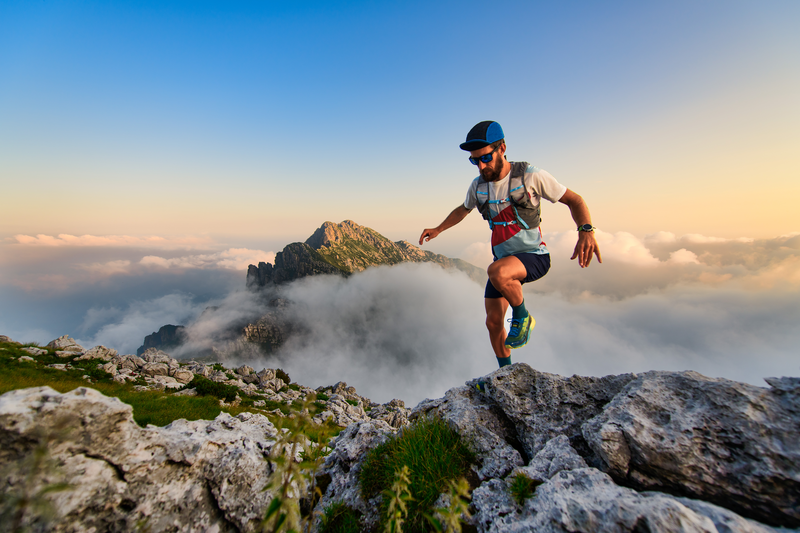
95% of researchers rate our articles as excellent or good
Learn more about the work of our research integrity team to safeguard the quality of each article we publish.
Find out more
ORIGINAL RESEARCH article
Front. Pediatr. , 15 July 2024
Sec. Pediatric Infectious Diseases
Volume 12 - 2024 | https://doi.org/10.3389/fped.2024.1383748
Introduction: Pneumococcal conjugate vaccines (PCVs), including higher valency vaccines such as PCV20, have the potential to reduce pediatric otitis media. We assessed serotype distribution, potential PCV coverage, and antimicrobial susceptibility of Streptococcus pneumoniae isolates cultured from middle ear fluid (MEF) of US children age ≤5 years.
Methods: S. pneumoniae isolates identified from US hospitals participating in the SENTRY Antimicrobial Surveillance program from 2011 to 2021 were included. Serotypes were determined by in silico analysis based on Pneumococcal Capsular Typing methodology. The percentage of isolates belonging to serotypes included in PCV13 (serotypes 1, 3, 4, 5, 6A, 6B, 7F, 9V, 14, 18C, 19A, 19F, 23F), PCV15 (PCV13 plus 22F, 33F), and PCV20 (PCV13 plus, 8, 10A, 11A, 12F, 15B, 22F and 33F) was calculated. Antimicrobial susceptibility testing was performed by broth microdilution and interpreted using CLSI criteria. Nonsusceptibility was defined as isolates that were intermediate or resistant to a selected antimicrobial.
Results: Among the 199 S. pneumoniae isolates that were identified, 56.8% were from children age <2 years. Six serotypes accounted for around 60% of isolates: 35B (16.6%), 15B (14.6%), 15A (7.5%), 19A (7.5%), 19F (7.5%), and 3 (7.0%). Serotypes included in PCV13, PCV15, and PCV20 accounted for 23.1%, 30.2%, and 54.8% of isolates, respectively. Overall, 45.2% of isolates were penicillin non-susceptible, and 13.6% were MDR, of which 48% were serotype 19A. Seven serotypes (19A, 15A, 15B, 15C, 23A, 33F, and 35B) accounted for the majority of non-susceptible isolates.
Discussion: PCVs, particularly PCV20, may prevent a substantial fraction of S. pneumoniae otitis media (OM), including OM due to non-susceptible serotypes. The addition of serotypes 15A, 23A, and 35B would improve coverage against susceptible and non-susceptible pneumococcal OM.
Streptococcus pneumoniae is one of the most common bacterial pathogens causing otitis media (OM). In 2000, a 7-valent pneumococcal conjugate vaccine (PCV7) was licensed and incorporated into the pediatric national immunization program (NIP) in the US, which was replaced by the 13-valent pneumococcal conjugate vaccine (PCV13) in 2010 (1). Among US children born between 2017 and 2018, 82.3% had received four or more doses of PCV13 by age 24 months (2). Since the introduction of pneumococcal conjugate vaccines (PCV) into the pediatric population, there has been a substantial reduction of vaccine serotype OM among children (3–6). However, despite the success of PCVs, OM disease burden remains substantial (7). Further, a greater proportion of pneumococcal OM cases due to non-PCV13 vaccine serotypes have been reported in the US in recent years (8, 9).
The emergence of non-PCV13 vaccine serotypes has led to the development and recommendation of higher-valency PCVs, including a 15-valent PCV (10) and a 20-valent PCV (11). Both vaccines have been approved for use among US children and indicated for prevention of invasive pneumococcal disease (IPD) due to the serotypes contained in the vaccines (10, 11). PCV20 is also indicated for prevention of OM caused by the original seven serotypes covered by PCV7 (11). Decisions pertaining to recent recommendations for pediatric PCV use have largely been informed by data from IPD whereas data from OM has been limited (12). While nasopharyngeal samples collected from children with OM have been used to approximate OM serotype distribution (13, 14), middle ear fluid (MEF) is still considered the gold standard specimen for detection and characterization of the causative OM agents.
The objective of this study was to assess the pneumococcal serotype distribution and antimicrobial susceptibility including multidrug resistance (MDR), and potential PCV coverage among isolates cultured from MEF obtained from children in the US.
The SENTRY Antimicrobial Surveillance Program was established in 1997 to monitor pathogens and changes in antimicrobial resistance patterns of organisms collected from patients with various infections (15). Every year, participating laboratories at medical centers in all 9 US Census Divisions identify requested surveillance pathogens by routine microbiologic methods and submit a subculture to the SENTRY program, along with basic demographic information about the case patient and limited information about the clinical setting where the case patient was treated. Pathogen confirmation is conducted by the central laboratory (JMI Laboratories, North Liberty, Iowa) by various techniques including colony morphology, biochemical algorithms, MALDI-TOF MS, PCR assays, and/or sequencing, as needed. From the SENTRY program collection of stored pathogens, S. pneumoniae isolates cultured from MEF samples from children 5 years of age or younger that were submitted from 35 participant laboratories during 2011–2021 were included in this study. Because tympanocentesis is not routinely performed among children with OM in the US and collection technique was not reported, we assumed that the MEF had been collected from a tympanocentesis performed on children with complicated OM (children with recurrent OM, children with a poor response to conventional therapy or children with a clinical relapse) or with a swab from otorrhea from a spontaneously perforated OM (16–18). We restricted serotyping to S. pneumoniae isolates collected from 2011 through 2021, after introduction of PCV13 into the US pediatric immunization program.
Serotypes were identified using in silico analysis based on Pneumococcal Capsular Typing methodology (PneumoCaT). Specifically, DNA from S. pneumoniae isolates was extracted using the KingFisher Cell and Tissue DNA kit on a KingFisher™ Flex Magnetic Particle Processor (Thermo Scientific) workstation. Total genomic DNA was used as input material for library construction and sequencing on a NextSeq 1000 Sequencer (Illumina, San Diego, California, USA) using NextSeq™1000/2000 P2 Reagents (300 cycles). The DNA libraries for the NextSeq1000 Sequencer were prepared using the Illumina DNA™ library construction protocol and index kit. Rigorous quality control metrics were applied to library construction including verification that the “Quality Score” (% ≥Q30) for the run was above 75%, the percentage of clusters passing filter was ≥60%, and the loading concentration was ≥95. Each raw data set was de novo assembled using SPAdes 3.11.1. PneumoCaT (v. 1.2.1) used a two-step approach to assign capsular type to S. pneumoniae genomic data. In the first step, if the reads matched >90% to one or more of the 92 serotypes for S. pneumoniae plus 2 additional subtypes/molecular types, a capsular type was assigned. If more than one loci matched, then in the second step, a variant-based approach that utilizes the capsular type variant database to distinguish serotypes within a subgroup/genogroup was applied to make a call on the serotype. If the coverage value against the reference sequence was ≤90%, then the serotype analysis reported “Failed” as its value. Average depth of coverage across the matching region of ≥30X was considered acceptable for PneumoCaT to analyze the data (19, 20). The nucleotide sequences used to assign a serotype were submitted to the National Center for Biotechnology Information (NCBI) and were assigned accession numbers SAMN41612263 - SAMN41612461.
Minimum Inhibitory Concentrations (MICs) were determined by the broth microdilution method using Clinical and Laboratory Standards Institute (CLSI) guidelines (2018) (21). Reference broth microdilution panels were manufactured at JMI Laboratories (2015–2021) or purchased from Thermo Fisher Scientific (2011–2014) (Cleveland, Ohio, USA) using freshly prepared drug stocks and stored at −80°C until use. Testing was performed in cation-adjusted Mueller-Hinton broth supplemented with 2.5% to 5.0% lysed horse blood (MHB-LHB). Antimicrobial susceptibility testing was performed for amoxicillin-clavulanic acid, penicillin, ceftriaxone, clindamycin, erythromycin, trimethoprim/sulfamethoxazole (TMP/SMX), levofloxacin, and vancomycin. MIC results were interpreted as susceptible, intermediate, or resistant according to the CLSI recommendations (22). Non-meningitis breakpoints were used for penicillin and ceftriaxone. Non-susceptible isolates were defined as those isolates that were intermediate or resistant to a selected antimicrobial agent. Multidrug resistance (MDR) was defined as resistant to three or more classes of antimicrobials. The MIC50 was defined as the MIC of a given antimicrobial drug that inhibited growth of 50% of isolates, and MIC90 was defined as the MIC that inhibited growth of 90% of the isolates.
Percentages of cases due to each serotype, non-susceptible to the selected antimicrobials, and covered by PCVs were calculated. PCV coverage was estimated for PCV7 (4, 6B, 9 V, 14, 18C, 19F, and 23F), PCV13 non-PCV7 (1, 3, 5, 6A, 7F, and 19A), PCV13 (PCV7 + PCV13 non-PCV7 serotypes), PCV15 (PCV13, 22F, and 33F), PCV15 non-PCV13 (22F and 33F), PCV20 non-PCV13 (8, 10A, 11A, 12F, 15B, 22F, and 33F), PCV20 (PCV13, PCV20 non-PCV13 serotypes), and non-PCV20 (serotypes not covered by PCV20). While serotypes 6C and 15C are not included in any PCV formulations, we also present PCV coverage estimates where these were grouped with PCVs that contained conjugates 6A or 15B due to potential prevention based on immunological or epidemiological evidence of cross-protection (23–25). Percentages were also stratified into two age groups (<2 years and 2–5 years) and two periods (to reflect potential changes associated with PCV13 introduction; Period 1 [P1: 2011–2016] and Period 2 [P2: 2017–2021]). Periods and age groups were compared for individual serotypes and PCV serotype groups by Chi-square test; p-values < 0.05 were considered significant. Non-susceptibility data were stratified by PCV group (PCV13, PCV20 non-PCV13, PCV15 non-PCV13, Non-PCV20). Analyses were performed using STATA (26).
A total of 199 S. pneumoniae isolates from middle ear fluid among children 5 years of age or younger were collected by the SENTRY program from 2011 to 2021. All 199 S. pneumoniae were serotyped (by sequencing) and tested for antimicrobial susceptibility (Table 1). Of these, 113 isolates (56.8%) were from children <2 years of age. Most isolates were collected from children receiving care in the ear, nose, and throat (ENT; n = 89, 44.7%) or pediatrics (n = 61, 30.7%) departments. Approximately 60% of isolates came from male children, and about 50% were isolated between 2017 and 2021. While isolates were reported from all 9 US Census regions, most (57.3%) originated from the West North Central region.
Among the 199 S. pneumoniae isolates that were serotyped, twenty-three unique serotypes were identified. Roughly 60% of isolates were represented by 6 serotypes: 35B (16.6%), 15B (14.6%), 15A (7.5%), 19A (7.5%), 19F (7.5%), and 3 (7.0%) (Figure 1). Serotype 3 represented a higher percentage of total isolates among children 2–5 years of age than among those age <2 years (12.8% vs. 2.7%, respectively; p-value = 0.01), whereas serotype 15B represented a smaller percentage in older children (9.3% vs. 18.6%, respectively; p-value = 0.06). Comparing study periods, serotypes 35B and 15B represented the highest percentage of detected serotypes in P1 and P2, respectively (Figure 2 and Supplementary Table S1) whereas the percentage due to serotype 19A was higher in P1 than in P2 (10.8% vs. 4.1%, respectively; p-value = 0.08) and serotype 19F was higher in P2 than in P1 (11.3% vs. 3.9%, respectively; p-value = 0.048; Figure 2 and Supplementary Table S1). Serotype distribution by age group and study period are presented in Supplementary Table S1.
Figure 1 Distribution of S. pneumoniae serotypes isolated from MEF samples among children, by age group. Serotypes included in each PCV are: PCV7 serotypes = 4, 6B, 9V, 14, 18C, 19F, 23F; PCV13 serotypes = PCV7 serotypes and 1, 3, 5, 6A, 7F, 19A; PCV15 serotypes = PCV13 serotypes and 22F and 33F; PCV20 serotypes = PCV13 serotypes and 8, 10A, 11A, 12F, 15B, 22F, 33F.
Figure 2 Distribution of S. pneumoniae serotypes isolated from MEF samples among children ≤5 years, by study period. Serotypes included in each PCV are: PCV7 serotypes = 4, 6B, 9V, 14, 18C, 19F, 23F; PCV13 serotypes = PCV7 serotypes and 1, 3, 5, 6A, 7F, 19A; PCV15 serotypes = PCV13 serotypes and 22F and 33F; PCV20 serotypes = PCV13 serotypes and 8, 10A, 11A, 12F, 15B, 22F, 33F.
In children aged ≤5 years, PCV13, PCV15, and PCV20 serotypes accounted for 23.1%, 30.2%, and 54.8% of S. pneumoniae isolates, respectively and 45.2% of cases were due to Non-PCV20 serotypes (Table 2). The PCV20 non-PCV13 serotypes and PCV15 non-PCV13 serotypes contributed 31.7% and 7.5% of cases, respectively (Table 2). Considering the age group, while PCV20 coverage was similar for children in both age groups, PCV20 non-PCV13 coverage was higher for children <2 years than in children 2–5 years of age (37.2% vs. 20.9%, respectively; p-value = 0.01) and this was due to a larger percentage of serotype 15B isolates detected in the younger age group. Overall, the percentage of serotypes included in PCV20 was higher in P2 than P1 (57.7% vs. 48.0%, respectively; p-value = 0.17) as was also true for PCV20 non-PCV13 serotypes (35.1% vs. 25.5%, respectively; p-value = 0.14) (Table 2). Serotype distribution by age group and study period are presented in Supplementary Table S2.
Table 2 Potential PCV serotype group coverage of S. pneumoniae isolated from MEF by age group (<2 years and 2–5 years) and study period (P1: 2011–2016 and P2: 2017–2021).
Non-susceptibility to penicillin, erythromycin, and TMP/SMX was common overall (45.2%, 49.8%, and 37.4%, respectively), but only 9.6% for amoxicillin-clavulanic acid (Table 3). Overall, 13.6% (n = 27) of isolates were MDR; most were PCV13 serotypes (n = 15) (Table 3). The proportions of non-susceptible isolates were similar across time periods for these antimicrobials, except for PCV13, for which proportions were lower in P2 than in P1 (Supplementary Table S3). Non-susceptibility to at least one antimicrobial was observed among 17 of the 23 serotypes identified. The majority of non-susceptibility was accounted for by serotypes 19A, 15A, 15B, 15C, 23A, 33F, and 35B (Table 4). More than 50% of serotype 19A isolates were non-susceptible to all antimicrobials tested, and 87% (n = 13) were MDR.
Table 3 Antimicrobial susceptibility of S. pneumoniae isolated from MEF obtained from children ≤5 years, 2011–2021.
Table 4 Antimicrobial nonsusceptibility of S. pneumoniae serotypes isolated from MEF from children ≤5 years, 2011–2021.
In this study we assessed the serotype distribution, potential coverage by available pneumococcal vaccines, and antimicrobial susceptibility for pneumococcal isolates cultured from the MEF of US children with OM. Our results showed that more than half of S. pneumoniae isolates were serotypes covered by PCV20 (52.8%), 30.2% were covered by PCV15, and 22.6% were covered by PCV13. Serotypes 35B (16.6%) and 15B (14.6%) were the most common serotypes overall followed by serotypes 15A, 3, 19A, and 19F, each accounting for approximately 7% of cases. Together, these serotypes accounted for nearly two-thirds (61%) of pneumococcal isolates identified from MEF samples.
Other studies from US and European children that cultured and serotyped S. pneumoniae from MEF also reported that these serotypes were commonly identified. In these studies, and ours, the most common PCV13 serotypes collected from MEF were serotypes 3, 19A, and 19F (27–30). In the two US studies conducted during the PCV13 period among children with intact or perforated OM, serotypes 15A, 15B/C, 23B, and 35B also were among the most commonly identified (27, 29). In German and French children, serotypes 15B/C, 23B, and 35B, but not 15A as in our study, were among the most common serotypes detected in MEF. In addition, serotypes 10A, 11A, 16F, 24F were also common among French children only (28, 30).
Several explanations could account for these aggregate results. For PCV13 serotypes 3, 19A, and 19F, pre-licensure immunogenicity studies demonstrated that PCV13 elicited a robust immune response and functional antibody activity for each of these serotypes, particularly after the booster dose (31). Post-licensure studies reported that following PCV introduction population-based reductions (i.e., considering the combination of direct and indirect protection) declined significantly due to serotypes 19A and 19F and also for serotype 3, albeit not significantly (32), while PCV13 protected against each of these three serotypes in Israel (3). These data suggest that residual disease due to these serotypes could be attributed to lower effectiveness against carriage, including potentially against carriage acquisition, density, or duration of the immune response (33–36). For example, in our study, serotype 3 was more common among children 2–5 years (12.8%) than children <2 years of age (2.7%), which could reflect a shorter duration of immunity for serotype 3 (37). Alternatively, residual disease due to these serotypes could also be due to a lower amount of antibody present in the MEF or with a capsular phenotype of some serotypes (e.g., serotype 3) that facilitates progression to disease (38).
For the most common non-PCV13 serotypes identified in this study (15A, 15B, 15C, 23B, 35B), not only have they become increasingly common colonizers over subsequent eras of PCV use, but they have also been frequently associated with antimicrobial nonsusceptibility, especially serotypes 15A and 35B (9, 27, 39). Serotype 35B has also become both a more common invasive serotype and acquired multiclass non-susceptibility among US children with IPD (40). The emergence of these non-susceptible strains likely results from selection pressure created by exposure to antimicrobials (41). Strains possessing both non-susceptibility and virulence genes may become more common causes of systemic and mucosal disease as was observed among certain non-vaccine serotypes after PCV7 introduction (40, 42).
Overall, a substantial proportion of isolates in each PCV serotype group was non-susceptible to at least one antimicrobial. While non-susceptibility was seen for at least one isolate for most of the 23 serotypes identified, most non-susceptible isolates were accounted for by 7 serotypes: 19A, 15A, 15B, 15C, 23A, 33F, and 35B, most of which were also reported by other studies (9, 40). We also observed a decrease in the proportion of non-susceptible and MDR PCV13 serotypes over the study time periods, primarily driven by the decrease of serotype 19A, as has been reported previously (40, 43). PCV15 includes serotypes 19A and 33F and PCV20 also includes serotypes 15B and possibly 15C through cross protection (24), with the latter potentially covering 39% of penicillin non-susceptible isolates. Serotypes 15A, 23A, and 35B are not currently covered by any higher valency PCVs, and while disease incidence for these serotypes has not increased, non-susceptibility to antimicrobials among these serotypes is common, which makes them important candidates to include in future higher valency PCVs (40). Importantly, serotype inclusion and PCV coverage estimates may not linearly translate to protection due to immune interference and the possible need for higher levels of antibody to prevent mucosal disease (36).
Our study had limitations. Because the source of many S. pneumoniae isolates was presumably from children with spontaneously perforated OM, the serotype distribution of these cases may not be representative of all OM cases. Specifically, the majority of children were seen in the ENT or pediatrics departments which could be indicative of prior antibiotic treatment failure. Clinical data such as previous use of antimicrobials and vaccine history were not available, and therefore, it was not possible to associate S. pneumoniae isolation with therapeutic failure or vaccine breakthrough infection. Also, the SENTRY program is a laboratory-based surveillance system designed to observe distribution of pathogens and antimicrobial resistance patterns for any given infection based on a prespecified target number of pathogens per year. Therefore, the true prevalence of S. pneumoniae serotypes cannot be ascertained with these sampling criteria. However, this study demonstrates that antimicrobial resistance surveillance systems can be leveraged to understand serotype distribution of pneumococcal isolates from MEF samples.
Our study showed that certain PCV13 serotypes – 3, 19A, and 19F – are frequently isolated from MEF of children even in the context of a mature pediatric PCV13 program, emphasizing the need for continued monitoring following the introduction of higher valency vaccines such as PCV15 and PCV20. Furthermore, among the pneumococcal isolates in this study, an important fraction were attributed to serotypes beyond those in PCV13 that are covered by higher valency PCVs. Among the additional serotypes in PCV20 beyond PCV13, several were associated with antimicrobial non-susceptibility and MDR. Therefore, including PCV20 in existing pediatric national immunization programs may further reduce the frequency of overall and antimicrobial non-susceptible S. pneumoniae. With the introduction of higher valency PCVs, further studies are needed to monitor the changes in pneumococcal epidemiology in children with OM in the long term. Future PCVs should consider including non-PCV20 serotypes, particularly 15A, 23A, and 35B, to further reduce the burden of OM among children.
The raw data supporting the conclusions of this article will be made available by the authors, without undue reservation.
LG: Conceptualization, Data curation, Formal Analysis, Funding acquisition, Methodology, Project administration, Writing – original draft, Writing – review & editing. KA: Formal Analysis, Project administration, Writing – original draft. LD: Data curation, Formal Analysis, Project administration, Writing – review & editing. JK: Data curation, Formal Analysis, Project administration, Writing – review & editing. KH: Conceptualization, Data curation, Formal Analysis, Methodology, Writing – review & editing. QY: Data curation, Project administration, Writing – original draft, Writing – review & editing. RM: Data curation, Formal Analysis, Project administration, Writing – review & editing. AC: Conceptualization, Writing – review & editing. BG: Conceptualization, Funding acquisition, Writing – review & editing. AA: Conceptualization, Data curation, Formal Analysis, Funding acquisition, Methodology, Project administration, Writing – review & editing.
The author(s) declare financial support was received for the research, authorship, and/or publication of this article. The serotyping of S. pneumoniae isolates was funded by Pfizer Inc. The funder was not involved in the study design, analysis, interpretation of data, the writing of this article or the decision to submit it for publication.
LD, JK, and RM are employees of JMI, which is contracted by Pfizer and other companies to provide surveillance data on pathogen characteristics including antimicrobial resistance. LG, KA, KH, QY, AC, BG, and AA are employees of Pfizer and may own stock or stock options.
All claims expressed in this article are solely those of the authors and do not necessarily represent those of their affiliated organizations, or those of the publisher, the editors and the reviewers. Any product that may be evaluated in this article, or claim that may be made by its manufacturer, is not guaranteed or endorsed by the publisher.
The Supplementary Material for this article can be found online at: https://www.frontiersin.org/articles/10.3389/fped.2024.1383748/full#supplementary-material
1. Centers for Disease Control and Prevention. Licensure of a 13-valent pneumococcal conjugate vaccine (Pcv13) and recommendations for use among children - advisory committee on immunization practices (acip), 2010. MMWR Morb Mortal Wkly Rep. (2010) 59:258–61.20224542
2. Hill HA, Singleton JA, Yankey D, Elam-Evans LD, Pingali SC, Kang Y. Vaccination coverage by age 24 months among children born in 2017 and 2018-national immunization survey-child, United States, 2018–2020. MMWR Morb Mortal Wkly R. (2021) 70(41):1435–40. doi: 10.15585/mmwr.mm7041a1
3. Dagan R, Van Der Beek BA, Ben-Shimol S, Pilishvili T, Givon-Lavi N. Effectiveness of the 7- and 13-valent pneumococcal conjugate vaccines against vaccine-serotype otitis media. Clin Infect Dis. (2021) 73(4):650–8. doi: 10.1093/cid/ciab066
4. Marra LP, Sartori AL, Martinez-Silveira MS, Toscano CM, Andrade AL. Effectiveness of pneumococcal vaccines on otitis media in children: a systematic review. Value Health. (2022) 25(6):1042–56. doi: 10.1016/j.jval.2021.12.012
5. Pichichero M, Kaur R, Scott DA, Gruber WC, Trammel J, Almudevar A, et al. Effectiveness of 13-valent pneumococcal conjugate vaccination for protection against acute otitis media caused by Streptococcus pneumoniae in healthy young children: a prospective observational study. Lancet Child Adolesc Health. (2018) 2(8):561–8. doi: 10.1016/S2352-4642(18)30168-8
6. Suaya JA, Gessner BD, Fung S, Vuocolo S, Scaife J, Swerdlow DL, et al. Acute otitis media, antimicrobial prescriptions, and medical expenses among children in the United States during 2011–2016. Vaccine. (2018) 36(49):7479–86. doi: 10.1016/j.vaccine.2018.10.060
7. Hu TY, Done N, Petigara T, Mohanty S, Song Y, Liu Q, et al. Incidence of acute otitis media in children in the United States before and after the introduction of 7-and 13-valent pneumococcal conjugate vaccines during 1998–2018. Bmc Infect Dis. (2022) 22(1):294. doi: 10.1186/s12879-022-07275-9
8. Kaur R, Morris M, Pichichero ME. Epidemiology of acute otitis media in the postpneumococcal conjugate vaccine era. Pediatrics. (2017) 140(3):e20170181. doi: 10.1542/peds.2017-0181
9. Kaur R, Pham M, Yu KOA, Pichichero ME. Rising pneumococcal antibiotic resistance in the post-13-valent pneumococcal conjugate vaccine era in pediatric isolates from a primary care setting. Clin Infect Dis. (2021) 72(5):797–805. doi: 10.1093/cid/ciaa157
10. LLC MSD. Vaxneuvance [Package Insert] (2023). (February 2, 2024). Available online at: https://www.fda.gov/media/150819/download (Accessed February 2, 2024).
11. Pharmaceuticals W. Prevnar 20 [Package Insert] (2023). (February 2, 2024). Available online at: https://www.fda.gov/media/149987/download?attachment (Accessed February 2, 2024).
12. Gierke R. Current Epidemiology of Pediatric Pneumococcal Disease, United States (2023). (February 2, 2024). Available online at: https://www.cdc.gov/vaccines/acip/meetings/downloads/slides-2023-02/slides-02-22/pneumococcal-02-gierke-508.pdf (Accessed February 2, 2024).
13. Cohen R, Levy C, Bingen E, Koskas M, Nave I, Varon E. Impact of 13-valent pneumococcal conjugate vaccine on pneumococcal nasopharyngeal carriage in children with acute otitis media. Pediatr Infect Dis J. (2012) 31(3):297–301. doi: 10.1097/INF.0b013e318247ef84
14. Kaur R, Czup K, Casey JR, Pichichero ME. Correlation of nasopharyngeal cultures prior to and at onset of acute otitis media with middle ear fluid cultures. Bmc Infect Dis. (2014) 14:640. doi: 10.1186/s12879-014-0640-y
15. Diekema DJ, Hsueh PR, Mendes RE, Pfaller MA, Rolston KV, Sader HS, et al. The microbiology of bloodstream infection: 20-year trends from the sentry antimicrobial surveillance program. Antimicrob Agents Chemother. (2019) 63(7):e00355–19. doi: 10.1128/AAC.00355-19
16. Hoberman A, Paradise JL, Wald ER. Tympanocentesis technique revisited. Pediatr Infect Dis J. (1997) 16(2 Suppl):S25–6. doi: 10.1097/00006454-199702001-00007
17. Hoekelman RA. Do you do tympanocenteses? Pediatr Ann. (1991) 20(11):585–7. doi: 10.3928/0090-4481-19911101-05
18. Poole MD. It’s time to bring back diagnostic tympanocentesis. Ear Nose Throat J. (1994) 73(1):49–50. doi: 10.1177/014556139407300113
19. Kapatai G, Sheppard CL, Al-Shahib A, Litt DJ, Underwood AP, Harrison TG, et al. Whole genome sequencing of Streptococcus pneumoniae: development, evaluation and verification of targets for serogroup and serotype prediction using an automated pipeline. PeerJ. (2016) 4:e2477. doi: 10.7717/peerj.2477
20. Github. Readme for Pneumocat Tool. (February 2, 2024). Available online at: https://github.com/ukhsa-collaboration/PneumoCaT (Accessed February 2, 2024).
21. CLSI. Methods for Dilution Antimicrobial Susceptibility Tests for Bacteria That Grow Aerobically; Approved Standard: Eleventh Edition (2018). (February 2, 2024). Available online at: https://clsi.org/media/1928/m07ed11_sample.pdf (Accessed February 2, 2024).
22. CLSI. Performance Standards for Antimicrobial Susceptibility Testing: 31 Informational Supplement (2021) (February 2, 2024). 31 Edition. Available online at: https://clsi.org/about/press-releases/clsi-publishes-m100-performance-standards-for-antimicrobial-susceptibility-testing-31st-edition/# (Accessed February 2, 2024).
23. Andrews N, Kent A, Amin-Chowdhury Z, Sheppard C, Fry N, Ramsay M, et al. Effectiveness of the seven-valent and thirteen-valent pneumococcal conjugate vaccines in England: the indirect cohort design, 2006–2018. Vaccine. (2019) 37(32):4491–8. doi: 10.1016/j.vaccine.2019.06.071
24. Hao L, Kuttel MM, Ravenscroft N, Thompson A, Prasad AK, Gangolli S, et al. Streptococcus pneumoniae serotype 15B polysaccharide conjugate elicits a cross-functional immune response against serotype 15C but not 15A. Vaccine. (2022) 40(33):4872–80. doi: 10.1016/j.vaccine.2022.06.041
25. Savulescu C, Krizova P, Valentiner-Branth P, Ladhani S, Rinta-Kokko H, Levy C, et al. Effectiveness of 10 and 13-valent pneumococcal conjugate vaccines against invasive pneumococcal disease in European children: spidnet observational multicentre study. Vaccine. (2022) 40(29):3963–74. doi: 10.1016/j.vaccine.2022.05.011
26. LLC S. Stata User’s Guide: Release 18 (2023). Available online at: https://www.stata.com/manuals/u.pdf (cited Version: 18).
27. Hulten KG, Lin PL, Bradley JS, Peters TR, Tan TQ, Romero JR, et al. 1373. Vaccine effectiveness and pneumococcal serotypes in pediatric Otitis Media in the era of routine 13-valent pneumococcal vaccination in the United States. Presented at IDweek 2020. Abstract #1373; Date: 21–25 October 2020
28. Imöhl M, Perniciaro S, Busse A, van der Linden M. Bacterial spectrum of spontaneously ruptured otitis media in a 7-year, longitudinal, multicenter, epidemiological cross-sectional study in Germany. Front Med-Lausanne. (2021) 8:675225. doi: 10.3389/fmed.2021.675225
29. Kaur R, Fuji N, Pichichero ME. Dynamic changes in otopathogens colonizing the nasopharynx and causing acute otitis media in children after 13-valent (PCV13) pneumococcal conjugate vaccination during 2015–2019. Eur J Clin Microbiol Infect Dis. (2022) 41(1):37–44. doi: 10.1007/s10096-021-04324-0
30. Levy C, Varon E, Ouldali N, Wollner A, Thollot F, Corrard F, et al. Bacterial causes of otitis media with spontaneous perforation of the tympanic membrane in the era of 13 valent pneumococcal conjugate vaccine. Plos One. (2019) 14(2):e0211712. doi: 10.1371/journal.pone.0211712
31. Yeh SH, Gurtman A, Hurley DC, Block SL, Schwartz RH, Patterson S, et al. Immunogenicity and safety of 13-valent pneumococcal conjugate vaccine in infants and toddlers. Pediatrics. (2010) 126(3):E493–505. doi: 10.1542/peds.2009-3027
32. Ben-Shimol S, Givon-Lavi N, Leibovitz E, Raiz S, Greenberg D, Dagan R. Near-elimination of otitis media caused by 13-valent pneumococcal conjugate vaccine (PCV) serotypes in southern Israel shortly after sequential Introduction of 7-valent/13-valent PCV. Clin Infect Dis. (2014) 59(12):1724–32. doi: 10.1093/cid/ciu683
33. Dagan R, Patterson S, Juergens C, Greenberg D, Givon-Lavi N, Porat N, et al. Comparative immunogenicity and efficacy of 13-valent and 7-valent pneumococcal conjugate vaccines in reducing nasopharyngeal colonization: a randomized double-blind trial. Clin Infect Dis. (2013) 57(7):952–62. doi: 10.1093/cid/cit428
34. Deceuninck G, Brousseau N, Lefebvre B, Quach C, Tapiero B, Bui YG, et al. Effectiveness of thirteen-valent pneumococcal conjugate vaccine to prevent serotype 3 invasive pneumococcal disease in Quebec in children, Canada. Vaccine. (2023) 41(38):5486–9. doi: 10.1016/j.vaccine.2023.07.049
35. Jokinen JT, Åhman H, Kilpi TM, Mäkelä PH, Käyhty MH. Concentration of antipneumococcal antibodies as a serological correlate of protection: an application to acute otitis media. J Infect Dis. (2004) 190(3):545–50. doi: 10.1086/422531
36. Millar EV, O'Brien KL, Bronsdon MA, Madore D, Hackell JG, Reid R, et al. Anticapsular serum antibody concentration and protection against pneumococcal colonization among children vaccinated with 7-valent pneumococcal conjugate vaccine. Clin Infect Dis. (2007) 44(9):1173–9. doi: 10.1086/513199
37. Fuji N, Pham M, Kaur R, Pichichero ME. Serotype 3 antibody response and antibody functionality compared to serotype 19A following 13-valent pneumococcal conjugate immunization in children. Pediatr Infect Dis J. (2024) 43(3):294–300. doi: 10.1097/INF.0000000000004192
38. Luck JN, Tettelin H, Orihuela CJ. Sugar-coated killer: serotype 3 pneumococcal disease. Front Cell Infect Mi. (2020) 10:613287. doi: 10.3389/fcimb.2020.613287
39. Rybak A, Levy C, Ouldali N, Bonacorsi S, Bechet S, Delobbe JF, et al. Dynamics of antibiotic resistance of Streptococcus pneumoniae in France: a pediatric prospective nasopharyngeal carriage study from 2001 to 2022. Antibiotics (Basel). (2023) 12(6):1020. doi: 10.3390/antibiotics12061020
40. Bajema KL, Gierke R, Farley MM, Schaffner W, Thomas A, Reingold AL, et al. Impact of pneumococcal conjugate vaccines on antibiotic-nonsusceptible invasive pneumococcal disease in the United States. J Infect Dis. (2022) 226(2):342–51. doi: 10.1093/infdis/jiac154
41. Lattar SM, Wu XQ, Brophy J, Sakai F, Klugman KP, Vidal JE. A mechanism of unidirectional transformation, leading to antibiotic resistance, occurs within nasopharyngeal pneumococcal biofilm consortia. Mbio. (2018) 9(3):e00561–18. doi: 10.1128/mBio.00561-18
42. Farrell DJ, Klugman KP, Pichichero M. Increased antimicrobial resistance among nonvaccine serotypes of Streptococcus pneumoniae in the pediatric population after the Introduction of 7-valent pneumococcal vaccine in the United States. Pediatr Infect Dis J. (2007) 26(2):123–8. doi: 10.1097/01.inf.0000253059.84602.c3
Keywords: otitis media, Streptococcus pneumoniae, pneumococcal conjugate vaccine, serotype distribution, antimicrobial susceptibility
Citation: Grant LR, Apodaca K, Deshpande L, Kimbrough JH, Hayford K, Yan Q, Mendes R, Cané A, Gessner BD and Arguedas A (2024) Characterization of Streptococcus pneumoniae isolates obtained from the middle ear fluid of US children, 2011–2021. Front. Pediatr. 12: 1383748. doi: 10.3389/fped.2024.1383748
Received: 7 February 2024; Accepted: 17 June 2024;
Published: 15 July 2024.
Edited by:
Ana Friães, University of Lisbon, PortugalReviewed by:
Lucía Fernández-Delgado, Bellvitge University Hospital, Spain© 2024 Grant, Apodaca, Deshpande, Kimbrough, Hayford, Yan, Mendes, Cané, Gessner and Arguedas. This is an open-access article distributed under the terms of the Creative Commons Attribution License (CC BY). The use, distribution or reproduction in other forums is permitted, provided the original author(s) and the copyright owner(s) are credited and that the original publication in this journal is cited, in accordance with accepted academic practice. No use, distribution or reproduction is permitted which does not comply with these terms.
*Correspondence: Lindsay R. Grant, bGluZHNheS5ncmFudEBwZml6ZXIuY29t
Disclaimer: All claims expressed in this article are solely those of the authors and do not necessarily represent those of their affiliated organizations, or those of the publisher, the editors and the reviewers. Any product that may be evaluated in this article or claim that may be made by its manufacturer is not guaranteed or endorsed by the publisher.
Research integrity at Frontiers
Learn more about the work of our research integrity team to safeguard the quality of each article we publish.