmacol Drug Ther. (2016) 36(7):e83–138. doi: 10.1002/phar.1782
64. Inaba H, Cao X, Han AQ, Panetta JC, Ness KK, Metzger ML, et al. Bone mineral density in children with acute lymphoblastic leukemia. Cancer. (2018) 124(5):1025–35. doi: 10.1002/cncr.31184
65. Ghassemi A, Banihashem A, Ghaemi N, Elmi S, Sayyar R, Esmaily H. Evaluation of bone mineral density in children with acute lymphoblastic leukemia (ALL) and non-Hodgkin’s lymphoma (NHL): chemotherapy with/without radiotherapy. Int J Pediatr. (2016) 10:153–60.
66. Rohani F, Rafsanjani K, Bahoush G, Sabzehparvar M, Ahmadi M. Bone mineral density in survivors of childhood acute lymphoblastic leukemia. Asian Pac J Cancer Prev. (2017) 18:535–40. doi: 10.22034/APJCP.2017.18.2.535
67. Wasilewski-Masker K, Kaste SC, Hudson MM, Esiashvili N, Mattano LA, Meacham LR. Bone mineral density deficits in survivors of childhood cancer: long-term follow-up guidelines and review of the literature. Pediatrics. (2008) 121(3):e705–13. doi: 10.1542/peds.2007-1396
68. Crofton PM, Ahmed SF, Wade JC, Elmlinger MW, Ranke MB, Kelnar CJH, et al. Bone turnover and growth during and after continuing chemotherapy in children with acute lymphoblastic leukemia. Pediatr Res. (2000) 48(4):490–6. doi: 10.1203/00006450-200010000-00012
69. Bielik V, Kolisek M. Bioaccessibility and bioavailability of minerals in relation to a healthy gut microbiome. Int J Mol Sci. (2021) 22(13):1–18. doi: 10.3390/ijms22136803
Keywords: gut microbiota, LAB, child, Lactobacillus spp., alpha diversity
Citation: Šimiaková M and Bielik V (2024) The pros and cons of probiotic use in pediatric oncology patients following treatment for acute lymphoblastic leukemia. Front. Pediatr. 12:1427185. doi: 10.3389/fped.2024.1427185
Received: 3 May 2024; Accepted: 27 September 2024;
Published: 22 October 2024.
Edited by:
Davide Leardini, University of Bologna, ItalyReviewed by:
Szymon Skoczen, Jagiellonian University Medical College, PolandCopyright: © 2024 Šimiaková and Bielik. This is an open-access article distributed under the terms of the Creative Commons Attribution License (CC BY). The use, distribution or reproduction in other forums is permitted, provided the original author(s) and the copyright owner(s) are credited and that the original publication in this journal is cited, in accordance with accepted academic practice. No use, distribution or reproduction is permitted which does not comply with these terms.
*Correspondence: Viktor Bielik, viktor.bielik@uniba.sk