- Department of Pediatrics, Social Welfare Aiseikai, Suihoen, Japan
Mammalian target of rapamycin inhibitors (mTORi) have been used to treat pediatric tuberous sclerosis complex (TSC)-associated tumors, particularly in cases with contraindications to surgery or difficulties in complete tumor resection. However, some patients experience side effects and tumor regression after discontinuation of the treatment. Therefore, there is an urgent need to develop drugs that can be used in combination with mTORi to increase their efficacy and minimize their side effects. 1,25-Dihydroxyvitamin D3 (1,25-D), which has anticancer properties, may be a promising candidate for adjuvant or alternative therapy because TSC and cancer cells share common mechanisms, including angiogenesis, cell growth, and proliferation. Vitamin D receptor-mediated signaling can be epigenetically modified and plays an important role in susceptibility to 1,25-D. Therefore, vitamin D signaling may be a promising drug target, and in vitro studies are required to evaluate the efficacy of 1,25-D in TSC-associated tumors, brain development, and core symptoms of psychiatric disorders.
1 Introduction
Tuberous sclerosis complex (TSC) is an autosomal dominant disorder caused by inactivating mutations in either the TSC1 or TSC2 gene, which affects multiple organ systems and results in various clinical features (1). TSC1 encodes hamartin and TSC2 encodes tuberin. Since TSC1 and TSC2 are tumor suppressor genes, abnormalities in hamartin or tuberin lead to mammalian target of rapamycin (mTOR) overactivation and multisystemic cellular proliferation, migration, and differentiation abnormalities (1). The role of mTOR inhibitors (mTORi) in cancer (2) and their safety in TSC (3) have been established. However, for children with TSC-associated tumors, it is critical to have an alternative therapeutic option when mTORi are ineffective or cannot be used.
1,25-Dihydroxyvitamin D3 (1,25-D) activates DNA damage-inducible transcript 4 (DDIT4) (4), which activates the TSC1-TSC2 complex and ultimately represses mTOR (5). Daily vitamin D supplementation was shown to reduce overall cancer mortality (6). An association has been suggested between cancer and low levels of circulating 25-hydroxyvitamin D3 (25-D) in ovarian (7), prostate (8), and colorectal cancers (9). However, whether vitamin D can prevent cancer remains controversial. Therefore, it is important to investigate whether 1,25-D not only serves as an adjuvant therapy by inhibiting or reducing TSC-associated tumor cell proliferation but also improves mTORi tolerability.
In addition to its effects on mTOR suppression, 1,25-D negatively regulates energy metabolism in cancer cells, including glucose and lipid metabolism, protection from oxidative stress, and cancer progression (10). Higher serum 25-D levels are associated with a reduced risk of glioma in elderly men (11). Recent results from an in vitro study have led to a discussion on the potential clinical use of vitamin D for treating glioblastoma (12). Thus, 1,25-D may be a weak suppressor of TSC-associated tumor growth, including in cases of drug resistance and rapidly growing subependymal giant cell astrocytoma (SEGA). This article provides perspectives on the potential adjuvant therapy using 1,25-D in patients with TSC, along with a review and presentation of hypotheses associated with the underlying physiological mechanisms.
2 Need for an alternative or supportive drug to mTORi in pediatric TSC
In pediatric patients with TSC, SEGA (in ages ≥1 year) and refractory partial-onset seizures (as an adjunctive treatment, in ages ≥2 years) can be treated with mTORi (13). However, side effects are more common in children than in adults (13). The overall incidence of adverse events in children aged <9 years was 70.5% (24 of 34 patients), of which 33.3% (8 of 24 patients) had grade 3 side effects (13). Moreover, the mechanism of mTOR resistance in each tumor type has not been elucidated yet. Therefore, in addition to investigating the resistance mechanism, there is an urgent need to identify safe and effective drugs that can support mTORi treatment, including drug repurposing and combination therapy.
3 1,25-D inhibits vascular endothelial growth factor (VEGF) production and early angiogenesis in TSC-associated tumors
1,25 D causes transcriptional changes by binding to the intracellular vitamin D receptor (VDR). This binding forms a complex that interacts with specific DNA sequences called vitamin D-response elements (VDREs) located within the promoter regions of target genes (14).
1,25-D activates the production of the DDIT4 protein, which is induced by hypoxia and DNA damage via intracellular VDR (5). The DDIT4 inhibits mTOR complex 1 by promoting TSC1-TSC2 complex formation (15) (Figure 1A). Moreover, the DDIT4-TSC1/TSC2-mTOR feedback loop downregulates the production of hypoxia-inducible factor-1α (HIF-1α) and VEGF (Vascular Endothelial Growth Factor) (15). siRNA knockdown of DDIT4 eliminates the antiproliferative effect of 1,25-D (16). Thus, 1,25-D restricts HIF-1-dependent VEGF production in various human cancer cells under hypoxic conditions (17) and induces apoptosis in existing sprouted and elongated endothelial cells (18) Notably, TSC-associated tumors are angiogenic neoplasms (19) expressing high levels of VEGF (20). Through this mechanism, 1,25-D may suppress tumor cell proliferation by inhibiting the excessive activation of the HIF/VEGF pathway in the vasculature of TSC-associated tumors.
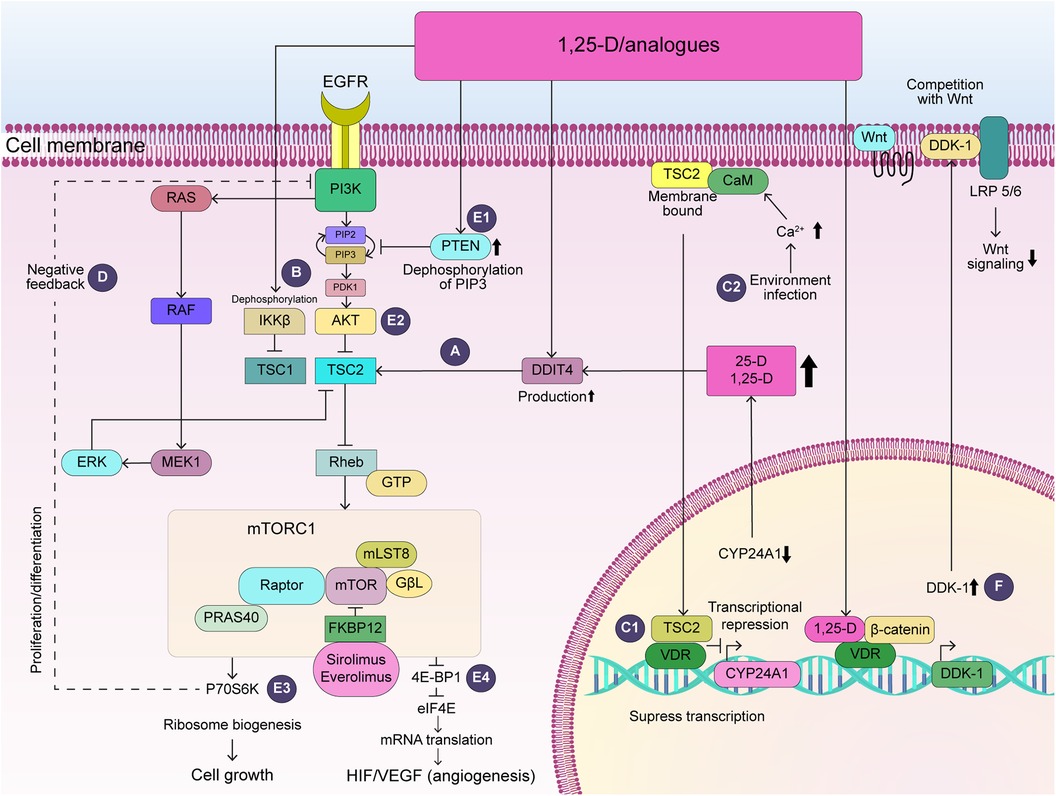
Figure 1. Potential action of vitamin D in TSC-associated tumors. (A) DDIT4 activates TSC2, which inhibits Rheb and ultimately suppresses mTOR activity. (B) 1,25-D dephosphorylates and inactivates IKKβ, which inhibits TSC1. (C1) TSC2-CaM complex translocates into the nucleus and represses CYP24A1 transcription. (C2) Increased intracellular calcium, which activates CaM by environmental factors including viral infection, could increase attenuation of transcription of VDR-sensitive genes. (D) S6K activation suppresses the PI3K/AKT and ERK pathway via negative feedback. (E) 1,25-D activates the production of PTEN (E1), which inhibits PI3K, leading to the dephosphorylation of Akt (E2), ultimately reducing p70S6K activity (E3) and 4E-BP1 (E4). Thus, 1,25-D may contribute to the suppression of cell growth and angiogenesis in TSC-associated tumors. (F) 1,25-D increases VDR/β-catenin binding, which increases transcription of the VDR-sensitive gene, DDK-1, competing with Wnt and decreasing Wnt signaling. AKT, RAC-alpha serine/threonine-protein kinase; CaM, calcium-calmodulin; CYP24A1, 24-hydroxylase; 1,25-D, 1,25-dihydroxyvitamin D3; DDK-1, Dickkopf-1; DDIT4, DNA damage inducible transcript 4; Deptor, DEP-domain-containing mTOR-interacting protein; 4E-BP1, eukaryotic initiation factor 4E-binding protein 1; eIF4E, eukaryotic initiation factor 4E; EGFR, epidermal growth factor receptor; ERK1-2, extracellular signal-related kinase; FKBP12, FK506-binding protein with a molecular weight of 12 kDa; GβL, G protein β subunit-like protein; GTP, guanosine triphosphate; HIF-1α, hypoxia inducible factor-1-alpha, IKKβ, IκB kinase β; Lamptor, late endosomal/lysosomal adaptor, mitogen-activated protein kinase, and mTOR activator; LRP5/6, low-density lipoprotein receptor-related proteins 5 and 6; MEK1-2, mitogen-activated protein kinase 1-2; mLST8, mammalian lethal with SEC13 protein 8; mTOR, mammalian target of rapamycin; mTORC1, mTOR complex 1; P70S6K, 70-kDa ribosome protein S6Kinase; PDK1, 3-phosphoinositide-dependent protein kinase-1; PIP2, phosphatidylinositol 4,5-bisphosphate; PIP3, phosphatidylinositol-3,4,5-trisphosphate; PI3K, phosphoinositide 3-kinase; PRAS40, proline-rich Akt substrate of 40 kDa; PTEN, phosphatase and tensin homologue; RAF, rapidly accelerated fibrosarcoma viral oncogene homologue; RTK, rector of tyrosine kinase; Raptor, regulatory associated protein of mTOR complex 1; RAS, rat sarcoma viral oncogene homologue; Rheb, Ras homologue enriched in the brain; TSC, tuberous sclerosis complex; VDR, vitamin D receptor; VEGF, vascular endothelial growth factor.
Moreover, activation of VEGF, HIF, and endothelial cell-dependent mechanisms contribute to vasculogenic mimicry (VM) in cancer (21). This involves the formation of microvascular channels composed of tumor cells that contribute to the resistance to anti-angiogenic therapy (22). Hypoxic conditions and the aberrant activation of the mTOR/HIF/VEGF pathway can occur in cancer and TSC-associated tumors. Indeed, astrocytomas have a VM-like structure (23), and VEGF- and HIF-dependent factors play an important role in their pathogenesis (20). This mechanism may contribute to therapy resistance in SEGA and to tumor regrowth after discontinuation. Therefore, it is reasonable to target the HIF/VEGF pathway with 1,25-D since a VM-like structure may be formed in TSC-associated tumors, including SEGA.
TSC1 is suppressed by IκB kinase β (IKKβ) phosphorylation, leading to the activation of the mTOR pathway and increased VEGF production and angiogenesis (24). 1,25-D induces direct VDR-IKKβ protein interaction, disrupting the formation of the IKK complex, which consists of IKKα, β, and γ subunits, and abolishes IKKβ phosphorylation (25) (Figure 1B). Through this mechanism, 1,25-D suppresses VEGF-mediated angiogenesis.
4 Impaired interaction between TSC2 and VDR may contribute to TSC severity
In tumor-derived endothelial cells, the transcription of one of the VDR target genes, 24-hydroxylase (CYP24A1) is upregulated and this promotes the metabolism of 25-D and 1,25-D. The activation of CYP24A1 promotes 25-D metabolism, thereby potentially reducing the cellular availability of 1,25-D. Moreover, cell cycle arrest, growth inhibition, and apoptosis are induced by epigenetic silencing of CYP24A1 (26). Importantly, plasma membrane-bound TSC2 binds to calcium/calmodulin (CaM), and this complex is translocated to the nucleus, partially attenuating CYP24A1 transcription under normal conditions (27) (Figure 1C1). Mutations in TSC2 lead to more severe clinical features compared to those in TSC1 This, in turn, could contribute to the severity of TSC and the formation of TSC-associated tumors. This, in turn, could contribute to the severity of TSC and the formation of TSC-associated tumors. Therefore, along with inhibiting angiogenesis and regressing existing immature capillaries, 1,25-D treatment produces effects in tumor cells similar to those of epigenetic silencing of CYP24A1.
5 Second hit by epigenetic alterations in the vitamin D metabolism and VDR signaling pathway may be associated with clinical features in TSC
The correction of histone hyperacetylation in hippocampal neurons using histone deacetylase (HDAC) inhibitors improves abnormal synaptic plasticity and epilepsy in TSC (28). While second-hit mutagenesis may play an important role in the phenotypic diversity of renal lesions in TSC (29), the VDR and VDR-responsive genes can be epigenetically modified (30), similar to histone hyperacetylation in neurons. Therefore, postnatal epigenetic modifications of VDR-mediated signaling and vitamin D metabolism may serve as a second hit and contribute to the clinical variability and severity of TSC, including the development of tumors, abnormal synaptic plasticity, and epilepsy. As discussed above, CaM plays an important role in the TSC2-VDR interaction. Therefore, increased intracellular calcium and CaM activation caused by viral infection could increase the attenuation of VDR-sensitive gene transcription, including that of CYP24A1, by enhancing the TSC2-CaM interaction or inducing epigenetic modifications that affect the transcriptional activity of VDR-related genes (Figure 1C2).
Epigenetic silencing of VDR, which is also mediated by HDAC3, is crucial in 1,25-D resistance (30), and the treatment of VDR promoter hypermethylation with 5-aza-2′-deoxycytidine restored VDR mRNA expression (31). In addition, hypermethylation of CYP27B1, which activates 25-D to 1,25-D, can reduce the tissue levels of 1,25-D (30). High blood 25-D and 1,25-D levels may be required in this condition.
6 SEGA responds to 1,25-D under increased VDR expression and sensitivity
Although VDR expression is increased in human glioblastoma (GB) cells (32), high VDR expression in GB is associated with 1,25-D treatment success (33). In an in vitro study, calcitriol and vitamin D analogue blocked the stem-like properties of glioma cells (34), induced DNA fragmentation (35), and inhibited the migration of GB cells (33). Furthermore, 1,25-D restores responsiveness to itself by upregulating VDR expression (36). Based on these observations, VDR expression in SEGA potentially correlates with 1,25-D sensitivity or aggressiveness. It's also speculated that SEGA, exhibiting resistance or recurrence to mTOR inhibitors, may respond to 1,25-D similar to GB.
Vitamin D3 analogue can suppress the activation of the phosphatidylinositol 3-kinase (PI3K)/RAC-alpha serine/threonine-protein kinase (AKT) pathway and extracellular signal-regulated kinase (ERK)/Mitogen-activated protein kinase (MAPK) pathway due to mTORi therapy.
S6Kinase (S6K) activation via mTORC1 suppresses the PI3K/AKT and ERK pathways via negative feedback (Figure 1D). Therefore, S6K inhibition by mTORi contributes to the activation of both pathways, thereby contributing to the pathology of mTORi-resistance. Therefore, combination therapy with MAPK inhibitors may be useful (37). Notably, vitamin D and its analogs restrict gliomas by inducing cell cycle arrest via multiple mechanisms, including the PI3K/AKT pathway (12). Importantly, while therapy with mTORi and PI3Kα inhibitor is necessary for GB treatment (38), activation of the PI3K/AKT (39) and MAPK/ERK pathways (40) also plays an important role in SEGA development. Moreover, in TSC, the Raf-1/MAPK/ERK cascade, in addition to mTOR, leads to 4E-binding protein 1 phosphorylation, increased cyclin D protein levels, and increased protein synthesis (41). The active vitamin D analog, maxacalcitol, decreased hyperphosphorylation of MAPK-38p and ERK1/2 in the brain tissue of a mouse model of Alzheimer's disease (42). Therefore, the vitamin D3 analog may suppress the growth of renal cancer and SEGA in the same way. Particularly in patients with mutations in the tumor suppressor gene phosphatase and tensin homologue (PTEN), PI3K/AKT activation leads to tuberin phosphorylation and decreased activity of tuberin-hamartin complex, resulting in the activation of mTOR/70kDa-S6K1 signaling (43). A novel vitamin D3 analog, Gemini-23-yne-26,27-hexafluoro-D3, not only increased the expression of PTEN and caused the dephosphorylation of Akt, Ark target proteins, and mTOR, but also decreased the phosphorylation of its downstream effectors, S6Ks, and eukaryotic translation initiation factor 4E-binding protein 1, thereby suppressing protein synthesis and tumor proliferation (44) (Figures 1E1–E4). Similarly, tumors associated with Tuberous Sclerosis Complex (TSC), such as SEGA, might see improved treatment outcomes with novel vitamin D derivatives. These derivatives activate PTEN, suppress the PI3K/AKT/mTOR pathway, and enhance the effectiveness of mTOR inhibitors, potentially leading to safer and more effective therapies compared to using mTOR inhibitors alone.
7 Suppressing wingless/int-1 (Wnt)/β-catenin signaling
Abnormal Wnt/β-catenin signaling plays an important role in tumorigenesis (45). The TSC1-TSC2 complex negatively regulates cell proliferation through β-catenin signaling (46), which plays an important role in the pathogenesis of angiomyolipomas and lymphangioleiomyomatosis (47). Importantly, 1,25-D increases VDR/β-catenin binding, which, in turn, increases the transcription of one of the VDR target genes, the Wnt inhibitor Dickkopf-1, to a greater degree than that of the T-cell factor (48) (Figure 1F). As a result, 1,25-D decreases the transcription of β-catenin/T-cell factor-target genes that regulate cell proliferation, cell cycle regulation, and cellular metabolism (48). Therefore, 1,25-D may inhibit the growth of TSC-associated tumors.
8 Identification of 1,25-D-induced microRNAs (miRNAs) that suppress the growth of TSC-associated tumors and re-sensitize mTORi-resistant tumors
miRNAs are short noncoding RNAs with a wide range of gene regulatory activities at the post-transcriptional level (49). 1,25-D re-sensitizes everolimus-resistant hepatocellular carcinoma by upregulating miRNA-375, which regulates the oncogenes responsible for drug resistance (50). In addition, miRNA-22 mediates the suppression of several genes by 1,25-D, contributing to its antiproliferative and antimigratory effects in colon cancer cells (49). Therefore, it's worth exploring if 1,25-D also triggers miRNA-mediated antitumor effects in TSC and enhances the effectiveness or sensitivity to mTORi.
9 Initiating 1,25-D therapy in infancy improves brain development in TSC
Vitamin D is essential for brain development (51), and loss of TCS2 function in brain endothelial cells, neurons, oligodendrocytes, astrocytes, and microglia may increase the degradation of vitamin D and decrease its bioavailability by the same mechanism discussed above. Therefore, central nervous system vitamin D deficiency in TSC might be one of the drivers of impaired neural and synaptic maturation, as well as of tumorigenesis. Notably, blood levels of 25-D higher than 40 ng/ml can improve the autism spectrum disorder (ASD) rating (52). Thus, the same therapeutic effects can be expected in TSC. If the sensitivity of neural and brain endothelial cells to vitamin D is increased, early vitamin D treatment from infancy can improve brain development and pediatric TSC-associated neuropsychiatric disorders, including the core symptoms of ASD and refractory epilepsy.mTOR-dependent synaptic hyperconnectivity is implicated in ASD pathogenesis in Tsc2+/− mice (53). mTORi are effective in rescuing synaptic hyperconnectivity and controlling autistic behavior (53) and epilepsy in patients with TSC (54).
Neurological problems can be associated with decreased 1,25-D bioavailability in neurons and neuronal unresponsiveness to 1,25-D in the developing brain, owing to the epigenetic silencing of VDR-mediated signaling. In either case, the combination of mTORi and 1,25-D may synergistically improve brain development.
In addition, 1,25-D inhibits IKKβ phosphorylation and suppresses nuclear factor-κB (25), which plays an important role in the switch from oxidative stress to inflammation that contributes to epileptogenesis (55). Thus, 1,25-D may be promising for the reversal of TSC-associated brain pathological conditions and may play a role in suppressing the development of SEGA and reducing the clinical severity of comorbid neurological disorders.
10 Potential considerations in vitamin D treatment: therapy resistance and tumor growth
Since mTOR inhibition provides survival advantage for tumor cells (56), it is important to consider the risk of 1,25-D increasing tumor growth by inhibiting mTOR through DDIT4 activation. In silico analysis has shown that high DDIT4 expression, but not PI3K/mTOR activation, is associated with poor prognosis in some cancers, suggesting that DDIT4 inhibitors may be effective in these cases (56).
Tumor hypoxia, a condition in which solid tumors have hypoxic regions with an insufficient oxygen supply, contributes to resistance to chemotherapy (57). This may also occur in TSC-associated tumors. Under hypoxia, HIF-1α production is activated, which transcriptionally upregulates DDIT4 through a negative feedback loop of suppression against mTOR inhibition (15). Thus, when DDIT4 is overexpressed in TSC-associated tumors, mTOR/HIF-1α may persistently be overactivated by this mechanism, leading to a resistance to mTORi and 1,25-D. Under these conditions, DDIT4 inhibitors, but not 1,25-D, can minimize the dose of mTORi and avoid the need to discontinue treatment owing to side effects. Therefore, in vitro studies are important to determine the effectiveness of 1,25-D or its potential to exacerbate tumor progression, particularly in cases of high DDIT4 levels within the tumor tissue.
Moreover, while cholecalciferol exerts beneficial antidepressant effects through the activation of brain-derived growth factor/tyrosine receptor kinase B (TrkB) signaling in the prefrontal cortex (58), TrkB signaling plays an important role in TSC-associated neuropsychiatric disorders and epileptogenesis (59). While activation of the brain-derived neurotrophic factor/TrkB pathway by early 1,25-D therapy may improve brain development in children with TSC, epidemiological studies are required to investigate whether long-term 1,25-D treatment may improve neuropsychiatric disorders and epilepsy.
11 Epidemiological studies using large medical datasets may be useful for testing hypothesis
Considering the above discussion, it cannot be excluded that the clinical phenotypic variability in TSC, including tumor development, progression, and neurological problems, is partly due to the second hit in epigenetic modification of VDR and vit D-metabolizing enzyme genes by the environment, as well as dietary and supplemental vitamin D intake, use of multiple anticonvulsants, and reduced sun exposure. It is necessary to investigate whether 1,25-D treatment can reduce the risk or worsen the severity of TSC-associated tumors, refractory epilepsy, and neuropsychiatric conditions, including the core symptoms of ASD.
Analyzing medical big data or conducting retrospective studies on tumors, cancer incidence, prognosis, cognitive performance, and epilepsy severity in patients who received 1,25-D therapy compared to those who didn't, including assessing blood 25-D levels and the duration of 1,25-D therapy, may help determine if early and long-term treatment can benefit patients with TSC and TSC-associated tumors. Additionally, comparing the efficacy and side effects of mTORi alone vs. in combination with 1,25-D could provide valuable insights. However, conducting prospective studies raises ethical concerns due to the essential role of vitamin D in bone growth and immunity. Therefore, the analysis of medical big data could contribute to the discussion of the advantages and disadvantages of early 1,25-D therapy in patients with TSC.
12 Discussion
Since vitamin D has beneficial effects on several signaling pathways involved in the mechanism of TSC-associated tumors, 1,25-D and its analogs may be the first treatment choice. In children with TSC who have undertaken polytherapy of antiepileptic drugs, if vitamin D supplementation can not only prevent or slow tumor development but also improve brain development and reduce the core symptoms of TSC-associated neuropsychiatric disorders, it should be started immediately after diagnosis, preferably in infancy, in and added to anticonvulsant therapy. In addition, the possible increased metabolism of 25-D in TSC-associated tumors and the downregulation of the transcriptional activity of VDR-sensitive genes at a relatively high dose (blood levels of 25-D > 40 ng/ml) seems reasonable. As 1,25-D is relatively inexpensive and safe, children who are intolerant to mTORi, those in long-term care facilities, those receiving home care, or those unable to receive expensive medical care may benefit from 1,25-D therapy. Thus, 1,25-D may be a promising drug candidate for enhancing the effects of mTORi and improving tolerability, although it is also important to study whether it has any side effects. Particularly, the study of VDR signaling, including the epigenome, in TSC may have implications for drug discovery. Therefore, it is necessary to study vitamin D signaling and search for novel vitamin D analogs that are more effective and have fewer side effects, such as hypercalcemia in TSC-associated tumors.
In vitro studies are required to evaluate the efficacy of 1,25-D in TSC associated tumors, brain development, and core symptoms of psychiatric disorders.
Data availability statement
The original contributions presented in the study are included in the article/Supplementary Material, further inquiries can be directed to the corresponding author.
Author contributions
TN: Writing – original draft, Writing – review & editing.
Funding
The author declares that no financial support was received for the research, authorship, and/or publication of this article.
Acknowledgments
I thank Dr. T. Yokokura for providing me with the opportunity to conduct this study.
Conflict of interest
The author declares that the research was conducted in the absence of any commercial or financial relationships that could be construed as a potential conflict of interest.
Publisher's note
All claims expressed in this article are solely those of the authors and do not necessarily represent those of their affiliated organizations, or those of the publisher, the editors and the reviewers. Any product that may be evaluated in this article, or claim that may be made by its manufacturer, is not guaranteed or endorsed by the publisher.
Abbreviations
1,25-D, 1,25-dihydroxyvitamin D3; 25-D, 25-hydroxyvitamin D3; Akt, RAC-alpha serine/threonine-protein kinase; ASD, autism spectrum disorder; CaM, calcium/calmodulin; DDIT4, DNA damage-inducible transcript 4; ERK, extracellular signal-regulated kinase; GB, glioblastoma; HDAC, histone deacetylase; HIF-1α, hypoxia-inducible factor-1α; IκB, kinase β; MAPK, Mitogen-activated protein kinase; miRNA, microRNA; mTOR, mammalian target of rapamycin; mTORi, mTOR inhibitors; PI3K, phosphatidylinositol 3-kinase; PTEN, phosphatase and tensin homologue; S6K, S6Kinase; SEGA, subependymal giant cell astrocytoma; TrkB, tyrosine receptor kinase B; TSC, tuberous sclerosis complex; VDR, vitamin D receptor; VEGF, vascular endothelial growth factor; VM, vasculogenic mimicry; Wnt, wingless/int-1.
References
1. Henske EP, Jóźwiak S, Kingswood JC, Sampson JR, Thiele EA. Tuberous sclerosis complex. Nat Rev Dis Primers. (2016) 2:16035. doi: 10.1038/nrdp.2016.35
2. Panwar V, Singh A, Bhatt M, Tonk RK, Azizov S, Raza AS, et al. Multifaceted role of mTOR (mammalian target of rapamycin) signaling pathway in human health and disease. Signal Transduct Target Ther. (2023) 8:375. doi: 10.1038/s41392-023-01608-z
3. Kingswood JC, Belousova E, Benedik MP, Budde K, Carter T, Cottin V, et al. Tuberous sclerosis registry to increase disease awareness (TOSCA) post-authorisation safety study of everolimus in patients with tuberous sclerosis complex. Front Neurol. (2021) 12:630378. doi: 10.3389/fneur.2021.630378
4. Wang H, Wang J, Qu H, Wei H, Ji B, Yang Z, et al. In vitro and in vivo inhibition of mTOR by 1,25-dihydroxyvitamin D3 to improve early diabetic nephropathy via the DDIT4/TSC2/mTOR pathway. Endocrine. (2016) 54:348–59. doi: 10.1007/s12020-016-0999-1
5. Lisse TS, Hewison M. Vitamin D: a new player in the world of mTOR signaling. Cell Cycle. (2011) 10:1888–9. doi: 10.4161/cc.10.12.15620
6. Keum N, Chen QY, Lee DH, Manson JE, Giovannucci E. Vitamin D supplementation and total cancer incidence and mortality by daily vs. infrequent large-bolus dosing strategies: a meta-analysis of randomised controlled trials. Br J Cancer. (2022) 127:872–8. doi: 10.1038/s41416-022-01850-2
7. Ong JS, Dixon-Suen SC, Han X, An J, Esophageal Cancer Consortium; 23 and Me Research Team; Liyanage Uet al.A comprehensive reassessment of the association between vitamin D and cancer susceptibility using mendelian randomization. Nat Commun. (2021) 12:246. doi: 10.1038/s41467-020-20368-w
8. Kim MH, Yoo S, Choo MS, Cho MC, Son H, Jeong H. The role of the serum 25-OH vitamin D level on detecting prostate cancer in men with elevated prostate-specific antigen levels. Sci Rep. (2022) 12:14089. doi: 10.1038/s41598-022-17563-8
9. Jenab M, Bueno-de-Mesquita HB, Ferrari P, van Duijnhoven FJ, Norat T, Pischon T, et al. Association between pre-diagnostic circulating vitamin D concentration and risk of colorectal cancer in European populations: a nested case-control study. Br Med J. (2010) 340:b5500. doi: 10.1136/bmj.b5500
10. Sheeley MP, Andolino C, Kiesel VA, Teegarden D. Vitamin D regulation of energy metabolism in cancer. Br J Pharmacol. (2022) 179:2890–905. doi: 10.1111/bph.15424
11. Zigmont V, Garrett A, Peng J, Seweryn M, Rempala GA, Harris R, et al. Association between prediagnostic serum 25-hydroxyvitamin D concentration and glioma. Nutr Cancer. (2015) 67:1120–30. doi: 10.1080/01635581.2015.1073757
12. Lo CS, Kiang KM, Leung GK. Anti-tumor effects of vitamin D in glioblastoma: mechanism and therapeutic implications. Lab Invest. (2022) 102:118–25. doi: 10.1038/s41374-021-00673-8
13. Ruiz-Falcó Rojas ML, Feucht M, Macaya A, Wilken B, Hahn A, Maamari R, et al. Real-world evidence study on the long-term safety of everolimus in patients with tuberous sclerosis complex: final analysis results. Front Pharmacol. (2022) 13:802334. doi: 10.3389/fphar.2022.802334
14. Peixoto RD, Oliveira LJC, Passarini TM, Andrade AC, Diniz PH, Prolla G, et al. Vitamin D and colorectal cancer—a practical review of the literature. Cancer Treat Res Commun. (2022) 32:100616. doi: 10.1016/j.ctarc.2022.100616
15. Brugarolas J, Lei K, Hurley RL, Manning BD, Reiling JH, Hafen E, et al. Regulation of mTOR function in response to hypoxia by REDD1 and the TSC1/TSC2 tumor suppressor complex. Genes Dev. (2004) 18:2893–904. doi: 10.1101/gad.1256804
16. Lisse TS, Liu T, Irmler M, Beckers J, Chen H, Adams JS, et al. Gene targeting by the vitamin D response element binding protein reveals a role for vitamin D in osteoblast mTOR signaling. FASEB J. (2011) 25:937–47. doi: 10.1096/fj.10-172577
17. Ben-Shoshan M, Amir S, Dang DT, Dang LH, Weisman Y, Mabjeesh NJ. 1α,25-dihydroxyvitamin D3 (calcitriol) inhibits hypoxia-inducible factor-1/vascular endothelial growth factor pathway in human cancer cells. Mol Cancer Ther. (2007) 6:1433–9. doi: 10.1158/1535-7163.MCT-06-0677
18. Mantell DJ, Owens PE, Bundred NJ, Mawer EB, Canfield AE. 1α,25-dihydroxyvitamin D3 inhibits angiogenesis in vitro and in vivo. Circ Res. (2000) 87:214–20. doi: 10.1161/01.res.87.3.214
19. Arbiser JL, Brat D, Hunter S, D’Armiento J, Henske EP, Arbiser ZK, et al. Tuberous sclerosis-associated lesions of the kidney, brain, and skin are angiogenic neoplasms. J Am Acad Dermatol. (2002) 46:376–80. doi: 10.1067/mjd.2002.120530
20. Young LR, Inoue Y, McCormack FX. Diagnostic potential of serum VEGF-D for lymphangioleiomyomatosis. N Engl J Med. (2008) 358:199–200. doi: 10.1056/NEJMc0707517
21. Wei X, Chen Y, Jiang X, Peng M, Liu Y, Mo Y, et al. Mechanisms of vasculogenic mimicry in hypoxic tumor microenvironments. Mol Cancer. (2021) 20:7. doi: 10.1186/s12943-020-01288-1
22. Fathi Maroufi N, Taefehshokr S, Rashidi MR, Taefehshokr N, Khoshakhlagh M, Isazadeh A, et al. Vascular mimicry: changing the therapeutic paradigms in cancer. Mol Biol Rep. (2020) 47:4749–65. doi: 10.1007/s11033-020-05515-2
23. Seftor RE, Hess AR, Seftor EA, Kirschmann DA, Hardy KM, Margaryan NV, et al. Tumor cell vasculogenic mimicry: from controversy to therapeutic promise. Am J Pathol. (2012) 181:1115–25. doi: 10.1016/j.ajpath.2012.07.013
24. Lee DF, Kuo HP, Chen CT, Hsu JM, Chou CK, Wei Y, et al. IKKβ suppression of TSC1 links inflammation and tumor angiogenesis via the mTOR pathway. Cell. (2007) 130:440–55. doi: 10.1016/j.cell.2007.05.058
25. Chen Y, Zhang J, Ge X, Du J, Deb DK, Li YC. Vitamin D receptor inhibits nuclear factor κB activation by interacting with IκB kinase β protein. J Biol Chem. (2013) 288:19450–8. doi: 10.1074/jbc.M113.467670
26. Chung I, Karpf AR, Muindi JR, Conroy JM, Nowak NJ, Johnson CS, et al. Epigenetic silencing of CYP24 in tumor-derived endothelial cells contributes to selective growth inhibition by calcitriol. J Biol Chem. (2007) 282:8704–14. doi: 10.1074/jbc.M608894200
27. Kazami M, Sakamoto T, Suzuki T, Inoue H, Kato H, Kobayashi KI, et al. Ca2+/calmodulin induces translocation of membrane-associated TSC2 to the nucleus where it suppresses CYP24A1 expression. Biosci Biotechnol Biochem. (2022) 87:45–53. doi: 10.1093/bbb/zbac174
28. Basu T, O’Riordan KJ, Schoenike BA, Khan NN, Wallace EP, Rodriguez G, et al. Histone deacetylase inhibitors restore normal hippocampal synaptic plasticity and seizure threshold in a mouse model of tuberous sclerosis complex. Sci Rep. (2019) 9:5266. doi: 10.1038/s41598-019-41744-7
29. Pietrobon A, Stanford WL. Tuberous sclerosis complex kidney lesion pathogenesis: a developmental perspective. J Am Soc Nephrol. (2023) 34:1135–49. doi: 10.1681/ASN.0000000000000146
30. Fetahu IS, Höbaus J, Kállay E. Vitamin D and the epigenome. Front Physiol. (2014) 5:164. doi: 10.3389/fphys.2014.00164
31. Marik R, Fackler M, Gabrielson E, Zeiger MA, Sukumar S, Stearns V, et al. DNA methylation-related vitamin D receptor insensitivity in breast cancer. Cancer Biol Ther. (2010) 10:44–53. doi: 10.4161/cbt.10.1.11994
32. Salomón DG, Fermento ME, Gandini NA, Ferronato MJ, Arévalo J, Blasco J, et al. Vitamin D receptor expression is associated with improved overall survival in human glioblastoma multiforme. J Neurooncol. (2014) 118:49–60. doi: 10.1007/s11060-014-1416-3
33. Rehbein S, Possmayer AL, Bozkurt S, Lotsch C, Gerstmeier J, Burger M, et al. Molecular determinants of calcitriol signaling and sensitivity in glioma stem-like cells. Cancers (Basel). (2023) 15:5249. doi: 10.3390/cancers15215249
34. Gerstmeier J, Possmayer AL, Bozkurt S, Hoffmann ME, Dikic I, Herold-Mende C, et al. Calcitriol promotes differentiation of glioma stem-like cells and increases their susceptibility to temozolomide. Cancers (Basel). (2021) 13:3577. doi: 10.3390/cancers13143577
35. Baudet C, Chevalier G, Naveilhan P, Binderup L, Brachet P, Wion D. Cytotoxic effects of 1 alpha,25-dihydroxyvitamin D3 and synthetic vitamin D3 analogues on a glioma cell line. Cancer Lett. (1996) 100:3–10. doi: 10.1016/0304-3835(95)04054-4
36. Davoust N, Wion D, Chevalier G, Garabedian M, Brachet P, Couez D. Vitamin D receptor stable transfection restores the susceptibility to 1,25-dihydroxyvitamin D3 cytotoxicity in a rat glioma resistant clone. J Neurosci Res. (1998) 52:210–9. doi: 10.1002/(SICI)1097-4547(19980415)52:2%3C210::AID-JNR9%3E3.0.CO;2-D
37. Carracedo A, Ma L, Teruya-Feldstein J, Rojo F, Salmena L, Alimonti A, et al. Inhibition of mTORC1 leads to MAPK pathway activation through a PI3K-dependent feedback loop in human cancer. J Clin Invest. (2008) 118:3065–74. doi: 10.1172/JCI34739
38. Eckerdt FD, Bell JB, Gonzalez C, Oh MS, Perez RE, Mazewski C, et al. Combined PI3Kα-mTOR targeting of glioma stem cells. Sci Rep. (2020) 10:21873. doi: 10.1038/s41598-020-78788-z
39. Gao C, Zabielska B, Jiao F, Mei D, Wang X, Kotulska K, et al. Subependymal giant cell astrocytomas in tuberous sclerosis complex-current views on their pathogenesis and management. J Clin Med. (2023) 12:956. doi: 10.3390/jcm12030956
40. Bongaarts A, van Scheppingen J, Korotkov A, Mijnsbergen C, Anink JJ, Jansen FE, et al. The coding and non-coding transcriptional landscape of subependymal giant cell astrocytomas. Brain. (2020) 143:131–49. doi: 10.1093/brain/awz370
41. Cohen JD, Gard JM, Nagle RB, Dietrich JD, Monks TJ, Lau SS. ERK crosstalks with 4EBP1 to activate cyclin D1 translation during quinol-thioether-induced tuberous sclerosis renal cell carcinoma. Toxicol Sci. (2011) 124:75–87. doi: 10.1093/toxsci/kfr203
42. Saad El-Din S, Rashed L, Medhat E, Emad Aboulhoda B, Desoky Badawy A, Mohammed Shamseldeen A, et al. Active form of vitamin D analogue mitigates neurodegenerative changes in Alzheimer’s disease in rats by targeting Keap1/Nrf2 and MAPK-38p/ERK signaling pathways. Steroids. (2020) 156:108586. doi: 10.1016/j.steroids.2020.108586
43. Manning BD, Tee AR, Logsdon MN, Blenis J, Cantley LC. Identification of the tuberous sclerosis complex-2 tumor suppressor gene product tuberin as a target of the phosphoinositide 3-kinase/akt pathway. Mol Cell. (2002) 10:151–62. doi: 10.1016/s1097-2765(02)00568-3
44. O’Kelly J, Uskokovic M, Lemp N, Vadgama J, Koeffler HP. Novel gemini-vitamin D3 analog inhibits tumor cell growth and modulates the akt/mTOR signaling pathway. J Steroid Biochem Mol Biol. (2006) 100:107–16. doi: 10.1016/j.jsbmb.2006.04.003
45. Yu F, Yu C, Li F, Zuo Y, Wang Y, Yao L, et al. Wnt/β-catenin signaling in cancers and targeted therapies. Signal Transduct Target Ther. (2021) 6:307. doi: 10.1038/s41392-021-00701-5
46. Mak BC, Takemaru K, Kenerson HL, Moon RT, Yeung RS. The tuberin-hamartin complex negatively regulates β-catenin signaling activity. J Biol Chem. (2003) 278:5947–51. doi: 10.1074/jbc.C200473200
47. Mak BC, Kenerson HL, Aicher LD, Barnes EA, Yeung RS. Aberrant β-catenin signaling in tuberous sclerosis. Am J Pathol. (2005) 167:107–16. doi: 10.1016/s0002-9440(10)62958-6
48. Larriba MJ, González-Sancho JM, Barbáchano A, Niell N, Ferrer-Mayorga G, Muñoz A. Vitamin D is a multilevel repressor of wnt/b-catenin signaling in cancer cells. Cancers (Basel). (2013) 5:1242–60. doi: 10.3390/cancers5041242
49. Alvarez-Díaz S, Valle N, Ferrer-Mayorga G, Lombardía L, Herrera M, Domínguez O, et al. MicroRNA-22 is induced by vitamin D and contributes to its antiproliferative, antimigratory and gene regulatory effects in colon cancer cells. Hum Mol Genet. (2012) 21:2157–65. doi: 10.1093/hmg/dds031
50. Provvisiero DP, Negri M, de Angelis C, Di Gennaro G, Patalano R, Simeoli C, et al. Vitamin D reverts resistance to the mTOR inhibitor everolimus in hepatocellular carcinoma through the activation of a miR-375/oncogenes circuit. Sci Rep. (2019) 9:11695. doi: 10.1038/s41598-019-48081-9
51. Kesby JP, Turner KM, Alexander S, Eyles DW, McGrath JJ, Burne THJ. Developmental vitamin D deficiency alters multiple neurotransmitter systems in the neonatal rat brain. Int J Dev Neurosci. (2017) 62:1–7. doi: 10.1016/j.ijdevneu.2017.07.002
52. Zhang M, Wu Y, Lu Z, Song M, Huang X, Mi L, et al. Effects of vitamin D supplementation on children with autism spectrum disorder: a systematic review and meta-analysis. Clin Psychopharmacol Neurosci. (2023) 21:240–51. doi: 10.9758/cpn.2023.21.2.240
53. Pagani M, Barsotti N, Bertero A, Trakoshis S, Ulysse L, Locarno A, et al. mTOR-related synaptic pathology causes autism spectrum disorder-associated functional hyperconnectivity. Nat Commun. (2021) 12:6084. doi: 10.1038/s41467-021-26131-z
54. Aronica E, Specchio N, Luinenburg MJ, Curatolo P. Epileptogenesis in tuberous sclerosis complex-related developmental and epileptic encephalopathy. Brain. (2023) 146:2694–710. doi: 10.1093/brain/awad048
55. Arena A, Zimmer TS, van Scheppingen J, Korotkov A, Anink JJ, Mühlebner A, et al. Oxidative stress and inflammation in a spectrum of epileptogenic cortical malformations: molecular insights into their interdependence. Brain Pathol. (2019) 29:351–65. doi: 10.1111/bpa.12661
56. Pinto JA, Rolfo C, Raez LE, Prado A, Araujo JM, Bravo L, et al. In silico evaluation of DNA damage inducible transcript 4 gene (DDIT4) as prognostic biomarker in several malignancies. Sci Rep. (2017) 7:1526. doi: 10.1038/s41598-017-01207-3
57. Hompland T, Fjeldbo CS, Lyng H. Tumor hypoxia as a barrier in cancer therapy: why levels matter. Cancers (Basel). (2021) 13:499. doi: 10.3390/cancers13030499
58. Kouba BR, Torrá ACNC, Camargo A, Rodrigues ALS. The antidepressant-like effect elicited by vitamin D3 is associated with BDNF/TrkB-related synaptic protein synthesis. Metab Brain Dis. (2023) 38:601–11. doi: 10.1007/s11011-022-01115-0
Keywords: tuberous sclerosis complex, tumor, 1,25-Dihydroxyvitamin D3, vitamin D receptor, mTOR
Citation: Nobutoki T (2024) Vitamin D in tuberous sclerosis complex-associated tumors. Front. Pediatr. 12:1392380. doi: 10.3389/fped.2024.1392380
Received: 27 February 2024; Accepted: 10 May 2024;
Published: 23 May 2024.
Edited by:
Cristina Vassalle, Gabriele Monasterio Tuscany Foundation (CNR), ItalyReviewed by:
Xiaojuan Wu, Huazhong University of Science and Technology, China© 2024 Nobutoki. This is an open-access article distributed under the terms of the Creative Commons Attribution License (CC BY). The use, distribution or reproduction in other forums is permitted, provided the original author(s) and the copyright owner(s) are credited and that the original publication in this journal is cited, in accordance with accepted academic practice. No use, distribution or reproduction is permitted which does not comply with these terms.
*Correspondence: Tatsuro Nobutoki, bmJ0ay5rYW1ha3VyYS5wZWRAdGVub3Iub2NuLm5lLmpw