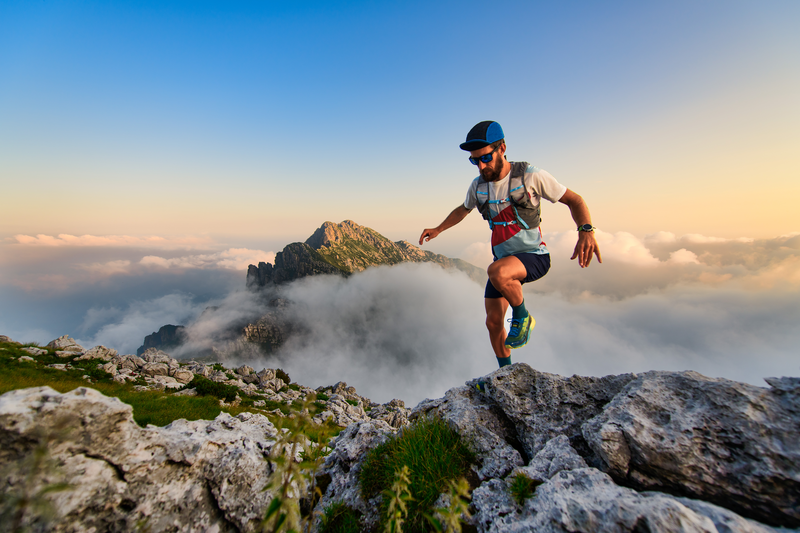
95% of researchers rate our articles as excellent or good
Learn more about the work of our research integrity team to safeguard the quality of each article we publish.
Find out more
ORIGINAL RESEARCH article
Front. Pediatr. , 25 October 2024
Sec. Pediatric Infectious Diseases
Volume 12 - 2024 | https://doi.org/10.3389/fped.2024.1388132
Aims: To investigate the epidemiological and clinical characteristics of children with Mycoplasma pneumoniae pneumonia (MPP) in Hubei, China.
Methods: We retrospectively analyzed inpatients with MPP from 20 hospitals in Hubei, China from January 2021 to December 2022. The co-detected pathogens of Mycoplasma pneumoniae (M. pneumoniae) were investigated using targeted next-generation sequencing (tNGS), and 23S rRNA gene mutations were analyzed to assess the macrolide resistance.
Results: M. pneumoniae infected 20.7% of patients with CAP, with cough (96.59%) and fever (80.28%) being the most prevalent symptoms. The infection rates in children younger than 1, 1–2, 3–6, 7–12, and older than 12 years were 6.17%, 19.98%, 26.97%, 43.93%, and 2.95%, respectively. Among 1,349 patients undergoing tNGS, the overall co-detection rate was 59.45%, with Streptococcus pneumoniae (29.30%), Haemophilus influenzae (23.57%), and Human rhinovirus (17.21%) being the most commonly co-detected pathogens. In 635 patients undergoing the 23S rRNA gene mutation test, 86.30% exhibited positive mutations (A2063G, 98.00%; A2064G, 1.50%; A2067G, 0.50%). Despite a significant age difference (P = 0.037) between macrolide-resistant M. pneumoniae and macrolide-sensitive M. pneumoniae groups, there were no significant differences in symptoms, lab data, or disease severity.
Conclusions: In Hubei Province, the prevalence of exhibited consistent changes during the COVID-19 pandemic. MPP was prevalent year-round, particularly in summer and autumn, with school-age children being more susceptible. Co-detections of viruses and bacteria were frequent in MPP cases, and macrolide resistance exceeded 85%. Ongoing surveillance of M. pneumoniae in children is crucial for understanding the healthcare impact of MPP.
Mycoplasma pneumoniae (M. pneumoniae) is a common causative pathogen in community-acquired pneumonia (CAP), accounting for 8%–40% of CAP cases (1–4). M. pneumoniae has traditionally been recognized as the predominant pathogen affecting children aged 5–14 years (2, 5). However, there has been a notable rise in reported cases among younger children and newborns (6). The clinical manifestations of M. pneumoniae infection are usually mild and self-limiting. In recent years, an increasing number of cases have progressed to severe M. pneumoniae pneumonia (SMPP) (7–9) and refractory MPP (RMPP) (10), resulting in multiorgan dysfunction, pleural effusion (11), and serious long-term sequelae, such as bronchiolitis obliterans and bronchiectasis, posing significant challenges for pediatricians.
Previous studies found that M. pneumoniae is often co-infected with other pathogens. Compared with M. pneumoniae mono-infection, co-infection may lead to more severe inflammatory responses and clinical manifestations, which may prolong or aggravate the course of MPP, suggesting that M. pneumoniae co-infection with other pathogens may be one of the causes of SMPP or RMPP (12).
Macrolides are the first-line antimicrobial agents used to treat M. pneumoniae infections. However, since 2000, macrolide-resistant strains have become increasingly common, coinciding with the more widespread usage of macrolides (13). More than 85% of M. pneumoniae strains among pediatric patients in China have been reported as macrolide-resistant M. pneumoniae (MRMP) (14). MRMP infection and excessive immunological inflammation may play important roles in the occurrence and development of RMPP. Therefore, it is essential to dynamically monitor M. pneumoniae infections in children and comprehend their epidemiological changes to develop effective preventive measures.
M. pneumoniae infection appears as a cyclic epidemic disease worldwide, with intervals of 3–7 years, and can persist for 1–2 years (15). M. pneumoniae infection is sporadic throughout the year, with variable high-incidence seasons in different regions. The prevalence of M. pneumoniae infection may be influenced by factors such as geography, season, temperature, humidity, and anti-epidemic policies. In this study, we systematically investigated the distribution of MPP in children by age, symptoms, co-detected pathogens, and macrolide resistance. We also provided epidemiological evidence based on a large sample size to shed insights into the prevalence of MPP in central China.
This is a multicenter retrospective study conducted in 20 hospitals across Hubei, China, under the leadership of Wuhan Children's Hospital. The study was approved by the Ethics Committee of Wuhan Children's Hospital (No. 2022R100-E02). We incorporated patients younger than 18 years who were hospitalized with CAP between 1 January 2021 and 30 December 2022. Patient information included demography, symptoms, diagnosis, laboratory measurements, and chest CT scans. We excluded the patients if they were not diagnosed with M. pneumoniae-infected pneumonia or if patients with a negative M. pneumoniae within 48 h of admission leaving a final cohort of 6,401 inpatients with MPP. To study the distribution of co-detected pathogens, we further analyzed 1,349 patients who underwent targeted next-generation sequencing (tNGS) testing using throat swabs or bronchoalveolar lavage fluid. Of these, 635 patients underwent 23S rRNA gene mutation testing to identify macrolide resistance in M. pneumoniae (Figure 1).
Figure 1. Flowchart of the study population selection. CAP, community-acquired pneumonia; M. pneumoniae, Mycoplasma pneumoniae; MPP, Mycoplasma pneumoniae pneumonia; tNGS, targeted next-generation sequencing; MRMP, macrolide-resistant Mycoplasma pneumoniae; MSMP, macrolide-sensitive Mycoplasma pneumoniae.
The diagnostic criteria for CAP included the following (16): (a) clinical manifestations, including fever, cough, and/or dyspnea; (b) auscultatory findings, such as abnormal breath sounds, wheezes, or crackles; and (c) radiographic evidence, including consolidation, other infiltrates, or pleural effusion. CAP patients with positive results for M. pneumoniae DNA or RNA or an M. pneumoniae IgG titer ≥160 were considered M. pneumoniae-positive. Patients were considered to have M. pneumoniae mono-detection if only M. pneumoniae were detected. Co-detection was defined as detecting M. pneumoniae with at least one additional bacterial or viral pathogen.
Nasopharyngeal swabs (NS) or bronchoalveolar lavage fluid (BALF) were obtained for M. pneumoniae molecular testing. NS samples were collected from the enrolled patients within 24 h of admission. Electronic bronchoscopy was performed when necessary, and BALF samples were collected during these procedures simultaneously within the hospitalization period. Serum samples were collected within 24 h of admission to detect anti-M. pneumoniae titers.
The principle of tNGS detection in this study involved multiple polymerase chain reaction (PCR) combined with NGS technology to specifically target the highly conserved region of 198 respiratory pathogens. Specific primers were designed for PCR amplification, which was conducted in an amplification tube to enrich the target pathogens. Subsequently, sequencing joints were connected through a second round of PCR to differentiate the source of the samples. High-throughput sequencing data were obtained using the gene sequencer KM MiniSeqDx-CN, manufactured by Guangzhou Jinquirui Biotechnology Co., Ltd. Bioinformatics software was used to filter the sequencing data and perform a subsequent comparison with the reference genome, thereby enabling the interpretation of pathogen detection outcomes. In addition, the concurrent detection of human DNA within the sample served as a means to monitor the sample's quality.
The tNGS test also encompassed three distinct categories of genetic tests for drug resistance, namely, carbapenem-resistant Enterobacteriaceae (CRE), methicillin-resistant Staphylococcus aureus (MRSA), and macrolide-resistant M. pneumoniae. Point mutations at nucleotide positions A2063, A2064, A2067, and C2617 in domain V of the M. pneumoniae 23S rRNA were detected to identify macrolide resistance in M. pneumoniae.
Statistical analyses were performed using SPSS version 22.0 (International Business Machines Corp., New York, USA) and GraphPad Prism 6.0 (GraphPad Software, San Diego, CA, USA). Continuous variables conforming to a normal distribution were presented as mean ± standard deviation (SD), while those not conforming to a normal distribution were represented by the median. Categorical variables were described as numbers or percentages. Quantitative data were compared using the t-test or Mann–Whitney U-test. Group comparisons of categorical variables were performed using the chi-square test. A P-value of <0.05 indicated statistical significance.
Out of 30,923 CAP inpatients, 6,401 (20.70%) were positive for M. pneumoniae in our study. The monthly distribution of all the MPP patients is shown in Figure 2. In 2022, the peak infection rate of MPP occurred between June and September, accounting for approximately 25%–34% of monthly CAP patients. However, in 2021, the MPP spread throughout the year, with no obvious peak (an infection rate of around 17%).
Figure 2. Monthly distribution of MPP patients from January 2021 to December 2022. CAP, community-acquired pneumonia; MPP, Mycoplasma pneumoniae pneumonia.
Among all MPP patients, 2,953 were girls (46.13%) and 3,448 (53.87%) were boys, indicating that the prevalence of MPP was almost evenly distributed in males and females. The median age of children with MPP was 3.79 years. The distribution of MPP patients among children with CAP under 1, 1–2, 3–6, 7–12, and above 12 years was 395 (6.17%), 1,279 (19.98%), 1,726 (26.97%), 2,812 (43.93%), and 189 (2.95%), respectively (Figure 3). Therefore, the MPP rate was higher in older children than in younger ones, with an increasing tendency.
Among the 6,401 MPP patients, the most common symptoms were cough (96.59%) and fever (80.28%), followed by wheezing (18.37%), vomiting and diarrhea (14.37%), and dyspnea (7.31%) (Figure 4). Extrapulmonary manifestations were observed in 562 patients (8.78%), including skin rash in 432 (6.75%) and neurological symptoms in 130 (2.03%). Systemic underlying diseases were present in 487 patients (7.61%), with the most common being recurrent respiratory infection in 447 (6.98%), asthma in 30 (0.47%), congenital heart diseases in 51 (0.80%), bronchopulmonary dysplasia in 27 (0.42%), malnutrition in 18 (0.28%), immunodeficiency diseases in 8 (0.12%), neurological diseases in 38 (0.59%), and malignant tumors in 3 (0.05%).
To understand M. pneumoniae co-detection, we analyzed the tNGS results of 1,349 patients. Among them, 547 patients had M. pneumoniae mono-detection, while 802 patients had M. pneumoniae co-detection, yielding a 59.45% overall co-detection rate. The top 10 co-detected pathogens were S. pneumoniae (235, 29.30%), H. influenzae (189, 23.57%), human rhinovirus (138, 17.21%), human adenovirus (87, 10.85%), influenza A (52, 6.48%), human boca virus (46, 5.74%), Klebsiella pneumoniae (43, 5.36%), parainfluenza virus type 3 (33, 4.11%), Moraxella catarrhalis (33, 4.11%), and respiratory syncytial virus (32, 3.99%) (Figure 5).
In total, 635 MPP patients underwent testing for M. pneumoniae 23S rRNA gene mutations, including A2063G, A2064G, A2067G, and C2617G mutations. Among these, 548 (86.30%) patients were confirmed to have 23S rRNA mutations, with A2063G being the most prevalent mutation (98.00%), which is consistent with its high prevalence, in Asian countries, followed by A2064G (1.50%) and A2067G (0.50%) (Table 1).
According to macrolide resistance, the 635 patients were divided into macrolide-sensitive M. pneumoniae group (MSMP, n = 87) and MRMP group (n = 548). The MRMP group was younger than the MSMP group (P = 0.037) (Table 2). There were no remarkable differences in clinical symptoms or laboratory test results between the two groups. Although the MRMP group had more severe pneumonia patients than the MSMP group, there was no statistical difference (P = 0.154). Furthermore, no significant difference was found in the co-detection rate between the two groups. There was no statistically significant difference between the MSMP group and the MRMP group in terms of the proportion of patients receiving macrolide treatment, nor in the proportion of patients experiencing a fever duration exceeding seven days following the administration of effective antibiotics.
M. pneumoniae is a common pathogen that causes CAP in children (2). In the present study, the M. pneumoniae infection rate in CAP patients was 20.7%. Although M. pneumoniae infection occurs throughout the year, the peak period is usually from the end of summer to the beginning of winter (17, 18). Different from the usual seasonal patterns, our study shows that M. pneumoniae infections in Hubei Province remained low in 2021, which was similar respiratory virus trends in Wuhan (19), before peaking in the summer of 2022. During the COVID-19 outbreak in Wuhan, the epicenter, the continuous ongoing use of non-pharmaceutical interventions (NPIs) demonstrated their effectiveness in preventing respiratory infectious diseases, which supports findings from previous studies. However, a prolonged period of reduced exposure to M. pneumoniae also leads to an immunity gap (20). We posit that the primary factor contributing to increased susceptibility to M. pneumoniae infection is this immunity gap, which is characterized by a decline in humoral immunity. In addition, the M. pneumoniae infection rate increased with age, with the highest rate found in school-age children (7–12 years). This trend could be partially explained by the fact that M. pneumoniae outbreaks are closely related to close contact among individuals and the population density in kindergartens and schools is relatively high (21).
Consistent with earlier observations (22), our study indicated that the majority of patients exhibited cough (96.59%) and fever (80.28%) as the most common clinical symptoms of MPP. In addition, we discovered that 18.37% of MPP children experienced wheezing. This symptom may be related to the anatomical structure and physiological developmental stage of the respiratory tract in children. We speculate that after M. pneumoniae infiltrates the respiratory tract, it may promote the release of inflammatory mediators by inflammatory cells, resulting in inflammatory changes in the respiratory system and increasing airway reactivity, leading to wheezing. Therefore, in children with wheezing episodes, in addition to viral infection, full attention should be paid to the possibility of M. pneumoniae infection. The main clinical manifestations of MPP in children are consistent with those observed before the COVID-19 pandemic. However, recent years have seen an increase in RMPP or SMPP cases, which can lead to the prolonging of symptoms such as cough and fever and the aggravation of wheezing and shortness of breath. Multiple factors, including M. pneumoniae load, macrolide resistance, systemic inflammatory response, and co-infection, may contribute to the development of SMPP and RMPP (12, 23, 24).
Co-infection with other pathogens is common in MPP patients. In the present study, 59.45% of CAP patients were co-detected with M. pneumoniae and other pathogens, which is slightly higher than the earlier recorded rates of 10%–56% (12, 18). This could be attributed to the variations in climate, geographic location, and detection methods. The sensitivity and wide coverage of the tNGS respiratory pathogen panel could contribute to the higher positive rate. We found that the top five co-detected pathogens were S. pneumoniae, H. influenzae, human rhinovirus, human adenovirus, and influenza A. According to a previous report (18), the most common co-detected viruses in children with MPP were human adenovirus and human rhinovirus. Another study found that the most common bacterial co-infection in children with MPP was S. pneumoniae, followed by H. influenzae (25), which was consistent with our findings. Interestingly, the nasopharyngeal microbiota of M. pneumoniae carriers also had a higher abundance of H. influenzae (26). Given the strong association with H. influenzae, we recommend including appropriate antibiotic coverage for H. influenzae in cases of suspected pneumonia in children. In addition, M. pneumoniae carriers were found to have a less diverse microbiota, with an overrepresentation of disease-associated microbiota members compared to non-carriers. However, further research and exploration is needed to understand how M. pneumoniae infection affects the evolution of the respiratory bacteriome and virome.
Macrolides are the first-line antibiotics for treating MPP. However, with the increasing prevalence of macrolide resistance in M. pneumoniae infections worldwide, especially severe in Asian countries (13, 27), concerns have been raised about the efficacy of macrolides in treating MPP in children. Macrolide resistance is conferred by mutations at several positions in domain V of the 23S rRNA gene, including A2063G, A2064G, A2067G, and C2617G, where A2063G and A2064G mutations confer high-level resistance to all macrolides. Epidemiological studies reported a macrolide resistance rate of 12%–88% among M. pneumoniae infections (27, 28). In our study, the prevalence of macrolide resistance was 86.3%, with A2063G being the major mutation. Moreover, we found that children with macrolide-resistant strains were younger than those with macrolide-sensitive ones (Table 2), which contrasts with prior research. To enhance the surveillance of macrolide resistance in M. pneumoniae, studies involving a more extensive population are required.
In the present study, we compared macrolide-resistant MPP children to macrolide-sensitive MPP children to reveal whether macrolide resistance affects clinical symptoms, laboratory data, imaging features, and disease severity. We found no significant differences in symptoms, such as fever, cough, and wheezing, macrolides treatment, as well as in laboratory and radiographic findings between MRMP and MSMP groups (Table 2). A study (29) also reported that MRMP and MSMP groups shared similar demographics and clinical characteristics. Therefore, it may be difficult to identify macrolide-resistant cases based on the initial presentations. In addition, there was no difference in disease severity of MPP between the two groups, suggesting that macrolide resistance alone is not a major contributor to the development of severe pneumonia. M. pneumoniae infection generally causes mild, and self-limiting disease, raising the question of whether antibiotic treatment is necessary. On the other hand, not only macrolide-sensitive cases but also some macrolide-resistant cases can benefit from macrolide administration due to its immunomodulatory effects. Thus, it remains debatable whether all MPP patients with macrolide resistance should be switched to other anti-mycoplasma drugs. However, alternative antibiotics might be warranted in patients with severe MPP.
There are some limitations to the presented study. As a retrospective clinical study, the techniques used for detecting M. pneumoniae were not uniform. In addition, not all MPP patients were tested for macrolide resistance through 23S rRNA gene mutation analysis; therefore, the study population analyzed for macrolide resistance characteristics did not represent the whole population. Further monitoring of macrolide resistance in M. pneumoniae is needed in future studies.
In Hubei Province, the prevalence of M. pneumoniae exhibited consistent changes during the COVID-19 pandemic. MPP remained prevalent year-round, particularly in summer and autumn, with school-age children being more susceptible. Co-detections of viruses and bacteria were frequent in MPP cases, and macrolide resistance exceeded 85%. Ongoing surveillance of M. pneumoniae in children is crucial for understanding the healthcare impact of MPP.
The original contributions presented in the study are included in the article/Supplementary Material, further inquiries can be directed to the corresponding authors.
The studies involving humans were approved by the Ethics Committee of Wuhan Children’s Hospital. The studies were conducted in accordance with the local legislation and institutional requirements. Written informed consent for participation in this study was provided by the participants’ legal guardians/next of kin.
HD: Conceptualization, Formal Analysis, Methodology, Writing – original draft. JuL: Conceptualization, Formal Analysis, Writing – original draft. XilL: Investigation, Writing – original draft. JZ: Formal Analysis, Writing – original draft. WLu: Data curation, Investigation, Writing – original draft. QZ: Data curation, Investigation, Writing – original draft. WLi: Data curation, Investigation, Writing – original draft. XinL: Data curation, Investigation, Writing – original draft. QL: Data curation, Investigation, Writing – original draft. SH: Data curation, Investigation, Writing – original draft. JM: Data curation, Investigation, Writing – original draft. RH: Data curation, Investigation, Writing – original draft. BS: Data curation, Investigation, Writing – original draft. LZ: Data curation, Investigation, Writing – original draft. JW: Data curation, Investigation, Writing – original draft. JY: Data curation, Investigation, Writing – original draft. HL: Data curation, Investigation, Writing – original draft. HC: Conceptualization, Supervision, Writing – original draft. YiL: Formal Analysis, Writing – original draft. YanL: Data curation, Writing – original draft. YaxL: Data curation, Formal Analysis, Writing – original draft. YuL: Investigation, Writing – original draft. YW: Conceptualization, Writing – review & editing. YLiu: Supervision, Writing – review & editing. JiL: Supervision, Writing – review & editing. XiaL: Conceptualization, Funding acquisition, Project administration, Writing – review & editing.
The author(s) declare financial support was received for the research, authorship, and/or publication of this article. This study was supported by the Health Commission of Hubei Province (grant number WJ2021M262).
The authors are grateful to all patients who participated in this study and the staff of 20 hospitals for their collaboration.
The authors declare that the research was conducted in the absence of any commercial or financial relationships that could be construed as a potential conflict of interest.
All claims expressed in this article are solely those of the authors and do not necessarily represent those of their affiliated organizations, or those of the publisher, the editors and the reviewers. Any product that may be evaluated in this article, or claim that may be made by its manufacturer, is not guaranteed or endorsed by the publisher.
1. Jiang ZL, Li SH, Zhu CM, Zhou RJ, Leung PHM. Mycoplasma pneumoniae infections: pathogenesis and vaccine development. Pathogens. (2021) 10:119. doi: 10.3390/pathogens10020119
2. Jain S, Williams DJ, Arnold SR, Ampofo K, Bramley AM, Reed C, et al. Community-acquired pneumonia requiring hospitalization among U.S. children. N Engl J Med. (2015) 372:835–45. doi: 10.1056/NEJMoa1405870
3. Meyer Sauteur PM, Jacobs BC, Spuesens EB, Jacobs E, Nadal D, Vink C, et al. Antibody responses to Mycoplasma pneumoniae: role in pathogenesis and diagnosis of encephalitis? PLoS Pathog. (2014) 10:e1003983. doi: 10.1371/journal.ppat.1003983
4. Xiao L, Ptacek T, Osborne JD, Crabb DM, Simmons WL, Lefkowitz EJ, et al. Comparative genome analysis of Mycoplasma pneumoniae. BMC Genomics. (2015) 16:610. doi: 10.1186/s12864-015-1801-0
5. Xu YC, Zhu LJ, Xu D, Tao XF, Li SX, Tang LF, et al. Epidemiological characteristics and meteorological factors of childhood Mycoplasma pneumoniae pneumonia in Hangzhou. World J Pediatr. (2011) 7:240–4. doi: 10.1007/s12519-011-0318-0
6. Gadsby NJ, Reynolds AJ, McMenamin J, Gunson RN, McDonagh S, Molyneaux PJ, et al. Increased reports of Mycoplasma pneumoniae from laboratories in Scotland in 2010 and 2011—impact of the epidemic in infants. Euro Surveill. (2012) 17:20110. doi: 10.2807/ese.17.10.20110-en
7. Chen LQ, Yin J, Liu XY, Liu J, Xu BP, Shen KL. Thromboembolic complications of Mycoplasma pneumoniae pneumonia in children. Clin Respir J. (2023) 17:187–96. doi: 10.1111/crj.13584
8. Lee KL, Lee CM, Yang TL, Yen TY, Chang LY, Chen JM, et al. Severe Mycoplasma pneumoniae pneumonia requiring intensive care in children, 2010–2019. J Formos Med Assoc. (2021) 120:281–91. doi: 10.1016/j.jfma.2020.08.018
9. Yan C, Xue GH, Zhao HQ, Feng YL, Li SL, Cui JH, et al. Molecular and clinical characteristics of severe Mycoplasma pneumoniae pneumonia in children. Pediatr Pulmonol. (2019) 54:1012–21. doi: 10.1002/ppul.24327
10. Tong L, Huang SM, Zheng C, Zhang YY, Chen ZM. Refractory Mycoplasma pneumoniae pneumonia in children: early recognition and management. J Clin Med. (2022) 11:2824. doi: 10.3390/jcm11102824
11. Luo XQ, Luo J, Wang CJ, Luo ZX, Tian DY, Xie XH. Clinical features of severe Mycoplasma pneumoniae pneumonia with pulmonary complications in childhood: a retrospective study. Pediatr Pulmonol. (2023) 58:2815–22. doi: 10.1002/ppul.26593
12. Zhang XX, Chen ZR, Gu WJ, Ji W, Wang YQ, Hao CL, et al. Viral and bacterial co-infection in hospitalised children with refractory Mycoplasma pneumoniae pneumonia. Epidemiol Infect. (2018) 146:1384–8. doi: 10.1017/S0950268818000778
13. Chen YC, Hsu WY, Chang TH. Macrolide-resistant Mycoplasma pneumoniae infections in pediatric community-acquired pneumonia. Emerg Infect Dis. (2020) 26:1382–91. doi: 10.3201/eid2607.200017
14. Guo DX, Hu WJ, Wei R, Wang H, Xu BP, Zhou W, et al. Epidemiology and mechanism of drug resistance of Mycoplasma pneumoniae in Beijing, China: a multicenter study. Bosn J Basic Med Sci. (2019) 19:288–96. doi: 10.17305/bjbms.2019.4053
15. Waites KB, Xiao L, Liu Y, Balish MF, Atkinson TP. Mycoplasma pneumoniae from the respiratory tract and beyond. Clin Microbiol Rev. (2017) 30:747–809. doi: 10.1128/CMR.00114-16
16. National Health Commission of the People’s Republic of China, State Administration of Traditional Chinese Medicine. Guideline for diagnosis and treatment of community-acquired pneumonia in children (2019 version) (in Chinese). Chin J Clin Infect Dis. (2019) 12:6–13. doi: 10.3760/cma.j.issn.1674-2397.2019.01.002
17. Meyer Sauteur PM, Unger WWJ, van Rossum AMC, Berger C. The art and science of diagnosing Mycoplasma pneumoniae infection. Pediatr Infect Dis J. (2018) 37:1192–5. doi: 10.1097/INF.0000000000002171
18. Chiu CY, Chen CJ, Wong KS, Tsai MH, Chiu CH, Huang YC. Impact of bacterial and viral coinfection on mycoplasmal pneumonia in childhood community-acquired pneumonia. J Microbiol Immunol Infect. (2015) 48:51–6. doi: 10.1016/j.jmii.2013.06.006
19. Li Y, Wu ZY, Yan Y, Shi Y, Huang JM, Du H, et al. Prevalence of respiratory viruses among hospitalized children with lower respiratory tract infections during the COVID-19 pandemic in Wuhan, China. Int J Infect Dis. (2024) 139:6–12. doi: 10.1016/j.ijid.2023.11.019
20. Liu BJ, Xu LN, Ma Y, Wang H, Xu XN, Wang YQ, et al. Evidence of immunity gap: Decline in antibodies against M. pneumoniae during the COVID-19 pandemic. J Infect. (2024) 89(2):106209. doi: 10.1016/j.jinf.2024.106209
21. Klement E, Talkington DF, Wasserzug O, Kayouf R, Davidovitch N, Dumke R, et al. Identification of risk factors for infection in an outbreak of Mycoplasma pneumoniae respiratory tract disease. Clin Infect Dis. (2006) 43:1239–45. doi: 10.1086/508458
22. Kuo CY, Tsai WC, Lee HF, Ho TS, Huang LM, Shen CF, et al. The epidemiology, clinical characteristics, and macrolide susceptibility of Mycoplasma pneumoniae pneumonia in children in southern Taiwan, 2019–2020. J Microbiol Immunol Infect. (2022) 55:611–9. doi: 10.1016/j.jmii.2021.09.010
23. Morozumi M, Takahashi T, Ubukata K. Macrolide-resistant Mycoplasma pneumoniae: characteristics of isolates and clinical aspects of community-acquired pneumonia. J Infect Chemother. (2010) 16:78–86. doi: 10.1007/s10156-009-0021-4
24. Zhang C, Zhang Q, Du JL, Deng D, Gao YL, Wang CL, et al. Correlation between the clinical severity, bacterial load, and inflammatory reaction in children with Mycoplasma pneumoniae pneumonia. Curr Med Sci. (2020) 40:822–8. doi: 10.1007/s11596-020-2261-6
25. Song Q, Xu BP, Shen KL. Bacterial co-infection in hospitalized children with Mycoplasma pneumoniae pneumonia. Indian Pediatr. (2016) 53:879–82. doi: 10.1007/s13312-016-0951-8
26. Koenen MH, de Groot RCA, de Steenhuijsen Piters WAA, Chu MLJN, Arp K, Hasrat R, et al. Mycoplasma pneumoniae carriage in children with recurrent respiratory tract infections is associated with a less diverse and altered microbiota. EBioMedicine. (2023) 98:104868. doi: 10.1016/j.ebiom.2023.104868
27. Hung HM, Chuang CH, Chen YY, Liao WC, Li SW, Chang IY, et al. Clonal spread of macrolide-resistant Mycoplasma pneumoniae sequence type-3 and type-17 with recombination on non-P1 adhesin among children in Taiwan. Clin Microbiol Infect. (2021) 27(1169):1169.e1–6. doi: 10.1016/j.cmi.2020.09.035
28. Yang TI, Chang TH, Lu CY, Chen JM, Lee PI, Huang LM, et al. Mycoplasma pneumoniae in pediatric patients: do macrolide-resistance and/or delayed treatment matter? J Microbiol Immunol Infect. (2019) 52:329–35. doi: 10.1016/j.jmii.2018.09.009
Keywords: Mycoplasma pneumoniae, children, prevalence, co-detection, macrolide resistance
Citation: Du H, Li J, Li X, Zhao J, Lu W, Zhang Q, Liu W, Luo X, Lu Q, Hu S, Ma J, He R, Sha B, Zhang L, Wu J, Yang J, Li H, Chen H, Li Y, Li Y, Lin Y, Liu Y, Wu Y, Liu Y, Li J and Lu X (2024) Impact of the COVID-19 pandemic on epidemiological and clinical characteristics of Mycoplasma pneumoniae pneumonia in children: a multicenter study from Hubei, China. Front. Pediatr. 12:1388132. doi: 10.3389/fped.2024.1388132
Received: 21 February 2024; Accepted: 30 September 2024;
Published: 25 October 2024.
Edited by:
Yongdong Yan, Children’s Hospital of Soochow University, ChinaReviewed by:
Eitan Naaman Berezin, Santa Casa of Sao Paulo, BrazilCopyright: © 2024 Du, Li, Li, Zhao, Lu, Zhang, Liu, Luo, Lu, Hu, Ma, He, Sha, Zhang, Wu, Yang, Li, Chen, Li, Li, Lin, Liu, Wu, Liu, Li and Lu. This is an open-access article distributed under the terms of the Creative Commons Attribution License (CC BY). The use, distribution or reproduction in other forums is permitted, provided the original author(s) and the copyright owner(s) are credited and that the original publication in this journal is cited, in accordance with accepted academic practice. No use, distribution or reproduction is permitted which does not comply with these terms.
*Correspondence: Xiaoxia Lu, bHVzaTc0QDE2My5jb20=; Jianmu Li, NTgzOTk5ODQ2QHFxLmNvbQ==; Yang Liu, d2h5eHp5eWVrQDE2My5jb20=; Yabin Wu, MzczMDI5MTA2QHFxLmNvbQ==
†These authors have contributed equally to this work and share first authorship
Disclaimer: All claims expressed in this article are solely those of the authors and do not necessarily represent those of their affiliated organizations, or those of the publisher, the editors and the reviewers. Any product that may be evaluated in this article or claim that may be made by its manufacturer is not guaranteed or endorsed by the publisher.
Research integrity at Frontiers
Learn more about the work of our research integrity team to safeguard the quality of each article we publish.