- Department of Neonatology, Xinhua Hospital Affiliated to Shanghai Jiaotong University School of Medicine, Shanghai, China
We will discuss a recent case of unexplained neonatal cyanosis, evaluate its origin, clinical presentation, diagnosis, and treatment, and share with you some of our clinical insights. We report a transient cyanosis in a newborn due to a mutation in the globulin gene (HBG2), as well as diagnosis and treatment. Clinically, the infant was in good overall health, and despite low oxygen saturation, the arterial oxygen partial pressure was always normal. Early respiratory support includes mechanical ventilation, nasal tube oxygen, and eventually stopping oxygen therapy. With the above treatment measures, the blood oxygen saturation of the child always fluctuated at 85%, but the arterial blood oxygen partial pressure was up to 306 mmHg. Further improvement of laboratory tests revealed elevated methemoglobin levels, reticulocytosis, mild anemia, and basically normal on chest x-ray and echocardiography. To clarify the etiology, WES testing was performed. The results showed heterozygous variation in HBG2 gene (c.190C>T. p.H64Y). There is heterozygous variation at this site in the proband father, and no variation at this site in the proband mother. Given the age of the affected infants, we hypothesized that the mutation originated in the gamma peptide chain of the head protein. The baby was discharged from the hospital 10 days after birth, with blood oxygen saturation fluctuating around 90%. The cyanosis disappeared 2 months after discharge, and the blood oxygen saturation level returned to normal.
Introduction
Neonatal cyanosis can be caused by mutations in the bead protein gene. In neonates with a mutation in the peptide gene, cyanosis is usually present in good general condition. The clinical features are consistently normal arterial partial pressure of oxygen despite hypoxemia and no combined pulmonary or cardiac disease. By summarizing the clinical features of transient neonatal cyanosis, we emphasize the role of genetic testing in the diagnosis and management of the disease, improve help in preventing misdiagnosis or missed diagnosis, and recommend genetic counseling for families with a relevant family history.
Case report
She was a baby girl born by cesarean section at 37 5/7 weeks gestation. The APGAR score was 8 and 9 at 1 and 5 min, respectively. Birth weight was 3,160 g (27%) and head circumference was 33.5 cm (27%). Physical examination found that the baby's lips were blue and the whole body was cyanosis, but the muscle strength and tension of the limbs were normal and the primitive reflex was present.
Since the child's skin was bluish after birth and did not improve after oxygen and positive pressure ventilation, the initial percutaneous oxygen saturation measured in ambient air using a pulse oximeter was 78%–88%. After immediate mask oxygen administration, tracheal intubation and pure oxygen supply, the blood oxygen saturation of the child still fluctuated around 85%. The auxiliary examination showed no obvious abnormality in electrocardiogram, echocardiogram, and chest x-ray. Subsequently, the child was extubated and changed to nasal catheter oxygen inhalation until oxygen was stopped. The blood oxygen saturation fluctuated between 80% and 85%, and the arterial blood PaO2 reached a maximum of 306.7 mmHg. Laboratory tests showed a mild elevation of methemoglobin, mild anemia and reticulocytosis. We use vitamin C as a reductase enzyme to reduce the methemoglobin to hemoglobin and relieve cyanosis. We also infused red blood cell suspension to improve the anemia. The anemia was corrected and the methemoglobin level returned to normal, but the oxygen saturation still fluctuated around 90%. Clinical features are shown in Table 1. Whole-exome sequencing revealed a heterozygous mutation (c.190C>T p.H64Y) in the patient's HBG2 gene, which originated from the father but not the mother. (Laboratory: Beijing Maikino Medical Laboratory; Test: WES013: Trios). Figure 1 depicts a schematic illustration of a hemoglobin mutation. The case is discharged after 10 days and has a blood oxygen saturation of about 90%. The baby's cyanosis goes away in around two months, followed by physical health. The proband's father denied having experienced transient neonatal cyanosis.
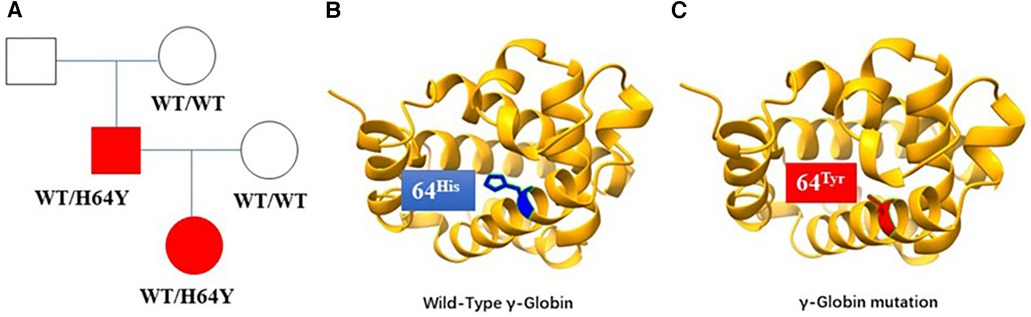
Figure 1. (A) Gamma-haemoglobin mutation lineages, with squares representing male members, circles representing female members, and shadows representing the case and her proband's father. (B) The schematic diagram of the wild-type structure of haemoglobin. (C) The predicted structure of the haemoglobin mutation in the patient. The heterozygous mutation of nucleotide 190 from cytosine C to thymine T (c.190C>T) resulted in the change of amino acid 64 from histidine to tyrosine.
Discussion
Neonatal cyanosis can be caused by a variety of genetic and acquired causes, and mutations in the gamma-globin gene are an uncommon but important cause of neonatal cyanosis. It is clinically characterized by decreased hemoglobin oxygen saturation in infants without arterial hypoxemia and or a positive family history. We describe a case of cyanosis with neonatal onset, which developed immediately after birth and did not improve with oxygen therapy and intubation. However, blood gas results showed normal arterial partial pressure of oxygen, partial pressure of carbon dioxide and ph. His hemoglobin saturation fluctuated around 85%, and his arterial PaO2 reached 306.7 mmHg, and he was in good general condition. Laboratory tests showed mild anemia, mildly elevated methemoglobin, and elevated reticulocyte count; red blood cell transfusion was given to improve the anemia and vitamin C was given to lower the methemoglobin; the anemia was corrected and the methemoglobin returned to normal, but cyanosis did not improve, and the oxygen level of the child fluctuated around 85%. At the same time, auxiliary examinations showed that the echocardiogram, chest x-ray and electrocardiogram were normal.
To clarify the etiology of the disease, a WES test was performed; the results showed that the patient was heterozygous for the HBG2 gene (c.190C>T p.H64Y). This variant was initially recognized as pathogenic according to the American College of Medical Genetics and Genomics (ACMG) criteria, and the diagnosis was confirmed by the presence of a heterozygous variant in the father's locus and the absence of the variant in the mother's locus. The child was discharged from the hospital 10 days after birth with oxygen saturation fluctuating at 90%. The child was followed up until 2 months postnatally, when cyanosis disappeared and oxygen levels remained normal. WES is a relatively simple and rapid diagnostic protocol and is therefore used in clinical practice. Each hemoglobin in the human body is made up of four hemoglobin (also referred to as ferrous protoporphyrin) and a protein bead in the middle. Each hemoglobin is made up of four pyrrole subunits that form a ring with a ferrous ion in the center. Each of the four polypeptide chains in a bead protein, when connected to a hemoglobin, forms a monomer, or subunit, of hemoglobin (1). In an electrolyte solution resembling the setting in the human body, the four hemoglobin subunits can be automatically assembled into the α2β2 form. The four peptide chains that make up the bead proteins in adult and fetal hemoglobin are different; adults' hemoglobin can be consist of two α chains and two β chains, Hb A, whereas fetal hemoglobin may consist of two alpha and two gamma chains, Hb F. Fetal hemoglobin, which has a high oxygen-carrying capacity, is replaced shortly after birth by Hb A. The center of hemoglobin contains Fe2+, which can only be organized with oxygen when it binds to a porphyrin ligand; otherwise, it loses its ability to carry oxygen when it binds to cyanide or carbon monoxide. An intrinsic aspect of the protein structure has been an ancient relationship between the oxygen binding site and the multimerization interface, with surface modification dramatically lowering oxygen affinity and even conferring cooperativity. The interfaces between numerous amino acids that hold molecular complexes together typically involve sterically close, electrostatically complementary interactions (1). Here the two alpha chains combine with the gamma chain of hemoglobin (alpha-2/gamma-2), which is encoded by the HBG2 and HBG1 genes, to form fetal hemoglobin; both chains have an amino acid at codon 136, HBG1 has an alanine at codon 136, while HBG2 has a glycine. Considering the age of the sick baby, we can also surmise that the mutation comes from the γ peptide chain of the bead protein. No quantitative or functional studies of hemoglobin were performed to confirm the diagnosis of this child, and the lack of a comprehensive examination is regretted in this article. A limitation of our study is that, in this instance, we were unable to use high-performance liquid chromatography or protein electrophoresis to further test the child's hemoglobin; ordinarily, these procedures would be carried out prior to the genetic studies.
Five M-hemoglobin variants have been identified, two of which, Hb M Boston (Hisα58→Tyr) and Hb M Saskatoon (Hisα63→Tyr), have their distal histidine replaced by a tyrosine residue. In the neonatal period, hemoglobin is predominantly α- and γ-chained, whereas in the adult period it is predominantly α- and β-chained, and thus the hemoglobin chains affected by the same mutation may be different. Thus, the mutated gene in this case is consistent with Hb M Saskatoon, but the hemoglobin chain predominantly affected by the HBG mutation in the neonatal period is the γ chain. Additionally, a variety of variants mentioned in earlier literature are listed in Table 2 (2–9). The patient's hometown serves as the inspiration for the mutation name. Previous reports of hemoglobin variations causing transient newborn cyanosis include Hb F-M-Osaka [G 63(E7) His→Tyr], Hb F-M-Fort Ripley [G 92(F8) His→Tyr], Hb F Circleville [G 63(E7) His→Leu] and Hb F-M Toms River [G 67(E11) Val→Met] (2, 4, 7, 9, 10). A shift in the location between the subunits and a change in the shape of the tetramer are the outcomes of a mutation in the His of a hemoglobin's gamma subunit. Like in the instance, positions 63 and 92 of both the beta and gamma chains' amino acid residues are occupied, despite there being 39 differences between them. The imidazole group of the normal histidine residue does not form a bond with heme iron; however, the presence of the tyrosine residue results in the formation of a covalent linkage between its phenolic portion and heme iron, which stabilizes the heme iron in the Fe3+ form. In patients with the hemoglobin Toms River mutation, methionine replaces valine at E11, thereby preventing oxygen access to the iron atom in the center of the hemoglobin ring and destabilizing the hemoglobin tetramer, leading to hemolysis and anemia, and triggering cyanosis (2). When this occurs, O2 can no longer bind to Hb, and cyanosis occurs. The mutation that changed the 64th amino acid from histidine to tyrosine (p.H64Y) in the gamma-globin gene also contributed to our patient's altered hemoglobin. This mutation causes cyanosis by decreasing hemoglobin's affinity for oxygen.
In conclusion, mutations in the gamma pearl protein gene are a rare but important cause of neonatal cyanosis. Clinical clues include reduced oxygen saturation in the absence of arterial hypoxemia, a positive family history, and an otherwise healthy infant with no evidence of cardiac or pulmonary disease. Definitive diagnosis by genetic testing can help provide accurate genetic and prognostic counseling and avoid missed diagnoses and misdiagnoses.
Data availability statement
The datasets presented in this article are not readily available because to preserve participant and patient anonymity. Requests to access the datasets should be directed to the corresponding author.
Ethics statement
The studies involving humans were approved by Ethics Committee of Xinhua Hospital Affiliated to Shanghai Jiao Tong University School of Medicine. The studies were conducted in accordance with the local legislation and institutional requirements. Written informed consent for participation in this study was provided by the participants’ legal guardians/next of kin. Written informed consent was obtained from the individual(s), and minor(s)' legal guardian/next of kin, for the publication of any potentially identifiable images or data included in this article.
Author contributions
YC: Formal Analysis, Funding acquisition, Project administration, Supervision, Writing – original draft, Writing – review & editing. JL: Data curation, Supervision, Validation, Writing – original draft. JQ: Conceptualization, Methodology, Writing – review & editing.
Funding
The author(s) declare financial support was received for the research, authorship, and/or publication of this article.
Supported by grants from the National Nature Science Foundation of China (82001592).
Conflict of interest
The authors declare that the research was conducted in the absence of any commercial or financial relationships that could be construed as a potential conflict of interest.
Publisher's note
All claims expressed in this article are solely those of the authors and do not necessarily represent those of their affiliated organizations, or those of the publisher, the editors and the reviewers. Any product that may be evaluated in this article, or claim that may be made by its manufacturer, is not guaranteed or endorsed by the publisher.
References
1. Pillai AS, Chandler SA, Liu Y, Signore AV, Cortez-Romero CR, Benesch JLP, et al. Origin of complexity in haemoglobin evolution. Nature. (2020) 581:480–5. doi: 10.1038/s41586-020-2292-y
2. Crowley MA. Brief report: a hemoglobin variant associated with neonatal cyanosis and anemia (vol 364, pg 1837, 2011). N Engl J Med. (2011) 365:281. doi: 10.1056/NEJMx110055
3. Priest JR, Watterson J, Jones RT, Faassen AE, Hedlund BE. Mutant fetal hemoglobin causing cyanosis in a newborn. Pediatrics. (1989) 83(5):734–6. 2470017
4. Glader BE. Hemoglobin FM-Fort Ripley: another lesson from the neonate. Pediatrics. (1989) 83:792–3. doi: 10.1542/peds.83.5.792
5. Glader BE, Zwerdling D, Kutlar F, Kutlar A, Wilson JB, Huisman TH. Hb F-M-Osaka or alpha 2G gamma 2(63)(E7)His—-Tyr in a Caucasian male infant. Hemoglobin. (1989) 13:769–73. doi: 10.3109/03630268908998852
6. Kohli-Kumar M, Zwerdling T, Rucknagel DL. Hemoglobin F-cincinnati, alpha 2G gamma 2 41(C7) Phe–>Ser in a newborn with cyanosis. Am J Hematol. (1995) 49:43–7. doi: 10.1002/ajh.2830490108
7. Molchanova TP, Wilson JB, Gu LH, Hain RD, Chang LS, Poon AO, et al. A second observation of the fetal methemoglobin variant Hb F-M-Fort Ripley or alpha 2G gamma 2(92)(F8)His—-Tyr. Hemoglobin. (1992) 16:389–98. doi: 10.3109/03630269209005690
8. Saller E, Kohne E, Dutly F, Frischknecht H. A new (G)γ-globin variant causing low oxygen affinity: Hb F-Brugine/Feldkirch [(G)γ105(G7)Leu→His; HBG2: c.317T>A]. Hemoglobin. (2014) 38:84–7. doi: 10.3109/03630269.2013.870079
9. Dainer E, Shell R, Miller R, Atkin JF, Pastore M, et al. Neonatal cyanosis due to a novel fetal hemoglobin: Hb F-Circleville [Gγ63(E7)His→Leu, CAT>CTT]. Hemoglobin. (2008) 32(6):596–600. doi: 10.1080/03630260802507915
Keywords: neonatal cyanosis, anemia, neonatal outcome, hypoxia, whole-exome sequencing
Citation: Chen Y, Lv J and Qian J (2024) Case Report: A case report and literature review of hemoglobin variation associated with neonatal cyanosis. Front. Pediatr. 12:1334757. doi: 10.3389/fped.2024.1334757
Received: 7 November 2023; Accepted: 29 January 2024;
Published: 13 February 2024.
Edited by:
Ann Anderson Berry, University of Nebraska Medical Center, United StatesReviewed by:
Christopher Thom, Children's Hospital of Philadelphia, United StatesDarja Paro-Panjan, University Children's Hospital Ljubljana, Slovenia
© 2024 Chen, Lv and Qian. This is an open-access article distributed under the terms of the Creative Commons Attribution License (CC BY). The use, distribution or reproduction in other forums is permitted, provided the original author(s) and the copyright owner(s) are credited and that the original publication in this journal is cited, in accordance with accepted academic practice. No use, distribution or reproduction is permitted which does not comply with these terms.
*Correspondence: Yanru Chen bHVja3luYW55YW5AMTI2LmNvbQ==