- 1Pediatric Rheumatology Unit, Department of Woman and Child Health, University of Padova, Padova, Italy
- 2Pediatric Diabetes and Pediatric Metabolism Unit, Department of Woman and Child Health, University of Padova, Padova, Italy
Background: Euthyroid sick syndrome (ESS) is characterized by low serum levels of free triiodothyronine (fT3) with normal or low levels of thyroid stimulating hormone (TSH) and free thyroxine (fT4) and is reported in different acute clinical situations, such as sepsis, diabetic ketoacidosis and after cardiac surgery. Our aim was to evaluate the predicting role of ESS for disease severity in patients with Multisystem Inflammatory Syndrome in children (MIS-C).
Methods: A single-centre observational study on consecutive patients with MIS-C. Before treatment clinical, and laboratory data were collected and, in a subset of patients, thyroid function tests were repeated 4 weeks later. Variables distribution was analyzed by Mann-Whitney U-test and correlations between different parameters were calculated by Spearman's Rho coefficient.
Results: Forty-two patients were included and 36 (85.7%) presented ESS. fT3 values were significantly lower in patients requiring intensive care, a strong direct correlation was shown between fT3 and Hb, platelet count and ejection fraction values. A significant inverse correlation was retrieved between fT3 levels and C-reactive protein, brain natriuretic peptide, IL-2 soluble receptor and S-100 protein. Subjects with severe myocardial depression (EF < 45%) had lower fT3 values than subjects with higher EF. The thyroid function tests spontaneously normalized in all subjects who repeated measurement 4 weeks after admission.
Conclusion: ESS is a frequent and transient condition in acute phase of MIS-C. A severe reduction of fT3 must be considered as important prognostic factor for severe disease course, with subsequent relevant clinical impact in the management of these patients.
Introduction
From May 2020, after the first reported cases of “Kawasaki-like disease” (1), a novel multisystem inflammatory syndrome in children (MIS-C) related to COVID19 infection was identified. It was defined by signs of systemic inflammation (fever, elevated inflammatory markers) and at least two organ dysfunctions in an individual aged <21 years, with current infection or exposed within 4 weeks to SARS-CoV2 (2). The clinical manifestations of MIS-C are very heterogeneous but cardiovascular and gastrointestinal systems appear to be the most affected (3). Few studies have explored possible manifestations affecting the endocrine system during this iperinflammatory disease related to SARS-CoV2.
Recently, an alteration of the thyroid axis known as euthyroid sick syndrome (ESS) has been reported in patients with MIS-C (4). This condition, described in children with severe clinical situations such as sepsis (5), diabetic ketoacidosis (6) or after cardiac surgery (7), is characterized by decreased levels of triiodothyronine (fT3), increased conversion of thyroxine (fT4) to the biologically inactive form of reverse T3 (rT3) without a compensatory rise in of thyroid-stimulating hormone (TSH) (8). The result is an overall reduction in the bioavailability of active fT3, thus miming central hypothyroidism (9).
ESS appears as an adaptive mechanism to reduce the energy expenditure of the organism. For this reason, there is no agreement to correct this condition although the severity of ESS is associated with poor outcome in some studies (10).
In this study, our aim was to collect and analyze the thyroid profile together with other laboratory and instrumental data in order to evaluate the possible prognostic role of ESS within a cohort of patients affected by MIS-C.
Patients and methods
Patients
A single-center observational study was conducted at the Pediatric Rheumatology Unit of the Department of Woman and Child Health of Padova between 30 November 2020 and 30 September 2022. Consecutive patients admitted for MIS-C, according to classification criteria proposed by the Center for Disease Control and Prevention (CDC), were included (3). Children with known thyroid, hypothalamic and pituitary disease or undergoing medical therapy with glucocorticoids or inotropes in the 48 h prior to admission were excluded. This study was approved by the Ethical Committees of Padova University Hospital (n. 338n/AO/23). Due to the nature of the study (observational cross-sectional), no informed consent was required from the patients and their caregivers.
Clinical data collection
Laboratory tests were performed for each patient upon admission and before any treatment. More precisely, collected data were: complete blood count (CBC), C-Reactive Protein (CRP), Erythrosedimentation Rate (ESR), ferritin, cytokines and biomarkers profile (interleukins IL-1α, IL-1β, IL-6, soluble IL2 receptor, IL2r, tumor necrosis factor α, TNF α, and S-100 protein), organ involvement indices such as glutamic-pyruvic transaminase (GPT), glutamic-oxaloacetic transaminase (GOT), troponin (TnI), brain natriuretic peptide (BNP), thyroid hormones (TSH, fT3, fT4).
Thyroid function tests were considered normal according to the range values of the hospital laboratory: TSH 0.3–5 mU/L; fT3 3.9–6.8 pmol/L; fT4 8–20 pmol/L.
A complete cardiological evaluation including Electrocardiogram (EKG) and echocardiography was performed upon admission. In a group of patients, a second dosage of TSH, fT3 and fT4 levels was performed 4 weeks after the onset of the disease.
Statistical analysis
The continuous variables were collected by calculating the main indicators of centrality and variability. The analysis of the differences in demographic and clinical features and in laboratory values between groups of subjects defined according to variables of interest was made by applying the non-parametric Mann-Whitney U-test, after verifying the non normality of the distributions of the variables under examination.
Fisher's exact test or the χ2 test were used for group comparison (ESS group vs. no ESS group). Spearman's Rho correlation coefficient was calculated to evaluate the correlation between the different laboratory values. A p-value less than 0.05 (two-tailed test) was considered statistically significant.
Post-hoc power analysis was calculated on the fT3 values variable (two-tailed hypothesis) given the observed probability level (0.05), the observed effect size (Hedges'g = 2.4202) and the total sample size. Hedges’g was chosen because more appropriate where very different sample sizes are considered, as in the present study. All analyses was performed using IBM SPSS statistical software. (Vers. 20.0).
Results
Demographic, clinical and laboratory features of MIS-C patients at onset
In the study we included 42 consecutive children with MIS-C (29 male—69%) with median age 9 years (range 0.7–17 years). As showed in Table 1 the most represented ethnicity was Caucasian (88%), although children of African descent appeared more often involved (9.5%) compared to other ethnic origin. Only 4 subjects had comorbidities [1 periodic fever with periodic fever, aphtous stomatitis, pharyngitis, adenitis (PFAPA syndrome), 2 glucose 6-phosphate dehydrogenase deficiency, 1 autism spectrum disorder]. According to case definition of MIS-C, all patients were exposed to SARS-CoV2. Thirty patients (71%) reported previous confirmed infection with a median time of 4 weeks (range 2–6 weeks).
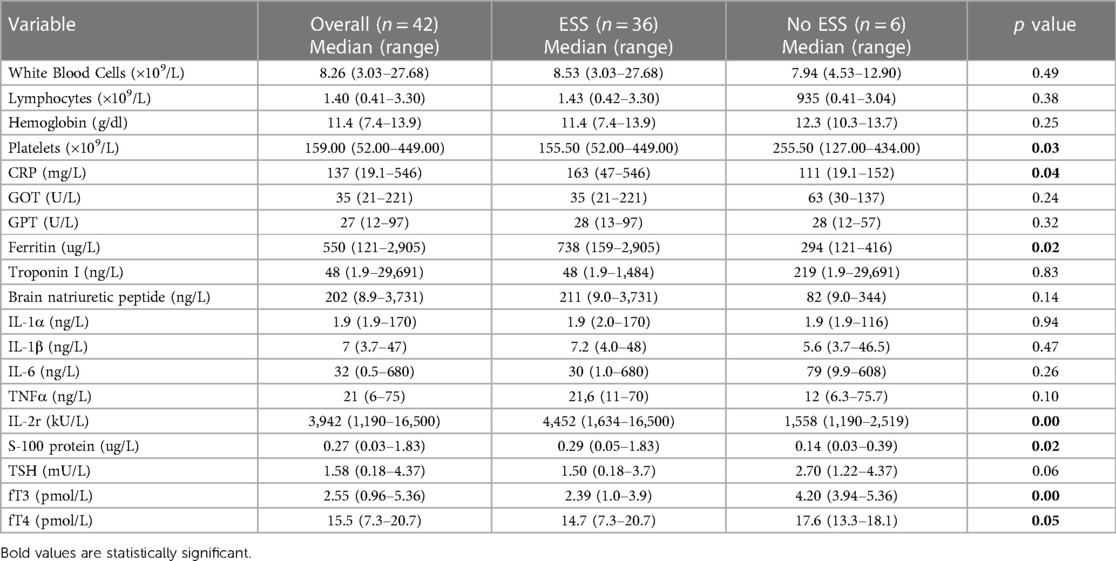
Table 1. Laboratory tests of whole MIS-C group and comparison between patients with ESS and patients with normal thyroid function tests (no ESS group) at the onset of disease.
Forty-one patients (98%) were treated with immunosuppressive therapy using high-dose immunoglobulin (2 g/kg) plus corticosteroid (methylprednisolone 2 mg/kg). Only one subject was treated only with corticosteroid monotherapy. Due to the severity of the disease onset, six subjects (14%) required treatment with interleukin 1 antagonist (Anakinra). The median time between onset of symptoms and initiation of therapy was 6 days (range 2–9 days).
In Supplementary Table S1, clinical manifestation at onset are reported. Gastrointestinal tract involvement was the most frequent, affecting 39 patients (93%): reported symptoms were mainly abdominal pain (67%), vomiting (45%) or diarrhea (40%). The involvement of cardiovascular system was observed in 34 patients (81%) presenting with severe arterial hypotension in 14 patients (33%), increased myocardial enzymes in 23 patients (54%), decreased cardiac contractility (Ejection fraction EF < 55%) in 16 (38%), or coronary artery abnormalities (coronary ectasias/aneurysms, coronaritis) in 7 (16%). Sixty-seven percent of individuals presented skin and mucosal involvement. Renal and Central Nervous System (CNS) involvement appeared less frequent, involving only 8 (19%). and 4 children (9%), respectively.
No statistically significant difference was found in clinical features between the ESS and no ESS group (Supplementary Table S1).
As shown in Table 1, on admission, 36 children (86%) presented ESS characterized by low fT3 levels (median 2.54 pmol/L, range 0.96–5.36): only one subject presented simultaneously low fT4 levels and another had reduced TSH levels. The post-hoc power analysis showed a very high value (0.9999) although the samples size (ESS group and no-ESS group) were very different.
Other relevant laboratory tests showed increased acute phase reactants such as CRP median 137 mg/L (range 19.1–546) and ferritin median 550 ug/L (range 121–2,905) associated with widely variable range of myocardial enzymes alterations as troponin median 48 ng/L (range 1.9–29,691) and BNP median 202 ng/L (range 8.9–3,731).
At disease onset patients in the ESS group showed a higher inflammatory profile compared with euthyroid subjects. In fact, as showed in Table 1, a statistically significant elevation of the main inflammatory markers such as CRP (p = 0.03), ferritin (p = 0.02), IL-2r (p = 0.003), and S-100 protein (p = 0.02) was observed in ESS patients compared with euthyroid ones. The difference in terms of platelet count (p = 0.03) also appeared significant.
In 16 subjects (2 of them admitted in ICU) the measurement of thyroid function tests was repeated 4 weeks after admission and showed normalization in all (TSH mean 2.57 mU/L, range 0.96–5.36 mU/L, fT3 mean 6,07 pmol/L range 5,2–6,7 pmol/L, fT4 mean 19,2 pmol/L range 15,1–20,1 pmol/L.
ESS and risk of intensive care support
Among MIS-C patients, 6/42 (14%) required admission to intensive care unit (ICU): these subjects documented much lower fT3 levels compared to other patients (median value 1.45 pmol/L vs. 2.76 pmol/L, p = 0.013), while no significant difference was observed for fT4 and TSH levels (Figure 1). Indeed, the subjects admitted to ICU, presented significantly higher inflammatory markers and white blood cells count: respectively WBC (median value 11,355/mmq vs. 8,060/mmq, p = 0.019), CRP (median value 229 mg/L vs. 143 mg/L, p = 0.008), ferritin (median value 1,383 ug/L vs. 467 ug/L, p = 0.034), BNP (median value 1,363 ng/L vs. 187 ng/L p = 0.004) and S100 protein (median value 1.08 ug/L vs. 0.24 ug/L, p = 0.002).
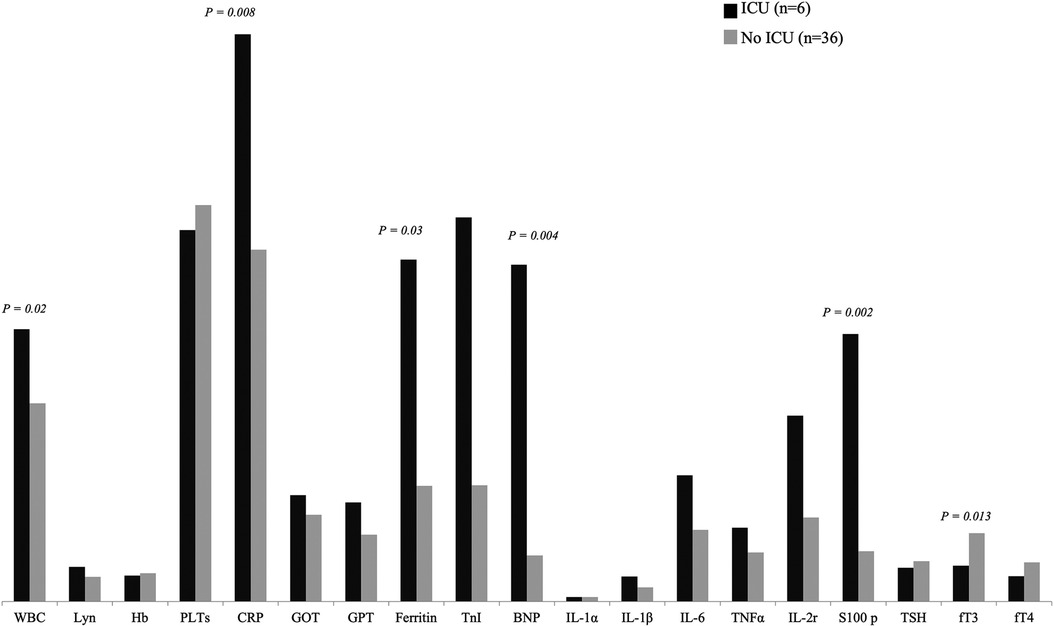
Figure 1. Comparison of laboratory features between patients requiring admission to ICU and patients admitted to general hospital ward.
ESS and risk of severe cardiac involvement
Severe depression of myocardial function, defined by EF ≤ 45%, was found in 5 patients (12%) of MIS-C cohort. This subgroup presented significantly lower fT3 values (median 1.55 pmol/L vs. 2.73 pmol/L; p = 0.003) and fT4 (median 9.64 pmol/L vs. 16.02 pmol/L; p = 0.009) if compared with EF > 45% subgroup, as showed in Figure 2. Conversely, no clear association between fT3 values and presence of coronary dilatation at the onset of the disease has been detected.
Prognostic role of ESS for severe clinical course
As shown in Figure 3A,B, statistical analysis demonstrated a direct correlation between ESS severity, expressed by fT3 values, and hemoglobin levels [correlation coefficient (ρ) 0.39, p = 0.01] or platelet count (ρ 0.44, p = 0.003). An inverse correlation was documented between fT3 levels and markers of systemic inflammation such as CRP (ρ 0.390, p = 0.009), soluble IL2 receptor (ρ 0.59, p = 0.001) and S-100 protein (ρ 0.39, p = 0.002) (Figure 3C,E,F). Moreover, a statistically significant inverse correlation was observed between triiodothyronine and BNP (ρ 0.49, p = 0.002) (panel D, Figure 3). About instrumental data, there was a direct correlation between fT3 levels and EF values on admission (ρ 0.36, p = 0.017), as shown in panel F of Figure 3. No statistically significant correlation between fT3 values and fever duration at the time of initiation of therapy, WBC (ρ −0.26, p = 0.09), lymphocytes count (ρ −0.16, p = 0.33), GPT (ρ −0.16, p = 0.3), GOT (ρ 0.10, p = 0.5), ferritin (ρ −0.15, p = 0.39), IL-1α (ρ −0.18, p = 0.26), IL-1β (ρ −0.26, p = 0.11), IL-6 (ρ 0.12, p = 0.45), TNF (ρ −0.33, p = 0.055), TnI levels (ρ −0.03, p = 0.82).
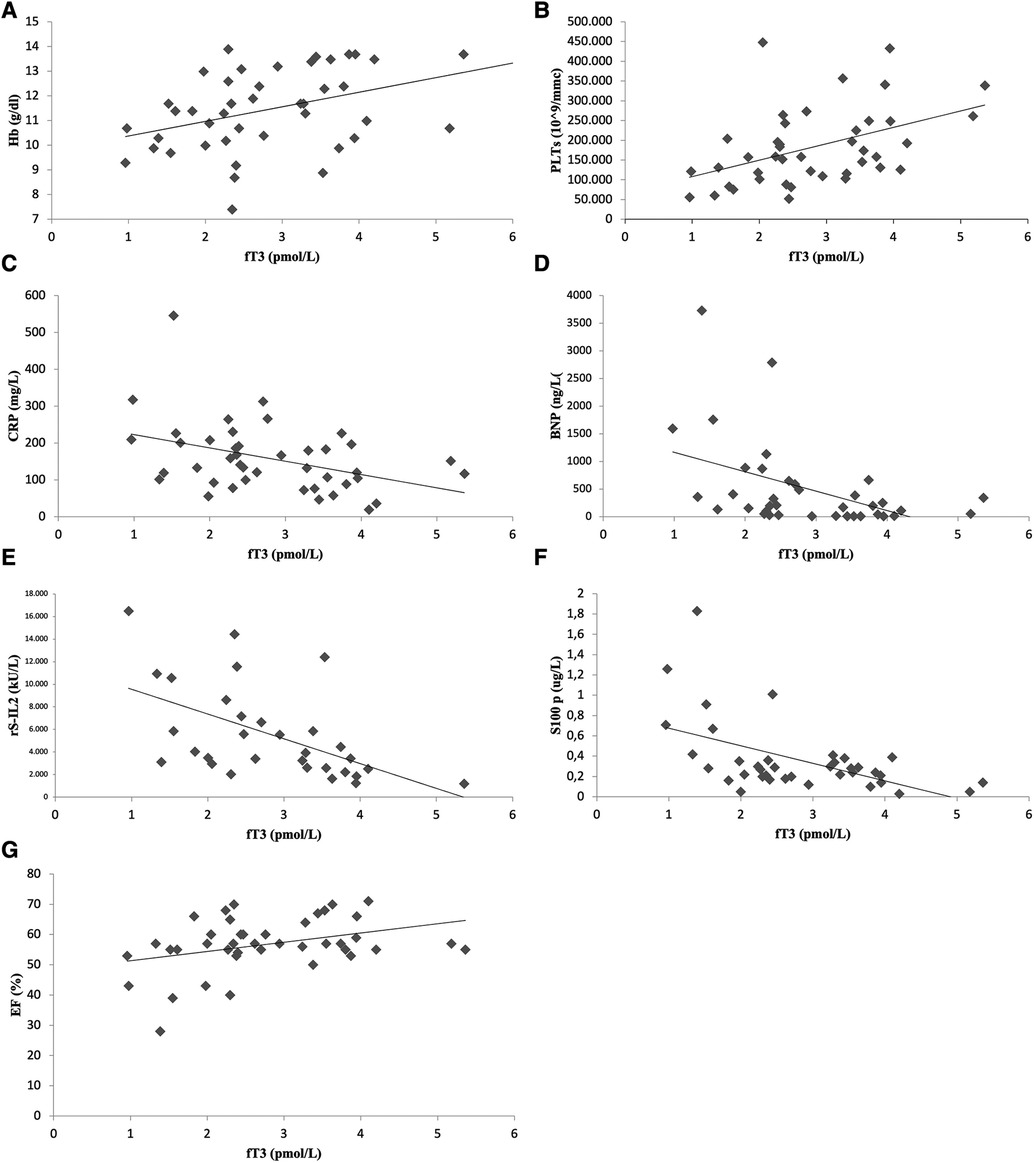
Figure 3. Significant correlation between laboratory-instrumental values and fT3 level: scatterplots show correlation between fT3 and hemoglobin (A), platelets (B), C-reactive protein (C), brain natriuretic peptide (D), soluble IL-2 receptor (E), S -100 protein (F) and ejection fraction (G).
Discussion
During the COVID19 pandemic, thyroid function alterations have been reported in patients not previously diagnosed with any thyroid conditions. The mechanisms underlying COVID-19-related dysfunction of endocrine glands consist of inflammation, vessel damage, necrosis, degeneration, immune and autoimmune processes (11–14). For the abnormalities of the hypothalamus-pituitary-thyroid axis associated with COVID19 several mechanisms have been suggested such as a disturbance in the TSH process via virus-related hypophysitis, or a subacute thyroiditis linked to the virus spread (11, 15). Subacute thyroiditis during COVID19 presents with thyroid hormone flare-up, usually is self-limited and does not require specific treatment (16, 17). Two major pathophysiological models have been implicated: a direct infection of thyroid cells by SARS-CoV-2 as they express Angiotensin-converting enzyme (ACE) 2 or an indirect effect caused by an immune-inflammatory abnormal response to the virus, especially at the moment of cytokine storm, in addition to multiple organ failure (18–21).
In patients with COVID19 other thyroid function parameters alterations, which are commonly referred as euthyroid sick syndrome (ESS) have been reported (22). ESS has been recognized since the 1970s when it was observed that acute illnesses and fasting may affect circulating levels of thyroid hormones in subjects without previously diagnosed thyroid disease. Most typically, in ESS plasma concentrations of fT3 decrease and those of rT3, the biologically inactive form of fT4, rise (23, 24). This suggests an inactivation of thyroid hormone in peripheral tissues likely mediated by activation of type 3 deiodinase (D3) or by suppressed activity of type 1 deiodinase (D1) (25, 26). As the severity and the length of ESS increases, also the fT4 levels can be deeply reduced (27).
ESS has been reported both in adult and pediatric populations in many severe clinical conditions such as sepsis, trauma, acute myocardial infarction, severe malnutrition, liver failure, cardiac surgery, and diabetic ketoacidosis (5–9). Zou et al. studied 149 COVID19 patients and found that those with ESS (27.5%) had more severe inflammatory responses, such as higher levels of C-reactive protein and erythrocyte sedimentation rate compared to those without ESS (22). In a cohort of subjects with acute COVID19 infection, the presence of ESS correlated with a more severe course of the disease, with higher inflammatory parameters level and risk of myocardial dysfunction compared with normal thyroid profile (28).
In pediatric age, beside a low rate of hospitalization and need for intensive support associated with direct SARS-CoV2 infection, a great burden of morbidity was observed because of the novel multisystem inflammatory syndrome in children (MIS-C) related to COVID19 (1, 2).
MIS-C is an immune-mediated disease that can manifest with multiple organ failure and even lead to shock. The etiology of this syndrome is not yet fully understood: host genetic factors associated to alterations of innate and adaptive immune system as well as mechanisms of molecular mimicry (29) can develop MIS-C as a result of a cytokine storm after the infection (30, 31).
The clinical features and the involvement of internal organs in MIS-C have been extensively studied while only few data are available on dysfunction of endocrine systems and its possible clinical relevance. Calcaterra et al. documented that 23/26 patients (88%) with MIS-C presented ESS at onset predominantly characterized by low fT3 values (65%) as compared to alterations of other thyroid hormones (4). Similarly, in a case-control study, lower fT3 levels were found in patients with MIS-C compared with healthy subjects with opposite results for fT4 levels (32).
In the present study, we assessed the thyroid function in patients with MIS-C and analyzed the potential value of ESS in predicting disease severity. In our series, similarly to previous studies, ESS was present in the majority of patients (86%) with an hormonal profile mainly characterized by reduced fT3 values. In our cohort we did not find statistically significant differences in clinical features between patients with ESS and those with normal thyroid levels, probably due to the small number of patients. Nevertheless, it is worth to note that signs of more severe course such as hypotension, coronary abnormalities, CNS and renal involvement were more frequent in patients with ESS, and this was confirmed by the observation that children requiring admission in intensive care unit were all from the ESS group.
Furthermore, our patients with ESS showed markedly more elevated inflammatory markers such as CRP, ferritin, rsIL-2 and S-100 protein and we observed a strong correlation between fT3 levels and different inflammatory markers while they did not correlate with the duration of the disease. These results suggest that the hyperinflammatory state could be principal responsible for the hormone dysfunction and related to its severity.
This evidence suggests ESS as a useful adaptation of the body to counteract excessive catabolism during illness and as a part of the acute phase response mediated by cytokines (33). In fact, proinflammatory cytokines, especially IL-1, IL-6, TNF-α, and interferon-γ, inhibit several genes involved in thyroid hormone metabolism in vitro (34, 35). The administration of cytokines in experimental models resulted in altered thyroid hormone metabolism exhibiting some, but not all, features of disease-related ESS. A possible causal role for IL-6 in the development of ESS was suggested since IL-6 knock out mice showed a less pronounced drop in serum T3 during illness (36). However, acute injection of cytokines induced a flu-like illness but failed to induce ESS like features, except for interferon gamma (IFN-γ) which reduced serum T3 and T4, therefore suggesting that the resulting illness, rather than the cytokines alone, accounted for the changes in thyroid hormones metabolism (37). Other studies showed that several components of the thyroid hormones synthesis pathway can be downregulated by cytokines directly on the level of the thyrocyte, ultimately leading to decreased secretion of T4 and T3 (38). Moreover, studies in vitro and in animal models showed that IL-1α and IL-1b may display multiple effect such as inhibition of the thyroglobulin (Tg) mRNA expression induced by TSH and subsequent Tg release in human cultured thyrocytes and decrease of 125I incorporation and T4 and T3 secretion from human thyrocytes (34, 39, 40). TNFα plays an important role in the acute phase response and in vitro studies showed that it inhibits the TSH-induced cAMP response and thyroglobulin production and release in cultured thyrocytes (39, 41, 42).
Some evidence shows that pro-inflammatory cytokines may contribute to development of ESS also by affecting the expression and the activity levels of the deiodinases. In particular, during inflammation, competition for co-factors by cytokine-induced pathways such as nuclear factor-kappa B (NF-kB) are associated with less transcription of the D1 gene in the liver and with increase in D2 expression in the hypothalamus (43–45).
Although with the limitation of a small number of patients our data suggest that both adaptation and cytokines hyperproduction probably act together to development of ESS which entity, in our cohort, directly correlate with severity of clinical picture. Moreover, our data suggest that repeated dosages of fT3 levels may be useful to monitor the disease course.
In agreement with the role of ESS as useful and transient mechanism of adaptation during severe diseases, such as MIS-C, to reduce catabolic processes, in our cohort the levels of thyroid hormones spontaneously turned to normal during the first month from disease onset.
In our study, among the 36 subjects with ESS, only two had alterations of TSH or fT4 levels: these data differ from those reported in literature where the rate of ESS with an isolated reduction of fT3 was observed in only 65% of patients (4). In our patients diagnosis and treatment were started quite early after disease onset, therefore this could suggest a greater peripheral block of conversion of fT3 than of fT4 and a lack of central inhibition of TSH in our cohort.
In our study patients who needed admission to ICU presented lower levels of fT3 and moreover we showed a strong direct correlation between fT3 levels and severity of myocardial depression on admission. In fact we retrieved that suppression of fT3 was associated with EF < 45% and inversely correlated with BNP levels.
The relationship between ESS and heart dysfunction has been extensively investigated. Low serum fT3 concentrations are a negative prognostic factor in patients with congestive heart failure, raising a question whether thyroid hormones may play a role in acute cardiac injury (46, 47). In children undergoing cardiac surgery more severe ESS changes are associated with prolonged hospital stays and increased ICU and mechanical ventilation requirements (48, 49).
A possible explanation of this relationship is that in cardiomyocytes, deiodinase D3 expression is low under physiological conditions while its activity is upregulated in case of myocardial infarction (50, 51). Another possible contributing factor is hypoxia because increased level of peripheral D3 are induced by hypoxemia due to decreased tissue perfusion during illness (52, 53).
Our study has some limitation due to the small number of patients secondary to the low prevalence of MIS-C and the monocentric nature of the study. Despite the very different samples size the post-hoc power analysis was very good, thus confirming the statistical significance of our findings. Indeed, further studies on larger populations should be needed to confirm the data.
Conclusion
Our study showed that most of MIS-C patients has ESS, mainly characterized by low levels of fT3. In addiction, results suggest that ESS, particularly fT3 level, is an independent risk factor for the disease severity of MIS-C. In fact, patients with ESS and lower fT3 had stronger inflammatory responses, more severe cardiac involvement and higher risk of ICU requirement. These findings may be helpful in clinical practice because fT3 is processed by conventional laboratory in urgency, therefore it could be used as an additional data in prompt decision making in patients with MIS-C.
Data availability statement
The original contributions presented in the study are included in the article/Supplementary Material, further inquiries can be directed to the corresponding author.
Ethics statement
This study was approved by Ethical Committees of Padua University.
Author contributions
MF data collection and analysis and manuscript writing, AM data collection and manuscript revision, JG data interpretation and manuscript revision, FV data analysis and interpretation, FT data collection and manuscript revision, FZ data interpretation and manuscript revision, GM study design, data analysis and interpretation and manuscript writing. All authors contributed to the article and approved the submitted version.
Conflict of interest
The authors declare that the research was conducted in the absence of any commercial or financial relationships that could be construed as a potential conflict of interest.
Publisher's note
All claims expressed in this article are solely those of the authors and do not necessarily represent those of their affiliated organizations, or those of the publisher, the editors and the reviewers. Any product that may be evaluated in this article, or claim that may be made by its manufacturer, is not guaranteed or endorsed by the publisher.
Supplementary material
The Supplementary Material for this article can be found online at: https://www.frontiersin.org/articles/10.3389/fped.2023.1217151/full#supplementary-material
References
1. Verdoni L, Mazza A, Gervasoni A, Martelli L, Ruggeri M, Ciuffreda M, et al. An outbreak of severe Kawasaki-like disease at the Italian epicentre of the SARS-CoV-2 epidemic: an observational cohort study. Lancet. (2020) 395(10239):1771–8. doi: 10.1016/S0140-6736(20)31103
2. Multisystem inflammatory syndrome in children (MIS-C) associated with coronavirus disease 2019 (COVID-19). Centers for Disease Control and Prevention Health Alert Network (2020).
3. Feldstein LR, Rose EB, Horwitz SM, Collins JP, Newhams MM, Son MBF, et al. Overcoming COVID-19 investigators; CDC COVID-19 response team. Multisystem inflammatory syndrome in U.S. Children and adolescents. N Engl J Med. (2020) 383(4):334–46. doi: 10.1056/NEJMoa2021680
4. Calcaterra V, Biganzoli G, Dilillo D, Mannarino S, Fiori L, Pelizzo G, et al. Non-thyroidal illness syndrome and SARS-CoV-2-associated multisystem inflammatory syndrome in children. J Endocrinol Invest. (2022) 45(1):199–208. doi: 10.1007/s40618-021-01647-9
5. Yildizdaş D, Onenli-Mungan N, Yapicioğlu H, Topaloğlu AK, Sertdemir Y, Yüksel B. Thyroid hormone levels and their relationship to survival in children with bacterial sepsis and septic shock. J Pediatr Endocrinol Metab. (2004) 17(10):1435–42. doi: 10.1515/JPEM.2004.17.10.1435
6. Hu YY, Li GM, Wang W. Euthyroid sick syndrome in children with diabetic ketoacidosis. Saudi Med J. (2015) 36(2):243–7. doi: 10.15537/smj.2015.2.10304
7. Lynch BA, Brown DM, Herrington C, Braunlin E. Thyroid dysfunction after pediatric cardiac surgery. J Thorac Cardiovasc Surg. (2004) 127(5):1509–11. doi: 10.1016/j.jtcvs.2003.11.014
8. Jacobs A, Vanhorebeek I, Van den Berghe G. Nonthyroidal illness in critically ill children. Curr Opin Endocrinol Diabetes Obes. (2019) 26(5):241–9. doi: 10.1097/MED.0000000000000494
9. Mebis L, Van den Berghe G. Thyroid axis function and dysfunction in critical illness. Best Pract Res Clin Endocrinol Metab. (2011) 25(5):745–57. doi: 10.1016/j.beem.2011.03.002
10. Fliers E, Boelen A. An update on non-thyroidal illness syndrome. J Endocrinol Invest. (2021) 44(8):1597–607. doi: 10.1007/s40618-020-01482-4
11. Caron P. Thyroid disorders and SARS-CoV-2 infection: from pathophysiological mechanism to patient management. Ann Endocrinol (Paris). (2020) 81(5):507–10. doi: 10.1016/j.ando.2020.09.001
12. Gorini F, Bianchi F, Iervasi G. COVID-19 and thyroid: progress and prospects. Int J Environ Res Public Health. (2020) 17(18):6630. doi: 10.3390/ijerph17186630
13. Gavriatopoulou M, Korompoki E, Fotiou D, Ntanasis-Stathopoulos I, Psaltopoulou T, Kastritis E, et al. Organ-specific manifestations of COVID-19 infection. Clin Exp Med. (2020) 20(4):493–506. doi: 10.1007/s10238-020-00648-x
14. Lazartigues E, Qadir MMF, Mauvais-Jarvis F. Endocrine significance of SARS-CoV-2’s reliance on ACE2. Endocrinology. (2020) 161(9):bqaa108. doi: 10.1210/endocr/bqaa108
15. Marazuela M, Giustina A, Puig-Domingo M. Endocrine and metabolic aspects of the COVID-19 pandemic. Rev Endocr Metab Disord. (2020) 21(4):495–507. doi: 10.1007/s11154-020-09569-2
16. Muller I, Cannavaro D, Dazzi D, Covelli D, Mantovani G, Muscatello A, et al. SARS-CoV-2-related atypical thyroiditis. Lancet Diabetes Endocrinol. (2020) 8(9):739–41. doi: 10.1016/S2213-8587(20)30266-7
17. Mattar SAM, Koh SJQ, Rama Chandran S, Cherng BPZ. Subacute thyroiditis associated with COVID-19. BMJ Case Rep. (2020) 13(8):e237336. doi: 10.1136/bcr-2020-237336
18. Li MY, Li L, Zhang Y, Wang XS. Expression of the SARS-CoV-2 cell receptor gene ACE2 in a wide variety of human tissues. Infect Dis Poverty. (2020) 9(1):45. doi: 10.1186/s40249-020-00662-x
19. Rotondi M, Coperchini F, Ricci G, Denegri M, Croce L, Ngnitejeu ST, et al. Detection of SARS-COV-2 receptor ACE-2 mRNA in thyroid cells: a clue for COVID-19-related subacute thyroiditis. J Endocrinol Invest. (2021) 44(5):1085–90. doi: 10.1007/s40618-020-01436-w
20. Caricchio R, Gallucci M, Dass C, Zhang X, Gallucci S, Fleece D, et al. Preliminary predictive criteria for COVID-19 cytokine storm. Ann Rheum Dis. (2021) 80(1):88–95. doi: 10.1136/annrheumdis-2020-218323
21. Lania A, Sandri MT, Cellini M, Mirani M, Lavezzi E, Mazziotti G. Thyrotoxicosis in patients with COVID-19: the THYRCOV study. Eur J Endocrinol. (2020) 183(4):381–7. doi: 10.1530/EJE-20-0335
22. Zou R, Wu C, Zhang S, Wang G, Zhang Q, Yu B, et al. Euthyroid sick syndrome in patients with COVID-19. Front Endocrinol (Lausanne). (2020) 11:566439. doi: 10.3389/fendo.2020.566439
23. Chopra IJ, Huang TS, Beredo A, Solomon DH, Chua Teco GN, Mead JF. Evidence for an inhibitor of extrathyroidal conversion of thyroxine to 3,5,3'-triiodothyronine in sera of patients with nonthyroidal illnesses. J Clin Endocrinol Metab. (1985) 60(4):666–72. doi: 10.1210/jcem-60-4-666
24. Warner MH, Beckett GJ. Mechanisms behind the non-thyroidal illness syndrome: an update. J Endocrinol. (2010) 205(1):1–13. doi: 10.1677/JOE-09-0412
25. Pappa TA, Vagenakis AG, Alevizaki M. The nonthyroidal illness syndrome in the non-critically ill patient. Eur J Clin Invest. (2011) 41(2):212–20. doi: 10.1111/j.1365-2362.2010.02395.x
26. Michalaki M, Vagenakis AG, Makri M, Kalfarentzos F, Kyriazopoulou V. Dissociation of the early decline in serum T(3) concentration and serum IL-6 rise and TNFalpha in nonthyroidal illness syndrome induced by abdominal surgery. J Clin Endocrinol Metab. (2001) 86(9):4198–205. doi: 10.1210/jcem.86.9.7795
27. Van den Berghe G. Non-thyroidal illness in the ICU: a syndrome with different faces. Thyroid. (2014) 24(10):1456–65. doi: 10.1089/thy.2014.0201
28. Zheng J, Cui Z, Shi N, Tian S, Chen T, Zhong X, et al. Suppression of the hypothalamic-pituitary-thyroid axis is associated with the severity of prognosis in hospitalized patients with COVID-19. BMC Endocr Disord. (2021) 21(1):228. doi: 10.1186/s12902-021-00896-2
29. Haslak F, Gunalp A, Kasapcopur O. A cursed goodbye kiss from severe acute respiratory syndrome-coronavirus-2 to its pediatric hosts: multisystem inflammatory syndrome in children. Curr Opin Rheumatol. (2023) 35(1):6–16. doi: 10.1097/BOR.0000000000000910
30. Lacina L, Brábek J, Fingerhutová Š, Zeman J, Smetana K Jr. Pediatric inflammatory multisystem syndrome (PIMS)—potential role for cytokines such is IL-6. Physiol Res. (2021) 70(2):153–9. doi: 10.33549/physiolres.934673
31. Alsaied T, Tremoulet AH, Burns JC, Saidi A, Dionne A, Lang SM, et al. Review of cardiac involvement in multisystem inflammatory syndrome in children. Circulation. (2021) 143(1):78–88. doi: 10.1161/CIRCULATIONAHA.120.049836
32. Elvan-Tüz A, Ayrancı İ, Ekemen-Keleş Y, Karakoyun İ, Çatlı G, Kara-Aksay A, et al. Are thyroid functions affected in multisystem inflammatory syndrome in children? J Clin Res Pediatr Endocrinol. (2022) 14(4):402–8. doi: 10.4274/jcrpe.galenos.2022.2022-4-7
33. Boelen A, Wiersinga WM, Kohrle J. Contributions of cytokines to nonthyroidal illness. Curr Opin Endocrinol Diabetes. (2006) 13:444–50. doi: 10.1097/01.med.0000244227.21776.70
34. Sato K, Satoh T, Shizume K, Ozawa M, Han DC, Imamura H, et al. Inhibition of 125I organification and thyroid hormone release by interleukin-1, tumor necrosis factor-alpha, and interferon-gamma in human thyrocytes in suspension culture. J Clin Endocrinol Metab. (1990) 70(6):1735–43. doi: 10.1210/jcem-70-6-1735
35. Tang KT, Braverman LE, DeVito WJ. Tumor necrosis factor-alpha and interferon-gamma modulate gene expression of type I 5'-deiodinase, thyroid peroxidase, and thyroglobulin in FRTL-5 rat thyroid cells. Endocrinology. (1995) 136(3):881–8. doi: 10.1210/endo.136.3.7867596
36. Boelen A, Maas MA, Lowik CW, Platvoet MC, Wiersinga WM. Induced illness in interleukin-6 (IL-6) knock-out mice: a causal role of IL-6 in the development of the low 3,5,3'-triiodothyronine syndrome. Endocrinology. (1996) 137(12):5250–4. doi: 10.1210/endo.137.12.8940342
37. Boelen A, Platvoet-ter Schiphorst MC, Bakker O, Wiersinga WM. The role of cytokines in the lipopolysaccharide-induced sick euthyroid syndrome in mice. J Endocrinol. (1995) 146(3):475–83. doi: 10.1677/joe.0.1460475
38. Bartalena L, Bogazzi F, Brogioni S, Grasso L, Martino E. Role of cytokines in the pathogenesis of the euthyroid sick syndrome. Eur J Endocrinol. (1998) 138(6):603–14. doi: 10.1530/eje.0.1380603
39. Rasmussen AK, Kayser L, Feldt-Rasmussen U, Bendtzen K. Influence of tumour necrosis factor-alpha, tumour necrosis factor-beta and interferon-gamma, separately and added together with interleukin-1 beta, on the function of cultured human thyroid cells. J Endocrinol. (1994) 143(2):359–65. doi: 10.1677/joe.0.1430359
40. Yamashita S, Kimura H, Ashizawa K, Nagayama Y, Hirayu H, Izumi M, et al. Interleukin-1 inhibits thyrotrophin-induced human thyroglobulin gene expression. J Endocrinol. (1989) 122(1):177–83. doi: 10.1677/joe.0.1220177
41. Deuss U, Buscema M, Schumacher H, Winkelmann W. In vitro effects of tumor necrosis factor-alpha on human thyroid follicular cells. Acta Endocrinol (Copenh). (1992) 127(3):220–5. doi: 10.1530/acta.0.1270220
42. Poth M, Tseng YC, Wartofsky L. Inhibition of TSH activation of human cultured thyroid cells by tumor necrosis factor: an explanation for decreased thyroid function in systemic illness? Thyroid. (1991) 1(3):235–40. doi: 10.1089/thy.1991.1.235
43. Nagaya T, Fujieda M, Otsuka G, Yang JP, Okamoto T, Seo H. A potential role of activated NF-kappa B in the pathogenesis of euthyroid sick syndrome. J Clin Invest. (2000) 106(3):393–402. doi: 10.1172/JCI7771
44. Fekete C, Gereben B, Doleschall M, Harney JW, Dora JM, Bianco AC, et al. Lipopolysaccharide induces type 2 iodothyronine deiodinase in the mediobasal hypothalamus: implications for the nonthyroidal illness syndrome. Endocrinology. (2004) 145(4):1649–55. doi: 10.1210/en.2003-1439
45. Zeöld A, Doleschall M, Haffner MC, Capelo LP, Menyhért J, Liposits Z, et al. Characterization of the nuclear factor-kappa B responsiveness of the human dio2 gene. Endocrinology. (2006) 147(9):4419–29. doi: 10.1210/en.2005-1608
46. Pingitore A, Landi P, Taddei MC, Ripoli A, L'Abbate A, Iervasi G. Triiodothyronine levels for risk stratification of patients with chronic heart failure. Am J Med. (2005) 118(2):132–6. doi: 10.1016/j.amjmed.2004.07.052
47. Frey A, Kroiss M, Berliner D, Seifert M, Allolio B, Güder G, et al. Prognostic impact of subclinical thyroid dysfunction in heart failure. Int J Cardiol. (2013) 168(1):300–5. doi: 10.1016/j.ijcard.2012.09.064
48. Marks SD, Haines C, Rebeyka IM, Couch RM. Hypothalamic-pituitary-thyroid axis changes in children after cardiac surgery. J Clin Endocrinol Metab. (2009) 94(8):2781–6. doi: 10.1210/jc.2008-2722
49. Allen DB, Dietrich KA, Zimmerman JJ. Thyroid hormone metabolism and level of illness severity in pediatric cardiac surgery patients. J Pediatr. (1989) 114(1):59–62. doi: 10.1016/s0022-3476(89)80601-8
50. Wassen FW, Schiel AE, Kuiper GG, Kaptein E, Bakker O, Visser TJ, et al. Induction of thyroid hormone-degrading deiodinase in cardiac hypertrophy and failure. Endocrinology. (2002) 143(7):2812–5. doi: 10.1210/endo.143.7.8985
51. Olivares EL, Marassi MP, Fortunato RS, da Silva AC, Costa-e-Sousa RH, Araújo IG, et al. Thyroid function disturbance and type 3 iodothyronine deiodinase induction after myocardial infarction in rats a time course study. Endocrinology. (2007) 148(10):4786–92. doi: 10.1210/en.2007-0043
52. Peeters RP, Wouters PJ, Kaptein E, van Toor H, Visser TJ, Van den Berghe G. Reduced activation and increased inactivation of thyroid hormone in tissues of critically ill patients. J Clin Endocrinol Metab. (2003) 88(7):3202–11. doi: 10.1210/jc.2002-022013
Keywords: children, COVID-19, euthyroid sick syndrome, multisystem inflammatory syndrome, SARS-CoV2
Citation: Fastiggi M, Meneghel A, Gutierrez de Rubalcava Doblas J, Vittadello F, Tirelli F, Zulian F and Martini G (2023) Prognostic role of euthyroid sick syndrome in MIS-C: results from a single-center observational study. Front. Pediatr. 11:1217151. doi: 10.3389/fped.2023.1217151
Received: 4 May 2023; Accepted: 17 July 2023;
Published: 10 August 2023.
Edited by:
Angela Mauro, Fate Bene Fratelli Hospital, ItalyReviewed by:
Ozgur Kasapcopur, Istanbul University-Cerrahpasa, TürkiyeEmanuele Bizzi, ASST Fatebenefratelli Sacco, Italy
© 2023 Fastiggi, Meneghel, Gutierrez de Rubalcava Doblas, Vittadello, Tirelli, Zulian and Martini. This is an open-access article distributed under the terms of the Creative Commons Attribution License (CC BY). The use, distribution or reproduction in other forums is permitted, provided the original author(s) and the copyright owner(s) are credited and that the original publication in this journal is cited, in accordance with accepted academic practice. No use, distribution or reproduction is permitted which does not comply with these terms.
*Correspondence: Giorgia Martini giorgia.martini@aopd.veneto.it
Abbreviations ACE, angiotensin-converting enzyme; BNP, brain natriuretic peptide; cAMP, cyclic adenosine monophosphate; CBC, complete blood count; CDC, Centers for Disease Control and Prevention; CNS, Central nervous system; Covid-19, CoronaVirus disease 2019; CRP, C-reactive protein; D1, deiodinase 1; D3, deiodinase 3; EF, ejection fraction; EKG, electrocardiogram; ESR, erythrosedimentation rate; ESS, euthyroid sick syndrome; fT3, free triiodothyronine; fT4, free thyroxine; GOT, glutamic-oxaloacetic transaminase; GPT, glutamic-pyruvic transaminase; Hb, haemoglobin; ICU, intensive care unit; IFN, interferon; IL, interleukin; IL-2R, soluble IL2 receptor; MIS-C, Multisystem Inflammatory Syndrome in children; PFAPA, periodic fever, aphtous stomatitis, pharyngitis, adenitis; rT3, reverse T3; S100, S-100 protein; SARS-CoV2, Severe Acute Respiratory Syndrome –CoronaVirus 2; Tg, thyroglobulin; TNF, tumor necrosis factor; TnI, troponin I; TSH, thyroid stimulating hormone; WBC, white blood cells.