- 1NESMOS Department, Pediatric Unit Sant’Andrea Hospital, Faculty of Medicine and Psychology, “Sapienza” University, Rome, Italy
- 2Department of Electronics, Information and Bioengineering, Politecnico di Milano University, Milano, Italy
- 3Restech Srl, Milan, Italy
Background: Evaluating oscillometry parameters separately for the inspiratory and expiratory breath phases and their within-breath differences can help to identify exercise-induced bronchoconstriction (EIB) in pediatric outpatients disclosing exercise-induced symptoms (EIS).
Aims: To assess the response in impedance parameters following an exercise challenge in patients reporting EIS.
Methods: Sixty-eight patients reporting EIS (34 asthmatics and 34 suspected of asthma, age mean = 10.8 years, range = 6.0–16.0) underwent an incremental treadmill exercise test. Spirometry was performed at baseline and 1, 5-, 10-, 15-, and 20-min post exercise. Oscillometry was performed at baseline and at 3- and 18-min post exercise. Bronchodilator response to 200 µg albuterol was then assessed. EIB was defined as a forced expiratory volume in 1 s (FEV1) fall ≥10% from baseline. Expiratory and inspiratory resistance (Rrs) and reactance (Xrs), their z-score (Ducharme et al. 2022), and their mean within-breath differences (ΔRrs = Rrsexp-Rrsinsp, ΔXrs = Xrsexp-Xrsinsp) were calculated. Receiver operating characteristic (ROC) curves and their areas (AUCs) were used to evaluate impedance parameters’ performances in classifying EIB.
Results: Asthmatic patients developed EIB more frequently than those suspected of asthma [18/34 (52.9%) vs. 2/34 (5.9%), p < 0.001]. In the 20 subjects with EIB, Rrsinsp, Rrsexp, Xrsinsp, and Xrsexp peaked early (3’), and remained steady except for Xrsinsp, which recovered faster afterward. ΔXrs widened 18 min following the exercise and reversed sharply after bronchodilation (BD) (−1.81 ± 1.60 vs. −0.52 ± 0.80 cmH2O × s/L, p < 0.001). Cutoffs for EIB leading to the highest AUCs were a rise of 0.41 in z-score Rrsinsp (Se: 90.0%, Sp: 66.7%), and a fall of −0.64 in z-score Xrsinsp (Se: 90.0%, Sp: 75.0%). Accepting as having “positive” postexercise oscillometry changes those subjects who had both z-scores beyond respective cutoffs, sensitivity for EIB was 90.0% (18/20) and specificity, 83.3% (40/48).
Conclusion: Oscillometry parameters and their within-breath differences changed markedly in pediatric patients presenting EIB and were restored after the bronchodilator. Strong agreement between z-scores of inspiratory oscillometry parameters and spirometry supports their clinical utility, though larger studies are required to validate these findings in a broader population.
Introduction
A common complaint in pediatric outpatients visiting the pulmonologist is the limitation of physical activity, which they often refer to as dyspnea, cough, chest pain, or other less-well-defined symptoms elicited by exercise. These patients embrace a heterogeneous group with some having a history of recurrent respiratory symptoms, while others refer to exercise limitation as their only complaint (1, 2). Consequently, children are less prone to get involved in sports with further physical and psychosocial drawbacks (1). Testing for exercise-induced bronchoconstriction (EIB) is suitable, even when an asthma diagnosis seems inconsistent with the clinical history (3–5). Conversely, the presence of EIB in yet-diagnosed asthmatic children suggests poor disease control (2, 3).
Classical exercise airway challenges entail repeated spirometry at baseline and in a timely manner after exercise, with skilled patients being able to perform these maneuvers. In children, oscillometry, which is also called the forced oscillation technique (FOT) needing quiet breaths, is feasible and reproducible (6, 7). Throughout input wave or pressure pulses at the mouth opening, oscillometry measures of respiratory resistance (Rrs) and reactance (Xrs) disclose contributions to the total respiratory impedance (Zrs) from proximal and peripheral airways and tissues, and their response to several bronchial stimuli (6, 8). Children tolerate well the midwave 8 Hz input frequency and several reference values for Rrs and Xrs at this frequency are now available (9–11).
A practical use of the oscillometry parameters is to compute them separately for the inspiratory and expiratory phases and assess their changes during the breath cycle. This approach is spreading as it may increase sensitivity and specificity to specific pathologies and conditions (6, 12). In particular, the within-breath difference between the mean inspiratory Xrs (Xrsinsp) and the mean expiratory Xrs (Xrsexp) increases with the occurrence of tidal expiratory airflow limitation (13). This approach has been useful to assess adult patients with chronic obstructed pulmonary disease (COPD) and children with cystic fibrosis (13–15). In this regard, no studies in pediatric patients have sought to evaluate the within-breath changes in reactance during EIB. Also, previous studies did not consider changes in z-scores of impedance parameters, but absolute or relative changes were reported. The use of z-scores may better evaluate the effect of exercise.
We hypothesized that EIB [as assessed by the maximal forced expiratory volume in 1 s (FEV1) fall], other than inducing changes in oscillometry parameters, also induces wide within-breath differences in Xrs that lessen as the bronchial patency restores. In keeping, we aimed to assess the response in impedance parameters following an exercise challenge in patients reporting exercise-induced symptoms (EIS), either without or with a previous diagnosis of asthma. For this purpose, we assessed Rrs, Xrs, at 8 Hz, and their within-breath differences at baseline and after the exercise challenge.
Materials and methods
Subjects
We enrolled 73 consecutive outpatients attending the pulmonary section of our Pediatric Unit (Sant’Andrea Hospital, Rome, Italy) for EIS, to perform a bronchial challenge with exercise. After excluding five subjects not fulfilling the inclusion criteria (below), the remaining 68 patients yielded two groups of 34 subjects each as follows: (1) EIS without a previous diagnosis of asthma (“suspected asthma”), and (2) EIS and asthma (“asthma”). The suspected asthma group comprised new-attendant children reporting exercise limitation as their main complaint, often associated with rhinitis, without a clinical history of asthma. None of these patients had hoarseness, stridor, chocking, or abnormal spirometry (flattening of the inspiratory portion of the flow volume) suspected of exercise-induced laryngeal obstruction (EILO). The asthma group included patients whose diagnosis was based on both clinical history and pulmonary function (responsiveness to bronchodilators or to bronchial provocation tests) and had at least a partly controlled level of symptom control (16). For all subjects, inclusion criteria were a baseline FEV1 ≥80% of the predicted value, absence of respiratory infections in the previous 4 weeks, avoidance of inhaled corticosteroids, leukotriene antagonists, and antihistamines in the last 10 days, long-acting beta-agonists in the last 24 h, short-acting in the last 12 h. Parents signed a written informed consent; the Ethical Review Board of our Institution approved the study.
Study design
During a single session, patients underwent a medical visit, and parents completed a questionnaire on respiratory health including current respiratory symptoms and asthma medications. The child yielded information on EIS such as cough, dyspnea, and chest pain/tightness. Patients followed skin prick testing, oscillometry, and the fraction of exhaled nitric oxide (FENO) measurements in this order before baseline spirometry and exercise testing.
Skin prick tests
Sensitization to common inhaled and food allergens was tested by skin prick tests (SPTs) (ALK-Abellò, Milan, Italy) including house dust mites (Dpt and Dpf), molds (Alternaria alternata), dog and cat fur, Parietaria judaica, mixed grass pollens, mixed tree pollens, egg, and milk. Positive and negative controls were Histamine 0.1 mg/mL and glycerol solution, respectively. Positive SPTs were defined by a wheal size of at least 3 mm after subtraction of the negative control.
Oscillometry
Impedance measurements were obtained by oscillometry at a mono frequency of 8 Hz using the Resmon Pro Full device (ResTech, Milano, Italy). Children were seated, connected to the bacterial filter mouthpiece (DAR™, Covidien IIc, Mansfield, USA), and breathed normally wearing a nose clip whereas the technician supported their cheeks. Three baseline replicates from at least 10 breaths each, free of artifacts, with a coefficient of variance (CV) ≤15% for Rrs were accepted (6). Tidal volume (Vt), respiratory rate (RR), and minute ventilation (V’E) for each set of measurements were also recorded. Expiratory and inspiratory Rrs and Xrs were expressed both as absolute means and as z-scores using reference values derived from the same model of device that we used (11) according to recent recommendations for technical standards in oscillometry measurements (6). Impedance modulus (Zrs) was also computed for both inspiratory and expiratory phases. Mean within-breath differences (Rrsexp-Rrsinsp, Xrsexp-Xrsinsp) were calculated and reported as ΔRrs and ΔXrs, respectively.
FENO
FENO was measured with an electrochemical device (HyPair FENO, Medisoft Group, Sorinnes, Belgium). Children did single-breath maneuvers at constant expiratory pressure and flow of 50 mL/s as recommended. Mean values from two measurements agreeing within 10% from at least three expiratory maneuvers were calculated (17).
Spirometry
Spirometry was performed with a Quark PFT (Cosmed Srl, Rome, Italy) according to the ATS/ERS guidelines (18). The best FEV1 from three measurements was recorded. Dynamic volumes and flows were both percentages and z-scores of the predicted values (19).
Exercise testing
After baseline oscillometry and spirometry, patients underwent an incremental treadmill exercise test (20). Running was set at 6 km/h with a pendant of 10% until children reached the target heart rate of over 85% of the predicted maximum, calculated as 220-age years (21). Room temperature (20–24°C) and relative humidity (50%–60%) were kept stable during the procedure. After the exercise, subjects repeated spirometry at 1, 5, 10, 15, and 20 min, and oscillometry at 3 and 18 min. The lowest FEV1 was taken to calculate its decline from the baseline; EIB was defined as the fall in FEV1 ≥10% from baseline after exercise (4).
Following the 20-min spirometry, subjects received albuterol 200 µg (MDI with spacer); after a 15 min interval, they repeated oscillometry and spirometry, in this order.
Postexercise changes in impedance from baseline were calculated and reported depending on the parameter: the maximal rise in Rrs or the maximal decrease in Xrs from their respective baseline values. The changes were both percentages from baseline and differences from baseline z-scores. To calculate differences, z-scores for impedance parameters at every postexercise step were computed; then the z-scores for postexercise values were subtracted from their respective baseline values.
Statistical analysis
Continuous variables were assessed for normal distribution (K-S test); pulmonary function variables were expressed as both means ± SD and z-scores using reference values for spirometry (19) and impedance (11); categorical variables were given as numbers and percentages. Postexercise changes and bronchodilator responses were expressed as medians and interquartile ranges (IQRs). The Mann–Whitney U test was used for unpaired comparisons and the non-parametric ANOVA Friedman with post hoc Dunnett's test to assess differences between postexercise and baseline measurements. The Wilcoxon test was used for paired comparisons between postbronchodilator and last postexercise measurements.
Contingency tables (χ2 with Fisher's correction) were applied to compare categorical variables. Receiver operating characteristic (ROC) curves were constructed to analyze the relationship between sensitivity (Se) and 1-specificity (Sp) for impedance measurements in classifying EIB. The areas (AUC) under ROC curves were computed and used to identify the oscillometry parameters that better classified EIB. The cutoff values were determined by maximizing the Youden index. We also computed the cutoff values that maximize the Youden index among the ones providing Sp >Se. Spearman's Rho tests were used to assess correlations between continuous variables. The SPSS software (Version 27; SPSS Inc., Chicago, IL, USA) was used for statistical analyses. Two-tailed p values of <0.05 were regarded as statistically significant.
Results
Sixty-eight patients reporting EIS (age 10.8 ± 2.6 years, range 6.0–16.0, 41 males) completed all measurements and were allocated in two subsets of 34 subjects each (suspected asthma, and asthma). The asthmatic patients had more frequent cigarette smoke exposure at home, atopy, use of inhaled corticosteroids, and slightly lower FEV1/forced vital capacity (FVC) and FEF25–75 but no different FEV1, FeNO, or impedance measurements than patients suspected of asthma (Table 1). Following the exercise, the asthmatic patients presented EIB more frequently than the suspected asthmatics [18/34 (52.9%) vs. 2/34 (5.9%), p < 0.001]. The pooled group of patients reporting EIS consisted of 20/68 patients with EIB (29.4%) and 48/68 (70.6%) without EIB.
Postexercise ventilatory changes and oscillometry
Vt (L) increased after exercise among patients with EIB (baseline: 0.67 ± 0.26, 3’: 0.80 ± 0.36, 18’: 0.77 ± 0.33, p = 0.029) but not in patients without EIB (0.64 ± 0.26, 0.71 ± 0.29, and 0.66 ± 0.27, respectively, p = 0.226). By contrast, RR (beats/min) remained stable in both groups, resulting in a non-significative increase in V’E (L/min) from baseline in both patients with EIB (baseline: 15.7 ± 5.0, 3’: 18.0 ± 7.0, 18’: 16.8 ± 4.5, p = 0.250) and without EIB (16.2 ± 5.2, 17.5 ± 6.0, and 16.2 ± 5.3, respectively, p = 0.610). In the pooled population, ventilation changes from baseline did not correlate with those in oscillometry at 3’post exercise, though, at 18’ post exercise, the increases in Vt and V’E slightly correlated with the fall in the Xrsexp z-score (ρ=−0.31 and ρ=−0.30, respectively, p < 0.05).
Lung function by EIB outcomes
Subjects with EIB had lower baseline FEV1 and higher Rrsinsp than subjects without EIB (FEV1%: 94.9 ± 9.8 vs. 100.4 ± 9.9; Rrsinsp cmH2O/L/s: 5.71 ± 1.36 vs. 4.74 ± 1.56, p < 0.05 for both comparisons). The differences among groups were more evident considering z-scores of baseline oscillometry parameters (EIB vs. without EIB: z-score Rrsinsp, 1.18 ± 1.02 vs. 0.22 ± 1.08, p < 0.01; z-score Xrsexp, −0.43 ± 1.26 vs. 0.30 ± 0.79, p < 0.05).
Postexercise Rrs, Xrs, ΔRrs, and ΔXrs remained almost unchanged from baseline in patients without EIB but changed markedly in those with EIB shortly after exercise. In subjects with EIB, Rrsinsp, Rrsexp, Xrsinsp, and Xrsexp peaked early (3’), and remained quite steady except for Xrsinsp, which tended to recover afterward (Figure 1). Consequently, ΔXrs fell markedly 18 min following the exercise (Figure 2). Also, in EIB patients, ΔXrs recovered sharply after bronchodilation (BD) (−1.81 ± 1.60 vs. −0.52 ± 0.80 cmH2O × s/L, p < 0.001). Maximal changes in both spirometry and oscillometry measurements after exercise and after bronchodilation clearly divided subjects with vs. without EIB (Table 2).
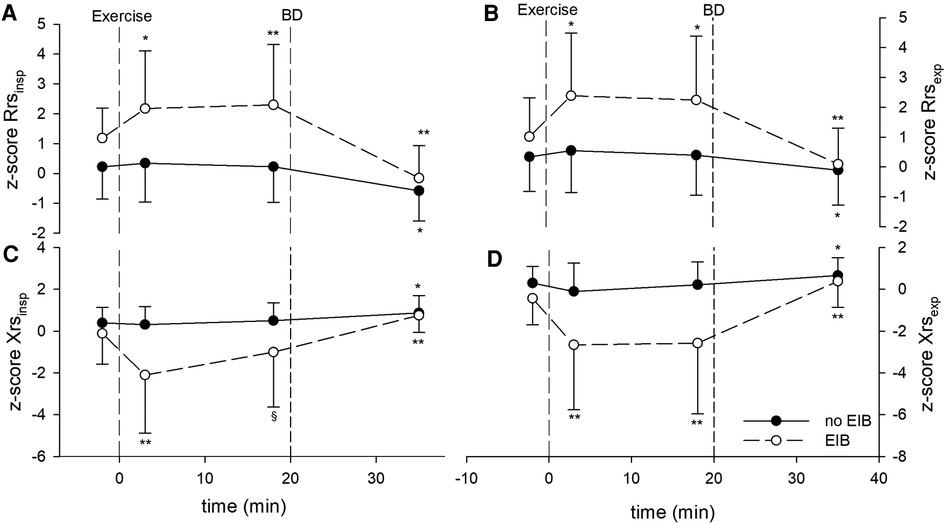
Figure 1. Z-scores of impedance parameters measured at baseline, after the exercise challenge, and after bronchodilation (BD) in patients with exercise-induced bronchoconstriction (EIB) and those without. (A) z-score Rrsinsp, (B) z-score Rrsexp, (C) z-score Xrsinsp, and (D) z-score Xrsexp. *p < 0.01 and **p < 0.001 for postexercise measurements (3 and 18 min) vs. baseline, or BD vs. 18 min after exercise.
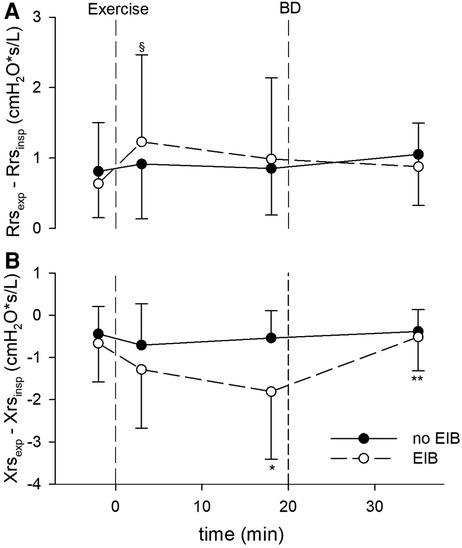
Figure 2. (A) Within-breath difference between expiratory and inspiratory resistance (ΔRrs), cmH2O/L/s, measured at baseline, after the exercise challenge, and after bronchodilation (BD) in patients with exercise-induced bronchoconstriction (EIB) and those without. §p < 0.05 vs. baseline. (B) Within-breath difference between expiratory and inspiratory reactance (ΔXrs), cmH2O/L/s, at the same intervals. *p < 0.01 vs. baseline; **p < 0.001 vs. 18 min after exercise.
Identifying EIB by oscillometry
In the pooled population, maximal percentage impedance changes correlated slightly better with maximal percentage changes in FEV1 than those in FEF25–75 after exercise, while the opposite was true after bronchodilation. All correlations improved by using maximal changes in Rrs and Xrs z-scores (Table 3).
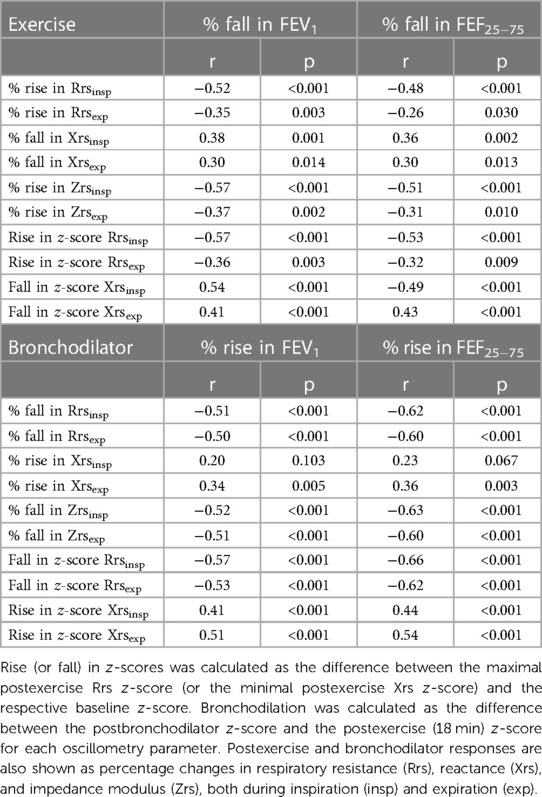
Table 3. Spearman's correlations between maximal changes in impedance and spirometry parameters after exercise testing and following bronchodilator administration in the pooled population (n = 68).
AUCs-ROC were higher when oscillometry parameters were expressed as z-scores. Inspiratory parameters resulted in better classification performances (Figure 3). Maximized Youden index cutoffs for EIB of oscillometry parameters leading to the highest AUC are reported in Table 4. More stringent cutoffs for impedance changes, i.e., providing Sp>Se, were an increase of 0.67 in z-score Rrsinsp (Se: 70.0%, Sp: 85.4%), a fall of −1.14 in z-score Xrsinsp (Se: 80.0%, Sp: 83.3%), and a 15.3% increase in Zrsinsp (Se: 75%, Sp: 85.4%).
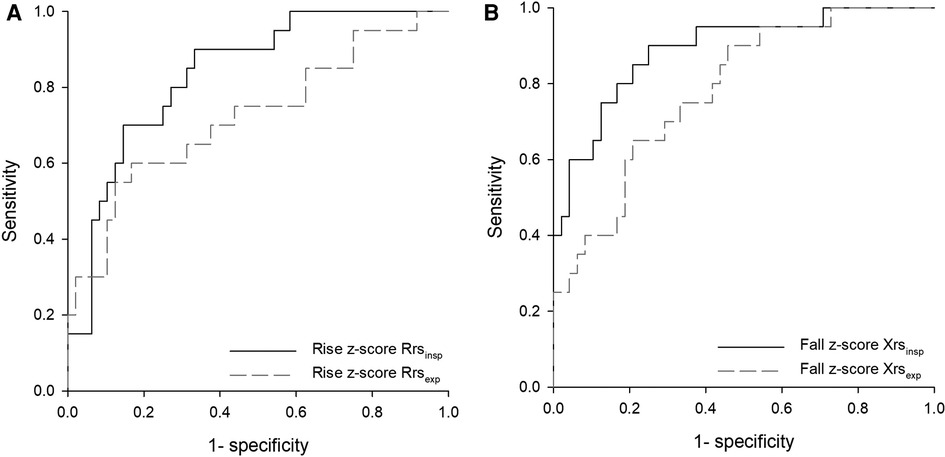
Figure 3. Receiver operating characteristic (ROC) curves for the postexercise change of respiratory impedance parameters measured at 8 Hz as predictors of exercise-induced bronchoconstriction (EIB). (A) Rise in z-scores of inspiratory and expiratory resistance (Rrsinsp, Rrsexp), areas under curves (AUCs): 0.84, p < 0.001; 0.72, p < 0.005. (B) Fall in z-scores of inspiratory and expiratory reactance (Xrsinsp, Xrsexp), AUCs 0.89 and 0.78, respectively; p < 0.001 for both.
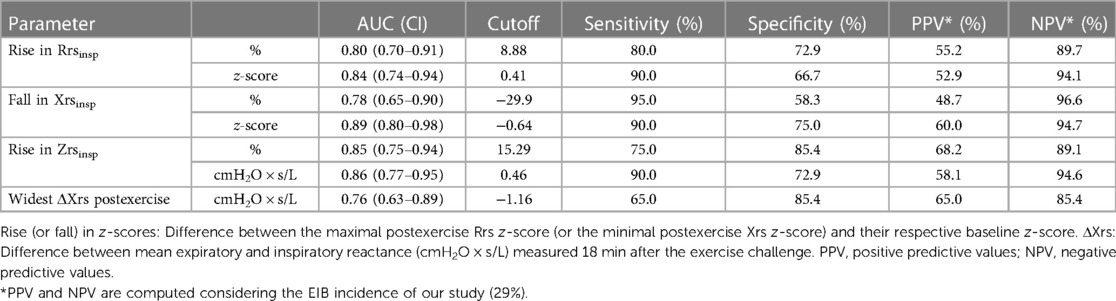
Table 4. EIB (fall in FEV1 ≥10%) by cutoff values of impedance for all the patients in the group (n = 68).
Postexercise outcome differences according to oscillometry and spirometry
The relationship between EIB defined by spirometry and changes in z-scores of inspiratory oscillatory parameters above the cutoffs maximizing the Youden index is described in Figure 4.
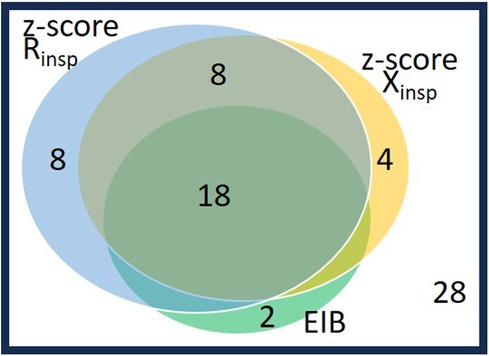
Figure 4. Venn diagram on the 40 patients with abnormal postexercise changes as defined either by spirometry or by oscillometry: 20 patients with EIB (fall in FEV1 ≥10%) and 20 patients without EIB and impedance changes beyond their cutoffs (n = 8: rise in Rrsinsp z-score>0.41; n = 4: fall in z-score Xrsinsp>−0.64; n = 8: both changes in Rrsinsp and Xrsinsp z-scores are abnormal). The other 28 patients (lower right corner), did not change by spirometry nor by oscillometry. See also maximized Youden index cutoffs for EIB of oscillometry parameters in Table 4.
Approximately 90% (18 subjects including 12 males) of the 20 spirometry-defined EIB subjects responded to exercise according to both z-scored Rrsinsp and Xrsinsp, whereas 10% (two boys) did not respond above these z-score cutoffs. Conversely, among the 48 subjects without EIB, eight (16.7%, all males) subjects responded to exercise according to both z-score Rrsinsp and z-score Xrsinsp, and 12 subjects (25.0%, nine males) responded in accordance to either z-score Rrsinsp or z-score Xrsinsp. These two subgroups had similar baseline lung function and body mass index (BMI) percentile to the remaining 28 (58.3%) non-responder subjects according to oscillometry and without EIB.
The eight subjects without EIB responding to exercise according to both oscillatory z-scores had larger bronchodilator responses (DFEV1: 7.0 ± 5.4 vs. 2.6 ± 4.0; DFEF25–75: 28.0 ± 19.7 vs. 10.5 ± 12.0, p < 0.05), male incidence (8/8 vs. 10/28, p < 0.001), but no different asthma incidence (4/8 vs. 9/28, p = 0. 422) than the 28 subjects not responding for both oscillometry and spirometry. The 12 subjects responding only for z-score Rrsinsp or z-score Xrsinsp did not differ from the non-responding group, except for a higher male incidence (9/12 vs. 10/28, p = 0.038).
Accepting as having “positive” postexercise oscillometry changes those subjects who had both z-scores beyond respective cutoffs (0.41 increase in z-score Rrsinsp and −0.64 fall in z-score Xrsinsp), sensitivity for EIB was 90.0% (18/20) and specificity, 83.3% (40/48).
Discussion
Our findings support the utility of impedance measurements following an exercise challenge in outpatients reporting EIS. The occurrence of EIB raised Rrs, decreased Xrs, and widened the within-breath difference in Xrs; such a difference reversed close to that baseline after bronchodilation with albuterol. To our knowledge, evaluating oscillometry parameters in terms of z-scores computed separately for inspiration and expiration and the within-breath analysis of reactance has not previously been described to assess the exercise challenge. Also, we added to the scarce data on exercise testing at 8 Hz (22), a suitable forcing frequency for the pediatric population.
Notwithstanding that exercise increases minute ventilation, preceding studies assessing breathing parameters in adults (23) and adolescents (24) have found uninfluential V’E (23) and changes in Vt, RR, and V’E on oscillometry measurements for assessing EIB. Neither did we find a relationship between changes in ventilation and those in oscillometry early after exercise; still, the rise in Vt and V’E did correlate slightly with the fall from baseline in Xrsexp (but not with Xrsinsp) 18 min after the exercise ended.
Oscillometry by EIB outcomes
Several studies have reported changes in respiratory impedance following exercise in subjects with asthma or asthma-like symptoms including young adults (23, 25, 26), children, adolescents (22, 24, 27–31), and preschool children (32, 33). These studies showed larger dynamic impedance changes from the few minutes following exercise in subsets of asthmatic patients or wheezy children than in control subjects, selected either as healthy (23, 33) or without ongoing airway hyperresponsiveness (26).
In our population of subjects reporting EIS, changes in oscillometry were not statistically significant in subjects without EIB. This is not unexpected, as there is a consistent percentage of subjects reporting EIS whose response to exercise cannot be objective by lung function tests (24, 34). BD improved oscillometry parameters in this group; however, improvements remained within the cutoff for BD response (relative percent change and z-score change) of healthy children aged 2–13 years (10).
Time changes in oscillometry parameters in EIB (spirometry assessed) subjects reported by previous studies (23, 24, 26, 27) showed an early worsening (at 5 min) of both Rrs and Xrs after exercise and a faster Xrs recovery than of Rrs. This suggests an earlier functional recovery of less proximal airways than of the relatively proximal airways. Our data hold up the early change in impedance parameters as shortly as 3 min after exercise and the slower Rrs recovery than Xrs in EIB subjects.
Both Rrsinsp and Rrsexp remained high after 18 min post exercise. Xrsinsp gradually recovered while Xrsexp remained persistently decreased, i.e., the Xrs recovery was supported from Xrsinsp only. This implies that the within-breath difference (ΔXrs) increased from baseline to the late postexercise step. Xrs reflects communicating lung volume and compliance (35) and is affected by ventilation heterogeneity. It changes minimally during tidal breathing in healthy subjects while it may drop sharply during expiration in subjects with tidal peripheral airway collapse (35, 36). Our data suggest that ΔXrs widens with acute airway obstruction, mostly peripheral, following exercise. These may be the results of different mechanisms. The development of flow limitation in some airways (13), the lower elastic recoil, and higher collapsibility of the small airways at lower volumes (37) leads to higher ΔXrs both directly and indirectly by increasing heterogeneity during expiration. Our data showed that increased ΔXrs was not the result of a further Xrsexp decrease but of a faster Xrsinsp recovery from the early postchallenge step fall. Measuring within-breath Xrs components may provide additional information for the analysis of changes in lung mechanics after exercise.
Identifying EIB by oscillometry
Changes in spirometry and oscillometry parameters were correlated but not totally overlapped.
Changes in z-scores rather than percentages from the baseline better assessed postexercise and postbronchodilator changes as detected by spirometry. This was especially verified for Xrs as drawbacks from using percentages of Xrs are especially evident when values close to zero are recorded (6).
Changes in z-scores of inspiratory rather than in expiratory oscillometry parameters best predicted EIB. Therefore, we agree with other studies reporting that inspiratory impedance parameters indicate EIB better than expiratory (24, 29). Conversely, in school-age children born extremely preterm, inspiratory impedance did not mirror EIB, suggesting a distinct prematurity-driven airway reactivity (38).
Percentage changes from baseline of Zrsinsp performed best against spirometry (Tables 3, 4), supporting the idea that this parameter, including contributions of both Rrsinsp and Xrsinsp changes, can better describe the changes in lung condition (24). However, reference equations are not available for Zrsinsp (and their intrabreath components) yet. Therefore, we cannot verify if using z-score Zrsinsp can further improve agreement with spirometry. The maximum postexercise widening of ΔXrs was also related to EIB even if it provided lower AUC than postexercise changes in z-scores of Rrsinsp or Xrsinsp. Reference values of ΔXrs are not available. Reporting ΔXrs as a z-score may improve the correlation with spirometry parameters when assessing responses to the exercise challenge.
Previous cutoffs for oscillometry with spirometry-based bronchial challenges in children refer to the methacholine testing, which (using different forced frequencies and devices) varies from a 45%–70% increase in Rrs and a 50%–80% drop in Xrs (6). Instead, cutoffs for oscillometry with exercise testing are less well established. A recent study described a 27% increase in R5 and a 47% decrease in X5 as predictors of EIB, based on a fall in FEV1 ≥10% in asthmatic adults (23). Another study disclosed an increase of 0.035 kPa/L/s in R5 after 5 min of exercise (and a 0.055 kPa/L/s as maximum increase) to distinguish asthmatic patients with vs. those without airway hyperresponsiveness to methacholine (26). The most recent study, in asthmatic children, reported a 14.1% increase in R5 within 30 min post exercise as a diagnostic of EIB (31). Differently, among adolescents with or without reported exercise-induced dyspnea, cutoffs were drawn from 95% oscillometry changes in those regarded as healthy. Such an approach identified z-score increases in Rrs of 0.68 and Xrs of 1.76 as cutoffs for EIB (24). Our cutoff providing Sp>Se for z-score Rrsinsp is close to the one determined on healthy children (0.67 vs. 0.68), though our z-score Xrsinsp at 8 Hz is lower than their change in Xrs5 (1.14 vs. 1.76), as expected using higher stimulation frequencies and considering only the inspiratory phase.
Postexercise outcome differences according to oscillometry and spirometry
There is increasing evidence that spirometry and oscillometry are not always in accordance as they describe lung mechanics under different conditions (6, 12, 37). Spirometry requires a high lung volume maneuver and FEV1 reflects mainly airflow resistance of proximal airways (39). Oscillometry requires tidal breaths at resting lung volume, is also sensitive to peripheral airways, and is deemed complemental to spirometry for assessing airway responsiveness (6, 12). Changes in inspiratory Rrs well document the attenuation effect of EIB in spirometry with deep inspirations in asthmatic children in contrast with healthy controls (29). Small degrees of airway narrowing in healthy children have been attributed to transient hyperemia of the airway wall leading to local edema (29), a mechanism that could explain why the impedance increased after exercise in some of our subjects without EIB.
The best agreement between postexercise changes in spirometry (EIB) and oscillometry was seen in subjects showing changes beyond their identified cutoffs in both z-scores of Rrsinsp and Xrsinsp. This supports the idea of the importance of considering changes in both Rrs and Xrs and correcting for anthropometric factors. However, as expected the two techniques did not lead to identical results because of different physiological lung conditions during the measurements and different difficulties in performing the measurements (6–8, 12). Two subjects who developed EIB (both males with asthma) did not show large changes in either z-scores of Rrsinsp or Xrsinsp. On the contrary, large changes in these two parameters occurred in eight male subjects without EIB who had evenly distributed asthma diagnoses (4:4).
Incongruent growth of the airway's caliber relative to the growth of lung parenchyma, known as airway dysanapsis (characterized by low FEV1/FVC despite normal FEV1 and FVC) is relevant in children (39, 40). Boys have smaller airways than girls proportional to their lung growth (40, 41); obesity is associated with airway dysanapsis in children with or without asthma, and this airway–lung incongruence is linked to worse asthma control (40). Our eight male subjects without EIB who had large changes in both z-scores of Rrsinsp and Xrsinsp yielded no different baseline lung function (including FEV1/FVC) nor BMI percentile than the remaining subjects without EIB. Because these eight subjects had more changes in inspiratory reactance than the other subjects without EIB, they could have peripheral airway obstruction unrevealed by spirometry. Gender differences in small airway physiology have been reported among asthmatic subjects, as larger methacholine-induced gas trapping was found in males than females (42). Our eight subjects also showed greater changes in spirometry than the non-responding subjects after BD. An alternative hypothesis is that these eight boys, having a high airway hyperresponsiveness, could be prone to smooth-muscle relaxation after deep inhalations from FVC maneuvers. If so, the effect of exercise is more evident during quiet breathing and easily identified by oscillometry as the maneuver required for spirometry partially reverses the changes in lung mechanics.
Limitations
We acknowledge the following limitations. We involved outpatients attending the pulmonology service for EIS in a real-life approach, thus, we are cautious about comparing results with other settings that also involve healthy controls. Still, oscillometry parameters drive their use for screening patients with EIS in similar outpatient settings. The mono frequency of 8 Hz that we used allows the exploration of both proximal and less proximal airways; thus, the changes in Rrsinsp and Xrsinsp evinced the involvement of different airway segments during EIB in our study.
Physiological ventilatory responses to exercise could influence our oscillometry measurements. However, the expiratory reactance was the sole parameter slightly influenced by Vt whereas no inspiratory impedance components, i.e., those better identifying EIB, correlated with the ventilatory parameters.
The broncho-motor effect of deep inspiration with forced expiratory maneuvers could also modify our measures (29, 43); to minimize this effect we did oscillometry maneuvers before those of spirometry. However, repeated maximal inspiratory maneuvers after the exercise challenge might result in broncho-protection leading to underestimating EIB, especially in asthmatic subjects whose response to deep inspirations depends on the degree of airway obstruction (43).
Our subjects ran without a facemask, despite it being preferred to inhale medical dry air for the exercise testing protocol (3, 4); this might decrease the number of positive test outcomes. Because more than half of our asthmatic patients had EIB, the overall blunt effect of deep inhalations on the broncho-motor tone or the environmental conditions during the test was probably modest.
We calculated ΔRrs and ΔXrs as mean within-breath differences (13–15), rather than computing the difference between the end-expiratory and the end-inspiratory impedance (i.e., both at zero flow). Although this recently suggested approach (44) has been indicated as potentially more sensitive to changes due to its reduced susceptibility to changes in breathing flow, our data exhibited very limited dependence on breathing patterns, which further strengthens the credibility of our findings.
In conclusion, we found marked changes in oscillometry parameters and raised within-breath differences in reactance among pediatric outpatients with self-reported EIS presenting EIB. Baseline oscillometry values were restored after the bronchodilator. The high agreement of z-scores of inspiratory parameters with spirometry supports their use for clinical evaluation although larger studies are needed to confirm the validity of the identified cutoff in a more general population. More studies are needed to establish the clinical role of within-breath differences in impedance during EIB in these children.
Data availability statement
The original contributions presented in the study are included in the article/Supplementary Material, further inquiries can be directed to the corresponding authors.
Ethics statement
The studies involving humans were approved by the Ethical Review Board of S. Andrea University Hospital, Rome. The studies were conducted in accordance with the local legislation and institutional requirements. Written informed consent for participation in this study was provided by the participants’ legal guardians/next of kin.
Author contributions
MB: Conceptualization, Formal analysis, Investigation, Methodology, Software, Visualization, Writing—original draft, Writing—review and editing, Supervision. CV: Conceptualization, Formal analysis, Visualization, Writing—review and editing. MC: Data curation, Investigation, Visualization, Writing—review and editing. ME: Conceptualization, Methodology, Supervision, Visualization, Writing—review and editing. PPo: Conceptualization, Supervision, Visualization, Writing—review and editing. MM: Data curation, Investigation, Visualization, Writing—review and editing. GR: Data curation, Investigation, Visualization, Writing—review and editing. JP: Investigation, Supervision, Visualization, Writing—review and editing. PPa: Conceptualization, Funding acquisition, Supervision, Visualization, Writing—review and editing.
Funding
The author(s) declare that no financial support was received for the research, authorship, and/or publication of this article.
Conflict of interest
PP is member of the board of directors of RESTECH srl, which manufactures devices for the measurement of lung function based on oscillometry.
The remaining authors declare that the research was conducted in the absence of any commercial or financial relationships that could be construed as a potential conflict of interest.
Publisher's note
All claims expressed in this article are solely those of the authors and do not necessarily represent those of their affiliated organizations, or those of the publisher, the editors and the reviewers. Any product that may be evaluated in this article, or claim that may be made by its manufacturer, is not guaranteed or endorsed by the publisher.
References
1. Aggarwal B, Mulgirigama A, Berend N. Exercise-induced bronchoconstriction: prevalence, pathophysiology, patient impact, diagnosis and management. NPJ Prim Care Respir Med. (2018) 28(1):31. doi: 10.1038/s41533-018-0098-2
2. Bonini M, Palange P. Exercise-induced bronchoconstriction: new evidence in pathogenesis, diagnosis and treatment. Asthma Res Pract. (2015) 1:2. doi: 10.1186/s40733-015-0004-4
3. Weiler JM, Brannan JD, Randolph CC, Hallstrand TS, Parsons J, Silvers W, et al. Exercise-induced bronchoconstriction update-2016. J Allergy Clin Immunol. (2016) 138(5):1292–1295.e36. doi: 10.1016/j.jaci.2016.05.029
4. Parsons JP, Hallstrand TS, Mastronarde JG, Kaminsky DA, Rundell KW, Hull JH, et al. American thoracic society subcommittee on exercise-induced bronchoconstriction. An official American thoracic society clinical practice guideline: exercise-induced bronchoconstriction. Am J Respir Crit Care Med. (2013) 187(9):1016–27. doi: 10.1164/rccm.201303-0437ST
5. Saglani S, Menzie-Gow AN. Approaches to asthma diagnosis in children and adults. Front Pediatr. (2019) 7:148. doi: 10.3389/fped.2019.00148
6. King GG, Bates J, Berger KI, Calverley P, de Melo PL, Dellacà RL, et al. Technical standards for respiratory oscillometry. Eur Respir J. (2020) 55(2):1900753. doi: 10.1183/13993003.00753-2019
7. Galant SP, Komarow HD, Shin HW, Siddiqui S, Lipworth BJ. The case for impulse oscillometry in the management of asthma in children and adults. Ann Allergy Asthma Immunol. (2017) 118(6):664–71. doi: 10.1016/j.anai.2017.04.009
8. Skylogianni E, Douros K, Anthracopoulos MB, Fouzas S. The forced oscillation technique in paediatric respiratory practice. Paediatr Respir Rev. (2016) 18:46–51. doi: 10.1016/j.prrv.2015.11.001
9. Hall GL, Sly PD, Fukushima T, Kusel MM, Franklin PJ, Horak F Jr, et al. Respiratory function in healthy young children using forced oscillations. Thorax. (2007) 62(6):521–6. doi: 10.1136/thx.2006.067835
10. Calogero C, Simpson SJ, Lombardi E, Parri N, Cuomo B, Palumbo M, et al. Respiratory impedance and bronchodilator responsiveness in healthy children aged 2−13 years. Pediatr Pulmonol. (2013) 48(7):707–15. doi: 10.1002/ppul.22699
11. Ducharme FM, Smyrnova A, Lawson CC, Miles LM. Reference values for respiratory sinusoidal oscillometry in children aged 3−17 years. Pediatr Pulmonol. (2022) 57(9):2092–102. doi: 10.1002/ppul.25984
12. Kaminsky DA, Simpson SJ, Berger KI, Calverley P, de Melo PL, Dandurand R, et al. Clinical significance and applications of oscillometry. Eur Respir Rev. (2022) 31(163):210208. doi: 10.1183/16000617.0208-2021
13. Dellacà RL, Santus P, Aliverti A, Stevenson N, Centanni S, Macklem PT, et al. Detection of expiratory flow limitation in COPD using the forced oscillation technique. Eur Respir J. (2004) 23(2):232–40. doi: 10.1183/09031936.04.00046804
14. Dellacà RL, Pompilio PP, Walker PP, Duffy N, Pedotti A, Calverley PM. Effect of bronchodilation on expiratory flow limitation and resting lung mechanics in COPD. Eur Respir J. (2009) 33(6):1329–37. doi: 10.1183/09031936.00139608
15. Zannin E, Nyilas S, Ramsey KA, Latzin P, Dellaca’ RL. Within-breath changes in respiratory system impedance in children with cystic fibrosis. Pediatr Pulmonol. (2019) 54(6):737–42. doi: 10.1002/ppul.24281
16. Global Initiative for Asthma. 2022 GINA Report, Global Strategy for Asthma Management and Prevention. Available at: https://ginasthma.org (Accessed April 18, 2023).
17. American Thoracic Society; European Respiratory Society. ATS/ERS recommendations for standardized procedures for the online and offline measurement of exhaled lower respiratory nitric oxide and nasal nitric oxide, 2005. Am J Respir Crit Care Med. (2005) 171(8):912–30. doi: 10.1164/rccm.200406-710ST
18. Graham BL, Steenbruggen I, Miller MR, Barjaktarevic IZ, Cooper BG, Hall GL, et al. Standardization of spirometry 2019 update. An official American Thoracic Society and European Respiratory Society Technical Statement. Am J Respir Crit Care Med. (2019) 200(8):e70–88. doi: 10.1164/rccm.201908-1590ST
19. Quanjer PH, Stanojevic S, Cole TJ, Baur X, Hall GL, Culver BH, et al. ERS global lung function initiative. Multi-ethnic reference values for spirometry for the 3−95-yr age range: the global lung function 2012 equations. Eur Respir J. (2012) 40(6):1324–43. doi: 10.1183/09031936.00080312
20. Barreto M, Villa MP, Olita C, Martella S, Ciabattoni G, Montuschi P. 8-isoprostane in exhaled breath condensate and exercise-induced bronchoconstriction in asthmatic children and adolescents. Chest. (2009) 135(1):66–73. doi: 10.1378/chest.08-0722
21. Crapo RO, Casaburi R, Coates AL, Enright PL, Hankinson JL, Irvin CG, et al. Guidelines for methacholine and exercise challenge testing−1999. This official statement of the American Thoracic Society was adopted by the ATS board of directors, July 1999. Am J Respir Crit Care Med. (2000) 161(1):309–29. doi: 10.1164/ajrccm.161.1.ats11-99
22. Timonen KL, Randell JT, Salonen RO, Pekkanen J. Short-term variations in oscillatory and spirometric lung function indices among school children. Eur Respir J. (1997) 10(1):82–7. doi: 10.1183/09031936.97.10010082
23. Seccombe LM, Peters MJ, Buddle L, Farah CS. Exercise-induced bronchoconstriction identified using the forced oscillation technique. Front Physiol. (2019) 10:1411. doi: 10.3389/fphys.2019.01411
24. Veneroni C, Pompilio PP, Alving K, Janson C, Nordang L, Dellacà R, et al. Self-reported exercise-induced dyspnea and airways obstruction assessed by oscillometry and spirometry in adolescents. Pediatr Allergy Immunol. (2022) 33(1):e13702. doi: 10.1111/pai.13702
25. Evans TM, Rundell KW, Beck KC, Levine AM, Baumann JM. Airway narrowing measured by spirometry and impulse oscillometry following room temperature and cold temperature exercise. Chest. (2005) 128(4):2412–9. doi: 10.1378/chest.128.4.2412
26. Lee JH, Lee YW, Shin YS, Jung YH, Hong CS, Park JW. Exercise-induced airway obstruction in young asthmatics measured by impulse oscillometry. J Investig Allergol Clin Immunol. (2010) 20(7):575–81.21313998
27. Driessen JM, Nieland H, van der Palen JA, van Aalderen WM, Thio BJ, de Jongh FH. Effects of a single dose inhaled corticosteroid on the dynamics of airway obstruction after exercise. Pediatr Pulmonol. (2011) 46(9):849–56. doi: 10.1002/ppul.21447
28. Ozdogan S, Hsia D, Elisan I, Johnson C, Hardy K. A comparison of impulse oscillometry to spirometry in the evaluation of exercise induced bronchoconstriction in children with asthma. J Pulm Respir Med. (2014) 4:180. doi: 10.4172/2161-105X.1000180
29. Schweitzer C, Abdelkrim IB, Ferry H, Werts F, Varechova S, Marchal F. Airway response to exercise by forced oscillations in asthmatic children. Pediatr Res. (2010) 68(6):537–41. doi: 10.1203/PDR.0b013e3181f851d2
30. Junior MAV C, Filho EAR, Tenório RCE, Albuquerque CG, Barbosa LC, Quirino PGC, et al. Comparison between impulse oscillometry parameters and spirometry for the diagnosis of exercise-induced bronchoconstriction in asthmatic children and adolescents. Pediatr Pulmonol. (2022) 57(10):2398–404. doi: 10.1002/ppul.26046
31. Gupta S, Mukherjee A, Gupta S, Jat KR, Sankar J, Lodha R, et al. Impulse oscillometry (IOS) for detection of exercise induced bronchoconstriction in children with asthma ages 6−15 years. J Asthma. (2023) 60(7):1336–46. doi: 10.1080/02770903.2022.2145219
32. Lenney W, Milner AD. Recurrent wheezing in the preschool child. Arch Dis Child. (1978) 53(6):468–73. doi: 10.1136/adc.53.6.468
33. Malmberg LP, Mäkelä MJ, Mattila PS, Hammarén-Malmi S, Pelkonen AS. Exercise-induced changes in respiratory impedance in young wheezy children and nonatopic controls. Pediatr Pulmonol. (2008) 43(6):538–44. doi: 10.1002/ppul.20805
34. Price OJ, Ansley L, Bikov A, Hull JH. The role of impulse oscillometry in detecting airway dysfunction in athletes. J Asthma. (2016) 53(1):62–8. doi: 10.3109/02770903.2015.1063647
35. Milne S, Jetmalani K, Chapman DG, Duncan JM, Farah CS, Thamrin C, et al. Respiratory system reactance reflects communicating lung volume in chronic obstructive pulmonary disease. J Appl Physiol (1985). (2019) 126(5):1223–31. doi: 10.1152/japplphysiol.00503.2018
36. Kelly VJ, Brown NJ, Sands SA, Borg BM, King GG, Thompson BR. Effect of airway smooth muscle tone on airway distensibility measured by the forced oscillation technique in adults with asthma. J Appl Physiol (1985). (2012) 112(9):1494–503. doi: 10.1152/japplphysiol.01259.2011
37. Veneroni C, Van Muylem A, Malinovschi A, Michils A, Dellaca’ RL. Closing volume detection by single-breath gas washout and forced oscillation technique. J Appl Physiol (1985). (2021) 130(4):903–13. doi: 10.1152/japplphysiol.00440.2020
38. Hamon I, Varechova S, Vieux R, Ioan I, Bonabel C, Schweitzer C, et al. Exercise-induced bronchoconstriction in school-age children born extremely preterm. Pediatr Res. (2013) 73(4 Pt 1):464–8. doi: 10.1038/pr.2012.202
39. Stanojevic S, Kaminsky DA, Miller MR, Thompson B, Aliverti A, Barjaktarevic I, et al. ERS/ATS technical standard on interpretive strategies for routine lung function tests. Eur Respir J. (2022) 60(1):2101499. doi: 10.1183/13993003.01499-2021
40. Forno E, Weiner DJ, Mullen J, Sawicki G, Kurland G, Han YY, et al. Obesity and airway dysanapsis in children with and without asthma. Am J Respir Crit Care Med. (2017) 195(3):314–23. doi: 10.1164/rccm.201605-1039OC
41. Taussig LM, Cota K, Kaltenborn W. Different mechanical properties of the lung in boys and girls. Am Rev Respir Dis. (1981) 123(6):640–3. doi: 10.1164/arrd.1981.123.6.640
42. Cohen J, Douma WR, Ten Hacken NH, Oudkerk M, Postma DS. Physiology of the small airways: a gender difference? Respir Med. (2008) 102(9):1264–71. doi: 10.1016/j.rmed.2008.04.007
43. Schweitzer C, Moreau-Colson C, Marchal F. Respiratory impedance response to a deep inhalation in asthmatic children with spontaneous airway obstruction. Eur Respir J. (2002) 19(6):1020–5. doi: 10.1183/09031936.02.00992001
Keywords: forced oscillation technique (FOT), respiratory impedance, exercise-induced bronchoconstriction (EIB), exercise-induced symptoms (EIS), spirometry, peripheral airways, asthma, children
Citation: Barreto M, Veneroni C, Caiulo M, Evangelisti M, Pompilio PP, Mazzuca MC, Raponi G, Pagani J and Parisi P (2024) Within-breath oscillometry for identifying exercise-induced bronchoconstriction in pediatric patients reporting symptoms with exercise. Front. Pediatr. 11:1324413. doi: 10.3389/fped.2023.1324413
Received: 19 October 2023; Accepted: 27 December 2023;
Published: 11 January 2024.
Edited by:
Milos Jesenak, Comenius University, SlovakiaReviewed by:
Christophe Delclaux, Hôpital Robert Debré, FranceCyril Etienne Schweitzer, Université de Lorraine, France
© 2024 Barreto, Veneroni, Caiulo, Evangelisti, Pompilio, Mazzuca, Raponi, Pagani and Parisi. This is an open-access article distributed under the terms of the Creative Commons Attribution License (CC BY). The use, distribution or reproduction in other forums is permitted, provided the original author(s) and the copyright owner(s) are credited and that the original publication in this journal is cited, in accordance with accepted academic practice. No use, distribution or reproduction is permitted which does not comply with these terms.
*Correspondence: Mario Barreto bWFyaW8uYmFycmV0b0Bmb25kYXppb25lLnVuaXJvbWExLml0 Pasquale Parisi cGFzcXVhbGUucGFyaXNpQHVuaXJvbWExLml0