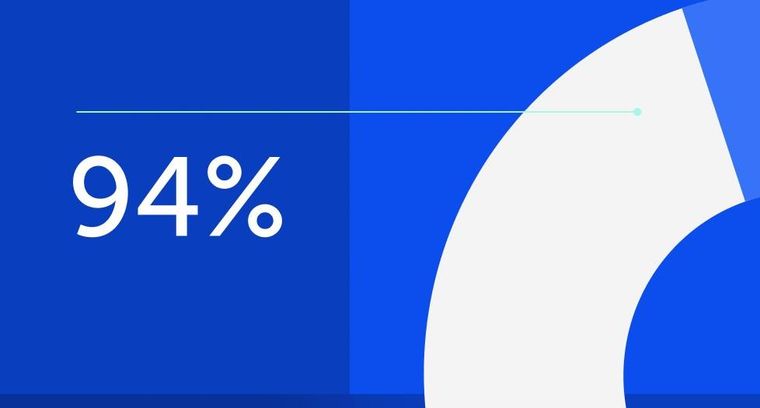
94% of researchers rate our articles as excellent or good
Learn more about the work of our research integrity team to safeguard the quality of each article we publish.
Find out more
ORIGINAL RESEARCH article
Front. Pediatr., 22 July 2024
Sec. Neonatology
Volume 12 - 2024 | https://doi.org/10.3389/fped.2024.1414185
Background: Circadian rhythms impact metabolism and the therapeutic effects of drugs. The purpose of this study was to determine the association between PER and CRY polymorphisms and caffeine citrate treatment response in infants with apnea of prematurity.
Methods: A total of 221 preterm infants of gestational age <34 weeks were included in this study (160 in the response group and 61 in the non-response group). The propensity score matching method was used to perform a 1:1 matching for all premature infants, and the general characteristics and clinical outcomes of the two groups were compared. The association between polymorphisms of the circadian transcription repressors PER and CRY and caffeine citrate treatment response in infants with apnea of prematurity was analyzed with co-dominant, dominant, recessive, and over-dominant models, as well as analysis of alleles. Generalized multifactor dimensionality reduction (GMDR) analysis was used to analyze the interaction between the PER and CRY genes.
Results: After propensity score matching, 45 preterm infants were included in each of the response and non-response groups, and there were no statistically significant differences in general characteristics between the two groups (P > 0.05). Infants in the non-response groups had a higher incidence of moderate and severe bronchopulmonary dysplasia (BPD) (P = 0.043), retinopathy of prematurity (ROP) (P = 0.035), and invasive ventilation (P = 0.027), and their duration of oxygen use (P = 0.041) was longer. When corrected for false discovery rate, the PER3 rs228669 recessive model (PFDR = 0.045) and the over-dominant model (PFDR = 0.045) were both associated with caffeine citrate treatment response. Preterm infants with the rs228669 CC genotype had a significantly lower rate of caffeine citrate non-response in the recessive model (OR = 0.28, 95% CI = 0.12–0.66), which was significantly higher in preterm infants with the CT genotype in the over-dominant model (OR = 4.18, 95% CI = 1.64–10.66). GMDR analysis revealed an interaction between the PER and CRY genes (P < 0.05).
Conclusions: Circadian rhythms may play a role in the response of premature infants to caffeine citrate, and polymorphisms of the PER and CRY genes may influence the effectiveness of caffeine citrate treatment for apnea of prematurity.
Apnea of prematurity (AOP), resulting from an immature brainstem and abnormal respiratory control in preterm infants, is a common phenomenon in the neonatal intensive care unit (NICU). AOP incidence has a negative correlation with gestational age, ranging in prevalence from 20% to 85% in infants born between 30 and 34 weeks of gestation, and reaching 100% for those born before 28 weeks (1). Caffeine citrate is the drug most commonly used to treat AOP, given its long half-life, high bioavailability, and minimal side effects, providing both short- and long-term clinical benefits (2). However, some preterm infants respond poorly to it despite adequate dosing (3–5). Its efficacy has been reported to be closely related to genetic factors as well as the infant's degree of development and the dose administered (6).
Recently, there has been increasing interest in environmental and temporal rhythmic cues in the NICU, including light cycles, temperature variations, sound levels, feeding rhythms, and timing of medication supply, and it has been recognized that these factors may impact newborn outcomes (7). Circadian rhythms, arising from natural selection during long-term evolution, aid in maintaining a variety of physiological functions in plants and animals and in regulating their adaptation to the internal and external environment. Disruption of circadian rhythms alters physiologic functions of human tissues and organs and disease occurrence, and affects drug pharmacokinetics within the body (8, 9).
The primary molecular mechanism generating circadian rhythms arises from the transcription-translation-feedback loop formed by the cyclic expression of circadian genes. There are four core clock components: the transcription activators circadian locomotor output cycles protein kaput (CLOCK) and brain and muscle arnt-like 1(BMAL1), and the transcription repressors cryptochrome (CRY) and period circadian protein (PER) (10). Caffeine delays the human circadian clock in vivo and in vitro, altering circadian rhythm phases (11) and affecting the sleep-wake cycle in preterm infants (12). CLOCK gene polymorphisms have been found to influence preterm infant response to caffeine citrate. This finding suggests that circadian rhythm genes may function in determining the clinical efficacy of caffeine citrate therapy for AOP (13). The transcription repressors PER and CRY are equally important for the formation and alternation of circadian rhythms, but their role in caffeine citrate response for AOP has not been determined. Therefore, this study sought to determine the impact of PER and CRY gene polymorphisms on the efficacy of caffeine citrate for treatment of AOP.
This prospective case-control study included premature infants cared for between October 2021 and June 2023 in the NICU of Women and Children's Hospital, School of Medicine, Xiamen University. Our study adhered to the principles of the Helsinki Declaration and was approved by the Ethics Committee of the Women and Children's Hospital, School of Medicine, Xiamen University (Approval No.: KY-2020-040).
Inclusion criteria: preterm infants born during this period at a gestational age of <34 weeks who were administered a standard dose of caffeine citrate within 24 h of birth for the treatment or prevention of AOP.
Exclusion criteria: Infants with (1) early onset sepsis (EOS); (2) grade III-IV intraventricular hemorrhage (IVH); (3) severe congenital malformations or chromosomal abnormalities; (4) failure to obtain umbilical cord blood samples or insufficient DNA concentration in umbilical cord blood; (5) discharge against medical advice based on parental decision.
For the included premature infants, general characteristics, including gestational age, birth weight, sex, mode of delivery, completion of antenatal corticosteroid therapy, small for gestational age (SGA), prolonged rupture of membranes (>18 h), 5-min Apgar score, patent ductus arteriosus (PDA), and neonatal respiratory distress syndrome (NRDS), and clinical treatment outcomes were collected. Clinical treatment outcomes included: moderate and severe bronchopulmonary dysplasia (BPD), retinopathy of prematurity (ROP), stage II or greater necrotizing enterocolitis (NEC), duration of oxygen use, use of invasive ventilation, reintubation after extubating, and duration of caffeine citrate administration.
(1) AOP: preterm infants (<37 weeks of gestational age) with cessation of breathing ≥20 s, or less than 20 s with decreased heart rate (<100 beats/min) and/or oxygen saturation (<85%) (1); (2) completion of antenatal corticosteroid therapy: two intramuscular doses of 12 mg of betamethasone to the mother over a 24-h period; (3) SGA: birth weight lower than the tenth percentile of infants of the same sex and gestational age, according to the 2013 Fenton curve; (4) PDA: persistence of a PDA for more than 72 h after birth, confirmed by echocardiography; (5) moderate (II) and severe (III) BPD: diagnosis and staging based on the National Institute of Child Health and Human Development (NICHD) diagnostic and grading criteria (2018) (14).; (6) stage II or greater NEC: diagnosis and staging based on the Bell criteria (15); (7) ROP: diagnosis according to the International Classification of Retinopathy of Prematurity, third edition (16); (8) NRDS: diagnosis according to the European Consensus Guidelines on the Management of Respiratory Distress Syndrome, 2022 (17); (9) EOS: blood or cerebrospinal fluid culture obtained within 72 h after birth with growth of a pathogenic bacterial species (18); (10) IVH: diagnosed and graded according to Papile et al. (19).
All preterm infants included in this study received appropriate respiratory support according to their condition on admission and were administered a standard dose of caffeine citrate within 24 h of admission: an intravenous loading dose of 20 mg/kg (1 ml:20 mg, containing 10 mg of caffeine and 10 mg of citrate, approved by the National Medical Products Administration with registration number H20163401 and manufactured by Chengdu Yuandong Biopharmaceutical Co., Ltd.) followed by a maintenance dose of 5 mg/kg/d. Caffeine citrate was switched to oral dosing once preterm infants achieved full enteral feeding (oral intake of 150 ml/kg/day). Caffeine citrate was discontinued once a corrected gestational age of 33–35 weeks was achieved and no AOP had occurred for 5–7 days. Based on the presence of AOP after caffeine citrate treatment, they were divided into non-response and response groups.
Definition of non-response: AOP occurring ≥2 times/day or once requiring bag and mask ventilation with supplemental oxygen after administration of the standard regimen within 3 d. Non-response preterm infants were given an additional dose of caffeine citrate at 5–10 mg/kg and the daily maintenance dose was increased to 10 mg/kg. If AOP persisted after this treatment, oxygen therapy was increased based on the infant's condition. Preterm infants who did not experience the above conditions were classified into the response group.
At birth of preterm infants, one ml of umbilical artery blood was collected in an EDTA anticoagulation tube and stored at −80 °C. DNA was extracted using the QIAamp DNA Blood Mini Kit (Qiagen, USA) according to the manufacturer's instructions, and DNA concentration was measured using a UV spectrophotometer (Thermo Fisher Scientific, USA). SNPs located in the 5' flanking sequence, 5' untranslated region (UTR), exons, introns, and 3' UTR of the PER and CRY genes with a minor allele frequency (MAF) ≥ 0.05 for East Asian populations were selected using the dbSNP database (https://www.ncbi.nlm.nih.gov/). The final selection included six SNPs for genotyping: CRY1 rs1056560, CRY2 rs1401419, PER1 rs2585405, PER2 rs934945, PER3 rs228669, and PER3 rs2640908. Genotyping was performed using the Agena Sequenom MassArray Analyzer 4 system (Matrix-Assisted Laser Desorption/ionization Time-Of Flight). Primer information is provided in the Supplementary Table S1.
Statistical analysis was conducted using SPSS V25.0. The Kolmogorov-Smirnov test was used to test normal distribution of continuous variables. Normally distributed samples are described using`X ± S and compared using the independent samples t-test, and non-normally distributed samples are described using the median (quartiles) and compared using the Mann-Whitney U rank sum test. Categorical variables are expressed as numbers and percentages and were compared using the χ2 test. A logistic regression model was established using gestational age as the independent variable and response to treatment with caffeine citrate as the dependent variable. Subsequently, 1:1 nearest neighbor matching was performed using a caliper value of 0.2 times the log standard deviation of propensity scores. SNP analyses were performed using co-dominant, dominant, recessive, and over-dominant models as well as alleles. A logistic regression analysis was used to calculate the odds ratio (OR) and 95% confidence interval (CI), and false discovery rate (FDR) correction was performed to reduce type I errors using the Benjamini-Hochberg procedure. Using GMDR Software Beta V 0.7, GMDR analysis was employed to explore the association between SNP interaction and the efficacy of caffeine citrate treatment for AOP. P < 0.05 was considered statistically significant.
A total of 287 preterm infants were treated for or given prophylactic therapy to prevent AOP within 24 h of birth using a standard dose of caffeine citrate during the study period, and 221 preterm infants were finally included after applying the exclusion criteria. The study enrolment process is shown in Figure 1.
Among the 221 preterm infants included, there were 160 (72.4%) infants in the response group and 61 (27.6%) infants in the non-response group. Statistical differences were identified between the two groups in gestational age, weight, 5 min Apgar score (P < 0.05). For clinical treatment outcomes, there were significant differences in PDA, and RDS, moderate and severe BPD, ROP, duration of oxygen use, invasive ventilation, reintubation after extubating, and duration of caffeine citrate use (P < 0.005).
Propensity score matching resulted in 45 preterm infants in both the response and non-response groups. There were no statistically significant differences (P > 0.05) in general characteristics between the two groups. Of clinical treatment outcomes, the non-response group had a higher incidence of moderate and severe BPD (P = 0.035), ROP (P = 0.035), and invasive ventilation (P = 0.027), as well as a longer duration of oxygen use (P = 0.041). The differences in the incidence of reintubation after extubating, NEC grade II or greater, and duration of caffeine citrate administration were not statistically significant (P > 0.05). Details are presented in Table 1.
Table 2 summarizes the comparison of genotypes and genetic models of the PER and CRY genes between the response and non-response groups. The genotype frequencies of the PER and CRY gene SNPs all conformed to the Hardy-Weinberg equilibrium (P > 0.05). The PER3 rs228669 allele frequency differed significantly between the groups (P < 0.05). Preterm infants with the rs228669 C allele had an increased rate of non-response to caffeine citrate (OR = 2.16, 95% CI = 1.11–4.20, P = 0.021). However, this result was not statistically significant after FDR correction (PFDR > 0.05).
Table 2 Association of PER and CRY gene polymorphisms with response to caffeine citrate in preterm infants.
In genetic model analysis, the genotype frequency differences between the response and non-response groups were statistically significant in the over-dominant model of CRY1 rs1056560, the over-dominant model of PER3 rs2640908, as well as the co-dominant, over-dominant, and recessive models of PER3 rs228669 (P < 0.05). CRY1 rs1056560 in the over-dominant model (OR = 0.36, 95% CI = 0.14–0.89, P = 0.025) and PER3 rs228669 in the recessive model (OR = 0.28, 95% CI = 0.12–0.66, P = 0.003) were associated with a decreased rate of non-response to caffeine citrate treatment in preterm infants. Conversely, PER3 rs228669 in the over-dominant model (OR = 4.18, 95% CI = 1.64–10.66, P = 0.002) and PER3 rs2640908 in the over-dominant model (OR = 2.77, 95% CI = 1.17–6.56, P = 0.019) were associated with an increased rate of non-response to caffeine citrate treatment. After FDR correction, the PER3 rs228669 recessive model (PFDR = 0.045) and the over-dominant model (PFDR = 0.045) remained associated with caffeine citrate treatment response. The rate of non-response to caffeine citrate was significantly lower in preterm infants with the rs228669 CC genotype in the recessive model (OR = 0.28, 95% CI = 0.12–0.66) and significantly higher in preterm infants with the CT genotype in the over-dominant model (OR = 4.18, 95% CI = 1.64–10.66). None of the remaining SNPs showed any association in any genetic models (P > 0.05).
GMDR analysis identified an interaction between the PER and CRY genes (Table 2 and Figure 2). The optimal SNP–SNP interaction models contained two and three genetic variants. The two-SNP model included PER rs228669 and PER rs2585405, with a cross-validation consistency of 9/10, a test accuracy of 0.6225, and a sign test of P = 0.0107. The three-SNP model included PER rs228669, PER rs2585405 and CRY2 rs1401419, with a cross-validation consistency of 7/10, a test accuracy of 0.6300, and a sign test of P = 0.0010 (Table 3).
Table 3 Association of gene-gene interactions of PER and CRY with response to caffeine citrate in preterm infants.
This study investigated the relationship between polymorphisms of the circadian transcription repressors PER and CRY, as well as their interactions, and the efficacy of caffeine citrate in treating AOP. After correction for multiple tests, the PER3 rs228669 polymorphism was found to be significantly associated with caffeine citrate response in preterm infants in both the recessive and over-dominant models (PFDR < 0.05). Specifically, preterm infants with the rs228669 CC genotype had a significantly lower rate of non-response to caffeine citrate in the recessive model (OR = 0.28, 95% CI = 0.12–0.66), and preterm infants with the CT genotype had a significantly higher rate of non-response to caffeine citrate in the over-dominant model (OR = 4.18, 95% CI = 1.64–10.66). In addition, GMDR analyses indicated that gene-gene interactions of the PER and CRY genes may increase the risk of preterm infants being non-responsive to caffeine citrate treatment (P < 0.05).
Caffeine citrate has been used to treat AOP in preterm infants for half a century, but considerable individual variation exists in preterm infant response, with some responding poorly despite adequate caffeine citrate dosing. Long et al. (6) reported that poor response may be associated with genetic factors, particularly polymorphisms in the adenosine receptor, aromatic hydrocarbon receptor, adenosine deaminase, and phosphodiesterase genes. Preterm infants with poor responses to treatment tend to have a worse prognosis, including longer duration of oxygen use and hospitalization, and an increased risk of invasive ventilation and death (3–5). On one hand, the increased duration of oxygen use and the use of invasive ventilation may lead to a higher risk of BPD and ROP. On the other hand, although caffeine may reduce the incidence of BPD through its anti-inflammatory effect, however, this effect may also be related to genetic factors. Kumral et al. (3) reported that the incidence of BPD was also significantly increased in preterm infants with adenosine receptor genotype that results in non-response to caffeine citrate. Our results showed an increase in the duration of oxygen administration (P < 0.05) and in the incidence of invasive ventilation, BPD, and ROP in the non-response group of preterm infants (P < 0.05), which is consistent with published reports. It seemed vital, then, to explore the genetics of the response to caffeine citrate treatment for AOP. Caffeine, as a potent adenosine receptor antagonist, not only delays central circadian rhythms, but also enhances circadian clock sensitivity to light (20). Both acute and long-term caffeine use can alter sleep homeostasis (21, 22). Recently, Guo et al. (13) reported that CLOCK gene polymorphisms involved in the expression of the CLOCK-BMAL1 heterodimers impact treatment of AOP with caffeine citrate, confirming for the first time that circadian genes are associated with the response of AOP to caffeine citrate treatment. Circadian rhythms are regulated by the central clock of the hypothalamic suprachiasmatic nucleus (SCN), which synchronizes with the external environment by receiving light signals from the retina and transmitting temporal information to nearly all of the body's cells (23). At the molecular level, at the beginning of a circadian rhythm cycle, CLOCK and BMAL1 form heterodimers in the cell nucleus and bind to the enhancer box elements (E-boxes) of the upstream promoter of the PER and CRY genes, initiating their transcription and translation. PER and CRY similarly form heterodimers and accumulate in the cytoplasm, subsequently translocating to the nucleus and repressing their own transcription by inhibiting BMAL1-CLOCK heterodimer activity, creating a negative feedback loop with a period of approximately 24 h (11). Circadian rhythms can influence the metabolic processes of drugs and affect the efficacy and toxicity of drugs based on the timing of administration (24). CLOCK gene polymorphisms are closely associated with circadian preference, sleep duration, and sleep disorders, and affect the efficacy of caffeine citrate in AOP treatment (13, 25). As two core transcription repressors, PER and CRY also play a vital role in regulating circadian rhythms. Therefore, we speculated that PER and CRY gene polymorphisms might impact caffeine citrate efficacy in treating AOP.
As we speculated, we found that the PER3 rs228669 polymorphism was significantly associated with AOP caffeine citrate treatment response in both the recessive and over-dominant models (PFDR < 0.05), with a significantly higher rate of caffeine citrate non-response in preterm infants with the CT genotype (OR = 4.18, 95% CI = 1.64–10.66), while preterm infants with the CC genotype had a significantly lower rate of non-response (OR = 0.28, 95% CI = 0.12–0.66). As an important gene regulating circadian rhythms, variants in the PER3 gene are predominantly associated with the occurrence of sleep disorders (26). SNPs rs228669 in PER3 gene are located in the exonic splicing enhancer (ESE) region, and the variant is synonymous. The SNP is located in the splice donor site and may alter the mRNA sequence, leading to either variations in protein structure or altered translation efficiency, thereby impacting the biological function of PER3. The rs228669 SNP affects cell metabolism and proliferation processes (27, 28). Our study identified a significant correlation between rs228669 and the response of premature infants to caffeine citrate, suggesting that the PER3 polymorphism may impact the efficacy of caffeine citrate treatment for AOP by altering circadian rhythms.
Due to the interconnection of proteins encoded by the various clock genes, the combination of polymorphisms in these genes may influence phenotype. GMDR analysis showed a statistically significant interaction between PER3 rs228669 and PER1 rs2585405 (P = 0.0107). PER1 rs2585405 is a missense variant, which changes the type and sequence of amino acids in the polypeptide chain. The rs2585405 missense variant is associated with susceptibility to noise-induced hearing loss and to prostate cancer in Chinese populations (29, 30). However, there have been no studies reporting how rs2585405 affects circadian rhythms. Future investigations may focus on the interaction between rs228669 and rs2585405 and how these impact circadian rhythms. In addition, the interactions among PER3 rs228669, PER1 rs2585405, and CRY2 rs1401419 also showed statistical significance (P = 0.0010). Premature infants carrying the rs228669 TC, rs2585405 CG, and rs1401419 TT had the highest rate of non-response to caffeine citrate. This observation suggested that while CRY didn't directly impact the caffeine citrate response in preterm infants, it might have interacted with the PER gene, and this interaction collectively determined the efficacy of caffeine citrate for AOP. The CRY1 and CRY2 genes are highly expressed in the SCN, and their mRNA levels show a cyclic oscillation pattern. The circadian period is shortened when the CRY1 gene is lacking, whereas it is lengthened when the CRY2 gene is lacking (31). Combined polymorphisms of the PER and CRY genes may alter the inhibitory effect of the PER-CRY heterodimers on the activity of CLOCK-BMAL1 heterodimers, thereby altering circadian rhythms and potentially influencing caffeine citrate treatment efficacy.
Our study has some limitations. Firstly, although we ensured the homogeneity of general characteristics of premature infants between the two groups through propensity score matching, other factors such as external stimuli, potential complications, and the NICU environment might also influence the response to caffeine citrate in preterm infants, we didn't take them into account. Secondly, we only studied a limited number of SNPs of the PER and CRY genes, the findings may not fully capture the genetic complexity of circadian genes involved in premature infants' response to caffeine citrate. Thirdly, the subjects of this study were limited to a single-center population in China, so the applicability of the study's conclusions to other populations may be limited. Finally, our study's findings need further external validation in independent cohort. In conclusion, our study found that PER and CRY gene polymorphisms were genetic factors influencing premature infants' response to caffeine citrate, suggesting that circadian rhythms may impact the efficacy of caffeine citrate therapy for AOP. While few investigations have been conducted on this subject, future studies may explore temporal variations for caffeine citrate based on this evidence, such as adjusting the timing and frequency of medication dosing according to circadian rhythms. Additionally, interventions to promote the maturation and stability of premature infants' circadian rhythms through appropriate external stimuli such as light, temperature, sound, and touch to enhance the efficacy of caffeine citrate treatment also merit investigation (32). Our future research will be conducted both at the in vivo and in vitro levels and aim to uncover the underlying mechanisms to further enhance the precision of caffeine citrate therapy for AOP.
The data presented in the study are deposited in the Figshare repository. The data is available here: https://doi.org/10.6084/m9.figshare.26304802
The studies involving humans were approved by The Ethics Committee of the Women and Children's Hospital, School of Medicine, Xiamen University. The studies were conducted in accordance with the local legislation and institutional requirements. Written informed consent for participation in this study was provided by the participants’ legal guardians/next of kin.
J-BX: Conceptualization, Data curation, Formal Analysis, Investigation, Methodology, Software, Writing – original draft. WZ: Formal Analysis, Methodology, Software, Validation, Writing – original draft. YZ: Conceptualization, Formal Analysis, Supervision, Writing – review & editing. ZZ: Project administration, Supervision, Writing – review & editing. Y-RH: Formal Analysis, Resources, Writing – review & editing. S-MM: Project administration, Resources, Writing – review & editing. X-ZL: Conceptualization, Funding acquisition, Project administration, Resources, Supervision, Writing – review & editing.
The author(s) declare financial support was received for the research, authorship, and/or publication of this article.
This work was supported by Xiamen Municipal Bureau of Science and Technology (3502Z20214ZD1225).
The authors declare that the research was conducted in the absence of any commercial or financial relationships that could be construed as a potential conflict of interest.
All claims expressed in this article are solely those of the authors and do not necessarily represent those of their affiliated organizations, or those of the publisher, the editors and the reviewers. Any product that may be evaluated in this article, or claim that may be made by its manufacturer, is not guaranteed or endorsed by the publisher.
The Supplementary Material for this article can be found online at: https://www.frontiersin.org/articles/10.3389/fped.2024.1414185/full#supplementary-material
1. Eichenwald EC, Committee on Fetus and Newborn, American Academy of Pediatrics. Apnea of prematurity. Pediatrics. (2016) 137(1):e20153757. doi: 10.1542/peds.2015-3757
2. Alhersh E, Abushanab D, Al-Shaibi S, Al-Badriyeh D. Caffeine for the treatment of apnea in the neonatal intensive care unit: a systematic overview of meta-analyses. Paediatr Drugs. (2020) 22(4):399–408. doi: 10.1007/s40272-020-00404-4
3. Kumral A, Tuzun F, Yesilirmak DC, Duman N, Ozkan H. Genetic basis of apnoea of prematurity and caffeine treatment response: role of adenosine receptor polymorphisms: genetic basis of apnoea of prematurity. Acta Paediatr. (2012) 101(7):e299–303. doi: 10.1111/j.1651-2227.2012.02664.x
4. He X, Qiu JC, Lu KY, Guo HL, Li L, Jia WW, et al. Therapy for apnoea of prematurity: a retrospective study on effects of standard dose and genetic variability on clinical response to caffeine citrate in Chinese preterm infants. Adv Ther. (2021) 38(1):607–26. doi: 10.1007/s12325-020-01544-2
5. Mokhtar WA, Fawzy A, Allam RM, Zidan N, Hamed MS. Association between adenosine receptor gene polymorphism and response to caffeine citrate treatment in apnea of prematurity; an Egyptian single-center study. Egypt Pediatr Assoc Gazette. (2018) 66(4):115–20. doi: 10.1016/j.epag.2018.09.001
6. Long JY, Guo HL, He X, Hu YH, Xia Y, Cheng R, et al. Caffeine for the pharmacological treatment of apnea of prematurity in the NICU: dose selection conundrum, therapeutic drug monitoring and genetic factors. Front Pharmacol. (2021) 12:681842. Published July 26, 2021. doi: 10.3389/fphar.2021.681842
7. Van Gilst D, Puchkina AV, Roelants JA, Kervezee L, Dudink J, Reiss IKM, et al. Effects of the neonatal intensive care environment on circadian health and development of preterm infants. Front Physiol. (2023) 14:1243162. Published August 31, 2023. doi: 10.3389/fphys.2023.1243162
8. Fagiani F, Di Marino D, Romagnoli A, Travelli C, Voltan D, Di Cesare Mannelli L, et al. Molecular regulations of circadian rhythm and implications for physiology and diseases. Signal Transduct Target Ther. (2022) 7(1):41. Published February 8, 2022. doi: 10.1038/s41392-022-00899-y
9. Dobrek L. Chronopharmacology in therapeutic drug monitoring-dependencies between the rhythmics of pharmacokinetic processes and drug concentration in blood. Pharmaceutics. (2021) 13(11):1915. Published November 12, 2021. doi: 10.3390/pharmaceutics13111915
10. Crnko S, Du Pré BC, Sluijter JPG, Van Laake LW. Circadian rhythms and the molecular clock in cardiovascular biology and disease. Nat Rev Cardiol. (2019) 16(7):437–47. doi: 10.1038/s41569-019-0167-4
11. Burke TM, Markwald RR, McHill AW, Chinoy ED, Snider JA, Bessman SC, et al. Effects of caffeine on the human circadian clock in vivo and in vitro. Sci Transl Med. (2015) 7(305):305ra146. doi: 10.1126/scitranslmed.aac5125
12. Supcun S, Kutz P, Pielemeier W, Roll C. Caffeine increases cerebral cortical activity in preterm infants. J Pediatr. (2010) 156(3):490–1. doi: 10.1016/j.jpeds.2009.10.033
13. Guo HL, Long JY, Hu YH, Liu Y, He X, Li L, et al. Caffeine therapy for apnea of prematurity: role of the circadian CLOCK gene polymorphism. Front Pharmacol. (2022) 12:724145. Published January 25, 2022. doi: 10.3389/fphar.2021.724145
14. Higgins RD, Jobe AH, Koso-Thomas M, Bancalari E, Viscardi RM, Hartert TV, et al. Bronchopulmonary dysplasia: executive summary of a workshop. J Pediatr. (2018) 197:300–8. doi: 10.1016/j.jpeds.2018.01.043
15. Bell MJ, Ternberg JL, Feigin RD, Keating JP, Marshall R, Barton L, et al. Neonatal necrotizing enterocolitis. Therapeutic decisions based upon clinical staging. Ann Surg. (1978) 187(1):1–7. doi: 10.1097/00000658-197801000-00001
16. Chiang MF, Quinn GE, Fielder AR, Ostmo SR, Paul Chan RV, Berrocal A, et al. International classification of retinopathy of prematurity, third edition. Ophthalmology. (2021) 128(10):e51–68. doi: 10.1016/j.ophtha.2021.05.031
17. Sweet DG, Carnielli VP, Greisen G, Hallman M, Klebermass-Schrehof K, Ozek E, et al. European consensus guidelines on the management of respiratory distress syndrome: 2022 update. Neonatology. (2023) 120(1):3–23. doi: 10.1159/000528914
18. Puopolo KM, Benitz WE, Zaoutis TE, Committee on Fetus and Newborn, Committee on Infectious Diseases. Management of neonates born at ≤34 6/7 weeks’ gestation with suspected or proven early-onset bacterial sepsis. Pediatrics. (2018) 142(6):e20182896. doi: 10.1542/peds.2018-2896
19. Papile LA, Burstein J, Burstein R, Koffler H. Incidence and evolution of subependymal and intraventricular hemorrhage: a study of infants with birth weights less than 1,500 gm. J Pediatr. (1978) 92(4):529–34. doi: 10.1016/s0022-3476(78)80282-0
20. Jha PK, Bouâouda H, Gourmelen S, Dumont S, Fuchs F, Goumon Y, et al. Sleep deprivation and caffeine treatment potentiate photic resetting of the master circadian clock in a diurnal rodent. J Neurosci. (2017) 37(16):4343–58. doi: 10.1523/JNEUROSCI.3241-16.2017
21. Weibel J, Lin YS, Landolt HP, Kistler J, Rehm S, Rentsch KM, et al. The impact of daily caffeine intake on nighttime sleep in young adult men. Sci Rep. (2021) 11(1):4668. Published February 25, 2021. doi: 10.1038/s41598-021-84088-x
22. Robillard R, Bouchard M, Cartier A, Nicolau L, Carrier J. Sleep is more sensitive to high doses of caffeine in the middle years of life. J Psychopharmacol. (2015) 29(6):688–97. doi: 10.1177/0269881115575535
23. Hughes S, Jagannath A, Hankins MW, Foster RG, Peirson SN. Photic regulation of clock systems. Methods Enzymol. (2015) 552:125–43. doi: 10.1016/bs.mie.2014.10.018
24. Ayyar VS, Sukumaran S. Circadian rhythms: influence on physiology, pharmacology, and therapeutic interventions. J Pharmacokinet Pharmacodyn. (2021) 48(3):321–38. doi: 10.1007/s10928-021-09751-2
25. Schuch JB, Genro JP, Bastos CR, Ghisleni G, Tovo-Rodrigues L. The role of CLOCK gene in psychiatric disorders: evidence from human and animal research. Am J Med Genet B Neuropsychiatr Genet. (2018) 177(2):181–98. doi: 10.1002/ajmg.b.32599
26. Hida A, Kitamura S, Katayose Y, Kato M, Ono H, Kadotani H, et al. Screening of clock gene polymorphisms demonstrates association of a PER3 polymorphism with morningness-eveningness preference and circadian rhythm sleep disorder. Sci Rep. (2014) 4:6309. Published September 9, 2014. doi: 10.1038/srep06309
27. Kovac U, Jasper EA, Smith CJ, Baer RJ, Bedell B, Donovan BM, et al. The association of polymorphisms in circadian clock and lipid metabolism genes with 2nd trimester lipid levels and preterm birth. Front Genet. (2019) 10:540. Published June 13, 2019. doi: 10.3389/fgene.2019.00540
28. Zhang Z, Ma F, Zhou F, Chen Y, Wang X, Zhang H, et al. Functional polymorphisms of circadian negative feedback regulation genes are associated with clinical outcome in hepatocellular carcinoma patients receiving radical resection. Med Oncol. (2014) 31(12):179. doi: 10.1007/s12032-014-0179-1
29. Chen H, Ding X, Ding E, Chen M, Wang H, Yang G, et al. A missense variant rs2585405 in clock gene PER1 is associated with the increased risk of noise-induced hearing loss in a Chinese occupational population. BMC Med Genomics. (2021) 14(1):221. Published September 8, 2021. doi: 10.1186/s12920-021-01075-x
30. Chu LW, Zhu Y, Yu K, Zheng T, Yu H, Zhang Y, et al. Variants in circadian genes and prostate cancer risk: a population-based study in China. Prostate Cancer Prostatic Dis. (2008) 11(4):342–8. doi: 10.1038/sj.pcan.4501024
31. Anand SN, Maywood ES, Chesham JE, Joynson G, Banks GT, Hastings MH, et al. Distinct and separable roles for endogenous CRY1 and CRY2 within the circadian molecular clockwork of the suprachiasmatic nucleus, as revealed by the Fbxl3(afh) mutation. J Neurosci. (2013) 33(17):7145–53. doi: 10.1523/JNEUROSCI.4950-12.2013
Keywords: apnea of prematurity, caffeine, gene polymorphism, circadian rhythm, PER gene, CRY gene
Citation: Xie J-B, Zhuang W, Zhu Y, Zheng Z, Huang Y-R, Ma S-M and Lin X-Z (2024) Association between PER and CRY gene polymorphisms and the response to caffeine citrate treatment in infants with apnea of prematurity. Front. Pediatr. 12: 1414185. doi: 10.3389/fped.2024.1414185
Received: 8 April 2024; Accepted: 4 July 2024;
Published: 22 July 2024.
Edited by:
Ted Steven Rosenkrantz, University of Connecticut, United StatesReviewed by:
Jonathan Michael Davis, Tufts University, United States© 2024 Xie, Zhuang, Zhu, Zheng, Huang, Ma and Lin. This is an open-access article distributed under the terms of the Creative Commons Attribution License (CC BY). The use, distribution or reproduction in other forums is permitted, provided the original author(s) and the copyright owner(s) are credited and that the original publication in this journal is cited, in accordance with accepted academic practice. No use, distribution or reproduction is permitted which does not comply with these terms.
*Correspondence: Xin-Zhu Lin, MTgyNTA4MDk2NzhAMTYzLmNvbQ==
†These authors have contributed equally to this work
Disclaimer: All claims expressed in this article are solely those of the authors and do not necessarily represent those of their affiliated organizations, or those of the publisher, the editors and the reviewers. Any product that may be evaluated in this article or claim that may be made by its manufacturer is not guaranteed or endorsed by the publisher.
Research integrity at Frontiers
Learn more about the work of our research integrity team to safeguard the quality of each article we publish.