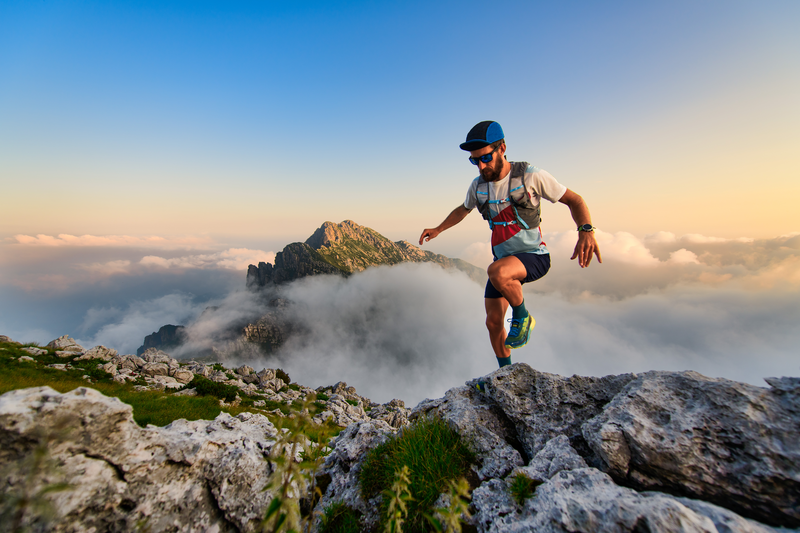
94% of researchers rate our articles as excellent or good
Learn more about the work of our research integrity team to safeguard the quality of each article we publish.
Find out more
ORIGINAL RESEARCH article
Front. Pediatr. , 15 August 2024
Sec. Pediatric Infectious Diseases
Volume 12 - 2024 | https://doi.org/10.3389/fped.2024.1393321
Background: Understanding the distinct immunologic responses to SARS-CoV-2 infection among pediatric populations is pivotal in navigating the COVID-19 pandemic and informing future public health strategies. This study aimed to identify factors associated with heightened antibody responses in children and adolescents to identify potential unique immune dynamics in this population.
Methods: Data collected between July and December 2023 from the Texas Coronavirus Antibody REsponse Survey (Texas CARES), a statewide prospective population-based antibody survey among 1-to-19-year-old participants, were analyzed. Each participant had the following data available for analysis: (1) Roche Elecsys® Anti-SARS-CoV-2 Immunoassay for Nucleocapsid protein antibodies (Roche N-test), (2) qualitative and semi-quantitative detection of antibodies to the SARS CoV-2 spike protein receptor binding domain (Roche S-test), and (3) self-reported antigen/PCR COVID-19 test results, vaccination, and health status. Statistical analysis identified associations between participant characteristics and spike antibody quartile group.
Results: The analytical sample consisted of 411 participants (mean age 12.2 years, 50.6% female). Spike antibody values ranged from a low of 6.3 U/ml in the lowest quartile to a maximum of 203,132.0 U/ml in the highest quartile in the aggregate sample. Older age at test date (OR = 1.22, 95% CI: 1.12, 1.35, p < .001) and vaccination status (primary series/partially vaccinated, one or multiple boosters) showed significantly higher odds of being in the highest spike antibody quartile compared to younger age and unvaccinated status. Conversely, fewer days since the last immunity challenge showed decreased odds (OR = 0.98, 95% CI: 0.96, 0.99, p = 0.002) of being in the highest spike antibody quartile vs. more days since last immunity challenge. Additionally, one out of every three COVID-19 infections were asymptomatic.
Conclusions: Older age, duration since the last immunity challenge (vaccine or infection), and vaccination status were associated with heightened spike antibody responses, highlighting the nuanced immune dynamics in the pediatric population. A significant proportion of children/adolescents continue to have asymptomatic infection, which has important public health implications.
Identifying distinct immunologic responses among children with SARS-CoV-2 infection is key to understanding multiple nuances of the COVID-19 pandemic. Pediatric patients are generally less symptomatic compared to adults, yet studies consistently show robust, long-lasting antibody responses to both infection and vaccination via nucleocapsid (N) and/or spike (S) antibodies, respectively, regardless of symptom or disease severity, lasting at least one year (1–3). Although some studies of adults have shown subsets of individuals who mount very high immune responses to vaccination or infection (4), or no response at all (5), this has not been widely investigated in children or adolescents. Understanding factors driving the variation in immune reactions in children is crucial for advancing pediatric immunology and shaping effective public health strategies as the pandemic to endemic transition continues (6).
SARS-CoV-2 infections in children typically lead to mild or asymptomatic cases, supported by studies indicating rare occurrences of severe acute infections, multisystem inflammatory syndrome in children (MIS-C), and long COVID (7–9). Current evidence points to various factors, such as innate immunity and local tissue responses, that are associated with a lower risk of severe disease in children (7, 8). Research has shown consistent, strong antibody responses in children, especially among those with hybrid immunity (acquired through both natural infection and vaccination) irrespective of symptom severity, symptomatic infection, age, sex, or body mass index (BMI) (10–15). Questions remain about what factors are associated with high to very high, or conversely, no immune response. Adult studies have shown younger age, female sex (6, 16), more vaccinations/boosters, fewer days since vaccination, absence of hypertension (6), and experiencing a breakthrough infection (17) are associated with higher immune response. Conversely, the presence of autoimmune disorders, diabetes and hypertension (18), kidney disease, being a smoker (19), being a transplant recipient (6), and race/ethnicity (20, 21) but not elevated BMI (22) showed a lower immune response.
Although underlying medical conditions have been shown to be important risk factors for COVID-19 disease in adult populations (23, 24), chronic diseases are relatively rare in pediatric populations, with the exception of obesity (25). Based on the current significant gaps in our knowledge regarding the factors influencing the variability in immune reactions to SARS-CoV-2 infection, immunization, or both (hybrid) among children, this analysis aimed to (1) identify factors associated with high SARS-CoV-2 spike and nucleocapsid antibody responses; and (2) explore the distribution of nucleocapsid antibody levels by spike antibody quartiles in children and adolescents. Based on the adult literature, it was hypothesized that (1) age, sex, vaccination history, and underlying medical conditions will contribute to spike antibody response variability; and (2) hybrid immunity will demonstrate the highest SARS-CoV-2 antibody responses.
The Texas Coronavirus Antibody REsponse Survey (CARES) is a prospective population-based seroprevalence program designed to assess the antibody status of individuals across Texas, a large and diverse population over time. Texas CARES includes participants spanning 0 to 90 years of age from the general population, with detailed study methods previously published (2–4). We report here results only from children and adolescents ages 1-to-19 years old who had at least one antibody test and one survey completed from July 2023 to December 2023. This collaborative initiative involves the University of Texas Health Science Center at Houston School of Public Health, the Texas Department of State Health Services (DSHS), the University of Texas System, and Clinical Pathology Laboratories (CPL), a network of over 200 statewide laboratory sites. All protocols were approved by the University of Texas Health Science Center's Committee for the Protection of Human Subjects and were endorsed as public health practice by the Texas Department of State Health Services' Institutional Review Board.
Statewide enrollment for Texas CARES began in October 2020. Families of potential pediatric participants received information through diverse channels, including healthcare providers, insurance carriers (for Medicaid-insured participants), media (radio, billboards), social media, school nurses and teachers, community events, and word-of-mouth. Information was disseminated in both English and Spanish to ensure inclusivity. This recruitment strategy aimed to engage a broad spectrum of potential participants, particularly those lacking access to conventional healthcare settings or awareness of other COVID-19 research studies. Collaboration with community partners further ensured accessibility across diverse backgrounds.
Interested volunteers were asked to visit a website (www.texascares.org) for more information and to participate. Parents or designated caregivers provided proxy informed consent for children and adolescents to join the Texas CARES study. Adolescents aged 12 years and above had the option to provide assent and complete the questionnaire, with none declining participation. Upon consenting to participate in Texas CARES, individuals completed a brief online questionnaire covering demographic information, employment status, baseline medical conditions, prior COVID-19 tests and diagnoses, physician-diagnosed COVID-19, height, weight, and other chronic illnesses. Following questionnaire completion, participants received orders to visit a CPL facility of their choice for the antibody status blood draw, typically receiving results within 48 h. The study team prioritized safety and convenience, allowing participants to select a laboratory facility convenient for them and providing information in both English and Spanish about the study and antibody test results.
Spike, or S, antibody response by quartile was considered the primary outcome variable of interest in the current analysis, while nucleocapsid, or N, antibody serostatus was also evaluated. The Roche Elecsys® Anti-SARS-CoV-2 Immunoassay (Roche N-test) was used to assess SARS-CoV-2 antibody status due to naturally acquired infection, utilizing a modified recombinant protein representing the nucleocapsid (N) antigen. This highly sensitive and specific assay, with a published sensitivity of 99.50% and specificity of 99.8%, detected high-affinity antibodies to SARS-CoV-2. A few months after the commencement of Texas CARES in 2020, the Roche S-test, an immunoassay detecting antibodies to the SARS-CoV-2 spike protein, was incorporated to monitor the combined impact of prior infection and COVID-19 vaccination. Both tests have a sensitivity and specificity exceeding 97% (26, 27).
Exposure to SARS-CoV-2 via natural infection or by vaccination, or both/hybrid, as well as age, body mass index, and chronic disease status, were primary exposures of interest. An online questionnaire, implemented in REDCap (27, 28) constituted Texas CARES' electronic questionnaire that captured information on infection and vaccination status was implemented at the time of each antibody test. Designed to take 10–15 min for parents or designated caregivers as proxy respondents, the questionnaire adapted questions from the COVID-19 PhenX Toolkit (29) and the Behavioral Risk Factor Surveillance System (30) questionnaires to enhance validity and reproducibility. US Census race/ethnicity questions were also replicated (31). The questionnaire seamlessly integrated with the informed consent process to streamline the respondent's experience and maximize survey completion.
Infection status was self-reported via diagnosis by a doctor, positive PCR test, or positive N-antibody test after symptoms and close contact with a positive case. Hospitalization and vaccination status were self-reported.
Weight categories were established based on calculated BMI using Centers for Disease Control and Prevention (CDC) age- and sex-adjusted BMI standardized values, categorizing participants into underweight (<5th percentile), healthy weight (≥5th to <85th percentile), overweight (≥85th to <95th percentile), and obesity (≥95th percentile) (32, 33).
Quartile analysis divided the main dataset into four equal groups by spike antibody levels and was used for all subsequent analyses. Specifically, the quartiles divide the main dataset into four intervals, each representing 25% of the total data. The first quartile corresponds to the 25th percentile, the second quartile represents the median and corresponds to the 50th percentile, and the third quartile corresponds to the 75th percentile of S antibody levels. Quartile analysis is particularly useful in understanding the spread of data and identifying potential skewness or outliers in a more granular manner compared to traditional summary statistics. Once quartiles were established, statistical approaches included Kruskal-Wallis rank sum tests, Pearson's Chi-squared tests, and Fisher's Exact Tests to compare pediatric participant characteristics (nucleocapsid antibody status, age at the test date, BMI, days since last reported infection or vaccine, reported infection status, and vaccination status) by S antibody quartile assignment. Odds ratios (OR) and 95% confidence intervals (CI) were calculated to examine if age at the test date, days since the last known immunity challenge (in tens), and vaccination status categories (Unvaccinated, Primary Series/Partially Vaccinated, One Booster, Multiple Boosters) were significant predictors of fourth quartile membership via logistic regression analyses. The statistical significance of all associations was determined using p-values, with a significance level set at 0.05. All statistical analyses were performed using RStudio.
Table 1 presents the distribution of SARS-CoV-2 spike antibody quartiles among TX Cares pediatric participants (N = 411, mean age at test date 12.2 years, 50.6% female). Among the aggregate sample, spike values ranged from a low of 6.3 U/ml in the first quartile to a maximum of 203,132.0 U/ml in the fourth quartile. Nucleocapsid antibody mean (SD) levels were lower overall in the highest quartile vs. the third, second and first (86.2 (83.6), 98.0 (92.5), 98.3 (93.1), and 68.7 (77.6) U/ml, respectively) as well as median levels (52.2, 72.1, 76.8, and 28.4 U/ml, respectively but these differences were not statistically significant. The first quartile (N = 103) had a minimum spike-value of 6.3 U/ml and a maximum of 2,633.0 U/ml, while the fourth quartile (N = 103) ranged from 22,730.0 U/ml to 203,132.0 U/ml. Spike antibody level was significantly associated with age at the test date (p < 0.001), with the mean spike-value increasing with age. Similarly, categorical age analysis revealed a significant difference across age groups, with the highest quartile more prevalent in the 15–19 years old group (50.2%, p = 0.004). In general, participants with longer durations since last reported infection (mean 626.6 days) tended to fall in first quartile compared to those in the second (mean 439.5 days), third (mean 431.0 days) and fourth (mean 465.5 days) quartiles, respectively, p = 0.034). As expected, similar patterns were observed for days since last vaccine (p = 0.001) and days since last immunity challenge (p < 0.001). Similarly, vaccination status showed a trend of higher quartiles corresponding to increased vaccination: the first quartile comprised of 86.4% who were unvaccinated, while the fourth quartile had 95% who had at least one vaccine (p < 0.001).
Table 1. SARS-CoV-2 spike (S) antibody quartiles by pediatric participant characteristics, Texas CARES 2020–2023 (N = 411).
Table 2 presents spike antibody quartiles among participants who reported at least one infection only. Overall results were similar to the aggregate sample findings; unvaccinated participants (38.5%) exhibit lower spike antibody levels compared to those with varying degrees of vaccination, including primary series/partially vaccinated (31.7%), one booster (13.1%), and multiple boosters (16.7%) (p < 0.001). Additionally, a significant association was shown between spike antibody quartile and the duration since the last reported infection, with decreasing mean values from 626.6 days (first quartile) to 382.9 days (fourth quartile) (p = 0.034). Age, place of care, and the number of reported infections, showed no significant associations with spike antibody quartiles.
Table 2. SARS-CoV-2 spike (S) antibody quartiles by pediatric participant characteristics with at least one infection, Texas CARES 2020–2023.
Among those who reported no infections, the nucleocapsid-values exhibit considerable variability across quartiles, with mean positive values of 45 (88.2), 32 (66.7), 29 (70.7), and 40 (80.0) for the first through fourth quartiles, respectively (p = 0.049) (Table 3).
Table 3. N antibody characteristics among those reporting no infections by S antibody quartile, Texas CARES pediatric population.
Age at the test date was associated with higher odds (OR = 1.22, 95% CI: 1.12, 1.35, p < .001) of being in the fourth spike antibody quartile, whereas days since the last immunity challenge, represented in tens, was negatively associated (OR = 0.98, 95% CI: 0.96, 0.99, p = 0.002). Vaccination status showed significant associations, with primary series/partially vaccinated (OR = 13.62, 95% CI: 2.42, 261.49, p = 0.016), one booster (OR = 21.33, 95% CI: 3.71, 409.32, p = 0.005), and multiple boosters (OR = 40.77, 95% CI: 7.11, 778.11, p < .001) showing substantially higher odds of being in the fourth spike antibody quartile compared to those who were not vaccinated (Table 4).
Table 4. Odds ratios and 95% confidence intervals of fourth quartile S values, Texas CARES pediatric participants.
Figure 1 shows the variability in mean nucleocapsid antibody levels by spike antibody quartile. Mean N antibody levels were almost the same for the second (98.0 U/ml) and third (98.3 U/ml) quartiles, and slightly lower in the first quartile (86.2 U/ml) and lowest in the fourth quartile (68.7 U/ml), but these differences were not significant.
Figure 1. Box plot of nucleocapsid antibody levels by spike antibody quartile, Texas CARES pediatric participants (n = 411).
The findings from this study provide a comprehensive overview of SARS-CoV-2 spike (S) antibody responses among TX Cares pediatric participants with several key findings. First, spike antibody levels varied widely across quartiles, ranging from a low value of 6.3 U/ml in the first quartile to a high of well over 200,000 U/ml in the fourth quartile. All pediatric participants showed evidence of some spike antibodies but there were children represented with no nucleocapsid antibodies in all four quartiles. Second, vaccine and infection status, and days since last immunity challenge, were key drivers in elevated immune response in children and adolescents. And third, the age at antibody test date emerged as a significant factor, with the highest spike antibody quartile more prevalent in the 15-to-19 years old (oldest) age group vs. younger ages. It should also be noted that anywhere from 28.4% in the third quartile to 43.7% in the first spike antibody quartile had positive N antibodies but reported no infection reflecting that roughly one out of every three COVID-19 infections in children and adolescents are asymptomatic. Finally, while it was found that the highest spike antibody quartile also had the lowest mean (68.7 vs. 98.3, 98.0 and 86.2 U/ml, respectively) and median (28.4 vs. 76.8, 72.1, and 52.2 U/ml, respectively) nucleocapsid antibody levels vs. the third, second and first quartiles, respectively none of the tested differences (overall and pairwise) were significant. Overall, these findings highlight the diverse spike and nucleocapsid antibody characteristics in pediatric participants both with and without natural infection(s) and vaccinations, align with recent findings on the robust mRNA vaccine responses in children (11). However, they also raise intriguing questions about why naturally-induced nucleocapsid antibody levels may be lowest among those with the highest spike values.
Variability in immune responses, evidenced by widely differing S-values across quartiles, highlights the complexity of the pediatric immune response (12). As expected, associations between spike antibody levels and the duration since the last reported infection, vaccine, or immunity challenge were found. Specifically, longer duration since last immunity challenge was associated with lower quartiles, underscoring the impact of time on antibody duration. This finding is in agreement with other studies (3), that show robust durability of nucleocapsid and spike antibodies in a large pediatric sample up to 12 months post-infection/vaccination (1–3). However, these studies, including a systematic review of 24 seroprevalence papers (1) were from the pre-Omicron and Omicron eras. To our knowledge, this is one of the first, if not the only analysis currently available in the literature that includes the new, and dominant, JN.1. variant as of January 5, 2024.
Vaccination status independently played a pivotal role in driving the highest spike antibody response, with a clear shift towards higher quartiles corresponding to increased vaccine doses. These findings were expected, as those with more complete vaccination series, including boosters, showed substantially higher odds of being in the fourth spike antibody quartile. These findings are consistent with the literature, and crucial for understanding immune response longevity as it pertains to pediatric populations (13, 34). It should also be noted that 35.5% of the aggregate sample remained unvaccinated.
Another key finding was the significant association of older age and higher spike antibody levels. The mean age increased from 11.2 years in the first quartile to 13.7 years in the fourth quartile, demonstrating a clear age-dependent trend in spike antibody responses. Categorically, the prevalence of the highest quartile was notably higher in the 15-to-19 years old group (50.5%), compared to the other age categories. These findings may be the result of vaccination only being available to older children and adolescents early in the pandemic and hesitancy of parents to vaccinate very young children. These findings are consistent with other literature demonstrating that age is positively correlated with spike protein antibody response among children and young adults (13).
Our results did not show any significant associations between BMI group and chronic conditions with S antibody quartiles. These finding are noteworthy, as it suggests that the immune responses to SARS-CoV-2 in pediatric individuals may not be significantly compromised by the presence of chronic health conditions, including obesity in the same way that it might be in certain adult populations. Indeed, adult studies show that comorbidities may enhance humoral response to SARS-CoV-2 due to increased pro-inflammatory states (14) while other studies show the presence of chronic conditions (autoimmune disorders, diabetes and kidney disease) (18) showed lower immune response.
Results showed that a substantial proportion (28.4%–43.7% across quartiles) of participants with positive N antibodies reported no COVID-19 infection(s). Conversely, only 1.0%–2.9% with negative N antibodies reported a previous infection. These results suggest that a significant number of children and adolescents continue to experience asymptomatic, and thus unreported COVID-19 infections, which has been an important hallmark of the COVID-19 pandemic since its inception (35).
Collectively, these findings are informative for pandemic science and public health. Specifically, findings here underscore the importance of understanding pediatric immune responses for developing effective vaccination strategies and managing reinfection risks and long-term health outcomes. Trends in both spike and nucleocapsid antibody levels, age-dependence, and vaccination status form a basis for targeted health interventions, especially in pediatric healthcare and vaccination efforts.
This study is not without limitations, notably, the reliance on self-reported data, which may introduce recall bias. Second, all COVID-19 disease and vaccination data were self-reported. Third, Texas CARES participants may be different in terms of awareness, willingness, and ability to participate vs. the overall state population on important social determinants of health (e.g., education, socioeconomic level, language other than English or Spanish), resulting in selection bias. Despite this, Texas CARES offers valuable insights into both the spike and nucleocapsid antibody response to SARS-CoV-2, supported by its robust recruitment strategy, sensitive antibody assays from Roche Diagnostics, and a comprehensive electronic questionnaire.
In summary, this study identified factors associated with heightened antibody responses in children and adolescents to identify potential unique immune dynamics in this population. Findings highlight considerable variability in S antibody levels across quartiles, with intriguing patterns in the relationship between S and N antibody levels. Older age, less days since last immunity challenge and vaccination status were associated with heightened S antibody immune response in children and adolescents. Notably, a significant percentage of participants with positive N antibodies reported no infection, underscoring the continued prevalence of asymptomatic cases in pediatric COVID-19 infections. Overall, these results contribute to our understanding of antibody characteristics in pediatric populations, aligning with broader trends observed in recent studies on vaccine responses in children.
Texas CARES investigators are committed to data sharing. Granular results and user-specified data summaries are currently publicly available on the Texas CARES portal (https://sph.uth.edu/projects/texascares/dashboard). After project completion, de-identified secondary data and detailed documentation will be made publicly available through the same portal as open-access data sources after de-identification and collapsing cells to protect individuals representing rare events or conditions. De-identified datasets will be made available upon reasonable request and within a reasonable time in accordance with the general spirit of colleagueship within the scientific community and with policies adopted by UTHealth Houston and the Texas Department of State Health Services. Please submit data requests toaW5zdGl0dXRpb25hbHJldmlld2JvYXJkQGRzaHMudGV4YXMuZ292.
The studies involving humans were approved by University of Texas Health Science Center's Committee for the Protection of Human Subjects. The studies were conducted in accordance with the local legislation and institutional requirements. Written informed consent for participation in this study was provided by the participants’ legal guardians/next of kin.
SM: Conceptualization, Investigation, Methodology, Resources, Supervision, Validation, Writing – review & editing. RA: Data curation, Formal Analysis, Writing – review & editing, Validation. EBe: Resources, Writing – original draft. HK: Investigation, Methodology, Validation, Writing – original draft, Writing – review & editing. MSw: Conceptualization, Data curation, Formal Analysis, Investigation, Methodology, Supervision, Validation, Writing – review & editing. YT: Conceptualization, Data curation, Formal Analysis, Visualization, Writing – review & editing. RS: Data curation, Methodology, Validation, Visualization, Writing – review & editing. HH: Data curation, Methodology, Resources, Validation, Writing – review & editing. MV-S: Supervision, Writing – review & editing, Conceptualization, Investigation, Methodology, Project administration, Resources. SD: Data curation, Formal Analysis, Validation, Writing – review & editing. AY: Data curation, Visualization, Writing – review & editing. HG: Data curation, Writing – review & editing. XA: Data curation, Project administration, Visualization, Writing – review & editing. JR: Project administration, Resources, Supervision, Writing – review & editing. LP: Project administration, Resources, Supervision, Writing – review & editing. MG: Data curation, Software, Visualization, Writing – review & editing. LW: Data curation, Software, Visualization, Writing – review & editing. MSi: Investigation, Writing – review & editing. DL: Investigation, Methodology, Resources, Writing – review & editing. JS: Investigation, Methodology, Project administration, Resources, Validation, Writing – review & editing. SP: Writing – review & editing. EBo: Conceptualization, Data curation, Formal Analysis, Funding acquisition, Investigation, Methodology, Project administration, Resources, Software, Supervision, Validation, Visualization, Writing – review & editing.
The author(s) declare financial support was received for the research, authorship, and/or publication of this article. Texas Department of State Health Services (Contract #HHS000866600001).
This work was supported by the Texas Department of State Health Services and the University of Texas System. This analysis would not have been possible without the partnership of many. The TX CARES investigation team would like to thank Children's Health System of Texas, Dallas, TX; Cook Children's Forth Worth, TX; Covenant Health, Lubbock, TX; Driscoll Children's, Corpus Christi, TX; El Paso Children's, El Paso, TX; UTHealth McGovern, Houston, TX; UTHealthRGV, Rio Grande Valley, TX; UTHealth Tyler, Tyler, TX; Ascension Health, Privia Health, Superior Health Plan, TX Association of Family Physicians, TX Medical Association, TX Pediatric Society, and Federally Qualified Health Care Centers statewide, for assisting with sharing information with families about this survey.
The authors declare that the research was conducted in the absence of any commercial or financial relationships that could be construed as a potential conflict of interest.
All claims expressed in this article are solely those of the authors and do not necessarily represent those of their affiliated organizations, or those of the publisher, the editors and the reviewers. Any product that may be evaluated in this article, or claim that may be made by its manufacturer, is not guaranteed or endorsed by the publisher.
1. Buonsenso D, Cusenza F, Passadore L, Bonanno F, De Guido C, Esposito S. Duration of immunity to SARS-CoV-2 in children after natural infection or vaccination in the omicron and pre-omicron era: a systematic review of clinical and immunological studies. Front Immunol. (2023) 13:1024924. doi: 10.3389/fimmu.2022.1024924
2. Messiah SE, DeSantis SM, Leon-Novelo LG, Talebi Y, Brito FA, Kohl HW, et al. Durability of SARS-CoV-2 antibodies from natural infection in children and adolescents. Pediatrics. (2022) 149(6):e2021055505. doi: 10.1542/peds.2021-055505
3. Messiah SE, Talebi Y, Swartz MD, Sabharwal R, Han H, Bergqvist E, et al. Long-term immune response to SARS-CoV-2 infection and vaccination in children and adolescents. Pediatr Res. (2023). doi: 10.1038/s41390-023-02857-y
4. Shrotri M, van Schalkwyk MCI, Post N, Eddy D, Huntley C, Leeman D, et al. T cell response to SARS-CoV-2 infection in humans: a systematic review. PLoS One. (2021) 16(1):e0245532. doi: 10.1371/journal.pone.0245532
5. Arkhipova-Jenkins I, Helfand M, Armstrong C, Gean E, Anderson J, Paynter RA, et al. Antibody response after SARS-CoV-2 infection and implications for immunity: a rapid living review. Ann Intern Med. (2021) 174(6):811–21. doi: 10.7326/M20-7547
6. Ebinger JE, Joung S, Liu Y, Wu M, Weber B, Claggett B, et al. Demographic and clinical characteristics associated with variations in antibody response to BNT162b2 COVID-19 vaccination among healthcare workers at an academic medical centre: a longitudinal cohort analysis. BMJ Open. (2022) 12(5):e059994. doi: 10.1136/bmjopen-2021-059994
7. Buonsenso D, Munblit D, Pazukhina E, Ricchiuto A, Sinatti D, Zona M, et al. Post-COVID condition in adults and children living in the same household in Italy: a prospective cohort study using the ISARIC global follow-up protocol. Front Pediatr. (2022) 10:834875. doi: 10.3389/fped.2022.834875
8. Centers for Disease Control and Prevention. Long COVID in Children: United States 2022. National Center for Health Statistics. Available online at: https://www.cdc.gov/nchs/products/databriefs/db479.htm (Accessed December 6, 2023).
9. Messiah SE, Hao T, DeSantis SM, Swartz MD, Talebi Y, Kohl HW 3rd, et al. Comparison of persistent symptoms following SARS-CoV-2 infection by antibody status in nonhospitalized children and adolescents. Pediatr Infect Dis J. (2022) 41(10):e409–17. doi: 10.1097/INF.0000000000003653
10. Chou J, Thomas PG, Randolph AG. Immunology of SARS-CoV-2 infection in children. Nat Immunol. (2022) 23:177–85. doi: 10.1038/s41590-021-01123-9
11. Kodde C, Tafelski S, Balamitsa E, Nachtigall I, Bonsignore M. Factors influencing antibody response to SARS-CoV-2 vaccination. Vaccines (Basel). (2023) 11(2):451. doi: 10.3390/vaccines11020451
12. Bartsch YC, St Denis KJ, Kaplonek P, Kang J, Lam EC, Burns MD, et al. SARS-CoV-2 mRNA vaccination elicits robust antibody responses in children. Sci Transl Med. (2022) 14(672):eabn9237. doi: 10.1126/scitranslmed.abn9237
13. Yang HS, Costa V, Racine-Brzostek SE, Acker KP, Yee J, Chen Z, et al. Association of age with SARS-CoV-2 antibody response. JAMA Netw Open. (2021) 4(3):e214302. doi: 10.1001/jamanetworkopen.2021.4302
14. Costagliola G, Spada E, Consolini R. Age-related differences in the immune response could contribute to determine the spectrum of severity of COVID-19. Immun Inflamm Dis. (2021) 9(2):331–9. doi: 10.1002/iid3.404
15. Kreutmair S, Kauffmann M, Unger S, Ingelfinger F, Núñez NG, Alberti C, et al. Preexisting comorbidities shape the immune response associated with severe COVID-19. J Allergy Clin Immunol. (2022) 150(2):312–24. doi: 10.1016/j.jaci.2022.05.019
16. Sabetta E, Noviello M, Sciorati C, Viganò M, De Lorenzo R, Beretta V, et al. A longitudinal analysis of humoral, T cellular response and influencing factors in a cohort of healthcare workers: implications for personalized SARS-CoV-2 vaccination strategies. Front Immunol. (2023) 14:1130802. doi: 10.3389/fimmu.2023.1130802
17. Das D, Raha FK, Adnan KM, Siraj MR, Shapla MJ, Shumy F, et al. Dynamic antibody response in SARS-CoV-2 infected patients and COVID-19 vaccine recipients alongside vaccine effectiveness in comorbid and multimorbid groups. Heliyon. (2023) 9(5):e16349. doi: 10.1016/j.heliyon.2023.e16349
18. Kung YA, Huang SY, Huang CG, Liu KT, Huang PN, Yu KY, et al. Factors influencing neutralizing antibody titers elicited by coronavirus disease 2019 vaccines. Microbes Infect. (2023) 25(1-2):105044. doi: 10.1016/j.micinf.2022.105044
19. Petersen MS, Pérez-Alós L, Armenteros JJA, Hansen CB, Fjallsbak JP, Larsen S, et al. Factors influencing the immune response over 15 months after SARS-CoV-2 infection: a longitudinal population-wide study in the Faroe Islands. J Intern Med. (2023) 293(1):63–81. doi: 10.1111/joim.13560
20. Reusch J, Wagenhäuser I, Gabel A, Eggestein A, Höhn A, Lâm TT, et al. Influencing factors of anti-SARS-CoV-2-spike-IgG antibody titers in healthcare workers: a cross-section study. J Med Virol. (2023) 95(1):e28300. doi: 10.1002/jmv.28300
21. Ahluwalia P, Vashisht A, Singh H, Sahajpal NS, Mondal AK, Jones K, et al. Ethno-demographic disparities in humoral responses to the COVID-19 vaccine among healthcare workers. J Med Virol. (2023) 95(9):e29067. doi: 10.1002/jmv.29067
22. Nilles EJ, Siddiqui SM, Fischinger S, Bartsch YC, de St Aubin M, Zhou G, et al. Epidemiological and immunological features of obesity and SARS-CoV-2. Viruses. (2021) 13(11):2235. doi: 10.3390/v13112235
23. Elezkurtaj S, Greuel S, Ihlow J, Michaelis EG, Bischoff P, Kunze CA, et al. Causes of death and comorbidities in hospitalized patients with COVID-19. Sci Rep. (2021) 11(1):4263. doi: 10.1038/s41598-021-82862-5
24. Sanyaolu A, Okorie C, Marinkovic A, Patidar R, Younis K, Desai P, et al. Comorbidity and its impact on patients with COVID-19. SN Compr Clin Med. (2020) 2(8):1069–76. doi: 10.1007/s42399-020-00363-4
25. Stierman B, Afful J, Carroll MD, Chen T-C, Davy O, Fink S, et al. National Health and Nutrition Examination Survey 2017–March 2020 Prepandemic Data Files Development of Files and Prevalence Estimates for Selected Health Outcomes. Published Date: 06/14/2021; Series: NHSR No. 158; Source: National Health Statistics Reports. Available online at: https://stacks.cdc.gov/view/cdc/106273 (Accessed July 26, 2024).
26. Roche. Elecsys® Anti-SARS-CoV-2. Package Insert 2020-07, V9.0; Material Numbers 09203095190 and 09203079190. US Food and Drug Administration. Available online at: https://www.fda.gov/media/137605/download (Accessed December, 2023).
27. Roche. Elecsys® Anti-SARS-CoV-2 S. Package Insert 2020-12, V1.0; Material Numbers 09289267190 and 09289275190. US Food and Drug Administration. Available online at: https://www.fda.gov/media/144037/download (Accessed December, 2023).
28. Harris PA, Taylor R, Thielke R, Payne J, Gonzalez N, Conde JG, et al. Research electronic data capture (REDCap)—a metadata-driven methodology and workflow process for providing translational research informatics support. J Biomed Inform. (2009) 42(2):377–81. doi: 10.1016/j.jbi.2008.08.010
29. Harris PA, Taylor R, Minor BL, Elliott V, Fernandez M, O'Neal L, McLeod L, et al. The REDCap consortium: building an international community of software platform partners. J Biomed Inform. (2019) 95:103208. doi: 10.1016/j.jbi.2019.103208
30. PhenX toolkit. Available online at: https://www.phenxtoolkit.org/covid19 (Accessed December, 2023).
31. Centers for Disease Control and Prevention. National Center for Chronic Disease Prevention and Health Promotion. Behavioral Risk Factor Surveillance System. Available online at: https://www.healthypeople.gov/2020/data-source/behavioral-risk-factorsurveillance-system (Accessed December, 2023).
32. Kuczmarski RJ, Ogden CL, Guo SS, Grummer-Strawn LM, Flegal KM, Mei Z, et al. 2000 CDC growth charts for the United States: methods and development. Vital Health Stat. (2002) 11(246):1–190. PMID: 12043359
33. Centers for Disease Control and Prevention. About child and teen BMI. Available online at: https://www.cdc.gov/healthyweight/assessing/bmi/childrens_bmi/about_childrens_bmi.html#HowIsBMICalculated (Accessed February, 2023).
34. Kim M, Cheng WA, Congrave-Wilson Z, Ruiz CJM, Turner L, Mendieta S, et al. Comparisons of pediatric and adult SARS-CoV-2-specific antibodies up to 6 months after infection, vaccination, or hybrid immunity. J Pediatr Infect Dis Soc. (2024) 13(1):91–9. doi: 10.1093/jpids/piad107
Keywords: SARS-CoV-2, children, adolescents, immune response, epidemiology
Citation: Messiah SE, Abbas R, Bergqvist E, Kohl III HW, Swartz MD, Talebi Y, Sabharwal R, Han H, Valerio-Shewmaker MA, DeSantis SM, Yaseen A, Gandhi HA, Amavisca XF, Ross JA, Padilla LN, Gonzalez MO, Wu L, Silberman MA, Lakey D, Shuford JA, Pont SJ and Boerwinkle E (2024) Factors associated with elevated SARS-CoV-2 immune response in children and adolescents. Front. Pediatr. 12:1393321. doi: 10.3389/fped.2024.1393321
Received: 28 February 2024; Accepted: 22 July 2024;
Published: 15 August 2024.
Edited by:
Kin Israel Notarte, Johns Hopkins University, United StatesReviewed by:
Janet R. Hume, University of Minnesota Medical Center, United StatesCopyright: © 2024 Messiah, Abbas, Bergqvist, Kohl, Swartz, Talebi, Sabharwal, Han, Valerio-Shewmaker, DeSantis, Yaseen, Gandhi, Amavisca, Ross, Padilla, Gonzalez, Wu, Silberman, Lakey, Shuford, Pont and Boerwinkle. This is an open-access article distributed under the terms of the Creative Commons Attribution License (CC BY). The use, distribution or reproduction in other forums is permitted, provided the original author(s) and the copyright owner(s) are credited and that the original publication in this journal is cited, in accordance with accepted academic practice. No use, distribution or reproduction is permitted which does not comply with these terms.
*Correspondence: Sarah E. Messiah, c2FyYWguZS5tZXNzaWFoQHV0aC50bWMuZWR1
Disclaimer: All claims expressed in this article are solely those of the authors and do not necessarily represent those of their affiliated organizations, or those of the publisher, the editors and the reviewers. Any product that may be evaluated in this article or claim that may be made by its manufacturer is not guaranteed or endorsed by the publisher.
Research integrity at Frontiers
Learn more about the work of our research integrity team to safeguard the quality of each article we publish.