- 1Department of Pediatrics, West China Second University Hospital, Sichuan University, Chengdu, China
- 2Key Laboratory of Birth Defects and Related Disease of Women and Children, Ministry of Education, Sichuan University, Chengdu, China
- 3Department of Clinical Medicine, BinZhou Medical College, Yantai, China
Background: Hypoxia can threaten the metabolic functions of different systems in immature neonates, particularly the central nervous system. The red blood cell distribution width (RDW) has recently been reported as a prognostic factor in neurologic diseases. Herein, we examined the correlation between RDW and regional cerebral tissue oxygen saturation (rcSO2).
Methods: This cross-sectional study included 110 preterm infants born at a gestational age (GA) of <32 weeks, or with a birth weight (BW) of <1,500 g at our institution between January and June 2,022. The rcSO2 was monitored using near-infrared spectroscopy, and RDW was extracted from the complete blood count during the first 14 days after birth. RDW and rcSO2 measurements were analyzed using a cross-sectional research method.
Results: We divided the study population into two groups, with a mean rcSO2 value over the first 14 days. Fifty-three preterm had rcSO2 ≥ 55% and 57% < 55%. The 14-days-mean in the study population showing an association of lower rcSO2 values with higher RDW values. Significantly higher RDW values were observed in the low rcSO2 group compared with those in the high rcSO2 group. Threshold effect analysis showed that rcSO2 decreased with RDW values ≥18% (β, −0.03; 95% CI, −0.04 and −0.02; p ≥ 0.0001). After adjusting for potential confounders, an RDW of ≥18% was determined as the predictive cutoff value for preterm infants with low rcSO2 (Model I: OR, 3.31; 95% CI, 1.36–8.06; p = 0.009; and Model II: OR, 3.31; 95% CI, 1.28–8.53; p = 0.013).
Conclusions: An RDW of ≥18% in the first 14 days is associated with rcSO2 of <55% in preterm infants.
1. Introduction
Despite recent improvements in perinatal care, brain vulnerability in critically ill preterm newborns still represents a challenge. Brain hypoperfusion during the immediate postnatal period has been reported to be related to cerebral damage in sick preterm infants (1). If not corrected in time, hypoxia can lead to poor clinical outcomes, including cerebral palsy, different brain disabilities or death (2, 3).
1.1. Red blood cell distribution width
Red cell distribution width (RDW) is an automatically measured complete blood count (CBC) parameter that reflects the degree of heterogeneity in erythrocyte size (4). The RDW is calculated from the distribution of red blood cell (RBC) volumes, measured by impedance, using the following formula:
The “width” in RDW refers to the width of the volume distribution histogram, expressed as a coefficient of variation. In samples with mixtures of small and large RBCs, the histogram is wider; thus, the RDW value is higher. In contrast, in samples with a homogeneous red cell size, the histogram is narrower; thus, the RDW is lower. The normal reference range for RDW among healthy adults used in most laboratories is 11% (lower reference interval limit) to 15% (upper reference interval limit) (5). In 1987, Dr. Robert Novak reported that the normal range of RDW in infants ranged from 11.5% to 14.5% (6). A recent study of 144 infants reported that the lower reference limit for RDW in premature infants at <72 h of age is 14.2%, while the upper reference limit is 21.8% (4). However, due both to the difference in methods of measuring RBC size, instruments, researchers, laboratory standards, and statistical approaches between laboratories, as well as the ethical and practical challenges unique to pediatric care, thus far, no universal reference range has yet been established for pediatric patients (7).
Recently, RDW levels have been shown to be associated with neonatal mortality and/or significant morbidity, including neonatal sepsis, intrauterine growth restriction (IUGR), and bronchopulmonary dysplasia (BPD), etc., (8–10). The RDW has also been indicated as a useful tool for evaluating the medical conditions of newborns, especially preterm infants (11, 12).
1.2. Preterm cerebral oxygen
Regional cerebral tissue oxygen saturation (rcSO2) can be measured by using near-infrared spectroscopy (NIRS), a frequently used noninvasive technique (13). RcSO2 primarily reflects the brain venous compartment, as it is larger than other compartments; however, it is also sensitive to changes in arterial parameters. RcSO2 increases with time after birth, and tends to reach a plateau at 8–10 min after birth (14, 15). However, great individual variability can be seen among patients, and depending on the location. A prior publication provided an rcSO2 range in preterm infants of 55%–85% (16). An rScO2 value of 55% is close to or below the thresholds reported to be associated with neuronal damage and adverse neurodevelopmental outcome (17).
Conventional pulse oximeter measures arterial oxygen saturation around the limb, meaning that pulse oxygen saturation (SpO2) does not include information on local tissue oxygen utilization (18). Cerebral oxygenation is influenced by three main components: the blood oxygen content, cerebral perfusion, and oxygen consumption. Each of these factors are themselves influenced by several other variables, including SpO2, blood glucose level, partial pressure of carbon dioxide, blood pressure, and hemoglobin level (19). NIRS measures composite arterial, capillary, and venous blood saturation in the brain tissue; as such, rcSO2 can reflect real-time subtle changes in tissue oxygenation in the brain (20). Studies have found that the neonates with adverse outcome (including intraventricular haemorrhage) had significantly lower rcSO2 values during the immediate transition, although there was no difference concerning SpO2 (21, 22). Therefore, potential cerebral hypoxia could not be identified with SpO2 monitoring. Although in a research involving extremely preterm infants, treatment guided by cerebral oximetry monitoring in the first 72 h after birth did not lead to a lower incidence of death or severe brain injury at 36 weeks' postmenstrual age than usual care (23), a previously conducted interventional trial performed by the SafeBoosCII study group demonstrated that the combination of cerebral oxygenation monitoring by NIRS combined with an evidence-based treatment guideline significantly reduced the burden of cerebral hypoxia (24, 25). Other studies have reported high sensitivity of NIRS-detected variables of rcSO2 to hypoxemic and ischemic events occurring in preterm infants (26).
1.3. Relationship between RDW and rcSO2
Due to their ability to deform and flow in the microvascular network, RBCs have many vital physiological functions in our body, including carrying oxygen and carbon dioxide and gas exchange between blood and tissues (27). However, Patel et al. found that when the RDW exceeds 14% in microvascular disorders, the deformability of RBCs decreases (28), which may affect these aforementioned functions. In newborns, ineffective erythropoiesis due to prematurity (4), hemolysis, erythrocyte transfusions, and alterations in osmolality under some pathophysiological conditions can all decrease the ability of RBCs to deform, triggering an increase in RDW, thereby resulting in low microvascular perfusion (29, 30). We hypothesized that a high RDW value would be associated with an increased risk of low rcSO2 based on analyses of existing clinical data.
This study was therefore conducted to investigate the relationship between RDW and rcSO2 in preterm neonates.
2. Methods
2.1. Study population
The study population consisted of 110 preterm infants born at <32 weeks or with birth weight (BW) <1,500 g between January and June 2022 at the neonatal intensive care unit (NICU) of the West China Second University Hospital, Sichuan University. The exclusion criteria were as follows: advanced resuscitation in the delivery room, cardiovascular instability requiring treatment with inotropes, anemia, and major congenital or chromosomal anomalies. This study was approved by the Ethics Committee of the West China Second University Hospital, Sichuan University (2022–67). Written and verbal informed consent was obtained from the infants' parents, and the study was registered on ChineseClinicalTrials.gov (09/04/2022, ChiCTR2200058482).
2.2. Study design
This study had a cross-sectional design. An EGOS-600B Cerebral/Somatic Oximeter device was used for rcSO2 monitoring during the study period, with neonatal NIRS sensors (NIRSensor, Enginmed, A1Z2104110037) placed on the frontoparietal regions of the infants. NIRS monitoring was performed thrice daily for the first 14 days after birth. All data were obtained when the infants were mostly quiet or sleeping in the supine position. No handling or intervention was performed during NIRS monitoring. Recorded values were stored and used for offline analyses. NIRS data analysis was performed after the data were transferred to a computer in Excel format using a customized programmer for the EGOS device. Those with markers likely related to an artifact (during handling, positioning, etc.) were excluded for data cleaning and were averaged afterward.
Blood samples were extracted from all preterm infants once a week on the first and second week of life. The volume for blood sampling was 0.50 ml. RDW values were extracted from the CBC, and the average was calculated. Neonatal and perinatal characteristics, including gestational age (GA), BW, sex, mode of delivery, IUGR, hypertension during pregnancy, chorioamnionitis (CA), gestational diabetes mellitus (GDM), premature rupture of membranes (PROM), multiple pregnancies, overall dose of antenatal corticosteroid treatment, invasive mechanical ventilation, breast feeding, and blood transfusion, were recorded prospectively. Neonatal morbidities included early-onset sepsis (EOS), late-onset sepsis (LOS), and intraventricular hemorrhage (IVH).
2.3. Statistical analysis
According to the existing literature and clinical judgment, we divided the study population into two groups, with a mean rcSO2 value over the first 14 days of 55% as the cut-off limit. For descriptive analyses, continuous variables are described as the means ± standard deviations (SD), and categorical data are presented as numbers and percentages (31). The distribution of covariates in the rcSO2 ≥ 55% and rcSO2 < 55% groups was compared using the t-test (normal distribution) or Kruskal–Wallis rank sum test (non-normal distribution) for continuous variables, and the chi-squared test for categorical data (Table 1).
We used a generalized additive model (GAM) to investigate the dose–response relationship between RDW values and rcSO2 (Figure 1), and subsequently used a two-piece-wise linear regression model to examine the threshold effect of the RDW value on rcSO2 (Table 2). The threshold level (i.e., inflection point) was determined using a trial-and-error approach. This involved selecting inflection points along a predefined interval, and choosing the inflection point that resulted in the maximum model likelihood. We further conducted a log-likelihood ratio test to compare the one-line linear regression model with the two-piecewise linear model, as previously described (32).
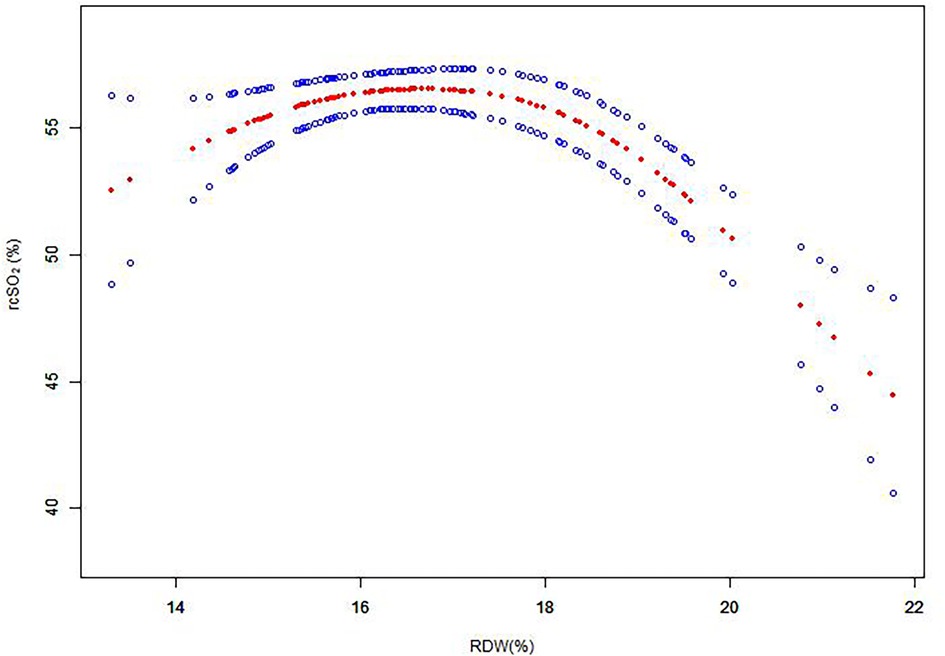
Figure 1. Association between RDW and rcSO2 over the first 14 days after birth. A threshold, nonlinear association between RDW and rcSO2 was determined (p < 0.05) from a generalized additive model. Red line: smooth curve fit between variables; blue bands: 95% CI from the fit. RDW, red blood cell distribution width; rcSO2, regional cerebral tissue oxygen saturation; CI, confidence interval.
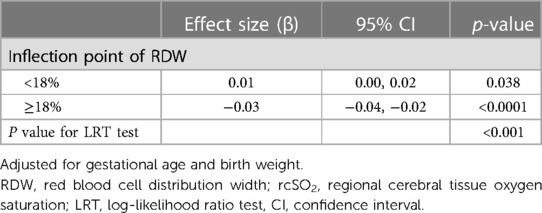
Table 2. Threshold effect analysis of the RDW and rcSO2 over the first 14 days after birth using piece-wise linear regression.
Next, univariate logistic regression (Table 3) and multivariate logistic regression models (Table 4) were applied to examine whether a high RDW was associated with low rcSO2 levels in preterm neonates. The statistical results are displayed as odds ratio (OR), with the corresponding 95% CI. We adjusted for variables deemed to be confounders according to the existing literature and clinical judgment. All statistical analyses were performed using EmpowerStats (www.empowerstats.com, X&Y solutions, Inc., Boston, MA) and R version 4.2.0 (http://www.r-project.org).
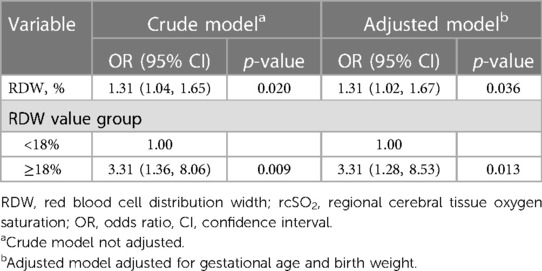
Table 4. Relationship between the RDW and rcSO2 < 55% over the first 14 days after birth in different models.
3. Results
3.1. Baseline characteristics
This study enrolled 110 preterm neonates, of whom 53 and 57 had rcSO2 levels of ≥55% and <55%, respectively. The general characteristics of the study population are summarized in Table 1. There was no noticeable difference between the two groups in terms of GA (31 ± 2 vs. 31 ± 2 weeks; p = 0.273), BW (1524 ± 384 vs. 1432 ± 463 g; p = 0.265), female (47% vs. 40%; p = 0.471), VD (26% vs. 35%; p = 0.325), CA (43% vs. 46%; p = 0.815), hypertension during pregnancy (17% vs. 19%; p = 0.753), GDM (28% vs. 33%; p = 0.568), IUGR (11% vs. 10%; p = 0.894), PROM (36% vs. 37%; p = 0.914), multiple pregnancies (40% vs. 46%; p = 0.526), overall dose of antenatal corticosteroid treatment (75% vs. 72%; p = 0.717), invasive mechanical ventilation (42% vs. 44%; p = 0.803), breast feeding (36% vs. 32%; p = 0.636), blood transfusion (25% vs. 40%; p = 0.077), IVH (13% vs. 19%; p = 0.388), EOS (8% vs. 14%; p = 0.275), or LOS (8% vs. 11%; p = 0.587).
3.2. Association of RDW levels with rcSO2
To investigate the association between RDW values and rcSO2, the participants were divided into two groups according to the RDW value. The piece-wise linear regression threshold effect analysis of RDW and rcSO2 showed that the rcSO2 decreased with RDW values >18% (β, −0.03; 95% CI, −0.04– −0.02; p < 0.0001) (Figure 1, Table 2). Univariate analysis (Table 3) revealed that the RDW was significantly correlated with preterm rcSO2 values <55% over the first 14 days (OR, 1.31; 95% CI, 1.04–1.65; p = 0.020). After multivariable risk adjustment for potential confounding factors (Table 4), including GA and BW in the adjusted model, a high RDW was still positively associated with low rcSO2 in preterm neonates. In addition, an RDW of ≥18% over the first 14 days could significantly increase the risk of preterm rcSO2 values <55% over the first 14 days (adjusted model: OR, 3.31; 95% CI, 1.28–8.53, p = 0.013).
4. Discussion
Despite significant improvements in survival rates in preterm infants in recent years, the rate of major neurodevelopmental impairment in survivors has not decreased (33). As such, the burden of neurodevelopmental disability due to preterm birth remains a significant challenge for neonatologists and a significant burden on public health. As a result, strategies designed to reduce adverse neurological outcomes have been a major focus of perinatal research.
Experiments on piglets have demonstrated that cerebral oxygen saturation (ScO2) values under a certain threshold for prolonged periods are associated with cerebral damage (34). Low rcSO2 values were further associated with lower developmental scores in very preterm infants at 2 years of age, and children surviving with sequelae had lower rcSO2 values than children without sequelae (35). Further, a direct correlation was found between the time spent with an ScO2 of ≤40% and adverse cerebral outcomes or early death (34).
The relationship between SpO2 and rcSO2 has not been well established. SpO2 is not a reliable tool to fully inform about rcSO2 (36, 37); therefore, automated FiO2 control may be difficult to further improve the stability of rcSO2 (38). In adults, current studies have validated that RDW statistically correlates with the National Institutes of Health stroke scale scores and grading in patients with stroke (39). In another study, Söderholm et al. postulated that the incidence of stroke and cerebral infarction increases with RDW level in the general population. In addition, they found evidence of an association between high RDW values and increased intimal-medial thickness in the common carotid artery, which has also been recognized as a risk factor in ischemic stroke (40, 41).
Various factors, including chronic fetal hypoxia in utero, infection, inflammation, physiological immaturity of blood cell production, hemolytic anemia, and erythrocyte transfusions, could lead to an elevated RDW in preterm neonates (4, 8, 9, 30). An increased RDW mirrors a profound deregulation of erythrocyte homeostasis (42). Whether RDW plays an active role in health and disease, or simply acts as a biomarker is of increasingly interest and its clinical use should now be broadened beyond its conventional application for troubleshooting anemia.
A retrospective study of 596 critically ill pediatric patients by Ramby et al. found an association between RDW and prolonged pediatric intensive care unit (PICU) stay in those without sepsis, with a 1.17 increased odds for each 1% increase in RDW (6). An elevated RDW in preterm newborns and infants with IUGR was also significantly associated with early mortality (6), as well as worse clinical parameters, including PICU mortality. Thus, RDW could be a promising prognostic factor with the advantages of simple and easy measurement in critically ill pediatric patients (43).
Our data suggest that RDW over the first 14 days could be a useful indicator. The influence of some confounding factors, such as GA, BW, and nursing factors, were excluded by multivariate logistic regression models. Our study identified a statistically significant positive correlation between the RDW and preterm rcSO2. An RDW of ≥18% over the first 14 days could significantly increase the risk of a preterm rcSO2 of <55%. This value may be applied in clinical practice and could be used during routine monitoring processes for preterm rcSO2 in the NICU.
Several high-risk factors that may increase RDW should be avoided in the early stages to reduce cerebral hypoxia injury in preterm infants. For example, identifying the strict indications of erythrocyte transfusions, infection prevention and control, and avoiding fetal hypoxia in utero are all important factors. Overall, our findings emphasize the necessity of improving medical measures to decrease the incidence of cerebral hypoxia in preterm neonates.
This study has several limitations. First, the sample size was small, and all patients were enrolled from a single center, which may have restricted the accuracy and generalizability of the results. Moreover, we did not collect SpO2 values during the measurement period, and the exact relationship between SpO2 and rcSO2 therefore could not be elucidated. Further research is needed to explore this factor.
5. Conclusion
Overall, the results of the present study showed that an RDW of ≥18% in the first 14 days is associated with rcSO2 of <55% in preterm infants. RDW is an easy, simple, inexpensive, and rapid tool that could be used to ensure the early prediction of preterm infants with low rcSO2, which is correlated with cerebral hypoxia.
Data availability statement
The raw data supporting the conclusions of this article will be made available by the authors, without undue reservation.
Ethics statement
The studies involving human participants were reviewed and approved by the Ethics Committee of the West China Second University Hospital, Sichuan University (2022-67). Written informed consent to participate in this study was provided by the patients/participants’ legal guardian/next of kin.
Author contributions
HW conceptualized and designed the work, provided feedback on the study design, helped draft the initial manuscript, assisted in the creation of the tables and figures, and critically reviewed and revised the manuscript. YM performed the background research and data gathering, assisted in the study methodology, performed the statistical analyses, developed the figures and tables, and reviewed and revised the article. DM helped review and revise the manuscript. MT, YH, YF, RL, QH, SJ, JH, and SD assisted in data gathering and sorting. All authors contributed to the article and approved the submitted version.
Funding
This work was supported by the Critical Care Project of National Natural Science Foundation of China (H16 82241036) and the Department of Science and Technology of Sichuan Province (2021YJ0171).
Acknowledgments
The authors would like to thank Sichuan University West China Second University Hospital for their assistance with data collection.
Conflict of interest
The authors declare that the research was conducted in the absence of any commercial or financial relationships that could be construed as a potential conflict of interest.
Publisher's note
All claims expressed in this article are solely those of the authors and do not necessarily represent those of their affiliated organizations, or those of the publisher, the editors and the reviewers. Any product that may be evaluated in this article, or claim that may be made by its manufacturer, is not guaranteed or endorsed by the publisher.
References
1. Osborn DA, Evans N, Kluckow M. Hemodynamic and antecedent risk factors of early and late periventricular/intraventricular hemorrhage in premature infants. Pediatrics (2003) 112(1 Pt 1):33–9. doi: 10.1542/peds.112.1.33
2. Kurinczuk JJ, White-Koning M, Badawi N. Epidemiology of neonatal encephalopathy and hypoxic-ischaemic encephalopathy. Early Hum Dev. (2010) 86(6):329–38. doi: 10.1016/j.earlhumdev.2010.05.010
3. Zhao P, Ji G, Xue H, Yu W, Zhao X, Ding M, et al. Isoflurane postconditioning improved long-term neurological outcome possibly via inhibiting the mitochondrial permeability transition pore in neonatal rats after brain hypoxia-ischemia. Neuroscience. (2014) 280:193–203. doi: 10.1016/j.neuroscience.2014.09.006
4. Güler Kazancı E, Üstündağ Y, Akdoğan M, Yıldırım F, Arıkan EY, Huysal K. Red cell distribution width to platelet count ratio reference intervals in premature infants beyond the first week of life. Fetal Pediatr Pathol. (2023) 42(4):581–8. doi: 10.1080/15513815.2023.2178268
5. Danese E, Lippi G, Montagnana M. Red blood cell distribution width and cardiovascular diseases. J Thorac Dis. (2015) 7(10):E402–11. doi: 10.3978/j.issn.2072-1439.2015.10.04
6. Silva Litao MK, Kamat D. Back to basics: red blood cell distribution width: clinical use beyond hematology. Pediatr Rev. (2018) 39(4):204–9. doi: 10.1542/pir.2017-0118
7. Zierk J, Hirschmann J, Toddenroth D, Arzideh F, Haeckel R, Bertram A, et al. Next-generation reference intervals for pediatric hematology. Clin Chem Lab Med. (2019) 57(10):1595–607. doi: 10.1515/cclm-2018-1236
8. Liu Z-Y, Jiang H-Z, Wang L, Chen M-X, Wang H-T, Zhang J-X. Diagnostic accuracy of red blood cell distribution width for neonatal sepsis. Minerva Pediatr. (2022) 74(2):202–12. doi: 10.23736/S2724-5276.21.06149-1
9. Kim SY, Lee SM, Sung SJ, Han SJ, Kim BJ, Park C-W, et al. Red cell distribution width as a potential prognostic biomarker in fetal growth restriction. J Matern-Fetal Neonatal Med. (2019) 34(6):883–8. doi: 10.1080/14767058.2019.1622665
10. Go H, Ohto H, Nollet KE, Sato K, Ichikawa H, Kume Y, et al. Red cell distribution width as a predictor for bronchopulmonary dysplasia in premature infants. Sci Rep. (2021) 11(1):7221. doi: 10.1038/s41598-021-86752-8
12. Said AS, Spinella PC, Hartman ME, Steffen KM, Jackups R, Holubkov R, et al. RBC distribution width: biomarker for red cell dysfunction and critical illness outcome?. Pediatr Crit Care Med. (2017) 18(2):134–42. doi: 10.1097/PCC.0000000000001017
13. Verhagen EA, Van Braeckel KN, van der Veere CN, Groen H, Dijk PH, Hulzebos CV, et al. Cerebral oxygenation is associated with neurodevelopmental outcome of preterm children at age 2 to 3 years. Dev Med Child Neurol. (2015) 57(5):449–55. doi: 10.1111/dmcn.12622
14. Pichler G, Binder C, Avian A, Beckenbach E, Schmölzer GM, Urlesberger B. Reference ranges for regional cerebral tissue oxygen saturation and fractional oxygen extraction in neonates during immediate transition after birth. J Pediatr. (2013) 163(6):1558–63. doi: 10.1016/j.jpeds.2013.07.007
15. Watanabe T, Ito M, Miyake F, Ogawa R, Tamura M, Namba F. Measurement of brain tissue oxygen saturation in term infants using a new portable near-infrared spectroscopy device. Pediatr Int. (2017) 59(2):167–70. doi: 10.1111/ped.13099
16. Chock VY, Smith E, Tan S, Ball MB, Das A, Hintz SR, et al. Early brain and abdominal oxygenation in extremely low birth weight infants. Pediatr Res. (2022) 92(4):1034–41. doi: 10.1038/s41390-022-02082-z
17. Alderliesten T, Dix L, Baerts W, Caicedo A, van Huffel S, Naulaers G, et al. Reference values of regional cerebral oxygen saturation during the first 3 days of life in preterm neonates. Pediatr Res. (2015) 79(1):55–64. doi: 10.1038/pr.2015.186
18. Kato R, Hosono S, Takahashi S. Reference value of brain tissue oxygen saturation in newborns immediately after birth. Adv Exp Med Biol. (2020) 12(32):19–24. doi: 10.1007/978-3-030-34461-0_3
19. Pichler G, Goeral K, Hammerl M, Perme T, Dempsey EM, Springer L, et al. Cerebral regional tissue oxygen saturation to guide oxygen delivery in preterm neonates during immediate transition after birth (COSGOD III): multicentre randomised phase 3 clinical trial. Br Med J. (2023) 381:1102. doi: 10.1136/bmj-2022-072313
20. Ozawa J, Watanabe T, Ito M, Miyake F, Nagano N, Ogawa R, et al. Defining the reference range of regional cerebral tissue oxygen saturation using a new portable near-infrared spectroscopy device for term infants. Early Human Dev. (2020) 141. doi: 10.1016/j.earlhumdev.2019.104941
21. Baik N, Urlesberger B, Schwaberger B, Schmölzer GM, Avian A, Pichler G. Cerebral haemorrhage in preterm neonates: does cerebral regional oxygen saturation during the immediate transition matter? Arch Dis Child Fetal Neonatal Ed. (2015) 100(5):422–7. doi: 10.1136/archdischild-2014-307590
22. Wolfsberger CH, Pichler-Stachl E, Höller N, Mileder LP, Schwaberger B, Avian A, et al. Cerebral oxygenation immediately after birth and long-term outcome in preterm neonates-a retrospective analysis. BMC Pediatr. (2023) 23(1):145. doi: 10.1186/s12887-023-03960-z
23. Hansen ML, Pellicer A, Hyttel-Sørensen S, Ergenekon E, Szczapa T, Hagmann C, et al. Cerebral oximetry monitoring in extremely preterm infants. N Engl J Med. (2023) 388(16):1501–11. doi: 10.1056/NEJMoa2207554
24. Pellicer A, Greisen G, Benders M, Claris O, Dempsey E, Fumagally M, et al. The SafeBoosC phase II randomised clinical trial: a treatment guideline for targeted near-infrared-derived cerebral tissue oxygenation versus standard treatment in extremely preterm infants. Neonatology. (2013) 104(3):171–8. doi: 10.1159/000351346
25. Plomgaard AM, van Oeveren W, Petersen TH, Alderliesten T, Austin T, Van Bel F, et al. The SafeBoosC II randomized trial: treatment guided by near-infrared spectroscopy reduces cerebral hypoxia without changing early biomarkers of brain injury. Pediatr Res. (2016) 79(4):528–35. doi: 10.1038/pr.2015.266
26. Petrova A, Mehta R. Near-infrared spectroscopy in the detection of regional tissue oxygenation during hypoxic events in preterm infants undergoing critical care. Pediatr Crit Care Med. (2006) 7(5):449–54. doi: 10.1097/01.PCC.0000235248.70482.14
27. Tomaiuolo G, Lanotte L, D’Apolito R, Cassinese A, Guido S. Microconfined flow behavior of red blood cells. Med Eng Phys. (2016) 38(1):11–6. doi: 10.1016/j.medengphy.2015.05.007
28. Patel KV, Mohanty JG, Kanapuru B, Hesdorffer C, Ershler WB, Rifkind JM. Association of the red cell distribution width with red blood cell deformability. Adv Exp Med Biol. (2013) 765:211–6. doi: 10.1007/978-1-4614-4989-8_29
29. Reinhart WH, Piety NZ, Goede JS, Shevkoplyas SS. Effect of osmolality on erythrocyte rheology and perfusion of an artificial microvascular network. Microvasc Res. (2015) 98:102–7. doi: 10.1016/j.mvr.2015.01.010
30. Christensen RD, Yaish HM, Henry E, Bennett ST. Red blood cell distribution width: reference intervals for neonates. J Matern-Fetal Neonatal Med. (2014) 28(8):883–8. doi: 10.3109/14767058.2014.938044
31. Chang L, Chen X, Lian C. The association between the non-HDL-cholesterol to HDL-cholesterol ratio and 28-day mortality in sepsis patients: a cohort study. Sci Rep. (2022) 12(1):3476. doi: 10.1038/s41598-022-07459-y
32. Lin L, Chen CZ, Yu XD. The analysis of threshold effect using empower stats software. Zhonghua Liu Xing Bing Xue Za Zhi. (2013) 34(11):1139–41.24517951
33. Fanaroff AA, Stoll BJ, Wright LL, Carlo WA, Ehrenkranz RA, Stark AR, et al. Trends in neonatal morbidity and mortality for very low birthweight infants. Am J Obstet Gynecol. (2007) 196(2):147.e1–8. doi: 10.1016/j.ajog.2006.09.014
34. Kurth CD, Levy WJ, McCann J. Near-infrared spectroscopy cerebral oxygen saturation thresholds for hypoxia-ischemia in piglets. J Cereb Blood Flow Metab. (2002) 22(3):335–41. doi: 10.1097/00004647-200203000-00011
35. Cerbo RM, Orcesi S, Scudeller L, Borellini M, Croci C, Ravelli C, et al. Near-infrared spectroscopy monitoring, superior vena cava flow, and neurodevelopmental outcome at 2 years in a cohort of very low-birth-weight infants. Am J Perinatol. (2016) 33(11):1093–8. doi: 10.1055/s-0036-1586103
36. Janvier A, Khairy M, Kokkotis A, Cormier C, Messmer D, Barrington KJ. Apnea is associated with neurodevelopmental impairment in very low birth weight infants. J Perinatol. (2004) 24(12):763–8. doi: 10.1038/sj.jp.7211182
37. Martin RJ, Wang K, Köroğlu O, Di Fiore J, Kc P. Intermittent hypoxic episodes in preterm infants: do they matter. Neonatology. (2011) 100(3):303–10. doi: 10.1159/000329922
38. Dani C, Pratesi S, Luzzati M, Petrolini C, Montano S, Remaschi G, et al. Cerebral and splanchnic oxygenation during automated control of inspired oxygen (FiO2) in preterm infants. Pediatr Pulmonol. (2021) 56(7):2067–72. doi: 10.1002/ppul.25379
39. Kara H, Degirmenci S, Bayir A, Ak A, Akinci M, Dogru A, et al. Red cell distribution width and neurological scoring systems in acute stroke patients. Neuropsychiatr Dis Treat. (2015) 11:733–9. doi: 10.2147/NDT.S81525
40. Söderholm M, Borné Y, Hedblad B, Persson M, Engström G. Red cell distribution width in relation to incidence of stroke and carotid atherosclerosis: a population-based cohort study. PLoS One. (2015) 10(5):e0124957. doi: 10.1371/journal.pone.0124957
41. Rosvall M, Janzon L, Berglund G, Engström G, Hedblad B. Incidence of stroke is related to carotid IMT even in the absence of plaque. Atherosclerosis. (2005) 179(2):325–31. doi: 10.1016/j.atherosclerosis.2004.10.015
42. Salvagno GL, Sanchis-Gomar F, Picanza A, Lippi G. Red blood cell distribution width: a simple parameter with multiple clinical applications. Crit Rev Clin Lab Sci. (2015) 52(2):86–105. doi: 10.3109/10408363.2014.992064
Keywords: red blood cell distribution width, regional cerebral tissue oxygen saturation, cerebral hypoxia, near-infrared spectroscopy, preterm neonates
Citation: Mu Y, Wang H, Tian M, Hu Y, Feng Y, Lu R, He Q, Jiang S, Huang J, Duan S and Mu D (2023) Cross-sectional association between red blood cell distribution width and regional cerebral tissue oxygen saturation in preterm infants in the first 14 days after birth. Front. Pediatr. 11:1238762. doi: 10.3389/fped.2023.1238762
Received: 14 June 2023; Accepted: 16 October 2023;
Published: 31 October 2023.
Edited by:
Bernhard Schwaberger, Medical University of Graz, AustriaReviewed by:
Ena Suppan, Medical University of Graz, AustriaMaria Fernanda Blasina Viera, Universidad de la República, Uruguay
© 2023 Mu, Wang, Tian, Hu, Feng, Lu, He, Jiang, Huang, Duan and Mu. This is an open-access article distributed under the terms of the Creative Commons Attribution License (CC BY). The use, distribution or reproduction in other forums is permitted, provided the original author(s) and the copyright owner(s) are credited and that the original publication in this journal is cited, in accordance with accepted academic practice. No use, distribution or reproduction is permitted which does not comply with these terms.
*Correspondence: Hua Wang d2FuZ2h1YUBzY3UuZWR1LmNu