- Department of Orthopedics, The Third Affiliated Hospital of Zhengzhou University, Zhengzhou, Henan Province, China
Objective: This study offers a bibliometric analysis of the current situation, hotspots, and cutting-edge domains of genetic factors of adolescent idiopathic scoliosis (AIS).
Methods: All publications related to genetic factors of AIS from January 1, 1992, to February 28, 2023, were searched from the Web of Science. CiteSpace software was employed for bibliometric analysis, collecting information about countries, institutions, authors, journals, and keywords of each article.
Results: A cumulative number of 308 articles have been ascertained. Since 2006, publications relating to genetic factors of AIS have significantly increased. China leads in both productivity and influence in this area, with the Chinese Academy of Medical Sciences being the most productive institution. The most prolific scholars in this field are Y. Qiu and Z. Z. Zhu. The publications that contributed the most were from Spine and European Spine Journal. The most prominent keywords in the genetic factors of AIS were “fibrillin gene”, “menarche”, “calmodulin”, “estrogen receptor gene”, “linkage analysis”, “disc degeneration”, “bone mineral density”, “melatonin signaling dysfunction”, “collagen gene”, “mesenchymal stem cell”, “LBX1”, “promoter polymorphism”, “Bone formation”, “cerebrospinal fluid flow” and “extracellular matrix”.
Conclusion: This analysis provides the frontiers and trends of genetic factors in AIS, including relevant research, partners, institutions and countries.
Introduction
Adolescent idiopathic scoliosis (AIS) is a tridimensional structural abnormality of the spine; that impacts adolescents from 10 years old to maturity (1, 2). The main diagnosis is based on the coronal curved Cobb angle, which must be >10° on standard anterior and posterior radiographs (3). AIS affects 1%–4% of teenagers (2), and is more common in girls (3). It can lead to high and low shoulders, trunk displacement, and other appearance deformities (2, 4). The development of AIS involves a variety of factors such as genetics, tissues, spinal biomechanics, and hormones (5). Of these, genetics is the major factor. Early identification of relevant genetic variants can help in the diagnosis and prevention of the disease.
Bibliometrics evaluates published research and predicts research trends (6). It can analyze scientific movements, including relationships among countries, institutions, authors, journals, and keywords (7), and has been widely used in various fields. The knowledge map examines the progress and boundaries of the discipline, facilitating researchers to understand the research hotspots and guiding them in their research directions.
Although various studies have focused on the genetic factors of AIS, bibliometric analysis remains scarce. The purpose of this study is to organize relevant studies of AIS genetic factors from January 1, 1992, to February 28, 2023. It summarizes genetic factors and provides researchers with a macro perspective in this area of research. The study includes analysis of the quantity of published articles, associations and symbioses between authors or countries, co-citation of references, and hot areas associated with keywords in the global research and analysis of genetic factors of AIS.
Method
Data sources
All data are obtained from the core collection of the Internet database Web of Science (WoS). The literature on genetic factors of AIS published from January 1, 1992, to February 28, 2023, was searched on WoS. Retrieval strategy: TS = (“adolescent idiopathic scoliosis”) and TS = (gene or DNA or “base sequence” or “nucleic acid” or “copy number variants” or “single nucleotide polymorphism” or “SNP”). The database is updated daily and data are collected in a 24-hour period to prevent potential bias. Primary researches and literature reviews were included. Letters, corrections, meeting abstracts, editorial materials, proceeding papers and book chapters were excluded.
Data analysis
Two separate evaluators (X.W.J. and M.X.Z.) conducted a comprehensive review of the study based on the title and abstract. The compilation and export of references and citations were done in a simple text format. Every bibliographic entry includes a title, author, keywords, abstract and references.
CiteSpace (a Java-based software) was used to perform a bibliometric analysis of the WoS Core Collection. It visualizes information and is frequently employed for evaluating patterns in research (7). It is capable of recognizing leading authors, institutions, and nations, and forming collaborative research connections among these entities. To examine research collaborative connections, a network analysis of references, scholars, and articles using co-citation was proceeded. Extend the co-word network analysis of keywords to provide up-to-the-minute viewpoints and research trends. Co-citation relationships show the recurrence with which a terminology or citation is mentioned over a given period. Co-occurrence surges manifest the recurrence with which a keyword or citation appears over a period of time.
The dimensions of the nodes in the visual network graph represent the extent of co-occurrence or recurrence. The line between nodes is the relationship of cooperation, co-occurrence and co-citation. The width and the extent of the lines indicate the proximity of cooperation between nations, organizations, and scholars. The lines depict the relationship among the nodes. Their color shows the publication year.
This study is primarily descriptive. Absence of statistics analysis, the quantity and proportion (%) of every index demonstrates the dispersion and change trends of different years, countries, organizations, publications, and scholars.
Results
Publication outputs
Between January 1, 1992, and February 28, 2023, we retrieved a total of 330 records, of which 308 articles were eligible based on inclusion and exclusion criteria. With this group, there were 270 articles classified as original research and 38 articles categorized as reviews. English publications overwhelmingly dominated, accounting for more than 99% (306), while French and German publications were represented by only 1 paper each. From 1992 to 2022, research on AIS genetic factors exhibited a consistent upward trend, with an annual growth rate of 5.93%. The year 2019 stood out as the peak, with the highest number of papers at 40 (Figure 1). Initially, a limited number of publications were observed from 1992 to 2005, but since 2006, there has been a rapid increase. In total, 308 publications garnered 5,771 citations.
Country/region distribution
40 countries have published articles on AIS genetic factors. China was involved in the publication of most articles (50.3%), followed by the United States (25%), Canada (9.4%), Japan (8.8%) and the United Kingdom (4.2%). Figure 2 shows the geographic distribution of publications. Figure 3 shows the cooperation between different regions. Table 1 shows that the H-index (a common indicator of academic influence) (8) of China, the United States, Canada, Japan and the United Kingdom is 28, 27, 14, 18 and 7 respectively.
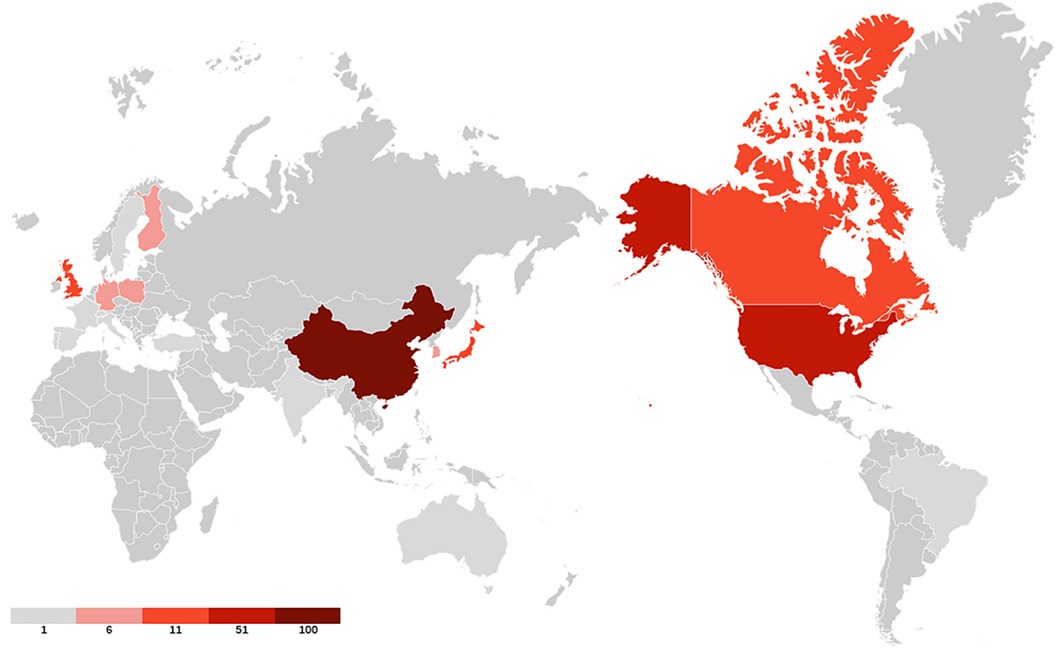
Figure 2. The geographic distribution of publications. Darker colors indicate greater number of publications.
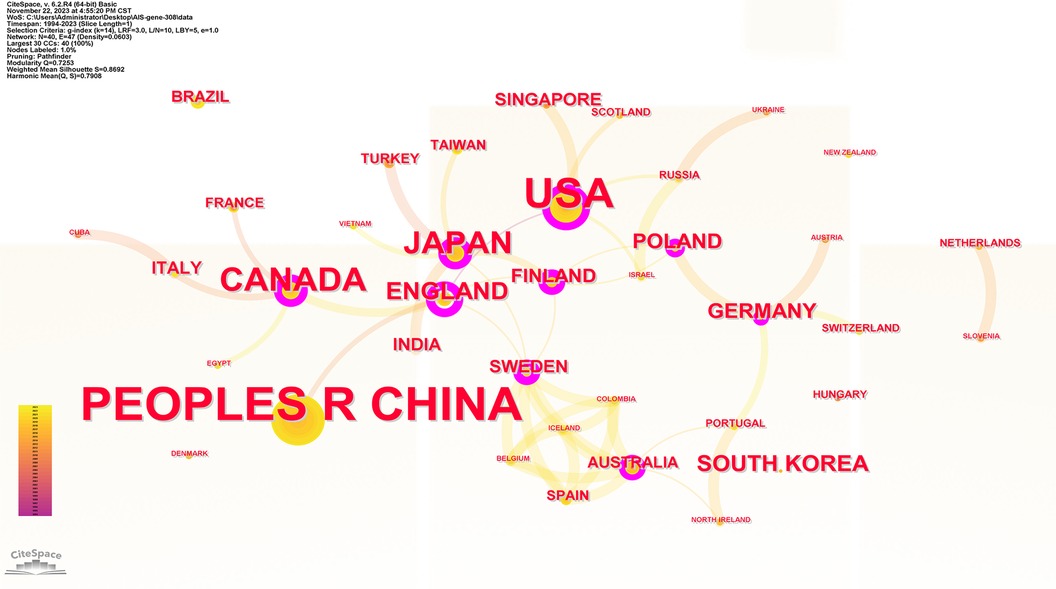
Figure 3. Collaboration network of productive countries/regions. Nodes represent countries/regions, and lines indicate co-citation relationships. Node colors correspond to different years. Larger nodes indicate higher publication numbers.
Institution analysis
Table 2 shows the ranking of institutions involved in publishing articles on genetic factors in AIS. Chinese Academy of Medical Sciences was involved in the largest number of published papers (69; 22.4%), followed by Nanjing University (61; 19.8%), University of Hong Kong (30; 9.7%), RIKEN (21; 6.8%), University of Texas System (21; 6.8%). Figure 4 presents the cooperation among institutions in this field.
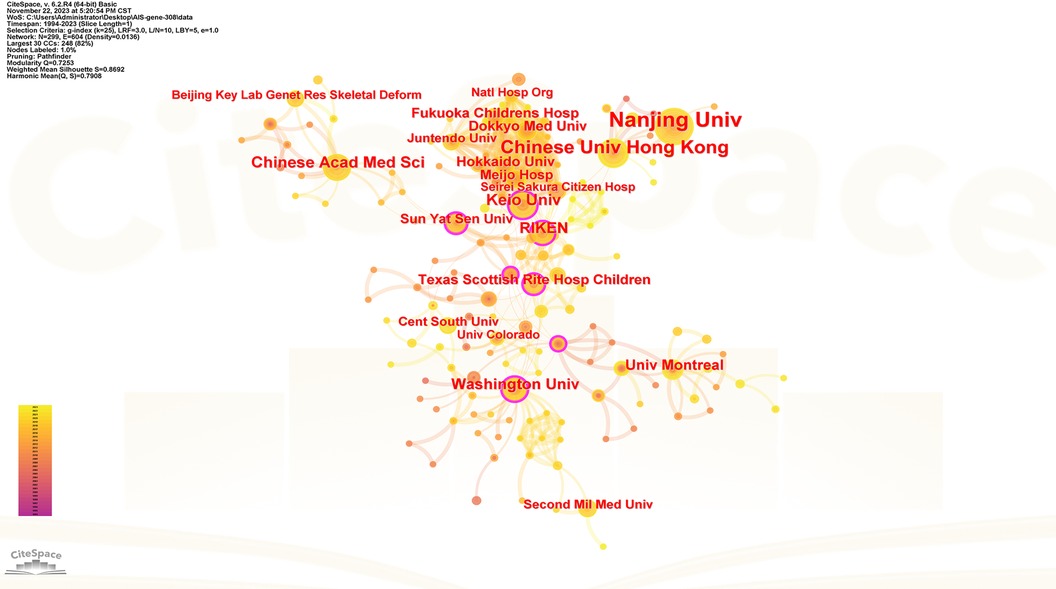
Figure 4. Collaboration network of productive institutions. Nodes represent institutions, lines represent co-citation relationships. Node colors indicate different years. Node size reflects publication numbers.
Journal analysis
Table 3 shows the ranking of journals that published articles on AIS genetic factors. Most papers were published in Spine (62; 20.1%), followed by European Spine Journal (17; 5.5%) and BMC Musculoskeletal Disorders (11; 3.6%), Scientific Reports (11; 3.6%) and Human Molecular Genetics (8; 2.6%). They accounted for 35.4% of all publications. Figure 5 shows the cooperation among these journals.
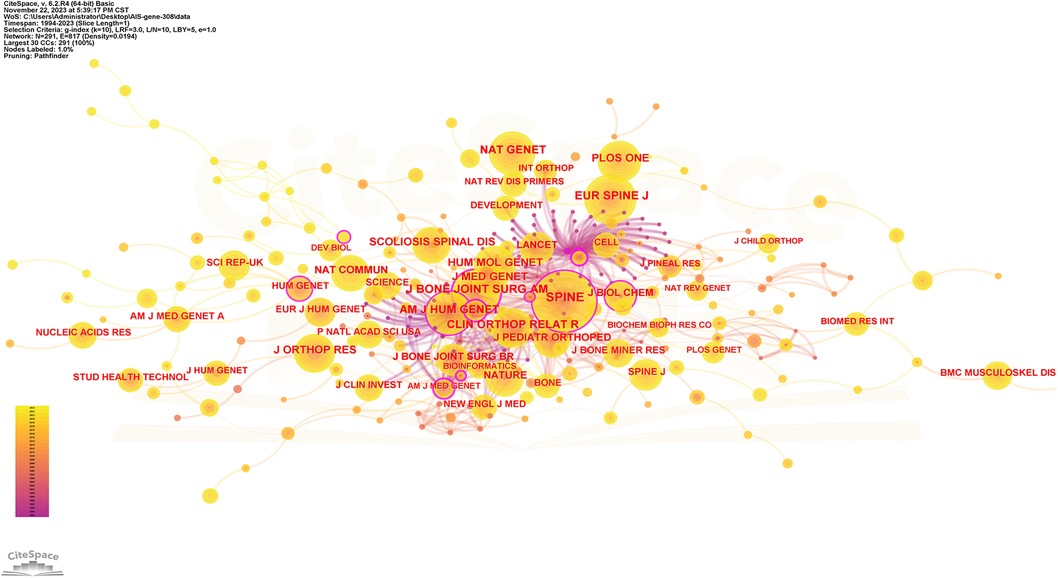
Figure 5. Collaboration network of cited journals. Nodes represent journals, lines represent co-citation relationships. Node colors indicate different years. Node size reflects the number of co-citations.
Author analysis
One thousand five hundred five scholars participated in the publication of 308 articles. Table 4 shows the 10 most prolific scholars. Figure 6 presents the collaboration network of the scholars. Y. Qiu and Z. Z. Zhu contributed to the publication of 59 and 36 papers respectively. The top 3 most cited scholars are Y. Takahashi (113), S. L. Weinstein (111) and N. H. Miller (105). When 2 articles refer to the same article, a common reference link is created. Figure 7 shows the influential authors associated with the genetic factors of AIS. Authors or co-authors of papers working in identical nations appear in the bibliographic link.
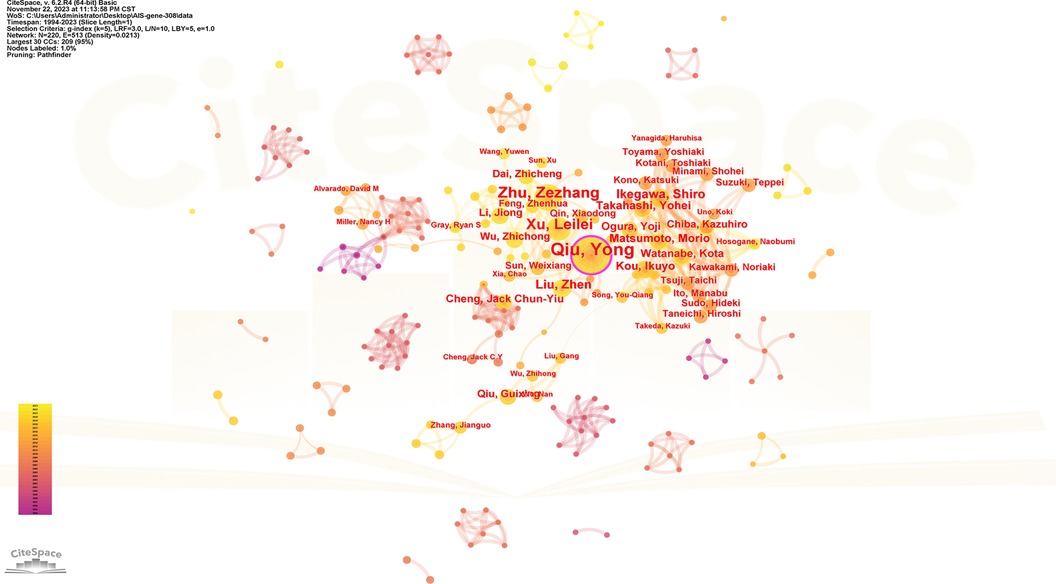
Figure 6. Collaboration network of productive authors. Nodes represent authors, lines represent collaborations. Node colors indicate different years. Node size reflects publication count.
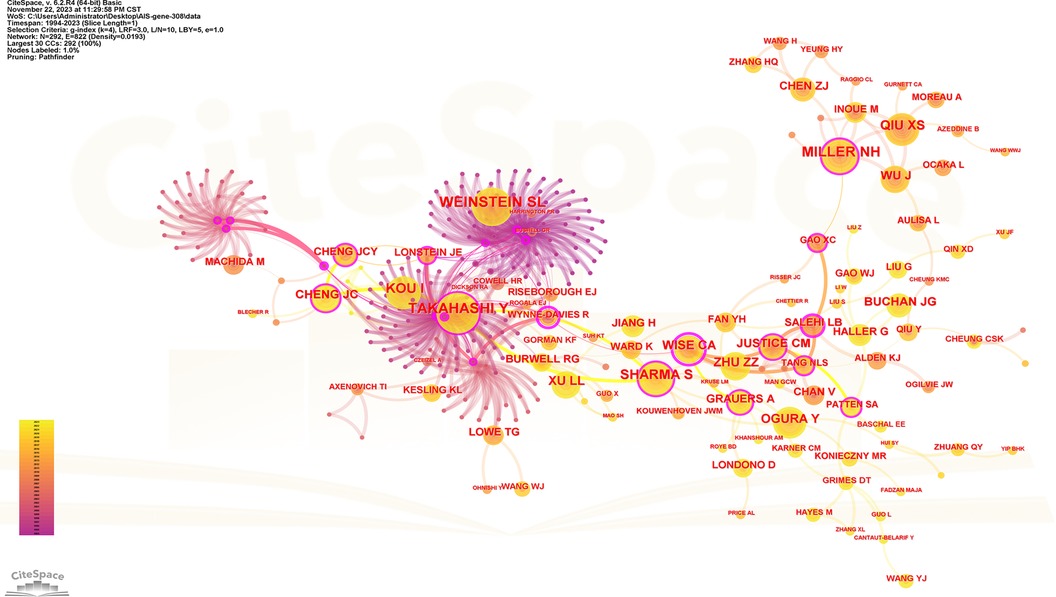
Figure 7. Collaboration network of cited authors. Nodes represent co-cited authors. Lines represent co-citation relationships. Node colors indicate different years. Node size reflects the number of co-citations.
Reference analysis
The spacing of references in co-citation analysis reveals the co-citation relationship. Table 5 enumerates the top 10 cited articles regarding genetic factors in AIS. Among the 10 articles, 4 originate from China, 3 from Japan, 2 from the United States, and 1 from the United Kingdom. Figure 8 displays the network of cited references, depicting the co-citation relationships among them.
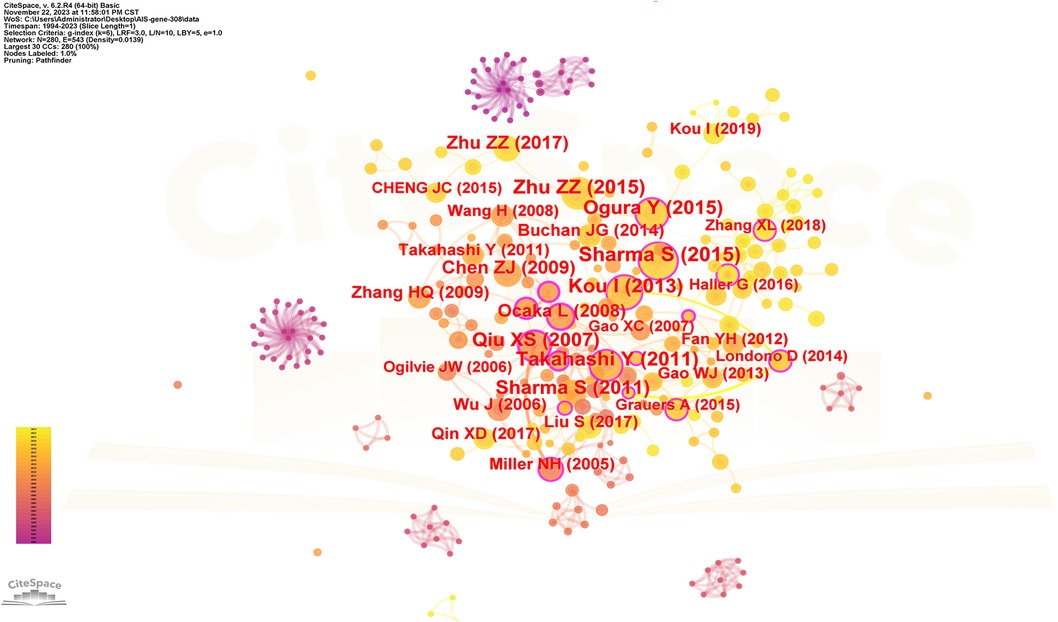
Figure 8. The network of cited references. Nodes represent references, lines represent co-citation relationships. Node colors indicate different years. Node size reflects the number of co-citations.
Keyword analysis and research hotspots
Keywords serve as indicators of the study's subject matter. Summarizing frequently occurring and highly prominent keywords proves useful for describing research focal points and trends. The larger the nodes within the keyword co-occurrence map, the more substantial the keyword weight. Enhanced connections are portrayed by reduced distances between nodes. Bolder lines signify an amplified frequency of 2 phrases being referenced simultaneously. Figure 9 presents the network of keywords. Keyword clustering involves grouping words and phrases that exhibit clear domain characteristics. It utilizes feature extraction algorithms to classify text and perform domain-based clustering of words. By managing word frequency, it identifies both general and specific domain-related terms. Figure 10 shows the 12 clusters in this study: “rare variant” #0, “novel locus” #1, “developmental theory” #2, “estrogen receptor gene polymorphism” #3, “GWAS-associated loci” #4, “BMP4 IL6 leptin MMP3” #5, “culture system” #6, “human melatonin” #7, “physiological aging” #8, “potential role” #9, “genetic susceptibility” #10 and “diagnostic biomarker” #11.
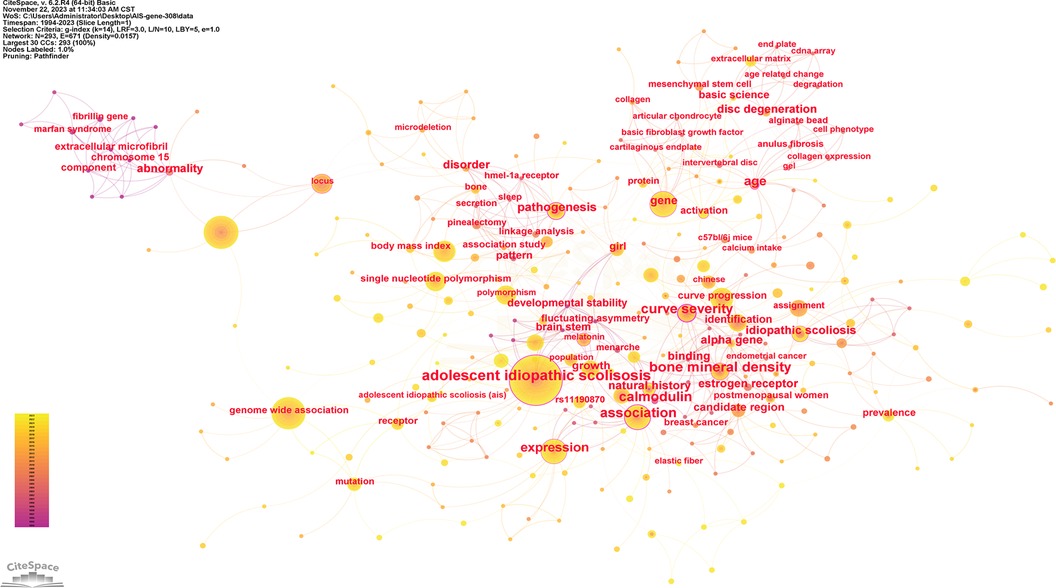
Figure 9. The network of keywords. Nodes represent keywords, lines represent co-occurrence relationships. Colors in the nodes indicate different years. Node size reflects its frequency.
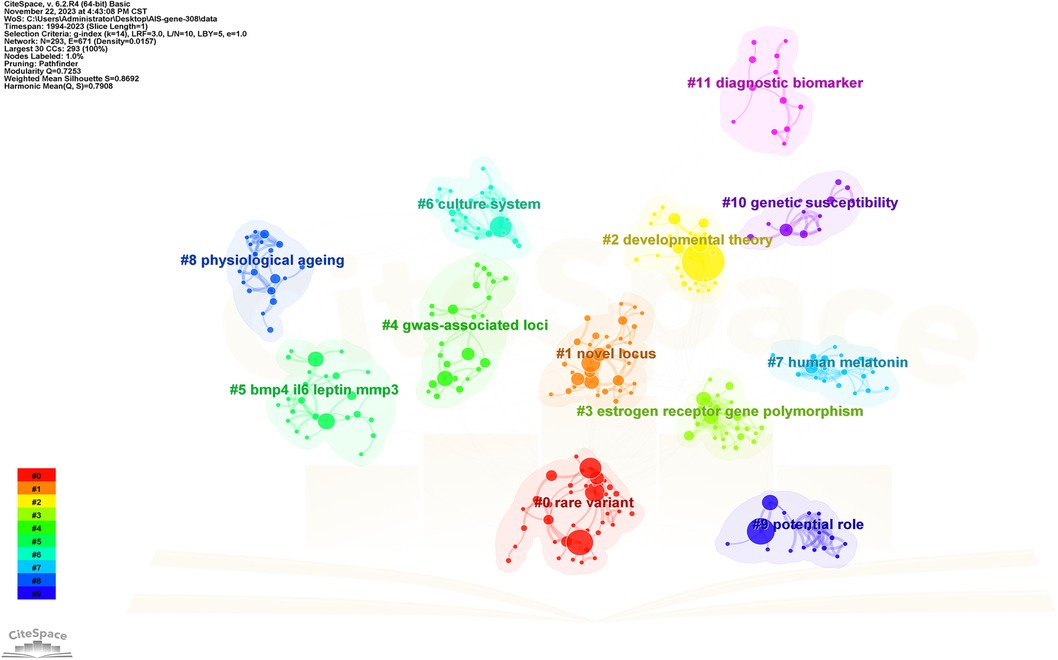
Figure 10. The cluster view map. Each grid contains a cluster of locations. The lower the number, the higher the number of keywords included in the clusters.
Figure 11 presents the top 71 cited keywords. The red and blue bars indicate common and uncommon keywords correspondingly. The most popular keywords in AIS genetic factors were “fibrillin gene”, “menarche”, “calmodulin”, “estrogen receptor gene”, “linkage analysis”, “disc degeneration”, “bone mineral density”, “melatonin signaling dysfunction”, “collagen gene”, “mesenchymal stem cell”, “LBX1”, “promoter polymorphism”, “Bone formation”, “cerebrospinal fluid flow” and “extracellular matrix”.
Discussion
The research showcases the results of a bibliometric analysis of 308 articles on AIS genetic factors published from January, 1, 1992, to February, 28, 2023 utilizing the WoS database and CiteSpace software. The evolution from 1992 to 2023 is described by 2 phases: 1992–2005, a period of slow growth, and 2006–2023, a phase of swift progress. The number of papers published in 2019 is the largest, but this does not mean that the field has reached its peak. Scholars persist in their field with ongoing research, promising more influential publications ahead.
Analysis of the distribution of countries, institutions and scholars shows international collaboration in this field. According to the article count and the H-index, China stood out as the foremost nation in terms of productivity and impact in AIS genetic factor research. Leveraging its sizable population and proficiency in sample collection from AIS patients, China has witnessed accelerated development in studying AIS genetic factors, propelled by economic, technological, and intellectual advancements. The advancement of the economy has stimulated investment in the healthcare sector, leading to a surge in research productivity (6, 9). In addition, young Chinese spine surgeons excel in basic research and article publication. The USA, a powerhouse of economy, science, and technology, assumes a vital role in studying the AIS genetic factors. When analyzing the dispersion of academic papers by nation, organization, and author, Japan was also a noteworthy participant in research efforts.
Qiu Yong and Zhu Zezhang, affiliated with Drum Tower Hospital, Nanjing University, China, have published extensively on this topic. They have maintained a close collaboration. The quantity of co-authors serves as a significant metric for the status of the research. The visual map of this study indicates inadequate connections among countries, institutions, and authors. Enhancing academic collaboration is imperative.
Journal analysis assists researchers in selecting appropriate publications. Spine and European Spine Journal are the mainstream journals on the topic. These journals might exhibit greater openness to researching genetic factors in AIS, and manuscripts featured in these journals have higher chances of gaining attention and citations.
Keywords reflect the focus and trajectory of the research. Keyword evolution analysis provides a crucial basis for exploring the research of AIS genetic factors. After removing keywords related to the topic, the most prominent burst keywords of AIS genetic factors are “fibrillin gene”, “menarche”, “calmodulin”, “estrogen receptor gene”, “linkage analysis”, “disc degeneration”, “bone mineral density”, “melatonin signaling dysfunction”, “collagen gene”, “mesenchymal stem cell”, “LBX1”, “promoter polymorphism”, “Bone formation”, “cerebrospinal fluid flow” and “extracellular matrix”. The results of the burst keywords and clustering revealed that the prominent theme in this field over the last 30 years has been the expression and verification of genes related to the spine and its accessory structures.
Most of the 10 most cited papers investigated the genetic factors of AIS through genome-wide association studies. In 2007, Xu et al. found that the MTNR1B gene is lowly expressed in osteoblasts from AIS patients but is not involved in disease progression (10). Subsequently, AIS-related susceptibility genes continue to emerge, including MATN1 (11), LBX1 (12), PAX1 (13), BNC2 (14) and GPR126 (15, 16). Except for the BNC2 gene, all genes were lowly expressed in the tissue cells of AIS patients. AIS-related signaling pathways have also shown remarkable results. Sharma et al. found a possible association between the clinical phenotype of horizontal gaze palsy with progressive scoliosis in patients with compound heterozygous mutations in ROBO3 and the involvement of the ROBO3 gene in the regulation of the axon guidance pathway (17). Zhu et al. found that the proportion of type I fibers was higher on the convex side than on the concave side in patients with AIS, which may be related to the asymmetric expression of the Wnt/β-catenin pathway in the paraspinal muscles on both sides of the spine (18). These studies suggest that AIS may be the result of the combined effects of multiple gene expression and different signaling pathways.
Combining keyword burst analysis and keyword clustering analysis, we summarized the new perspectives of AIS genetic factors research papers published in the last 5 years as: urotensin and its related receptors, cilia, chondrocytes and osteogenesis, muscle tissue, neural crest cells, and connective tissue.
Urotensin and its related receptors
Zebrafish, genetically similar to humans, are ideal subjects for studying scoliosis due to their inherent susceptibility to the condition (19, 20). The circulation of cerebrospinal fluid (CSF) is associated with spinal development and can result in zebrafish developing spinal deformities (21–23). When CSF flows through neurons in contact with CSF(CSF-cNs) in the central canal, these neurons produce the urotensin-related peptides Urp1 and Urp2 (24–26). These peptides can bind to Uts2r receptors on the membrane of dorsal slow-twitch myocytes in zebrafish embryos, causing the myocytes to contract dorsally and straighten the body axis. Reducing these peptides during zebrafish growth causes spinal deformities similar to human spinal dysplasia (27, 28). Bearce et al. found that zebrafish lacking Uts2r3 have scoliosis in adulthood; and that Uts2r3, as a receptor of the urotensin family, may regulate spinal morphology (21). Research on Chinese Han patients with AIS has additionally verified a substantial correlation between UTS2R gene mutation and AIS (29). It can be concluded that mutations in the genes that control the production of urotensin and its receptor may lead to the development of AIS.
Cilia
Cilia are hairy organelles positioned outside the cell membrane (30). Active cilia produce cellular motility, drive fluid flow or generate signal gradients (22, 31). Cilia assembly and CSF flow are closely associated with AIS (22). Elizabeth et al. suggest that motile cilia in the zebrafish vertebral canal promote the synthesis of Reissner's fibers, which transport epinephrine to the central canal. Epinephrine acts on CSF-cNs to induce Urp peptides secretion. These urotensins signal the zebrafish dorsal slow-twitch muscles to elicit contractions that resolve the ventral curve and promote spinal straightening (28). The mutation rate of the POC5 gene in French-Canadian and British AIS patients was significantly higher than in normal controls (32). Its aberrant expression affects Urp peptides secretion, which limits the contraction of slow-twitch muscles and imbalances muscle strength on both sides of the spine, causing scoliosis (33). Similarly, variants in the TTLL11 gene were present in the majority of UK families with concomitant scoliosis; a zebrafish model with the gene knocked out replicated this condition (34). Abnormal ciliary movement may also lead to scoliosis. Zebrafish cilia contribute to Reissner fiber aggregation and straightening of the body axis; and knockout of the ciliary polarity and movement-related gene cfap298 (35) and ciliary dynamic protein axonal heavy chain 10 genes may affect Reissner fiber aggregation and lead to zebrafish spinal deformity (21, 36). Variants in genes dnaaf1 and zmynd10 related to cilia structure and function were also found in AIS patients in southern China by whole exome sequencing (37). Knockout of these genes in viable adult zebrafish recapitulated scoliosis (37). It can be concluded that mutations in cilia-related genes cause AIS by impeding CSF flow, Reissner fiber synthesis, and urotensin release allowing an imbalance of paraspinal musculature.
Chondrocytes and osteogenic process
Meta-analyses have summarized and confirmed that AIS-related gene mutations affect chondrocyte development and osteogenesis (such as CDH13, ABO and COMP) (38, 39). Among them, COMP gene expression is down-regulated in osteoblasts of AIS patients, which synthesize abnormal proteins with cytotoxicity, triggering chondrocyte underdevelopment or even death, resulting in impaired bone growth (39). A number of studies on signaling pathways involved in the process. The ERK1/2 signaling pathway activates the AKAP2 gene, promoting the proliferation and specialization of chondrocytes in the human growth plate, while AKAP2 gene expression was reduced in AIS patients (40). Upregulation of the RHOA gene in AIS patients inhibits the differentiation of MSCs to cartilage through the RHOA/ROCK signaling pathway and hinders bone growth (41). SPRY4 gene is the key to promoting osteogenic differentiation and melatonin response in mesenchymal stem cells. Its overexpression, along with melatonin, enhances osteogenesis, whereas knockout of SPRY4 hampers osteogenesis in AIS patients (42, 43). Low expression of ADGRG7, GREM1 and GPR126 genes affects osteogenesis in AIS patients (44–47). CHD7 and BOC gene expression even positively correlated with bone mineral content in AIS patients (48, 49).
Muscle tissue
AIS is often accompanied by differential expression of genes in paraspinal muscle cells. The abnormal expression of the LBX1 gene has been replicated in a large sample of multi-ethnic AIS patients (50, 51) and successfully verified in mouse experiments (52). Low expression of the LBX1 gene may reduce energy supply to skeletal muscle cells and accelerate disease progression by affecting galactose metabolism and glycolytic pathways (53). Genes also regulate the development of paraspinal muscles. High expression of the TENT5A gene facilitates myofiber maturation by promoting the proliferative migration of myofibroblasts and maintaining the stability of myogenin; whereas, the TENT5A gene is lowly expressed in the paraspinal muscles of patients with AIS (54). In addition to the genes mentioned above, numerous other genes are lowly expressed in the paraspinal muscles of AIS patients, and their functions have yet to be investigated, including PIEZO2 (55), CDH13 (56), ABO (57), SLC39A8 (58), ROBO3 (59), IRX1 (60), H19 (61) and SOCS3 (62).
Neural crest cell
AIS patients displayed chondroblasts in the curved convex growth plate, while the concave side had neuroblasts and glial blasts (63). Bilateral growth imbalance results in scoliosis. Neural crest cells migrate along a specific pathway during embryonic development to form neuroblasts and glial blasts (63, 64). During migration, neural crest cells experience epithelial-mesenchymal transition (65), facilitating their migration into the mesenchymal extracellular matrix (66). PAX3 gene is linked to the formation of mesenchymal extracellular matrix, including the expression of two multifunctional proteoglycan subtypes (V1 and V0) (67). Multifunctional proteoglycan can guide the migration of neural crest cells (68, 69). Cartilage differentiation of mesenchymal cells may influence the migration of neural crest cells (70). Therefore, the PAX3 gene may cause non-synchronous migration of neural crest cells and different phenotypes of cells on both sides of the spine, resulting in idiopathic scoliosis.
Connective tissue
Connective tissue plays a role in maintaining normal spine morphology, including intervertebral discs, ligaments, and tendons (71, 72). The ADGRG6 gene regulates the biomechanical structure of intervertebral discs and dense connective tissues to maintain normal spinal morphology through the cAMP/CREB signaling pathway; whereas AIS patients have defective expression of the ADGRG6 gene (73). The expression of the PAX-1 gene in the disc is involved in the formation of the spinal structure (74). In addition, upregulation of ERC2 and MAFB gene expression in AIS patients may promote hypertrophy of the ligamentum flavum to adapt to mechanical stresses generated by scoliosis via the TGF-β pathway (75). The FBN1 gene is crucial for connective tissue function (76), and its low expression reduces the synthesis of extracellular matrix proteins, which is detrimental to maintaining the stability of the biomechanical structure of connective tissues such as ligaments and intervertebral discs, leading to the progression of AIS (77). ADAMTSL2 and LTBPs can bind to FBN1 in vitro and upregulate the TGF-β signaling pathway in fibroblasts (78–81). Ryzhkov et al. found that the TGF-β signaling pathway, which is involved in the formation and degradation of extracellular matrix proteins, is more highly expressed on the concave side of the curve than on the convex side in patients with AIS, and may be involved in the process of disc tissue degeneration (82). Therefore, The interaction between ADAMTSL2 and LTBP4 is involved in the development of AIS through the TGF-β pathway (83). The above results show that connective tissue-related genes are involved in the onset and development of AIS.
Although research on genetic factors associated with AIS is recent and extensive, most studies are not in-depth and have some limitations.
1. Most studies have small sample sizes and selection bias, and the samples can not represent the patient group (33, 84).
2. Many studies only examine whether there is a correlation, but do not explain the causality and mechanism of action (14, 16).
3. Some studies are unable to obtain muscle, bone, and other tissues that may be directly related to AIS (85, 86).
4. Integrating the existing research results and establishing the mathematical model of AIS is beneficial for the diagnosis and prevention of the disease (87).
In the process of studying the etiology of AIS, researchers have gradually recognized that AIS is a disease caused by the combined effects of multiple factors. Over the past few decades, the study of AIS etiology has shifted from a macroscopic to a microscopic level, particularly with the rise of GWAS, which allows for comprehensive screening of genes associated with the occurrence and development of AIS. As a result, various tissues related to AIS have been included in the research. While these studies have provided abundant results, they have been scattered, making it difficult to identify the primary effect genes. Additionally, there is a lack of AIS samples from diverse ethnic backgrounds, preventing integration into a unified system.
Artificial intelligence (AI) is well known for its ability to analyze and process large amounts of data, and discover hidden relationships between variables. By consolidating clinical and GWAS data from previous AIS patients and constructing a database that is continuously updated, we can combine the vast clinical information and GWAS data of AIS patients with AI. This integration allows for the training of a dynamic AIS simulation system, which can uncover associations between potential genetic variations and specific clinical phenotypes, predict AIS risk and progression, simulate gene-targeted therapies for AIS, and even customize personalized disease risk assessment models based on individual AIS patients' genomic information and relevant clinical parameters. This approach provides targeted medical advice and treatment plans, contributing to the development of precision medicine and improving treatment outcomes and health management for each patient.
Advantages and constraints of this research
This is an inaugural bibliometric analysis of the genetic factors of AIS. This study discusses the current situation, progress and trend of the role of genetic factors in AIS, so that scholars can focus on the latest and most important hotspots. This study has several limitations. First, there is a temporal delay as this study did not incorporate recent publications. Second, it is not comprehensive. We only looked at articles from the WoS core collection. Finally, subjective bias exists in the interpretation of the results.
Conclusion
Research into the genetic factors of AIS indicates a worldwide pattern over time. Beginning in 2006, there has been a significant surge in the quantity of published research. China and the Chinese Academy of Medical Sciences emerge as the most productive countries and institutions in this regard. A large number of articles have been published in Spine and European Spine Journal. Y. Qiu and Z. Z. Zhu are both prolific authors. The strongest burst keywords among the genetic factors of AIS were “fibrillin gene”, “menarche”, “calmodulin”, “estrogen receptor gene”, “linkage analysis”, “disc degeneration”, “bone mineral density”, “melatonin signaling dysfunction”, “collagen gene”, “mesenchymal stem cell”, “LBX1”, “promoter polymorphism”, “Bone formation”, “cerebrospinal fluid flow” and “extracellular matrix”. In addition, scholars would find valuable insights from the prominent clusters, highly cited publications and references.
Author contributions
XJ: Conceptualization, Data curation, Formal Analysis, Methodology, Writing – original draft, Writing – review & editing. FL: Conceptualization, Writing – review & editing. MZ: Data curation, Formal Analysis, Visualization, Writing – original draft, Writing – review & editing. WH: Formal Analysis, Supervision, Writing – review & editing. YZ: Formal Analysis, Writing – review & editing. BX: Writing – review & editing. KX: Writing – review & editing.
Funding
The author(s) declare financial support was received for the research, authorship, and/or publication of this article.
This research was funded by the “Joint Project of Henan Medical Science and Technology Project (No. LHGJ20190384)”.
Conflict of interest
The authors declare that the research was conducted in the absence of any commercial or financial relationships that could be construed as a potential conflict of interest.
Publisher's note
All claims expressed in this article are solely those of the authors and do not necessarily represent those of their affiliated organizations, or those of the publisher, the editors and the reviewers. Any product that may be evaluated in this article, or claim that may be made by its manufacturer, is not guaranteed or endorsed by the publisher.
References
1. Weinstein SL, Dolan LA, Cheng JC, Danielsson A, Morcuende JA. Adolescent idiopathic scoliosis. Lancet. (2008) 371:1527–37. doi: 10.1016/s0140-6736(08)60658-3
2. Cheng JC, Castelein RM, Chu WC, Danielsson AJ, Dobbs MB, Grivas TB, et al. Adolescent idiopathic scoliosis. Nat Rev Dis Primers. (2015) 1:15030. doi: 10.1038/nrdp.2015.30
3. Negrini S, Donzelli S, Aulisa AG, Czaprowski D, Schreiber S, de Mauroy JC, et al. 2016 SOSORT guidelines: orthopaedic and rehabilitation treatment of idiopathic scoliosis during growth. Scoliosis Spinal Disord. (2018) 13:3. doi: 10.1186/s13013-017-0145-8
4. Lonstein JE. Scoliosis: surgical versus nonsurgical treatment. Clin Orthop Relat Res. (2006) 443:248–59. doi: 10.1097/01.blo.0000198725.54891.73
5. Peng Y, Wang SR, Qiu GX, Zhang JG, Zhuang QY. Research progress on the etiology and pathogenesis of adolescent idiopathic scoliosis. Chin Med J (Engl). (2020) 133:483–93. doi: 10.1097/cm9.0000000000000652
6. Lin GX, Kotheeranurak V, Mahatthanatrakul A, Ruetten S, Yeung A, Lee SH, et al. Worldwide research productivity in the field of full-endoscopic spine surgery: a bibliometric study. Eur Spine J. (2020) 29:153–60. doi: 10.1007/s00586-019-06171-2
7. Lin GX, Nan JN, Chen KT, Sun LW, Tai CT, Jhang SW, et al. Bibliometric analysis and visualization of research trends on oblique lumbar interbody fusion surgery. Int Orthop. (2022) 46:1597–608. doi: 10.1007/s00264-022-05316-1
8. Ali MJ. Forewarned is forearmed: the h-Index as a scientometric. Semin Ophthalmol. (2021) 36:1. doi: 10.1080/08820538.2021.1894889
9. Wang C, Liu Q. A turning point for clinical research in China? Lancet. (2013) 382:835–6. doi: 10.1016/s0140-6736(13)61804-8
10. Qiu XS, Tang NL, Yeung HY, Lee KM, Hung VW, Ng BK, et al. Melatonin receptor 1B (MTNR1B) gene polymorphism is associated with the occurrence of adolescent idiopathic scoliosis. Spine (Phila Pa 1976). (2007) 32:1748–53. doi: 10.1097/BRS.0b013e3180b9f0ff
11. Chen Z, Tang NL, Cao X, Qiao D, Yi L, Cheng JC, et al. Promoter polymorphism of matrilin-1 gene predisposes to adolescent idiopathic scoliosis in a Chinese population. Eur J Hum Genet. (2009) 17:525–32. doi: 10.1038/ejhg.2008.203
12. Takahashi Y, Kou I, Takahashi A, Johnson TA, Kono K, Kawakami N, et al. A genome-wide association study identifies common variants near LBX1 associated with adolescent idiopathic scoliosis. Nat Genet. (2011) 43:1237–40. doi: 10.1038/ng.974
13. Sharma S, Londono D, Eckalbar WL, Gao X, Zhang D, Mauldin K, et al. A PAX1 enhancer locus is associated with susceptibility to idiopathic scoliosis in females. Nat Commun. (2015) 6:6452. doi: 10.1038/ncomms7452
14. Ogura Y, Kou I, Miura S, Takahashi A, Xu L, Takeda K, et al. A functional SNP in BNC2 is associated with adolescent idiopathic scoliosis. Am J Hum Genet. (2015) 97:337–42. doi: 10.1016/j.ajhg.2015.06.012
15. Zhu Z, Tang NL, Xu L, Qin X, Mao S, Song Y, et al. Genome-wide association study identifies new susceptibility loci for adolescent idiopathic scoliosis in Chinese girls. Nat Commun. (2015) 6:8355. doi: 10.1038/ncomms9355
16. Kou I, Takahashi Y, Johnson TA, Takahashi A, Guo L, Dai J, et al. Genetic variants in GPR126 are associated with adolescent idiopathic scoliosis. Nat Genet. (2013) 45:676–9. doi: 10.1038/ng.2639
17. Sharma S, Gao X, Londono D, Devroy SE, Mauldin KN, Frankel JT, et al. Genome-wide association studies of adolescent idiopathic scoliosis suggest candidate susceptibility genes. Hum Mol Genet. (2011) 20:1456–66. doi: 10.1093/hmg/ddq571
18. Zhu Z, Xu L, Leung-Sang Tang N, Qin X, Feng Z, Sun W, et al. Genome-wide association study identifies novel susceptible loci and highlights wnt/beta-catenin pathway in the development of adolescent idiopathic scoliosis. Hum Mol Genet. (2017) 26:1577–83. doi: 10.1093/hmg/ddx045
19. Bagnat M, Gray RS. Development of a straight vertebrate body axis. Development. (2020) 147:dev175794–806. doi: 10.1242/dev.175794
20. Xie H, Li M, Kang Y, Zhang J, Zhao C. Zebrafish: an important model for understanding scoliosis. Cell Mol Life Sci. (2022) 79:506. doi: 10.1007/s00018-022-04534-5
21. Bearce EA, Irons ZH, O’Hara-Smith JR, Kuhns CJ, Fisher SI, Crow WE, et al. Urotensin II-related peptides, Urp1 and Urp2, control zebrafish spine morphology. Elife. (2022) 11:e83883–909. doi: 10.7554/eLife.83883
22. Muñoz-Montecinos C, Romero A, Sepúlveda V, Vira M, Fehrmann-Cartes K, Marcellini S, et al. Turning the curve into straight: phenogenetics of the spine morphology and coordinate maintenance in the zebrafish. Front Cell Dev Biol. (2021) 9:801652. doi: 10.3389/fcell.2021.801652
23. Grimes DT, Boswell CW, Morante NF, Henkelman RM, Burdine RD, Ciruna B. Zebrafish models of idiopathic scoliosis link cerebrospinal fluid flow defects to spine curvature. Science. (2016) 352:1341–4. doi: 10.1126/science.aaf6419
24. Cantaut-Belarif Y, Orts Del’Immagine A, Penru M, Pézeron G, Wyart C, Bardet PL. Adrenergic activation modulates the signal from the reissner fiber to cerebrospinal fluid-contacting neurons during development. Elife. (2020) 9:e59469–93. doi: 10.7554/eLife.59469
25. Lu H, Shagirova A, Goggi JL, Yeo HL, Roy S. Reissner fibre-induced urotensin signalling from cerebrospinal fluid-contacting neurons prevents scoliosis of the vertebrate spine. Biol Open. (2020) 9:bio052027–33. doi: 10.1242/bio.052027
26. Zhang X, Jia S, Chen Z, Chong YL, Xie H, Feng D, et al. Cilia-driven cerebrospinal fluid flow directs expression of urotensin neuropeptides to straighten the vertebrate body axis. Nat Genet. (2018) 50:1666–73. doi: 10.1038/s41588-018-0260-3
27. Grimes DT. Developmental biology: go with the flow to keep the body straight. Curr Biol. (2019) 29:R101–r103. doi: 10.1016/j.cub.2018.12.011
28. Bearce EA, Grimes DT. On being the right shape: roles for motile cilia and cerebrospinal fluid flow in body and spine morphology. Semin Cell Dev Biol. (2021) 110:104–12. doi: 10.1016/j.semcdb.2020.07.005
29. Dai Z, Wang Y, Wu Z, Feng Z, Liu Z, Qiu Y, et al. Novel mutations in UTS2R are associated with adolescent idiopathic scoliosis in the Chinese population. Spine (Phila Pa 1976). (2021) 46:E288–e293. doi: 10.1097/brs.0000000000003786
30. Malicki JJ, Johnson CA. The Cilium: cellular antenna and central processing unit. Trends Cell Biol. (2017) 27:126–40. doi: 10.1016/j.tcb.2016.08.002
31. Wang L, Dynlacht BD. The regulation of cilium assembly and disassembly in development and disease. Development. (2018) 145:dev151407–19. doi: 10.1242/dev.151407
32. Mathieu H, Spataru A, Aragon-Martin JA, Child A, Barchi S, Fortin C, et al. Prevalence of POC5 coding variants in French-Canadian and British AIS cohort. Genes (Basel). (2021) 12:1032–39. doi: 10.3390/genes12071032
33. Hassan A, Parent S, Mathieu H, Zaouter C, Molidperee S, Bagu ET, et al. Adolescent idiopathic scoliosis associated POC5 mutation impairs cell cycle, cilia length and centrosome protein interactions. PLoS One. (2019) 14:e0213269. doi: 10.1371/journal.pone.0213269
34. Mathieu H, Patten SA, Aragon-Martin JA, Ocaka L, Simpson M, Child A, et al. Genetic variant of TTLL11 gene and subsequent ciliary defects are associated with idiopathic scoliosis in a 5-generation UK family. Sci Rep. (2021) 11:11026. doi: 10.1038/s41598-021-90155-0
35. Marie-Hardy L, Cantaut-Belarif Y, Pietton R, Slimani L, Pascal-Moussellard H. The orthopedic characterization of cfap298(tm304) mutants validate zebrafish to faithfully model human AIS. Sci Rep. (2021) 11:7392. doi: 10.1038/s41598-021-86856-1
36. Cantaut-Belarif Y, Sternberg JR, Thouvenin O, Wyart C, Bardet PL (2018) The reissner fiber in the cerebrospinal fluid controls morphogenesis of the body axis. Curr Biol 28:2479–86.e2474. doi: 10.1016/j.cub.2018.05.079
37. Wang Y, Liu Z, Yang G, Gao Q, Xiao L, Li J, et al. Coding variants coupled with rapid modeling in zebrafish implicate dynein genes, dnaaf1 and zmynd10, as adolescent idiopathic scoliosis candidate genes. Front Cell Dev Biol. (2020) 8:582255. doi: 10.3389/fcell.2020.582255
38. Khanshour AM, Kou I, Fan Y, Einarsdottir E, Makki N, Kidane YH, et al. Genome-wide meta-analysis and replication studies in multiple ethnicities identify novel adolescent idiopathic scoliosis susceptibility loci. Hum Mol Genet. (2018) 27:3986–98. doi: 10.1093/hmg/ddy306
39. Mao SH, Qian BP, Shi B, Zhu ZZ, Qiu Y. Quantitative evaluation of the relationship between COMP promoter methylation and the susceptibility and curve progression of adolescent idiopathic scoliosis. Eur Spine J. (2018) 27:272–7. doi: 10.1007/s00586-017-5309-y
40. Wang B, Jiang B, Li Y, Dai Y, Li P, Li L, et al. AKAP2 Overexpression modulates growth plate chondrocyte functions through ERK1/2 signaling. Bone. (2021) 146:115875. doi: 10.1016/j.bone.2021.115875
41. Yang M, Chen K, Hou C, Yang Y, Zhai X, Chen K, et al. RHOA Inhibits chondrogenic differentiation of mesenchymal stem cells in adolescent idiopathic scoliosis. Connect Tissue Res. (2022) 63:475–84. doi: 10.1080/03008207.2021.2019247
42. Li J, Li N, Chen Y, Hui S, Fan J, Ye B, et al. SPRY4 Is responsible for pathogenesis of adolescent idiopathic scoliosis by contributing to osteogenic differentiation and melatonin response of bone marrow-derived mesenchymal stem cells. Cell Death Dis. (2019) 10:805. doi: 10.1038/s41419-019-1949-7
43. Tao L, Zhu Y. Melatonin regulates CRE-dependent gene transcription underlying osteoblast proliferation by activating src and PKA in parallel. Am J Transl Res. (2018) 10:86–100.29422996
44. Hassan A, Bagu ET, Levesque M, Patten SA, Benhadjeba S, Edjekouane L, et al. The 17β-estradiol induced upregulation of the adhesion G-protein coupled receptor (ADGRG7) is modulated by ESRα and SP1 complex. Biol Open. (2019) 8:bio037390–402. doi: 10.1242/bio.037390
45. Xu E, Shao W, Jiang H, Lin T, Gao R, Zhou X. A genetic variant in GPR126 causing a decreased inclusion of exon 6 is associated with cartilage development in adolescent idiopathic scoliosis population. Biomed Res Int. (2019) 2019:4678969. doi: 10.1155/2019/4678969
46. Xu E, Lin T, Jiang H, Ji Z, Shao W, Meng Y, et al. Asymmetric expression of GPR126 in the convex/concave side of the spine is associated with spinal skeletal malformation in adolescent idiopathic scoliosis population. Eur Spine J. (2019) 28:1977–86. doi: 10.1007/s00586-019-06001-5
47. Wang Y, Zhang H, Yang G, Xiao L, Li J, Guo C. Dysregulated bone metabolism is related to high expression of miR-151a-3p in severe adolescent idiopathic scoliosis. Biomed Res Int. (2020) 2020:4243015. doi: 10.1155/2020/4243015
48. Wu Z, Dai Z, Yuwen W, Liu Z, Qiu Y, Cheng JC, et al. Genetic variants of CHD7 are associated with adolescent idiopathic scoliosis. Spine (Phila Pa 1976). (2021) 46:E618–e624. doi: 10.1097/brs.0000000000003857
49. Dai Z, Wang Y, Wu Z, Feng Z, Sun X, Qiu Y, et al. Female-Specific susceptibility locus in BOC and SEC16B are associated with adolescent idiopathic scoliosis. Spine (Phila Pa 1976). (2021) 46:E1178–e1184. doi: 10.1097/brs.0000000000004098
50. Matsuhashi Y, Horiuchi K, Nakagawa T, Takahashi Y, Imabayashi H, Hosogane N, et al. Abrogation of LBX1 in skeletal muscle results in hypoplastic limbs and progressive kyphosis in mice. J Orthop Res. (2023) 41:884–90. doi: 10.1002/jor.25417
51. Xu L, Feng Z, Dai Z, Lee WYW, Wu Z, Liu Z, et al. A functional SNP in the promoter of LBX1 is associated with the development of adolescent idiopathic scoliosis through involvement in the myogenesis of paraspinal muscles. Front Cell Dev Biol. (2021) 9:777890. doi: 10.3389/fcell.2021.777890
52. Luo M, Zhang Y, Huang S, Song Y. The susceptibility and potential functions of the LBX1 gene in adolescent idiopathic scoliosis. Front Genet. (2020) 11:614984. doi: 10.3389/fgene.2020.614984
53. Wang Y, Li M, Chan CO, Yang G, Lam JC, Law BC, et al. Biological effect of dysregulated LBX1 on adolescent idiopathic scoliosis through modulating muscle carbohydrate metabolism. Spine J. (2022) 22:1551–65. doi: 10.1016/j.spinee.2022.04.005
54. Luo M, Yang H, Wu D, You X, Huang S, Song Y. Tent5a modulates muscle fiber formation in adolescent idiopathic scoliosis via maintenance of myogenin expression. Cell Prolif. (2022) 55:e13183. doi: 10.1111/cpr.13183
55. Wu Z, Wang Y, Xia C, Feng Z, Qiu Y, Cheng JC, et al. PIEZO2: a novel molecule involved in the development of AIS. Spine (Phila Pa 1976). (2020) 45:E120–e125. doi: 10.1097/brs.0000000000003224
56. Xu L, Wang Y, Wu Z, Dai Z, Liu Z, Qiu Y, et al. A novel coding variant in SLC39A8 is associated with adolescent idiopathic scoliosis in Chinese han population. Spine (Phila Pa 1976). (2020) 45:226–33. doi: 10.1097/brs.0000000000003244
57. Wu Z, Wang Y, Dai Z, Qiu Y, Xu L, Zhu Z. Genetic variants of ABO and SOX6 are associated with adolescent idiopathic scoliosis in Chinese han population. Spine (Phila Pa 1976). (2019) 44:E1063–e1067. doi: 10.1097/brs.0000000000003062
58. Zhang Z, Zhang Z, Shu L, Meng Y, Ma J, Gao R, et al. A genetic variant of the ROBO3 gene is associated with adolescent idiopathic scoliosis in the Chinese population. Spine (Phila Pa 1976). (2023) 48:E20–e24. doi: 10.1097/brs.0000000000004484
59. Xia C, Xue B, Wang Y, Qin X, Qiu Y, Zhu Z, et al. Investigating role of IRX family in development of female adolescent idiopathic scoliosis: which one is real cause? World Neurosurg. (2019) 127:e132–6. doi: 10.1016/j.wneu.2019.02.184
60. Jiang H, Yang F, Lin T, Shao W, Meng Y, Ma J, et al. Asymmetric expression of H19 and ADIPOQ in concave/convex paravertebral muscles is associated with severe adolescent idiopathic scoliosis. Mol Med. (2018) 24:48. doi: 10.1186/s10020-018-0049-y
61. Li Y, Wu Z, Xu L, Feng Z, Wang Y, Dai Z, et al. Genetic variant of TBX1 gene is functionally associated with adolescent idiopathic scoliosis in the Chinese population. Spine (Phila Pa 1976). (2021) 46:17–21. doi: 10.1097/brs.0000000000003700
62. Qiao J, Xiao L, Xu L, Qian B, Zhu Z, Qiu Y. Genetic variant of SOCS3 gene is functionally associated with lumbar adolescent idiopathic scoliosis. Clin Spine Surg. (2018) 31:E193–e196. doi: 10.1097/bsd.0000000000000628
63. Zaydman AM, Strokova EL, Kiseleva EV, Suldina LA, Strunov AA, Shevchenko AI, et al. A new look at etiological factors of idiopathic scoliosis: neural crest cells. Int J Med Sci. (2018) 15:436–46. doi: 10.7150/ijms.22894
64. Scaal M. Early development of the vertebral column. Semin Cell Dev Biol. (2016) 49:83–91. doi: 10.1016/j.semcdb.2015.11.003
65. Bronner-Fraser M, García-Castro M. Manipulations of neural crest cells or their migratory pathways. Methods Cell Biol. (2008) 87:75–96. doi: 10.1016/s0091-679x(08)00204-5
66. Perris R, Perissinotto D. Role of the extracellular matrix during neural crest cell migration. Mech Dev. (2000) 95:3–21. doi: 10.1016/s0925-4773(00)00365-8
67. Henderson DJ, Ybot-Gonzalez P, Copp AJ. Over-expression of the chondroitin sulphate proteoglycan versican is associated with defective neural crest migration in the Pax3 mutant mouse (splotch). Mech Dev. (1997) 69:39–51. doi: 10.1016/s0925-4773(97)00151-2
68. McGonnell IM, Graham A. Trunk neural crest has skeletogenic potential. Curr Biol. (2002) 12:767–71. doi: 10.1016/s0960-9822(02)00818-7
69. Pettway Z, Domowicz M, Schwartz NB, Bronner-Fraser M. Age-dependent inhibition of neural crest migration by the notochord correlates with alterations in the S103l chondroitin sulfate proteoglycan. Exp Cell Res. (1996) 225:195–206. doi: 10.1006/excr.1996.0170
70. Bronner ME, Simões-Costa M. The neural crest migrating into the twenty-first century. Curr Top Dev Biol. (2016) 116:115–34. doi: 10.1016/bs.ctdb.2015.12.003
71. Tsipouras P, Del Mastro R, Sarfarazi M, Lee B, Vitale E, Child AH, et al. Genetic linkage of the marfan syndrome, ectopia lentis, and congenital contractural arachnodactyly to the fibrillin genes on chromosomes 15 and 5. The international marfan syndrome collaborative study. N Engl J Med. (1992) 326:905–9. doi: 10.1056/nejm199204023261401
72. Miller NH, Mims B, Child A, Milewicz DM, Sponseller P, Blanton SH. Genetic analysis of structural elastic fiber and collagen genes in familial adolescent idiopathic scoliosis. J Orthop Res. (1996) 14:994–9. doi: 10.1002/jor.1100140621
73. Liu Z, Hussien AA, Wang Y, Heckmann T, Gonzalez R, Karner CM, et al. An adhesion G protein-coupled receptor is required in cartilaginous and dense connective tissues to maintain spine alignment. Elife. (2021) 10:e67781–807. doi: 10.7554/eLife.67781
74. Pedrosa AE, de Azevedo GBL, Cardoso JV, Guimarães JAM, Defino HLA, Perini JA. Polymorphisms in paired box 1 gene were associated with susceptibility of adolescent idiopathic scoliosis: a case-control study. J Craniovertebr Junction Spine. (2022) 13:318–24. doi: 10.4103/jcvjs.jcvjs_54_22
75. Seki S, Iwasaki M, Makino H, Yahara Y, Kondo M, Kamei K, et al. Association of ligamentum Flavum hypertrophy with adolescent idiopathic scoliosis progression-comparative microarray gene expression analysis. Int J Mol Sci. (2022) 23:5038–53. doi: 10.3390/ijms23095038
76. Biery NJ, Eldadah ZA, Moore CS, Stetten G, Spencer F, Dietz HC. Revised genomic organization of FBN1 and significance for regulated gene expression. Genomics. (1999) 56:70–7. doi: 10.1006/geno.1998.5697
77. de Azevedo GBL, Perini JA, Araújo Junior AEP, Moliterno LAM, Andrande RM, Guimarães JAM, et al. Association of FBN1 polymorphism with susceptibility of adolescent idiopathic scoliosis: a case-control study. BMC Musculoskelet Disord. (2022) 23:430. doi: 10.1186/s12891-022-05370-1
78. Le Goff C, Morice-Picard F, Dagoneau N, Wang LW, Perrot C, Crow YJ, et al. ADAMTSL2 Mutations in geleophysic dysplasia demonstrate a role for ADAMTS-like proteins in TGF-beta bioavailability regulation. Nat Genet. (2008) 40:1119–23. doi: 10.1038/ng.199
79. Robertson IB, Dias HF, Osuch IH, Lowe ED, Jensen SA, Redfield C, Handford PA (2017) The N-terminal region of fibrillin-1 mediates a bipartite interaction with LTBP1. Structure 25:1208–21.e1205. doi: 10.1016/j.str.2017.06.003
80. Sengle G, Tsutsui K, Keene DR, Tufa SF, Carlson EJ, Charbonneau NL, et al. Microenvironmental regulation by fibrillin-1. PLoS Genet. (2012) 8:e1002425. doi: 10.1371/journal.pgen.1002425
81. Hubmacher D, Wang LW, Mecham RP, Reinhardt DP, Apte SS. Adamtsl2 deletion results in bronchial fibrillin microfibril accumulation and bronchial epithelial dysplasia–a novel mouse model providing insights into geleophysic dysplasia. Dis Model Mech. (2015) 8:487–99. doi: 10.1242/dmm.017046
82. Ryzhkov II, Borzilov EE, Churnosov MI, Ataman AV, Dedkov AA, Polonikov AV. Transforming growth factor beta 1 is a novel susceptibility gene for adolescent idiopathic scoliosis. Spine (Phila Pa 1976). (2013) 38:E699–704. doi: 10.1097/BRS.0b013e31828de9e1
83. Liu B, Zhao S, Liu L, Du H, Zhao H, Wang S, et al. Aberrant interaction between mutated ADAMTSL2 and LTBP4 is associated with adolescent idiopathic scoliosis. Gene. (2022) 814:146126. doi: 10.1016/j.gene.2021.146126
84. Sheng F, Xia C, Xu L, Qin X, Tang NL, Qiu Y, et al. New evidence supporting the role of FBN1 in the development of adolescent idiopathic scoliosis. Spine (Phila Pa 1976). (2019) 44:E225–e232. doi: 10.1097/brs.0000000000002809
85. Kou I, Watanabe K, Takahashi Y, Momozawa Y, Khanshour A, Grauers A, et al. A multi-ethnic meta-analysis confirms the association of rs6570507 with adolescent idiopathic scoliosis. Sci Rep. (2018) 8:11575. doi: 10.1038/s41598-018-29011-7
86. Shi B, Xu L, Mao S, Xu L, Liu Z, Sun X, et al. Abnormal PITX1 gene methylation in adolescent idiopathic scoliosis: a pilot study. BMC Musculoskelet Disord. (2018) 19:138. doi: 10.1186/s12891-018-2054-2
Keywords: advances, genetic factors, adolescent idiopathic scoliosis, bibliometric analysis, CiteSpace
Citation: Jiang X, Liu F, Zhang M, Hu W, Zhao Y, Xia B and Xu K (2024) Advances in genetic factors of adolescent idiopathic scoliosis: a bibliometric analysis. Front. Pediatr. 11:1301137. doi: 10.3389/fped.2023.1301137
Received: 24 September 2023; Accepted: 11 December 2023;
Published: 3 January 2024.
Edited by:
Daniel Rodriguez-Almagro, University of Almeria, SpainReviewed by:
Anna Sowińska-Seidler, Poznan University of Medical Sciences, PolandDea Linia Romadhoni, University of Aisyiyah Surakarta, Indonesia
© 2024 Jiang, Liu, Zhang, Hu, Zhao, Xia and Xu. This is an open-access article distributed under the terms of the Creative Commons Attribution License (CC BY). The use, distribution or reproduction in other forums is permitted, provided the original author(s) and the copyright owner(s) are credited and that the original publication in this journal is cited, in accordance with accepted academic practice. No use, distribution or reproduction is permitted which does not comply with these terms.
*Correspondence: Fuyun Liu liufuyun111@126.com