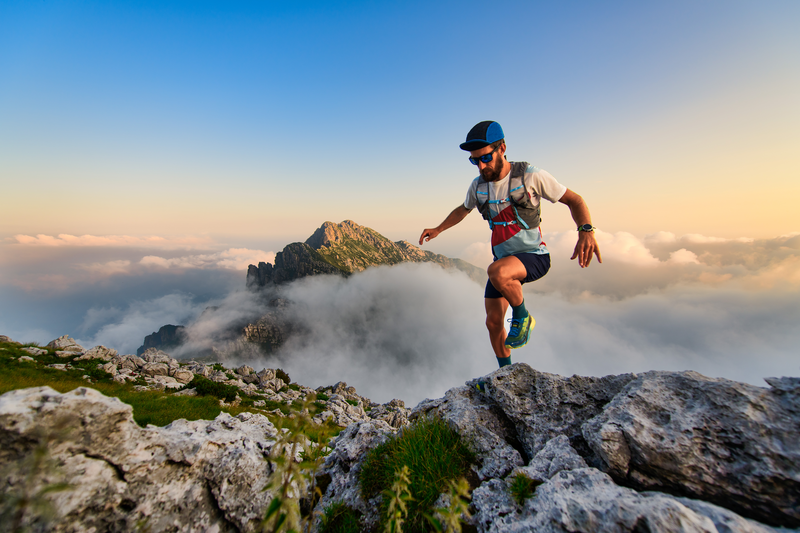
94% of researchers rate our articles as excellent or good
Learn more about the work of our research integrity team to safeguard the quality of each article we publish.
Find out more
ORIGINAL RESEARCH article
Front. Pediatr. , 12 December 2023
Sec. Pediatric Hematology and Hematological Malignancies
Volume 11 - 2023 | https://doi.org/10.3389/fped.2023.1272593
Objects: This study aimed to investigate the expression patterns and clinical significance of neural cell adhesion molecule-positive (CD56+) myelomonocytes in pediatric patients with moderate aplastic anemia (mAA).
Methods: Fifty-six pediatric patients with mAA were enrolled in this study. The patients' clinical characteristics, laboratory data, and response to cyclosporine therapy were obtained. CD56 expression on bone marrow myelomonocytic cells was investigated using flow cytometry. The association between aberrant CD56 expression and cyclosporine response was evaluated by a multivariate analysis.
Results: CD56+ myelomonocytes were detected in 43% of the mAA cases. Aberrant CD56 expression was frequent on immature CD45dimCD16dim granulocytes and mature CD45brightCD14bright monocytes. Compared with patients with CD56− myelomonocytes (CD56− patients), patients with CD56+ myelomonocytes (CD56+ patients) were in moderate hematological condition and had a distinct bone marrow cellular composition profile, which included an increased proportion of myeloid cells and CD56bright natural killer cells and a reduced proportion of CD4+ T cells, CD8+ T cells, and B cells. The multivariate analysis determined that CD56+ myelomonocytes were a favorable factor for achieving response at 6 months after cyclosporine therapy. There was a trend towards a lower 3-year rate of evolution to severe aplastic anemia or relapse among the CD56+ patients (8%) than the CD56− patients (22%).
Conclusion: CD56+ patients had an increased myeloid compartment and better prognosis compared with CD56− patients. The findings demonstrated the favorable role of CD56+ myelomonocytes in aplastic anemia progression.
Aplastic anemia (AA) is a rare but potentially life-threatening disorder (1). Most AA cases (70%–80%) are acquired and are caused by immune-mediated destruction of hematopoietic stem and progenitor cells (2, 3). The first-line therapy for AA depends on the patient's age and the severity of the disease. There is no standard management for patients with moderate aplastic anemia (mAA). Although mAA is generally recommended for observation alone until progression to severe aplastic anemia (sAA), the results of current studies justify an early intervention with cyclosporine (4, 5). However, the predictive factors for the efficacy of cyclosporine therapy have been less well studied.
Also known as neural cell adhesion molecule (NCAM), CD56 belongs to the immunoglobulin superfamily of cell adhesion molecules. CD56 mRNA is generated by alternative splicing from a single gene, but only three isoforms (NCAM-120, NCAM-140, NCAM-180) are commonly expressed (6, 7). Within the hematopoietic lineage, CD56 is an important marker that defines a population of natural cytotoxic cells, which include natural killer (NK) and NK-T cells (8). Furthermore, CD56 has been detected on other hematopoietic cells, including myeloid cells and monocytes (9–11). Laboratory and clinical observations suggested the unique role of CD56+ myelomonocytes in patients with malignant or autoimmune diseases. CD56 expression has been described in different acute myeloid leukemia (AML) genetic subtypes. Indeed, it is frequently associated with t(8;21) RUNX1-RUNX1T1 translocation, AML subtype generally associated with good prognosis (12), but also in the rare CBFA2T3-GLIS2 positive subtype, related with dismal prognosis (13). Patients with rheumatoid arthritis have increased CD56+ monocyte frequencies in the peripheral blood, where anti-TNF treatment reduces CD56+ monocyte frequency, and the reduction is associated with a better response (14).
Multiparameter flow cytometry reveals myelomonocytic CD56 expression in patients with AA (15). However, detailed analyses of aberrant CD56 expression in AA are limited. In the present study, we clarified the phenotype and function characteristics of CD56+ myelomonocytes and assessed whether CD56+ myelomonocytes had a predictive value for the outcomes of pediatric patients with AA.
Consecutive patients, diagnosed with mAA between January 2019 and December 2022, were enrolled. Bone marrow aspirate specimens for flow cytometric immunophenotyping were obtained from 56 patients before they received immunosuppressive therapy. Ten healthy pediatric stem cell donors served as normal controls. Ethical approval for this study was obtained from the Medical Ethics Committee of Wuhan Children's Hospital, Tongji Medical College, Huazhong University of Science and Technology.
The diagnosis of acquired AA was established according to the published criteria (16). Patients were considered to have mAA if they had ≥2 of the following: peripheral blood neutrophils <1.0 × 109 /L, platelets <50 × 109 /L, and reticulocytes <60 × 109 /L. sAA was defined as bone marrow cellularity <25% and at least two of the following: peripheral blood neutrophils <0.5 × 109/L, platelets <20 × 109 /L, and reticulocytes <20 × 109 /L.
The mAA patients received cyclosporine (5–6 mg/kg per day, serum level of 200–300 ng/ml) with or without the addition of granulocyte colony stimulating factor for patients with peripheral blood neutrophils <0.2 × 109 /L.
The hematologic response to cyclosporine was evaluated at 3 and 6 months after treatment initiation. A complete response (CR) was defined as achieving peripheral blood neutrophils >1.5 × 109 /L, platelets >100 × 109 /L, and hemoglobin >100 g/L. A partial response (PR) was defined as achieving peripheral blood neutrophils >1.0 × 109 /L, platelets >50 × 109 /L, and hemoglobin >80 g/L. Patients with no PR, no CR, or requirement for blood transfusion were deemed as having no response. Relapse was defined by conversion to no response from PR or CR and/or the requirement of blood product transfusion (17).
Bone marrow aspirate specimens were obtained at the time of first diagnosis of AA and before treatment initiation, where 3 × 105 nucleated cells per sample were analyzed. Immunophenotyping was performed by multiparameter flow cytometry (BD FACS Canto II system, BD Biosciences, San Jose, CA, USA). The flow panels included the markers CD2, CD3, CD4, CD5, CD7, CD8, CD10, CD11b, CD13, CD14, CD16, CD19, CD20, CD33, CD34, CD38, CD56, CD64, CD71, CD117, HLA-DR, TCRαβ, TCRγδ, and CD45. CD marker expression of >10% was deemed positive (18). Immature/mature granulocytes and monocytes were identified using a CD45/sideward scatter (SSC) backbone strategy and lineage-defined gating, as previously reported (19, 20). Briefly, granulocytes were identified as SSCint/highCD45dim/brightCD33dim, and monocytes were identified as SSCintCD45dim/brightCD64+. Granulocytes and monocytes were also identified by differentiation antigen CD14 and CD16, respectively. Aberrant expression was determined on the basis of deviation from normal patterns (expression intensity at least 0.5 decades disparate from the corresponding normal cells from healthy pediatric stem cell donors). The percentage of the CD56+ population in myelomonocytes was assessed in a graded regimen: +, positive in 10%–30%; ++, positive in 31%–60%; +++, positive in >60%; negative, positive in <10%. The mean fluorescence intensities of CD56+ myelomonocytes were recorded.
All calculations were performed using SPSS 20 (IBM, Armonk, NY, USA). Continuous variables with non-normal distribution are expressed as the median and range, and the Mann–Whitney U-test was used for statistical analysis. Categorical variables are presented as frequencies and proportions (%) and analyzed by the χ2 test (or Fisher's exact test for expected cell frequencies <5). The associations between clinical variables with response to cyclosporine were calculated by a multivariate logistic regression analysis. The Kaplan–Meier curve demonstrated the cumulative incidence of the response to cyclosporine and time to event, and the differences were evaluated by the log-rank test. P < 0.05 was considered statistically significant.
Of the 56 mAA patients enrolled, 29 were male and 27 were female (male:female ratio, 1.07:1). The median age was 4.7 years (range: 0.1–15.7 years). Table 1 presents the patients' characteristics. The median time between diagnosis and treatment was 7 days (range: 0–35 days). At mAA diagnosis, 14 patients (25%) presented with infection and the median C-reactive protein (CRP) level was 2.11 mg/L (range: 0.10–118.9 mg/L). Among the 56 patients, 24 had CD56+ myelomonocytes and were classified as CD56+ patients. The remaining 32 patients without CD56+ myelomonocytes were classified as CD56− patients. There were no significant differences between the CD56+ and CD56− patients in the gender and age distributions, duration between diagnosis and treatment, presence of infection, CRP concentration, and platelet count. However, the CD56+ patients had higher neutrophil counts and hemoglobin levels than the CD56− patients at the time of diagnosis.
Analysis of the patients' bone marrow immunophenotype data revealed that all cases had normal expression of lymphocyte subset cell surface markers. The lymphocytic antigens involved in this study included: the T cell markers CD2, CD3, CD4, CD5, CD7, and CD8; B cell markers CD19 and CD20; and the NK cell markers CD16 and CD56. Immature/mature granulocytes and monocytes exhibited normal myelomonocytic markers: CD4, CD11b, CD13, CD14, CD16, CD33, CD64, and HLA-DR.
Twenty-four patients (24/56, 43%) had CD56+ myelomonocytes [three cases (13%) with CD56+ granulocytes, seven cases (29%) with CD56+ monocytes, and 14 cases (58%) with both CD56+ granulocytes and CD56+ monocytes]. CD56 was expressed frequently on CD16dimCD33dim immature granulocytes (15/24, 62.5%) and CD14brightCD64+ mature monocytes (18/24, 75%). Aberrant CD56 expression was also observed on CD16brightCD33dim mature granulocytes (5/24, 20.8%) and CD14dimCD64+ immature monocytes (3/24, 12.5%), but were infrequent. Table 2 details the immunophenotyping results. Figure 1 depicts representative CD56 dot plot distributions of the CD56+ and CD56− patients on flow cytometry. Figure 2 shows the profile of mean fluorescence intensity of CD56+ myelomonocytes.
Figure 1. Flow cytometric analysis of myelomonocytic CD56 expression in normal control, CD56− patients, and CD56+ patients. Cell populations were identified using CD45 expression and sideward scatter (SSC). Granulocytes were defined as SSCint/high/CD45dim/bright/CD33dim cells. Monocytes were defined as SSCint/CD45dim/bright/CD64+ cells. CD16/CD56 and CD14/CD56 dot plots demonstrate aberrant CD56 expression on granulocytes and monocytes, respectively. (A) Normal CD56 expression in healthy control bone marrow. (B) Normal CD56 expression in mAA. (C) Aberrant CD56 expression on granulocytes and monocytes in mAA.
Figure 2. (A) Representative fluorescence spectrum showing CD56 expression in normal controls, CD56− patients and CD56+ patients. (B and C) Mean fluorescence intensity (MFI) of granulocytes (B) and monocytes (C) of normal controls, CD56− patients, and CD56+ patients. Values in bold denote statistical significance at the level P < 0.001.
The proportions of monocytes, granulocytes, and lymphocytes in nucleated cells were assessed by flow cytometry. Compared with the CD56− patients, the CD56+ patients had increased percentages of monocytes (median, 6.5% vs. 4.1%) and granulocytes (median: 42.4% vs. 17.8%; Figures 3A,B) and a higher proportion of mature monocytes (Figures 3C,D). The CD56+ patients had a higher percentage of immature granulocytes than the CD56− patients (median: 16.2% vs. 4.3%) (Figure 3E).
Figure 3. Cellular composition of bone marrow in CD56− and CD56+ patients. (A–F) Box plots depict the percentage of total monocytes (A), total granulocytes (B), mature monocytes (C), immature monocytes (D), immature granulocytes (E), and mature granulocytes (F) in CD56− and CD56+ patients. (G–J) Box plots depict the percentage of lymphocytes (G), B cells (H), CD4+ T cells (I), and CD8+ T cells (J) in CD56− and CD56+ patients. (K) Box plot depicts the lymphoid/myeloid ratios in CD56− and CD56+ patients. (L) Box plot depicts the percentage of CD56bright NK cells in CD56− and CD56+ patients. Lines in the boxes indicate medians; bottom and top whiskers indicate minimum and maximum values, respectively. The cell population percentage represented the proportion within all nucleated cells. Values in bold denote statistical significance at the level P < 0.05.
Paralleling the increased immature granulocytes, the CD56+ patients had an increased percentage of mature granulocytes compared with the CD56− patients (median: 17.6% vs. 6.3%; Figure 3F). Likely partly due to the increased myeloid cells, the CD56+ patients had a strongly reduced proportion of lymphocytes compared with the CD56− patients (median: 23.9% vs. 59.6%), which included reduced B cells, CD4+ T cells, and CD8+ T cells (Figures 3G–J). Unsurprisingly, the CD56+ patients had a lower lymphoid/myeloid ratio than the CD56− patients (median: 0.4 vs. 2.7) (Figure 3K). Interestingly, the CD56+ patients had an increased percentage of CD56bright NK cells compared with the CD56− patients (median: 9.7% vs. 5.8%, Figure 3L).
In China, cyclosporine is more frequently used for pediatric AA as it costs less and produces fewer adverse effects than anti-thymocyte globulin (21, 22). We evaluated the hematologic response of pediatric mAA patients to cyclosporine therapy at 3 and 6 months after treatment initiation. The 3-month response rate to cyclosporine did not differ significantly between the two groups (33% vs. 16%, P = 0.200, Figure 4A). At 6 months, 83% of the CD56+ patients achieved PR or CR. However, only 28% of the CD56− patients responded to cyclosporine (P < 0.001, Figure 4B). Kaplan-Meier analysis demonstrated that the cumulative response rate of the CD56+ patients was significantly higher than that of the CD56− patients (P < 0.001, Figure 4C). We also found that the cumulative response rates had no difference between patients with CD56+ mature monocytes and CD56+ immature monocytes (Supplementary Figure S1). Multivariate analysis of laboratory variables at the time of diagnosis revealed that age (older or younger than 4.7 years), sex (male or female), presence or absence of infection, peripheral platelet (more or less than 20 × 103 /µl), and reticulocyte count (more or less than 20 × 103/µl) could not be deemed as predictors. Only two parameters (peripheral neutrophils >500 /µl and abnormal CD56 expression) were significant factors associated with good response to cyclosporine therapy at 6 months (Table 3). Subsequently, we analyzed the patients' prognoses after cyclosporine therapy. The median follow-up time was 20.5 months (range: 10.2–40.4 months). In contrast to the response rate, the probability of evolution into sAA or relapse at 3 years tended to be higher in CD56− patients (22%) than in CD56+ patients (8%) (Figure 4D).
Figure 4. Response to cyclosporine therapy in CD56− and CD56+ patients. (A, B) Bar graphs depict the response rate to cyclosporine treatment at 3 (A) and 6 months (B) (C) Cumulative PR or CR incidence after cyclosporine treatment. (D) Cumulative incidence of sAA or relapse after cyclosporine treatment. CSA, cyclosporine. Values in bold denote statistical significance at the level P < 0.05.
Table 3. Multivariate analysis of predictive factors for response to cyclosporine therapy at 6 months.
In this study, we evaluated the bone marrow cellular component in CD56+ or CD56− mAA patients and the relationship between myelomonocytic CD56 expression and the response to cyclosporine therapy. We determined that the CD56+ patients had less severe myeloid cell reduction in comparison with the CD56− patients. Furthermore, we detected a better response to cyclosporine among the CD56+ patients than the CD56− patients.
To date, the functional role of CD56+ immune cells is not fully understood, but CD56 expression has been hypothesized to promote immune cell proliferation and differentiation (23, 24). A proposed mechanism for the functional role of CD56 depends on its binding to a number of proteins or extracellular matrix molecules. In particular, the homophilic interaction between CD56 molecules can induce the preferential activation and expansion of CD56+ immune cells (25, 26). Additionally, upregulated CD56 expression is strongly associated with activation of the MAPK signaling pathway, leading to increased glycolysis and resistance to apoptosis (27, 28). These pathophysiological mechanisms may explain the possible reason the CD56+ patients had better hematological conditions than the CD56− patients.
Several studies highlighted the immune regulation imbalance in patients with acquired AA (29–32). Immune dysfunction occurs mainly due to the cellular hyperimmune state and leads to a corresponding immune tolerance disorder, where the overactivated immune cells in such patients are unable to recognize auto-hematopoietic cells. CD8+ T lymphocytes are the main effector cells in the immune system. CD8+ T cell clonal amplification and overactivation inhibits bone marrow hematopoietic functions and induces hematopoietic cell apoptosis (31, 33). NK cells are crucial in AA pathogenesis (29). Specifically, CD56bright NK cells inhibit T cell immunity overactivation and subsequently reduce the T cell-induced damage of hematopoietic stem and progenitor cells in AA (34, 35). In the present study, the CD56+ patients demonstrated a strong decrease in CD8+ T cells and a significant increase in CD56bright NK cells compared with the CD56− patients. The homophilic interaction function of CD56 might explain the low percentage of cytotoxic lymphocytes in CD56+ patients. CD56 was reported to cooperate with leukocyte function-associated antigen-1 and -3 to enhance interactions between CD56+ immune cells, leading to CD56-mediated lysis (36, 37). As a consequence, we speculate that CD56+ populations might reshape the immune balance of the bone marrow microenvironment and be responsible for the higher response rate to cyclosporine therapy in mAA patients.
Several limitations in our study merit consideration. First, only 24 CD56+ patients were enrolled due to the small patient cohort. Second, sAA patients were not enrolled in this study due to the severe bone marrow hypocellularity. Testing for myelomonocytic CD56 expression at diagnosis had poor sensitivity for sAA. Finally, this study was comparatively preliminary, and the exact pathological mechanism of CD56+ myelomonocytes in pediatric patients with acquired AA remains unclear.
In summary, we demonstrated that CD56+ myelomonocytes accompanied an increased percentage of myeloid cells in the bone marrow of mAA patients, which suggested the remodeling effect of myelomonocytic CD56 expression on bone marrow cellular components. Importantly, we determined that myelomonocytic CD56 expression was associated with a better response to cyclosporine therapy. Additional studies with a larger patient cohort are needed to validate myelomonocytic CD56 expression as a response predictor in patients with acquired AA.
The raw data supporting the conclusions of this article will be made available by the authors, without undue reservation.
The studies involving humans were approved by Medical Ethics Committee of Wuhan Children's Hospital, Tongji Medical College, Huazhong University of Science and Technology. The studies were conducted in accordance with the local legislation and institutional requirements. Written informed consent for participation in this study was provided by the participants’ legal guardians/next of kin.
SQ: Funding acquisition, Writing – review & editing, Data curation, Formal Analysis, Investigation, Writing – original draft. YD: Data curation, Writing – review & editing, Methodology. MS: Methodology, Writing – review & editing, Software. LZ: Methodology, Writing – review & editing, Data curation. ZC: Data curation, Methodology, Writing – review & editing. HX: Writing – review & editing, Funding acquisition.
The author(s) declare financial support was received for the research, authorship, and/or publication of this article.
This study was supported by the Natural Science Foundation Program of Hubei Province (2020CFB364), Research Program on Applied Fundamentals and Frontier Technologies of Wuhan (2020020601012319), and Foundation Program of Wuhan Children's hospital (2021FE001).
We thank all the patients involved in the study for their cooperation.
The authors declare that the research was conducted in the absence of any commercial or financial relationships that could be construed as a potential conflict of interest.
All claims expressed in this article are solely those of the authors and do not necessarily represent those of their affiliated organizations, or those of the publisher, the editors and the reviewers. Any product that may be evaluated in this article, or claim that may be made by its manufacturer, is not guaranteed or endorsed by the publisher.
The Supplementary Material for this article can be found online at: https://www.frontiersin.org/articles/10.3389/fped.2023.1272593/full#supplementary-material
1. Brodsky RA, Jones RJ. Aplastic anaemia. Lancet (London, England). (2005) 365:1647–56. doi: 10.1016/S0140-6736(05)66515-4
2. Nissen-Druey C. Pathophysiology of aplastic anaemia. Blood Rev. (1990) 4:97–102. doi: 10.1016/0268-960X(90)90032-N
3. Wang L, Liu H. Pathogenesis of aplastic anemia. Hematology (Amsterdam, Netherlands). (2019) 24:559–66. doi: 10.1080/16078454.2019.1642548
4. Nishikawa E, Yagasaki H, Hama A, Yabe H, Ohara A, Kosaka Y, et al. Long-term outcomes of 95 children with moderate aplastic anemia treated with horse antithymocyte globulin and cyclosporine. Pediatr Blood Cancer. (2017) 64:1–6. doi: 10.1002/pbc.26305
5. Fattizzo B, Gurnari C, Cassanello G, Bortolotti M, Awada H, Giammarco S, et al. Deciphering treatment patterns in non-severe/moderate aplastic anemia: an international observational study. Leukemia. (2023) 37:2479–85. doi: 10.1038/s41375-023-02047-z
6. Walmod PS, Kolkova K, Berezin V, Bock E. Zippers make signals: NCAM-mediated molecular interactions and signal transduction. Neurochem Res. (2004) 29:2015–35. doi: 10.1007/s11064-004-6875-z
7. Ditlevsen DK, Povlsen GK, Berezin V, Bock E. NCAM-induced intracellular signaling revisited. J Neurosci Res. (2008) 86:727–43. doi: 10.1002/jnr.21551
8. Vivier E, Tomasello E, Baratin M, Walzer T, Ugolini S. Functions of natural killer cells. Nat Immunol. (2008) 9:503–10. doi: 10.1038/ni1582
9. Wells DA, Benesch M, Loken MR, Vallejo C, Myerson D, Leisenring WM, et al. Myeloid and monocytic dyspoiesis as determined by flow cytometric scoring in myelodysplastic syndrome correlates with the IPSS and with outcome after hematopoietic stem cell transplantation. Blood. (2003) 102:394–403. doi: 10.1182/blood-2002-09-2768
10. Westers TM, Ireland R, Kern W, Alhan C, Balleisen JS, Bettelheim P, et al. Standardization of flow cytometry in myelodysplastic syndromes: a report from an international consortium and the European LeukemiaNet working group. Leukemia. (2012) 26:1730–41. doi: 10.1038/leu.2012.30
11. Langebrake C, Klusmann JH, Wortmann K, Kolar M, Puhlmann U, Reinhardt D. Concomitant aberrant overexpression of RUNX1 and NCAM in regenerating bone marrow of myeloid leukemia of down’s syndrome. Haematologica. (2006) 91:1473–80. doi: 10.1002/pbc.26305
12. Sun Y, Wan J, Song Q, Luo C, Li X, Luo Y, et al. Prognostic significance of CD56 antigen expression in patients with De Novo non-M3 acute myeloid leukemia. BioMed Res Int. (2021) 2021:1929357. doi: 10.1155/2021/1929357
13. Zangrando A, Cavagnero F, Scarparo P, Varotto E, Francescato S, Tregnago C, et al. CD56, HLA-DR, and CD45 recognize a subtype of childhood AML harboring CBFA2T3-GLIS2 fusion transcript. Cytometry A. (2021) 99:844–50. doi: 10.1002/cyto.a.24339
14. Krasselt M, Baerwald C, Wagner U, Rossol M. CD56+ monocytes have a dysregulated cytokine response to lipopolysaccharide and accumulate in rheumatoid arthritis and immunosenescence. Arthritis Res Ther. (2013) 15:R139. doi: 10.1186/ar4321
15. Elghetany MT. Diagnostic utility of flow cytometric immunophenotyping in myelodysplastic syndrome. Blood. (2002) 99:391–2. doi: 10.1182/blood.V99.1.391
16. Heimpel H. Incidence of aplastic anemia: the relevance of diagnostic criteria. By the international agranulocytosis and aplastic Anemia study. Blood. (1987) 70:1718–21. doi: 10.1182/blood.V70.6.1718.1718
17. Kojima S, Hibi S, Kosaka Y, Yamamoto M, Tsuchida M, Mugishima H, et al. Immunosuppressive therapy using antithymocyte globulin, cyclosporine, and danazol with or without human granulocyte colony-stimulating factor in children with acquired aplastic anemia. Blood. (2000) 96:2049–54. doi: 10.1182/blood.V96.6.2049
18. Gadgeel M, AlQanber B, Buck S, Taub JW, Ravindranath Y, Savaşan S. Aberrant myelomonocytic CD56 expression in down syndrome is frequent and not associated with leukemogenesis. Ann Hematol. (2021) 100:1695–700. doi: 10.1007/s00277-021-04531-x
19. Chen W, Luu HS. Immunophenotyping by multiparameter flow cytometry. Methods Mol Biol (Clifton, N.J.). (2017) 1633:51–73. doi: 10.1007/978-1-4939-7142-8_4
20. Kern W, Haferlach C, Schnittger S, Haferlach T. Clinical utility of multiparameter flow cytometry in the diagnosis of 1013 patients with suspected myelodysplastic syndrome: correlation to cytomorphology, cytogenetics, and clinical data. Cancer. (2010) 116:4549–63. doi: 10.1002/cncr.25353
21. Liu C, Shao Z. Aplastic Anemia in China. J Transl Intern Med. (2018) 6:134–7. doi: 10.2478/jtim-2018-0028
22. Zhu XF, He HL, Wang SQ, Tang JY, Han B, Zhang DH, et al. Current treatment patterns of aplastic Anemia in China: a prospective cohort registry study. Acta Haematol. (2019) 142:162–70. doi: 10.1159/000499065
23. Kato J, Hisha H, Wang XL, Mizokami T, Okazaki S, Li Q, et al. Contribution of neural cell adhesion molecule (NCAM) to hemopoietic system in monkeys. Ann Hematol. (2008) 87:797–807. doi: 10.1007/s00277-008-0513-9
24. Grzywacz B, Moench L, McKenna D, Tessier KM Jr, Bachanova V, Cooley S, et al. Natural killer cell homing and persistence in the bone marrow after adoptive immunotherapy correlates with better leukemia control. J Immunothe (Hagerstown, Md.: 1997). (2019) 42:65–72. doi: 10.1097/CJI.0000000000000250
25. Nieda M, Terunuma H, Eiraku Y, Deng X, Nicol AJ. Effective induction of melanoma-antigen-specific CD8+ T cells via Vγ9γδT cell expansion by CD56(high+) interferon-α-induced dendritic cells. Exp Dermatol. (2015) 24:35–41. doi: 10.1111/exd.12581
26. Mace EM, Gunesch JT, Dixon A, Orange JS. Human NK cell development requires CD56-mediated motility and formation of the developmental synapse. Nat Commun. (2016) 7:12171. doi: 10.1038/ncomms12171
27. Correia MP, Costa AV, Uhrberg M, Cardoso EM, Arosa FA. IL-15 induces CD8+ T cells to acquire functional NK receptors capable of modulating cytotoxicity and cytokine secretion. Immunobiology. (2011) 216:604–12. doi: 10.1016/j.imbio.2010.09.012
28. Sasca D, Szybinski J, Schüler A, Shah V, Heidelberger J, Haehnel PS, et al. NCAM1 (CD56) promotes leukemogenesis and confers drug resistance in AML. Blood. (2019) 133:2305–19. doi: 10.1182/blood-2018-12-889725
29. Liu C, Li Z, Sheng W, Fu R, Li L, Zhang T, et al. Abnormalities of quantities and functions of natural killer cells in severe aplastic anemia. Immunol Investig. (2014) 43:491–503. doi: 10.3109/08820139.2014.888448
30. Fu R, Liu H, Zhang J, Liu C, Ding S, Li L, et al. Expression of NK-activating receptor-NKp46/NCR1 on NK cells in patients with severe aplastic Anemia. Clin Lab. (2015) 61:1221–9. doi: 10.7754/clin.lab.2015.150130
31. Luzzatto L, Risitano AM. Advances in understanding the pathogenesis of acquired aplastic anaemia. Br J Haematol. (2018) 182:758–76. doi: 10.1111/bjh.15443
32. Zhu C, Lian Y, Wang C, Wu P, Li X, Gao Y, et al. Single-cell transcriptomics dissects hematopoietic cell destruction and T-cell engagement in aplastic anemia. Blood. (2021) 138:23–33. doi: 10.1182/blood.2020008966
33. Balderman SR, Calvi LM. Biology of BM failure syndromes: role of microenvironment and niches. Hematology Am Soc Hematol Educ Program. (2014) 2014:71–6. doi: 10.1182/asheducation-2014.1.71
34. Zhang T, Yuan X, Liu C, Li Y, Liu H, Li L, et al. Decreased TIM-3 expression of peripheral blood natural killer cells in patients with severe aplastic anemia. Cell Immunol. (2017) 318:17–22. doi: 10.1016/j.cellimm.2017.03.003
35. Chen T, Liu C, Li L, Liu H, Wang T, Shao Z, et al. CD56(bright) natural killer cells exhibit abnormal phenotype and function in severe aplastic anemia. Int J Lab Hematol. (2019) 41:353–63. doi: 10.1111/ijlh.12982
36. Nitta T, Yagita H, Sato K, Okumura K. Involvement of CD56 (NKH-1/leu-19 antigen) as an adhesion molecule in natural killer-target cell interaction. J Exp Med. (1989) 170:1757–61. doi: 10.1084/jem.170.5.1757
Keywords: CD56+ myelomonocytes, cyclosporine, moderate aplastic anemia, pediatric aplastic anemia, response
Citation: Qi S, Du Y, Sun M, Zhang L, Chen Z and Xiong H (2023) Aberrant myelomonocytic CD56 expression predicts response to cyclosporine therapy in pediatric patients with moderate aplastic anemia. Front. Pediatr. 11:1272593. doi: 10.3389/fped.2023.1272593
Received: 4 August 2023; Accepted: 29 November 2023;
Published: 12 December 2023.
Edited by:
Tomasz Szczepanski, Medical University of Silesia, PolandReviewed by:
Marcin Wlodarski, University of Freiburg Medical Center, Germany© 2023 Qi, Du, Sun, Zhang, Chen and Xiong. This is an open-access article distributed under the terms of the Creative Commons Attribution License (CC BY). The use, distribution or reproduction in other forums is permitted, provided the original author(s) and the copyright owner(s) are credited and that the original publication in this journal is cited, in accordance with accepted academic practice. No use, distribution or reproduction is permitted which does not comply with these terms.
*Correspondence: Hao Xiong eGlvbmdoYW9fd2hjQHNpbmEuY29t
Abbreviations AA, aplastic anemia; AML, acute myeloid leukemia; CR, complete response; mAA, moderate aplastic anemia; CRP, C-reactive protein; NCAM, neural cell adhesion molecule; NK cells, natural killer cells; PR, partial response; sAA, severe aplastic anemia.
Disclaimer: All claims expressed in this article are solely those of the authors and do not necessarily represent those of their affiliated organizations, or those of the publisher, the editors and the reviewers. Any product that may be evaluated in this article or claim that may be made by its manufacturer is not guaranteed or endorsed by the publisher.
Research integrity at Frontiers
Learn more about the work of our research integrity team to safeguard the quality of each article we publish.