- 1Department of Critical Care Medicine and Pediatrics, Universidad de La Sabana, Fundación Cardioinfantil-Instituto de Cardiología, Bogotá, Colombia
- 2Department of Anesthesia and Cardiovascular Surgery, Fundación Cardioinfantil-Instituto de Cardiología, Bogotá, Colombia
- 3Department of Critical Care Medicine and Pediatrics, Universidad Nacional de Colombia, Bogotá, Colombia
Background: In refractory respiratory failure (RF), extracorporeal membrane oxygenation (ECMO) is a salvage therapy that seeks to reduce lung injury induced by mechanical ventilation. The parameters of optimal mechanical ventilation in children during ECMO are not known. Pulmonary ventilatory management during this therapy may impact mortality. The objective of this study was to evaluate the association between ventilatory parameters in children during ECMO therapy and in-hospital mortality.
Methods: A systematic search of PubMed/MEDLINE, Embase, Cochrane, and Google Scholar from January 2013 until May 2022 (PROSPERO 450744), including studies in children with ECMO-supported RF assessing mechanical ventilation parameters, was conducted. Risk of bias was assessed using the Newcastle-Ottawa scale; heterogeneity, with absence <25% and high >75%, was assessed using I2. Sensitivity and subgroup analyses using the Mantel-Haenszel random-effects model were performed to explore the impact of methodological quality on effect size.
Results: Six studies were included. The median age was 3.4 years (IQR: 3.2–4.2). Survival in the 28-day studies was 69%. Mechanical ventilation parameters associated with higher mortality were a very low tidal volume ventilation (<4 ml/kg; OR: 4.70; 95% CI: 2.91–7.59; p < 0.01; I2: 38%), high plateau pressure (mean Dif: −0.70 95% CI: −0.18, −0.22; p < 0.01), and high driving pressure (mean Dif: −0.96 95% CI: −1.83, −0.09: p = 0.03). The inspired fraction of oxygen (p = 0.09) and end-expiratory pressure (p = 0.69) were not associated with higher mortality. Patients who survived had less multiple organ failure (p < 0.01).
Conclusion: The mechanical ventilation variables associated with higher mortality in children with ECMO-supported respiratory failure are high plateau pressures, high driving pressure and very low tidal volume ventilation. No association between mortality and other parameters of the mechanical ventilator, such as the inspired fraction of oxygen or end-expiratory pressure, was found.
Systematic Review Registration: https://www.crd.york.ac.uk/prospero/display_record.php?ID=CRD42023450744, PROSPERO 2023 (CRD42023450744).
Introduction
In refractory respiratory failure, extracorporeal membrane oxygenation (ECMO) support is an increasingly used salvage therapy (1, 2). According to the records of the Extracorporeal Life Support Organization (ELSO), in the last five years, more than 100,000 people have received ECMO support, including patients with COVID-19 (2–4). Of these, more than 13,000 have been children, with an overall survival rate of 59%, slightly higher in those receiving respiratory ECMO (69%). Children with refractory respiratory failure without ECMO have an associated mortality rate greater than 90% (5–8). These patients, during ECMO support, should simultaneously receive invasive mechanical ventilation (MV) (9). Mechanical ventilation-induced pulmonary injury (VILI) and patient-induced self-inflicted injury (P-SILI) are ventilatory support-related complications that are associated with increased morbidity and mortality in children with acute respiratory distress syndrome (PARDS) (10, 11).
Mechanical ventilation-induced pulmonary injury can be produced by global or local overdistension as well as by cyclic closure and opening of alveolar units in PARDS (12). Additionally, the heterogeneous distribution of pulmonary perfusion and the presence of patient-ventilator asynchronies may favor the appearance of P-SILI (13). Many of these consequences are related to inappropriate mechanical ventilation parameters for the patient's condition (including children with ECMO) that magnify the inflammatory response and increase endothelial permeability (14). The occurrence of VILI can be minimized by adopting lung protection strategies (15). However, optimal lung protection strategies during pediatric and neonatal ECMO are unknown. The most recent ECMO ventilatory support guidelines are based on expert opinion (6, 9, 15). There are no controlled clinical trials evaluating the efficacy and safety of ventilatory parameters in children with MV and ECMO on mortality and clinical outcomes.
The Second Pediatric Acute Lung Injury Consensus Conference (PALICC-2) recently suggested considering ECMO support in patients with a potentially reversible cause of PARDS who do not have adequate gas exchange with MV protective strategies (15). However, the optimal mechanical ventilation strategy in terms of ventilatory modes and parameters during ECMO has not yet been defined (16–18). Inspired fractions of oxygen (FI02) greater than 50% after ECMO day three have been associated with higher mortality (46% vs. 22%; p = 0.001) than in patients with lower FI02 (19). Whether other parameters in mechanical ventilation in ECMO, such as tidal volume, end-expiratory pressure (PEEP), plateau pressure, or driving pressure, may be associated with worse outcomes in children has not been clearly defined (7, 20). In this review, we systematically sought to evaluate and summarize the main studies in children that describe the most commonly used mechanical ventilation parameters during ECMO therapy and their association with in-hospital mortality, organ failure, and complications.
Methods
Search strategy and selection criteria
A systematic search was conducted in the main databases without language or time restrictions from January 2013 until May 1, 2023. PubMed, Medline, EMBASE, the Cochrane Library, and Google Scholar were electronically searched. A prospective registration was made in PROSPERO (ID 450744 available at http://www.crd.york.ac.uk/PROSPERO). The list of publications, including reviews, was searched manually. The reference list of relevant studies was manually checked for additional publications that were useful for analysis. Only human research was included, and a report was made according to the PRISMA and MOOSE guidelines.
Inclusion and exclusion criteria
We included studies in our analysis that met the following criteria:
(1) studies involving children aged one month to 18 years old with refractory respiratory failure requiring ECMO support,
(2) studies describing mechanical ventilation support parameters during ECMO therapy in the first three days of admission to PICU,
(3) studies with any methodological design as well as gray literature (published in abstracts of major critical care conferences, OpenMD and OpenGrey) or peer-reviewed articles that reported complete information on sample size, type of support in VMI and associated outcomes,
(4) In the case of multiple studies with similar databases or overlapping populations, we selected the most recent for analysis.
Studies that met the following criteria were excluded: animal models, preclinical studies, neonatal stage research (including preterm and diaphragmatic hernia patients), letters to the editor, narrative reviews, and studies without clear descriptions of outcomes.
Study selection and data processing
Five independent reviewers (MCP, JDB, JG, MSM, JFS) searched the articles, including titles and abstracts, and determined the eligibility of the investigations. The inclusion criteria were applied, and a full-text review with data extraction was performed. If there were doubts about eligibility, a second group of researchers (LA, HM, YV, CS, DP, and DC) made the decision on whether to include the study. All relevant data were extracted independently from the included studies (JFS, MCP, and JDB). Data on the parameters of mechanical ventilation, ventilation mode, inspired fraction of oxygen (Fi02), end-expiratory pressure (PEEP), plateau pressure (Pplat), and driving pressure (DP) were extracted.
Methodological quality assessment
The quality of the studies was determined in several respects. External validity considered the target population, random error, and generalizability of results. Internal validity included evaluation of the design, description of the methodology, and form of evaluation and control of possible biases. We had planned to apply the Cochrane Risk of Bias Scale, version 2.0, but found no clinical trials to include in our analysis. Risk of bias in observational studies was assessed using the Newcastle-Ottawa scale (21). The criteria for evaluating the studies were sample selection, comparability, and outcomes.
Outcomes
The principal aim of our study was to evaluate the impact of the parameters used in mechanical ventilation support (tidal volume, PEEP, Fi02, plateau pressure, DP) in children with ECMO on in-hospital mortality. Secondary outcomes were the total duration of ventilation and the presence of associated multiple organ failure (MODS).
Data synthesis and statistical analysis
A descriptive analysis of the variables included in the study was initially performed. To compare quantitative variables, if the studies reported them as median or interquartile range, these were converted to means and standard deviations (SD) using the method proposed by Wan et al. (22). The risk of mortality was assessed with odds ratio (OR) with its respective 95% confidence interval (95% CI). The meta-analysis was conducted with a randomized effects model. Heterogeneity was assessed with visual inspection of forest plots, Q-test, and I2 statistics. A value less than 25% was considered without heterogeneity, mild from 25% to 50%, moderate from 50% to 75%, and high greater than 75%. Inverse-variance meta-analyses were performed by applying a logit transformation to the outcome, and the DerSimonian–Laird method (23) was used to estimate the heterogeneity of variance. The factors of sample size and methodological quality (high or poor) that could explain the heterogeneity were subjected to a subgroup and sensitivity analysis. Publication bias was assessed with the funnel plot, the Egger test for continuous variables, and the Peter's test for dichotomous variables. A p < 0.05 was considered statistically significant (except for the Egger test, for which p < 0.1 was considered significant).
The statistical analyses were done using Review Manager Version 5.4 (ReVman 5.4—Cochrane IMS, Cochrane Library, Oxford, United Kingdom) software.
Results
A total of 460 studies were found (Figure 1). After removing duplicate or non-relevant studies, 15 studies were assessed for eligibility. A total of six studies with 1,512 patients met the inclusion criteria (7, 17, 19, 24–26). All the studies were observational. No clinical trials comparing the safety and efficacy of different mechanical ventilation support parameters with the outcomes of interest were found. Of the included studies, five were cohort, two were prospective (7, 17), and three were retrospective (20, 22, 24). One study was a case series of patients with leukemia (25). We obtained information on pressures and other ventilator settings in all studies. They are summarized in Table 1.
The median age in the included studies was 3.4 years (IQR: 3.2–4.2). In all studies, patients received veno-venous ECMO support, except in one study in which 57% of the patients had veno-arterial cannulation (25). The studies were at low to moderate risk of bias (Figures 2A,B). All prospective studies were classified as at moderate risk of bias. Some studies were single-arm and did not describe all ventilation parameters associated with mortality. The predominant source of information was medical record review and was not shown to be valid or reliable.
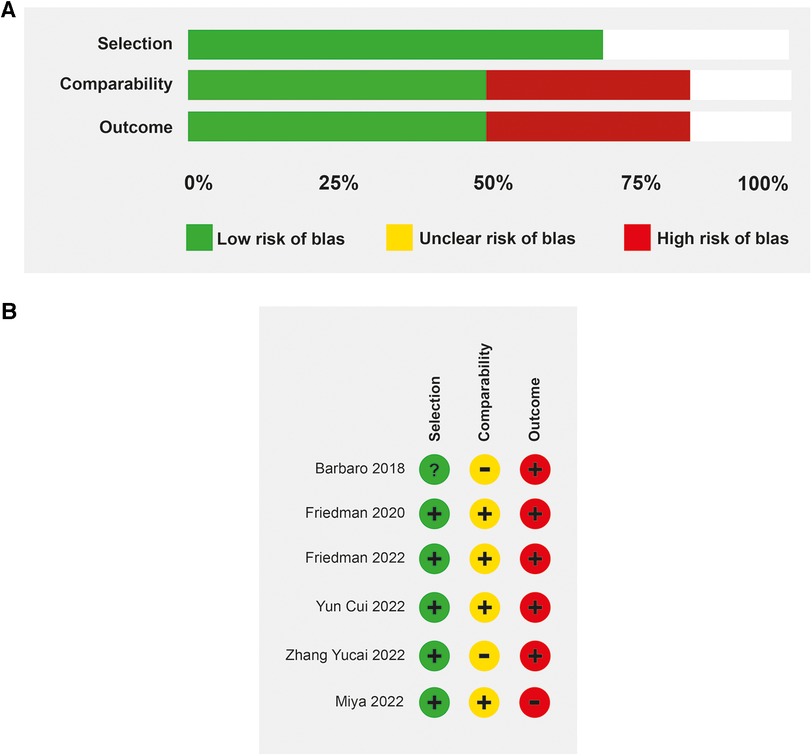
Figure 2. Risk of bias. (A) Evaluation of the risk of bias in the included studies on patients with mechanical ventilation and ECMO (B) Risk of bias determined by the Newcastle-Ottawa scale.
Outcomes
Mortality associated with mechanical ventilation parameters
The association between tidal volume (VT) and mortality was described in 291 patients. Overall survival in the studies was 69%. No differences in in-hospital mortality were observed in patients receiving low tidal volume ventilation (VT > 4 ml/kg) (p = 0.52). An increased risk of dying was found (OR: 4.70; 95% CI: 2.91–7.59; p < 0.01) among children receiving very low tidal volume ventilation (VT < 4 ml/kg), with low heterogeneity in the studies (I2 to 38%) (Figures 3A,B). In contrast, patients who survived had lower plateau pressures (mean Dif: −0.70; 95% CI: −0.18, −0.22; p < 0.01) compared to those who died. Patients with lower driving pressures (mean Dif: −0.96; 95% CI: −1.83; −0.09; p = 0.03) also had lower mortality. No differences were observed in the inspired fraction of oxygen between survivors and those who died (p = 0.09). No differences were observed between groups in the PEEP value (p = 0.69). The mechanical ventilation parameters included in the analysis are described in Figure 4.
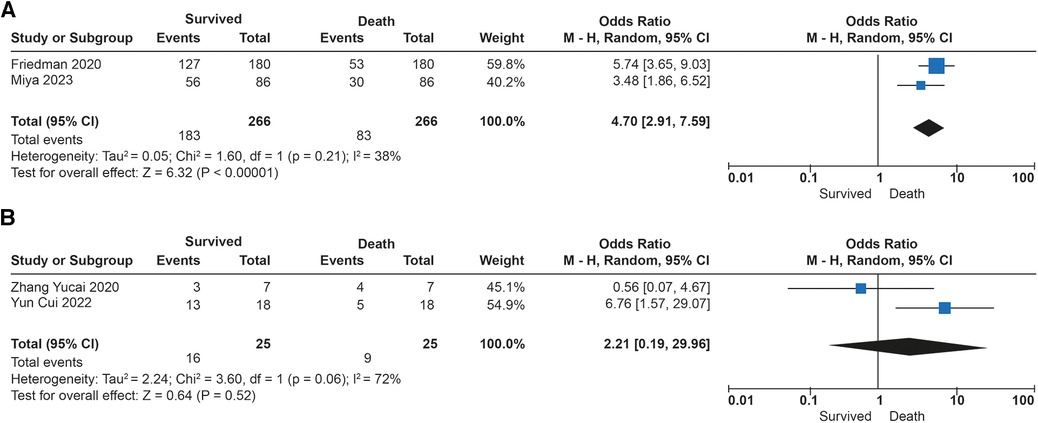
Figure 3. Forest plot de tidal volumen and mortality in patients with ECMO. (A) Subgroup analysis with Ultraprotective ventilation (<4 ml/kg). (B) Subgroup analysis in patients with protective ventilation (>4 ml/kg).
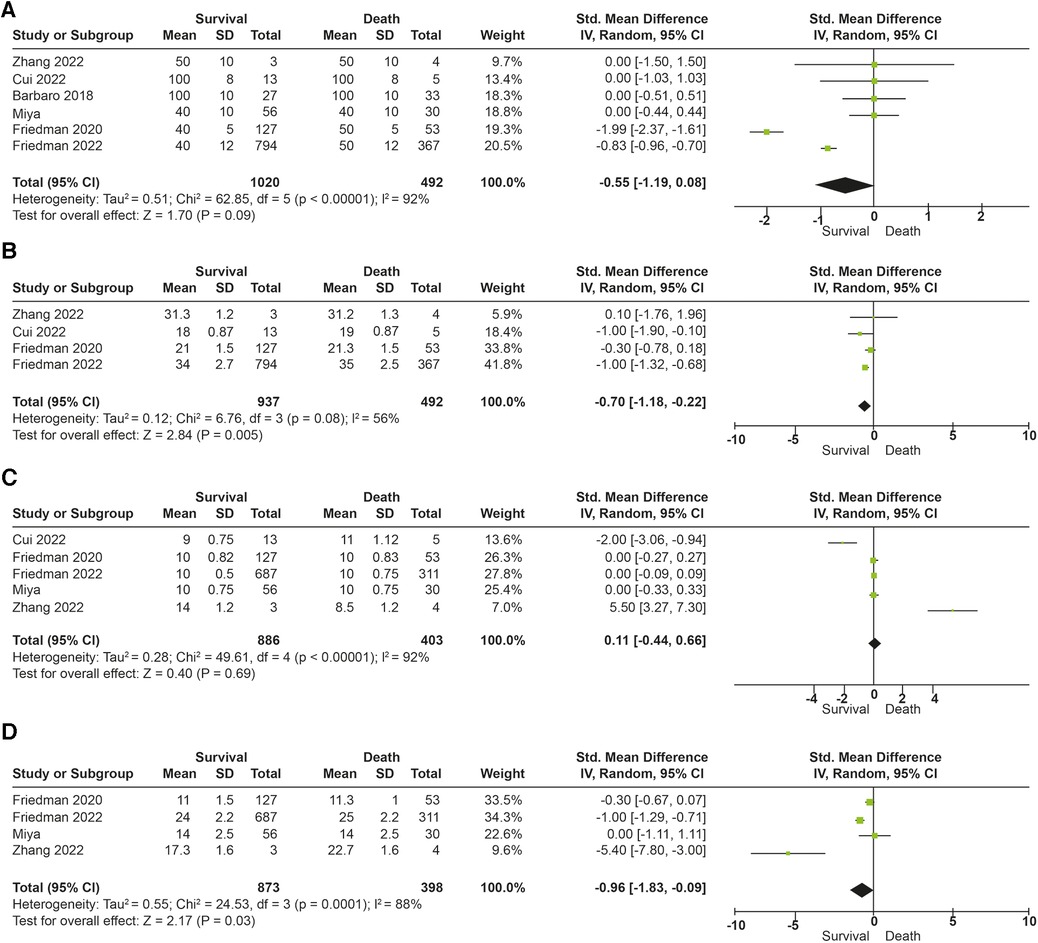
Figure 4. Forest plot parameters of mechanical ventilation and mortality (A) fraction of inspired oxygen (%). (B) Plateau pressure (cm/H20). (C) End expiratory pressure (PEEP). (D) Driving pressure (cm/H20).
Hospital stay and MODS
The survivors had a median hospital stay of 20 days (IQR: 13.2–38.4), and the non survivors had 13.8 days (IQR: 13.2–14.4) (p = 0.88). The presence of MODS was described in 152 patients. The group with higher survival had fewer dysfunctional organs compared to patients who died (mean Dif: −1.34, 95% CI: −2.08, −0.60; p = 0.01).
Sensitivity analysis was run for the primary outcome. Among the six included studies, Zhang's study (25) differed significantly in terms of the population included because they were exclusively leukemia patients in contrast to the other five studies reporting mechanical ventilation parameters in children with ECMO with sepsis, trauma, and ARDS of other etiologies. However, after excluding Zhang's study, the combined data from the other five studies aligned with each of the outcomes studied. Additionally, high- and low-risk studies were analyzed without finding differences in the overall results. This indicates that our findings were consistent and robust after sensitivity analysis because the results were not affected by the removal of individual studies.
Discussion
In this systematic review and meta-analysis in children with mechanical ventilation and ECMO, we found that the ventilator parameters associated with higher mortality were very low tidal volume ventilation (<4 ml/kg), high plateau pressure, and high driving pressure. We found no association between mortality and the inspired fraction of oxygen and PEEP provided in MV. Patients who died had more organs involved, with no difference in length of hospital stay.
The main objectives of mechanically ventilated support in patients with ECMO are to limit pulmonary strain, avoid atelectrauma, alveolar overdistension injuries, and resorptive atelectasis (associated with a high inspired oxygen fraction) (26–28). Pulmonary injury associated with mechanical ventilation occurs because of several mechanisms: barotrauma, volutrauma, atelectrauma, ergotrauma, myotrauma, and biotrauma. In large part, this injury is explained by excess strain, or alveolar stress, and shear forces applied during MV (28, 29). Limiting pressures (e.g., plateau airway pressures <30 cm/H20) and VT (4–8 ml/kg predicted body weight PBW) are often the recommended strategies that have been called pulmonary protective ventilation (14).
Very low tidal volume ventilation was initially suggested by animal researches as a better strategy of protective ventilation. Using a mouse model of acid-induced pulmonary injury, Frank et al. found that reducing the tidal volume from 12 ml/kg to 3–6 ml/kg with the same level of PEEP (10 cm/H20) could reduce mortality (30). It was suggested that decreased pulmonary edema and pulmonary injury might help protect the alveolar epithelium. In adults, research has recently been carried out comparing different tidal volumes in ARDS. Bein et al. compared the strategies of 3 ml/kg PBW vs. 6 ml/kg PBW in 79 adults with ARDS without finding differences in days off mechanical ventilation or mortality between groups (31). Recently, another randomized clinical trial of adults with ARDS and ECMO support evaluated the inflammatory response of very low tidal volume ventilation (2 ml/kg PBW) vs. conventional MV (4–8 ml/kg using the volume control mode). No differences were observed in the concentrations of interleukin-1 beta, interleukin-6, interleukin-8, or surfactant protein D. In fact, the study had to be suspended due to the futility of the results after the inclusion of 39 patients, and higher mortality was observed in the group with very low tidal volume ventilation (45% vs. 17%) (27).
Our findings are consistent with these descriptions. We found that children with VT less than 4 ml/kg had higher mortality than patients with ECMO, who received low tidal volume ventilation (VT 4–6 ml/kg). The two main studies that contributed to this finding (19, 26) used pressure-targeted ventilation modes in 90.3% and 77% of the patients, respectively. These patients may have been more seriously ill, with lower respiratory system distensibility. However, these findings could be explained by shear injuries in a severely diseased lung. Additionally, very low tidal volumes can contribute to injury due to cyclic opening and closing of healthy and sick alveolar units, and favor atelectrauma and more VILI (10). This damage occurs especially when the end-expiratory pressure drops below local airway closing pressures (8, 10). In the absence of large, randomized studies in children comparing very low tidal volume ventilation (VT < 4 ml/kg) with low tidal volume ventilation (VT 4–6 ml/kg) during ECMO support, a definitive recommendation cannot be made. PALICC-2 mentions that offering lung protective ventilation during ECMO is beneficial, but exact modes and settings remain elusive (15). They recommend maintaining a plateau pressure of less than 25 cm H20 from the start of ECMO support. Our findings suggest that low tidal volume ventilation should be used and other ventilation support parameters considered to decrease driving pressure and mechanical power. In the most severe cases, when DP cannot be decreased, very low tidal volume ventilation could be considered. However, clinical trials are needed to evaluate the safety and efficacy of this strategy.
In this sense, the MV guidelines in pediatric (5, 6, 9, 15) and adult (32) ECMO recommend maintaining adequate levels of PEEP and limiting Pplat but do not give a specific recommendation regarding tidal volume. In our study, high plateau and driving pressure were associated with higher mortality. During MV with ECMO, it is desirable to sustain a Pplat less than 30 cm H20 and preferably look for levels less than 25 cm H20 (12, 15, 32). Low levels of Pplateau have been associated with lower mortality in adult patients with ECMO (28% vs. 46%) (33). In fact, it has also been noted that, in adults, each increase of 1 cm/H20 in the Pplat decreases the in-hospital survival odds by 21% in patients with ARDS and ECMO support (33). Accordingly, with an improved Pplat and optimized PEEP, DP could be reduced, the elevation of which is directly associated with greater mortality. When patients have low DPs at the beginning of ECMO support, it is reasonable to think that they have greater respiratory system distensibility and, therefore, less severe disease, with a greater proportion of functionally uncompromised lung. Chiu Li-Chung et al. recently found that maintaining a DP lower than 21 cm/H20 on the third day of ECMO was associated with lower mortality (56% vs. 33%; p < 0.01) compared to patients with higher levels (34). Rambaud et al. found in children that a DP greater than 15 cm/h20 on the first day of ECMO was associated with a higher mortality odds ratio (OR: 2.23, 95% CI: (1.09–4.71), p = 0.03) (35). However, they found a higher risk of death with a high PEEP, which we did not find in our study.
Another important finding in our study is the lack of association between Fi02 and PEEP levels and mortality in patients with ECMO. With sufficient oxygenation delivered by the ECMO, it is possible to lower the Fi02 below 40% to avoid further biotrauma. This is not possible for all patients. It is most frequently recommended to maintain Fi02 at 100% in the ECMO at PEEP at the time of its initiation and gradually decrease the Fi02 of the mechanical ventilator below 60%, seeking saturation goals greater than 85% (32, 34). A native lung FiO2 above 60% on D14 has been associated with higher odds of mortality in children on ECMO (OR: 10.36, 95% CI: (1.51–116.15), p = 0.03) (35). End-expiratory pressure (PEEP) is a parameter that helps improve functional alveolar units and gas exchange. A tidal volume of 4–6 ml/kg with moderate positive end expiratory pressure (6–10 cm/H20) is the basis of mechanical protective ventilation. Fifty-eight percent of centers with adult ECMO report PEEP use of 6–10 cm/H20, and 22% more than 10 cm/H20 (7, 20). The use of a PEEP adjusted to the condition of each patient, guided by the ARDS net protocol, graphically or with compliance analysis, reduces atelectrauma and prevents alveolar collapse and reopening in each respiratory cycle. The ELSO and PALICC guidelines recommend that patients with ECMO should start with a PEEP of 10 cm/H20, although it is emphasized that it should be adjusted to the condition of each patient (5, 15). The beneficial effects of PEEP may be more related to DP reduction. In a recent meta-analysis of adults, the PEEP level was not related to outcomes or mortality, in line with our findings (36).
We consider that our study has several limitations. First, we found no controlled clinical trials evaluating the safety and efficacy of different ventilatory modes or specific parameters of MV in children with ECMO. We only found observational studies. These studies describe associations that are not necessarily causal. Furthermore, the possibility of residual confounders not being considered in each study having biased the results cannot be excluded. Additionally, we did not find in the studies that the programmed respiratory rate was reported in each patient. In pediatrics, this is an important point because we know that it can vary with age and can be one of the components of ergotrauma, or P-SILI, that affect outcomes. In this regard, patients with VT < 4 ml/kg had a higher mortality rate. They may have been sicker, with less respiratory distensibility. Thus, DP is directly proportional to VT. Patients with more severe disease may be the only ones to benefit from very low tidal volume to keep DP as low as possible. In the included studies the majority of patients received VLTV and only twenty-five patients were in the low tidal volume group. This may have limited the findings for this group. No study included considering DP as a variable to adjust tidal volume. Prospective studies are needed to clarify this finding. Some children in the studies were supported with high-frequency mechanical ventilation. We did not find a homogeneous or constant description of parameters in high-frequency oscillatory ventilation (HFOV) to include in the meta-analysis. However, this type of ventilation could help improve alveolar recruitment and some of the outcomes that bias results. In this same sense, we only found studies with veno-venous ECMO. We found no studies with veno-arterial ECMO that specifically described the support parameters in mechanical ventilation, which may limit the extrapolation of our findings. However, in adults with ARDS, the type of cannulation on ECMO is not considered to affect the outcomes of mechanically ventilated patients (31, 32). Finally, we did not find constant descriptions of the duration of mechanical ventilation before the start of ECMO. In experimental studies of adults, this has been shown to be one of the most important factors and determinants of prognosis. Previous mechanical ventilation lasting more than seven days could affect mortality outcomes. We need clinical trials in children that evaluate the impact of these variables on outcomes in children with ECMO.
Conclusion
This systematic review and meta-analysis found that the variables in mechanical ventilation in children with refractory respiratory failure who receive ECMO support associated with higher mortality are very low tidal volume (<4 ml/kg), high plateau pressures, and high driving pressures. We found no overlap between mortality and other parameters of the mechanical ventilator, such as the inspired fraction of oxygen or the pressure at the end of expiration. Clinical trials in children evaluating the safety and efficacy of the most used MV parameters in patients with ECMO are needed.
Data availability statement
The raw data supporting the conclusions of this article will be made available by the authors, without undue reservation.
Author contributions
JF: Writing – original draft, Writing – review & editing. MP: Investigation, Methodology, Project administration, Writing – review & editing. JB: Conceptualization, Investigation, Software, Writing – original draft. LA: Formal Analysis, Project administration, Validation, Writing – original draft. MS: Conceptualization, Funding acquisition, Project administration, Visualization, Writing – original draft. JG: Formal Analysis, Project administration, Resources, Software, Writing – original draft. CS: Conceptualization, Formal Analysis, Writing – original draft. DP: Investigation, Resources, Visualization, Writing – original draft. DC: Resources, Software, Validation, Writing – original draft. YR: Project administration, Resources, Supervision, Writing – original draft. HM: Writing – review & editing.
Funding
The author(s) declare financial support was received for the research, authorship, and/or publication of this article.
Our review was funded by the researchers' resources, Universidad de La Sabana (MED-256-2019) project and Fundacion Cardioinfantil-IC.
Acknowledgments
To all the medical and nursing staff and team of residents who have contributed to research studies in our PICU, Universidad de La Sabana for supporting the MED-256-2019 project from which this research was derived and Fundación Cardioinfantil-IC.
Conflict of interest
The authors declare that the research was conducted in the absence of any commercial or financial relationships that could be construed as a potential conflict of interest.
Publisher's note
All claims expressed in this article are solely those of the authors and do not necessarily represent those of their affiliated organizations, or those of the publisher, the editors and the reviewers. Any product that may be evaluated in this article, or claim that may be made by its manufacturer, is not guaranteed or endorsed by the publisher.
References
1. Cruces P. Pediatric acute respiratory distress syndrome: approaches in mechanical ventilation. Pediatr Crit Care Med. (2023) 24(2):e104–14. doi: 10.1097/PCC.0000000000003138
2. McMichael ABV, Ryerson LM, Ratano D, Fan E, Faraoni D, Annich GM. 2021 ELSO adult and pediatric anticoagulation guidelines. ASAIO J. (2022) 68:303–10. doi: 10.1097/MAT.0000000000001652
3. Gajkowski EF, Herrera G, Hatton L, Velia Antonini M, Vercaemst L, Cooley E. ELSO guidelines for adult and pediatric extracorporeal membrane oxygenation circuits. ASAIO J. (2022) 68(2):133–52. doi: 10.1097/MAT.0000000000001630
4. Ramanathan K, Shekar K, Ling RR, Barbaro RP, Wong SN, Tan CS, et al. Extracorporeal membrane oxygenation for COVID-19: a systematic review and meta-analysis. Crit Care. (2021) 25(1):211. doi: 10.1186/s13054-021-03634-1
5. Maratta C, Potera RM, van Leeuwen G, Castillo Moya A, Raman L, Annich GM. Extracorporeal life support organization (ELSO): 2020 pediatric respiratory ELSO guideline. ASAIO J. (2020) 66(9):975–9. doi: 10.1097/MAT.0000000000001223
6. Wild KT, Rintoul N, Kattan J, Gray B. Extracorporeal life support organization (ELSO): guidelines for neonatal respiratory failure. ASAIO J. (2020) 66(5):463–70. doi: 10.1097/MAT.0000000000001153
7. Friedman ML, Abu-Sultaneh S, Slaven JE, Mastropietro CW. Rest ventilator management in children on veno-venous extracorporeal membrane oxygenation. Int J Artif Organs. (2022) 45(2):174–80. doi: 10.1177/0391398821999386
8. Marhong JD, Telesnicki T, Munshi L, Del Sorbo L, Detsky M, Fan E. Mechanical ventilation during extracorporeal membrane oxygenation. An international survey. Ann Am Thorac Soc. (2014) 11(6):956–61. doi: 10.1513/AnnalsATS.201403-100BC
9. Dalton HJ, Macrae DJ, Pediatric acute lung injury consensus conference group. Extracorporeal support in children with pediatric acute respiratory distress syndrome: proceedings from the pediatric acute lung injury consensus conference. Pediatr Crit Care Med. (2015) 16:S111–7. doi: 10.1097/PCC.0000000000000439
10. Slutsky AS, Ranieri VM. Ventilator-induced lung injury. N Engl J Med. (2013) 369(22):2126–36. doi: 10.1056/NEJMra1208707
11. Cruces P, Erranz B, González C, Diaz F. Morphological differences between patient self-inflicted and ventilator-induced lung injury: an experimental study. Am J Respir Crit Care Med. (2023) 207(6):780–3. doi: 10.1164/rccm.202207-1360LE
12. Bhalla AK, Klein MJ, Modesto I, Alapont V, Emeriaud G, Kneyber MCJ, Medina A, et al. Pediatric acute lung injury and sepsis investigators (PALISI) network. Mechanical power in pediatric acute respiratory distress syndrome: a PARDIE study. Crit Care. (2022) 26(1):2. doi: 10.1186/s13054-021-03853-6
13. Grunwell JR, Dahmer MK, Sapru A, Quasney MW, Flori H. Pathobiology, severity, and risk stratification of pediatric acute respiratory distress syndrome: from the second pediatric acute lung injury consensus conference. Pediatr Crit Care Med. (2023) 24:S12–27. doi: 10.1097/PCC.0000000000003156
14. Emeriaud G, López-Fernández YM, Iyer NP, Bembea MM, Agulnik A, Barbaro R, et al. Executive summary of the second international guidelines for the diagnosis and management of pediatric acute respiratory distress syndrome (PALICC-2). Pediatr Crit Care Med. (2023) 24(2):143–68. doi: 10.1097/PCC.0000000000003147
15. Rambaud J, Barbaro RP, Macrae DJ, Dalton HJ. Extracorporeal membrane oxygenation in pediatric acute respiratory distress syndrome: from the second pediatric acute lung injury consensus conference. Pediatr Crit Care Med. (2023) 24:S124–34. doi: 10.1097/PCC.0000000000003164
16. Combes A, Peek GJ, Hajage D, Hardy P, Abrams D, Schmidt M, et al. ECMO for severe ARDS: systematic review and individual patient data meta-analysis. Intensive Care Med. (2020) 46:2048–57. doi: 10.1007/s00134-020-06248-3
17. Barbaro RP, Xu Y, Borasino S, Truemper EJ, Watson RS, Thiagarajan RR, et al. Does extracorporeal membrane oxygenation improve survival in pediatric acute respiratory failure? Am J Respir Crit Care Med. (2018) 197:1177–86. doi: 10.1164/rccm.201709-1893OC
18. Barbaro RP, Thiagarajan RR, Wypij D, Curley MAQ. Do we really doubt extracorporeal membrane oxygenation efficacy in pediatric acute respiratory distress syndrome? Am J Respir Crit Care Med. (2018) 198:409–10. doi: 10.1164/rccm.201803-0459LE
19. Friedman ML, Barbaro RP, Bembea MM, Bridges BC, Chima RS, Kilbaugh TJ, et al. Mechanical ventilation in children on venovenous ECMO. Respir Care. (2020) 65:271–80. doi: 10.4187/respcare.07214
20. Blauvelt DG, Inany HS, Furlong-Dillard JM, Bailly DK, Oishi P, Steurer MA, et al. Association of ventilator settings with mortality in pediatric patients treated with extracorporeal life support for respiratory failure. ASAIO J. (2022) 68:1536–43. doi: 10.1097/MAT.0000000000001697
21. Hartling L, Milne A, Hamm MP, Vandermeer B, Ansari M, Tsertsvadze A, et al. Testing the Newcastle Ottawa scale showed low reliability between individual reviewers. J Clin Epidemiol. (2013) 66(9):982–93. doi: 10.1016/j.jclinepi.2013.03.003
22. Wan X, Wang W, Liu J, Tong T. Estimating the sample mean and standard deviation from the sample size, median, range and/or interquartile range. BMC Med Res Methodol. (2014) 14:135. doi: 10.1186/1471-2288-14-135
23. DerSimonian R, Laird N. Meta-analysis in clinical trials. Control Clin Trials. (1986) 7:177–88. doi: 10.1016/0197-2456(86)90046-2
24. Cui Y, Shi J, Zhou Y, Dou J, Xiong X, Sun T, et al. Extracorporeal membrane oxygenation for paediatric refractory hypoxic respiratory failure caused by adenovirus in Shanghai: a case series. BMC Pediatr. (2022) 22(1):138. doi: 10.1186/s12887-022-03197-2
25. Zhang Y, Zhou Y, Shi J, Shan Y, Sun T, Wang C, et al. Extracorporeal membrane oxygenation for severe acute respiratory distress syndrome in children with leukemia/lymphoma: a retrospective case series. Front Pediatr. (2022) 10:955317. doi: 10.3389/fped.2022.955317
26. Miya TR, Furlong-Dillard JM, Sizemore JM, Meert KL, Dalton HJ, Reeder RW, et al. Association between mortality and ventilator parameters in children with respiratory failure on ECMO. Respir Care. (2023) 68(5):592–601. doi: 10.4187/respcare.10107
27. Guervilly C, Fournier T, Chommeloux J, Arnaud L, Pinglis C, Baumstarck K, et al. Ultra-lung-protective ventilation and biotrauma in severe ARDS patients on veno-venous extracorporeal membrane oxygenation: a randomized controlled study. Crit Care. (2022) 26(1):383. doi: 10.1186/s13054-022-04272-x
28. Abrams D, Agerstrand C, Beitler JR, Karagiannidis C, Madahar P, Yip NH, et al. Risks and benefits of ultra-lung-protective invasive mechanical ventilation strategies with a focus on extracorporeal support. Am J Respir Crit Care Med. (2022) 205(8):873–82. doi: 10.1164/rccm.202110-2252CP
29. Terragni PP, Del Sorbo L, Mascia L, Urbino R, Martin EL, Birocco A, et al. Tidal volume lower than 6 ml/kg enhances lung protection: role of extracorporeal carbon dioxide removal. Anesthesiology. (2009) 111:826–35. doi: 10.1097/ALN.0b013e3181b764d2
30. Frank JA, Gutierrez JA, Jones KD, Allen L, Dobbs L, Matthay MA. Low tidal volume reduces epithelial and endothelial injury in acid-injured rat lungs. Am J Respir Crit Care Med. (2002) 165:242–9. doi: 10.1164/ajrccm.165.2.2108087
31. Bein T, Weber-Carstens S, Goldmann A, Müller T, Staudinger T, Brederlau J, et al. Lower tidal volume strategy (≈3 ml/kg) combined with extracorporeal CO2 removal versus “conventional” protective ventilation (6 ml/kg) in severe ARDS: the prospective randomized Xtravent-study. Intensive Care Med. (2013) 39:847–56. doi: 10.1007/s00134-012-2787-6
32. Grasselli G, Calfee CS, Camporota L, Poole D, Amato MBP, Antonelli M, et al. ESICM guidelines on acute respiratory distress syndrome: definition, phenotyping and respiratory support strategies. Intensive Care Med. (2023) 49(7):727–59. doi: 10.1007/s00134-023-07050-7
33. Modrykamien AM, Hernandez OO, Im Y, Walters RW, Schrader CL, Smith LE, et al. Mechanical ventilation in patients with the acute respiratory distress syndrome and treated with extracorporeal membrane oxygenation: impact o hospital and 30 day post discharge survival. ASIO J. (2016) 62:607–12. doi: 10.1097/MAT.0000000000000406
34. Chiu LC, Hu HC, Hung CY, Chang CH, Tsai FC, Yang CT, et al. Dynamic driving pressure associated mortality in acute respiratory distress syndrome with extracorporeal membrane oxygenation. Ann Intensive Care. (2017) 1:12. doi: 10.1186/s13613-017-0236-y
35. Rambaud J, Broman LM, Chevret S, Visconti F, Leger PL, Guner Y, et al. Association between pediatric intensive care mortality and mechanical ventilation settings during extracorporeal membrane oxygenation for pediatric acute respiratory distress syndrome. Eur J Pediatr. (2023) 182(10):4487–97. doi: 10.1007/s00431-023-05119-5
36. Serpa Neto A, Schmidt M, Azevedo LC, Bein T, Brochard L, Beutel G, et al. Associations between ventilator settings during extracorporeal membrane oxygenation for refractory hypoxemia and outcome in patients with acute respiratory distress syndrome: a pooled individual patient data analysis: mechanical ventilation during ECMO. Intensive Care Med. (2016) 42:1672–84. doi: 10.1007/s00134-016-4507-0
Keywords: mortality, ECMO, children, respiratory failure, ARDS, mechanical ventilation, pneumonia
Citation: Fernandez-Sarmiento J, Perez MC, Bustos JD, Acevedo L, Sarta-Mantilla M, Guijarro J, Santacruz C, Pardo DF, Castro D, Rosero YV and Mulett H (2024) Association between mechanical ventilation parameters and mortality in children with respiratory failure on ECMO: a systematic review and meta-analysis. Front. Pediatr. 12:1302049. doi: 10.3389/fped.2024.1302049
Received: 26 September 2023; Accepted: 4 January 2024;
Published: 16 January 2024.
Edited by:
Thomas Schaible, University of Heidelberg, GermanyReviewed by:
Alba Perez Ortiz, University of Heidelberg, GermanyThomas Vincent Brogan, Seattle Children's Hospital, United States
© 2024 Fernandez-Sarmiento, Perez, Bustos, Acevedo, Sarta-Mantilla, Guijarro, Santacruz, Pardo, Castro, Rosero and Mulett. This is an open-access article distributed under the terms of the Creative Commons Attribution License (CC BY). The use, distribution or reproduction in other forums is permitted, provided the original author(s) and the copyright owner(s) are credited and that the original publication in this journal is cited, in accordance with accepted academic practice. No use, distribution or reproduction is permitted which does not comply with these terms.
*Correspondence: Jaime Fernandez-Sarmiento amFpbWVmZUB1bmlzYWJhbmEuZWR1LmNv