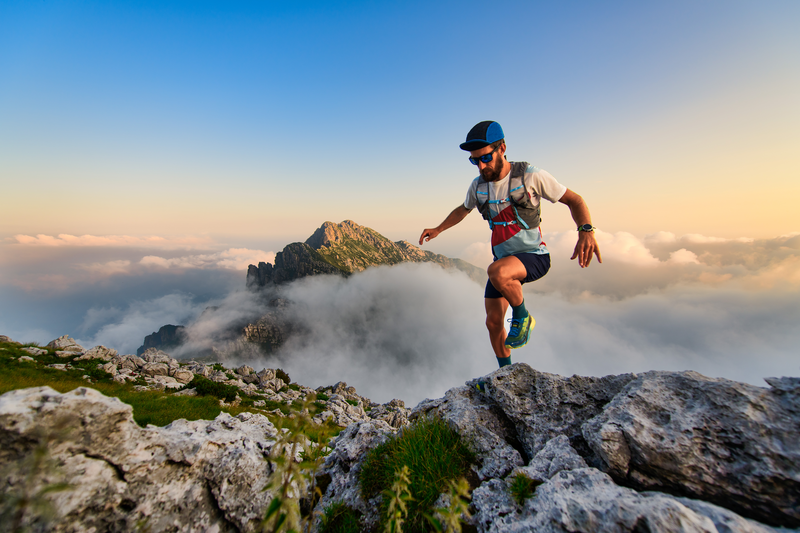
94% of researchers rate our articles as excellent or good
Learn more about the work of our research integrity team to safeguard the quality of each article we publish.
Find out more
ORIGINAL RESEARCH article
Front. Pediatr. , 28 April 2023
Sec. Pediatric Pulmonology
Volume 11 - 2023 | https://doi.org/10.3389/fped.2023.1167506
Background: Noninvasive positive-pressure ventilation (NPPV) can be effective in children with acute asthma. However, clinical evidence remains limited. The objective of the meta-analysis was to systematically assess NPPV's effectiveness and safety in treating children with acute asthma.
Methods: Relevant randomized controlled trials were obtained from electronic resources, including PubMed, Embase, Cochrane's Library, Wanfang, and CNKI databases. The influence of potential heterogeneity was taken into account before using a random-effect model to pool the results.
Results: A total of 10 RCTs involving 558 children with acute asthma were included in the meta-analysis. Compared to conventional treatment alone, additional use of NPPV significantly improved early blood gas parameters such as the oxygen saturation (mean difference [MD]: 4.28%, 95% confidence interval [CI]: 1.51 to 7.04, p = 0.002; I2 = 80%), partial pressure of oxygen (MD: 10.61 mmHg, 95% CI: 6.06 to 15.16, p < 0.001; I2 = 89%), and partial pressure of carbon dioxide (MD: −6.29 mmHg, 95% CI: −9.81 to −2.77, p < 0.001; I2 = 85%) in the arterial blood. Moreover, NPPV was also associated with early reduced respiratory rate (MD: −12.90, 95% CI: −22.21 to −3.60, p = 0.007; I2 = 71%), improved symptom score (SMD: −1.85, 95% CI: −3.65 to −0.07, p = 0.04; I2 = 92%), and shortened hospital stay (MD: −1.82 days, 95% CI: −2.32 to −1.31, p < 0.001; I2 = 0%). No severe adverse events related to NPPV were reported.
Conclusions: NPPV in children with acute asthma is associated with improved gas exchange, decreased respiratory rates, a lower symptom score, and a shorter hospital stay. These results suggest that NPPV may be as effective and safe as conventional treatment for pediatric patients with acute asthma.
As a prevalent chronic disease in childhood, asthma exacerbations or acute attacks have become one of the most common reasons for emergency department visits or hospitalization (1, 2). Although severe acute asthma can be prevented, it is a leading cause of pediatric patient mortality, especially in patients from developing nations (3, 4). An acute asthma attack refers to progressive respiratory symptoms (wheezing, cough, or dyspnea) and impaired pulmonary function (3, 4). Pathophysiologically, asthma exacerbation is characterized by bronchospasm, inflamed airways, mucous plugging, and an imbalance between ventilation and perfusion (5). Accordingly, conventional therapy for acute severe asthma includes the rapid reversal of airway obstruction and oxygen supplementation to correct hypoxia and prevent relapse (5). Despite these efforts, some children with acute severe asthma continue to have breathless symptoms, and respiratory failure may develop in these patients (6). For these children, noninvasive positive-pressure ventilation (NPPV) has been suggested to be an additional efficacious treatment (7). Early use of NPPV in high-risk children with acute severe asthma could theoretically reduce the work of breathing and give more time for the pharmacological treatment to achieve maximal efficacy, thereby reducing the risk of respiratory failure and subsequent need for mechanical ventilation (8). Although experimental clinical observations support the use of NPPV for children with acute severe asthma, evidence based on clinical trials is still lacking (9–11). In this meta-analysis, we aimed to systematically evaluate the efficacy and safety of NPPV for children with acute asthma by summarizing the results of available randomized controlled trials (RCTs).
During its design and implementation, this study followed PRISMA (Preferred Reporting Items for Systematic Reviews and Meta-Analyses) (12, 13) and Cochrane Handbook (14) guidelines.
The following strategies were used to search the databases PubMed, Embase, Cochrane Library, Wanfang, and China National Knowledge Infrastructure (CNKI): (1) “non-invasive” OR “noninvasive” OR “positive-pressure” OR “positive pressure” OR “pressure support” OR “pressure-support” OR “positive airway” or “positive-airway” OR “pressure control” OR “pressure-control” OR “bi-level” OR “NPPV” OR “NIPPV” OR “CPAP” OR “BiPAP” OR “ventilation” OR “ventilating”; (2) “asthma” OR “wheeze” OR “wheezing”; (3) “child” OR “children” OR “adolescent” OR “pediatric” OR “paediatric” OR “infant” OR “neonate” OR “newborn” OR “toddler”; and (4) “random” OR “randomly” OR “randomized” OR “randomised” OR “placebo”. Only studies involving human participants and published as full-length articles in a journal subject to peer review were considered. In addition to the final database search, references to relevant reviews and original articles were also investigated. The last database search was conducted on 31 December 2022.
The PICOS principle was followed in designating the meta-analysis inclusion criteria.
P (patients): Children (<18 years) with a confirmed diagnosis of acute asthma.
I (intervention): A treatment group of NPPV based on conventional therapy.
C (control): A control group of conventional therapy alone.
O (outcomes): Between-group difference of changes of one or more of the following results: (1) acute changes of parameters of blood gas analysis (BGA) evaluated within four hours after NPPV treatment, including oxygen saturation (SaO2), partial pressure of oxygen (PaO2), and partial pressure of carbon dioxide (PaCO2) in the arterial blood; (2) acute changes of the respiratory rate (RR) and/or clinical symptoms scores of asthma [such as the Clinical Asthma Score (CAS)] within four hours after NPPV treatment; and (3) length of hospitalization (LOH).
S (study design): Parallel-group or crossover RCTs published as full-length papers in Chinese or English.
Non-randomized studies, studies enrolling adult patients, studies with pediatric patients of other respiratory diseases rather than acute asthma, studies without the intervention of NPPV, or studies not reporting relevant results were excluded. Studies with a high-flow nasal cannula (HFNC) were also excluded because HFNC is not a form of NPPV. The key difference between HFNC and NPPV is that compared to HFNC, NPPV can create a much higher gas flow rate and positive airway pressure (15). In clinical practice, HFNC is used as a midway point between low-flow oxygen devices and NPPV (15). The study with the largest sample size was included in the meta-analysis for studies with overlapping patient populations.
Two authors worked independently on database searches, data collection, and quality assessment. If there were any disagreements, they were discussed with the corresponding author. We gathered information about each study's first author, publication year, study country, study design (blind or open-label), patient information (diagnosis, number of patients, and mean age), clinical setting, details about NPPV treatment and controls, treatment duration, and outcomes. The Cochrane Risk of Bias Tool was used to assess the quality of the included RCTs (14) by assigning random sequences, concealing allocations, blinding participants and personnel, blinding outcomes assessors, incomplete outcomes data, and selective outcome reporting.
The mean difference (MD) with a 95% confidence interval represented the effects of NPPV on the BGA parameters, RR, and LOH (CI). Because different scores were used, the effects of NPPV on clinical symptom scores were presented as standardized mean difference (SMD) and 95% CI. The Cochrane's Q test was used to evaluate heterogeneity (14). The I2 statistic was also calculated, and an I2 > 50% denotes significant heterogeneity(16). A random-effects model was used when calculating pooled analyses because it considers potential heterogeneity and yields more generalized results (14). In order to assess how each study affected the combined results, influencing analyses were carried out by removing one study at a time from the meta-analysis (14). Visual examination of funnel plots and the Egger's regression asymmetry test were used to assess publication bias (17). According to the guidelines in Cochrane's Handbook, shared intervention groups in studies with multiple comparisons were equally split and included as independent comparisons to avoid a unit-of-analysis error (14). Differences for p < 0.05 were deemed statistically significant. The RevMan (Version 5.1; Cochrane, Oxford, UK) and Stata (Version 12.0; Stata Corporation, College Station, TX) software packages were used to conduct the statistical analyses.
The study flow chart is shown in Figure 1. In a nutshell, database searches turned up 1,133 articles, and 961 of them were found after duplicate records were eliminated. Following that, 935 articles were disqualified based on their titles and abstracts, mainly because they had nothing to do with the objective of the meta-analysis. A total of 16 of the 26 articles that had received full-text reviews were later eliminated for the reasons shown in Figure 1. Ten RCTs (17–26) were ultimately determined to be qualified for the meta-analysis.
Tables 1, 2 provide a summary of the included studies. Overall, ten RCTs (18–27) involving 558 children with acute asthma were included in the meta-analysis. These studies were conducted in the United States and China and published between 2004 and 2021. As for the study design, all included studies were parallel-group RCTs except one crossover RCT (18). Two studies were performed in a pediatric intensive care unit (PICU) (18, 22) and one in the emergency department (25). The remaining studies were in pediatric wards (19–21, 23, 24, 26, 27). The sample sizes of the included studies were limited, varying from 20 to 94. The mean ages of the children were between 3.2 and 8.6 years, and the proportions of males were 51% to 72%. As for the comorbidities, three studies excluded patients with children with other pulmonary diseases, congenital heart diseases, or heart failure (23, 25, 27). The conventional therapies included inhaled beta 2-agonists and intravenous corticosteroids, which were balanced between the intervention and control groups, as mentioned in the original studies. Besides conventional therapy, NPPV was applied additionally in patients of the intervention group, while patients from the control groups received conventional therapy alone (including oxygen supplementation). As for the models of NPPV, bi-level positive airway pressure (BiPAP) was used in seven studies (18–20, 22–24, 26), and other models such as pressure support ventilation (PSV) and positive end-expiratory pressure (PEEP), and positive expiratory pressure (PEP) were used in the other studies (21, 25, 27). By definition, BiPAP delivers a set positive airway pressure during expiration and a higher pressure during inspiration to support inspiratory effort (28); PSV is a mode of positive pressure mechanical ventilation in which the patient triggers every breath, which could be used with PEEP (29); PEEP refers to the positive pressure that will remain in the airways at the end of the respiratory cycle (end of exhalation) that is greater than the atmospheric pressure in mechanically ventilated patients (30); PEP provides a back pressure to the airways during expiration, which is expected to improve respiratory status in acute asthma by recruiting collapsed alveoli, reversing atelectasis, and improving ventilation-perfusion mismatch (25). The observation duration was within PICU in one study (18) and within hospitalization in the others (19–27). Using Cochrane's Risk of Bias Tool, Table 3 provides a detailed analysis of the included RCTs. One included study was double-blind (25), while the others were open-label (18–24, 26, 27). Three studies reported random sequence generation details (19, 21, 27), and the details of allocation concealment were not reported. No evidence of incomplete outcome data, selective reporting, or other sources of bias was detected for all of the included RCTs.
Pooling the results of four studies (19, 21, 23, 27) including 277 patients showed that compared to conventional treatment alone, additional use of NPPV significantly improved SaO2 (MD: 4.28%, 95% CI: 1.51 to 7.04, p = 0.002; I2 = 80%; Figure 2A) within four hours after the initiation of the therapy. In addition, pooled results of five studies (19, 21, 23, 26, 27) including 357 patients indicated that NPPV also improved PaO2 (MD: 10.61 mmHg, 95% CI: 6.06 to 15.16, p < 0.001; I2 = 89%; Figure 2B) and PaCO2 (MD: −6.29 mmHg, 95% CI: −9.81 to −2.77, p < 0.001; I2 = 85%; Figure 2C) acutely compared to controls with conventional treatment only. The results were not affected by excluding one study at a time from the analysis.
Figure 2. Forest plots for the meta-analysis evaluating the influences of NPPV on acute changes of BGA parameters in children with acute asthma; (A) Meta-analysis for the influences of NPPV on SaO2; (B) Meta-analysis for the influences of NPPV on PaO2; and (C) meta-analysis for the influences of NPPV on PaCO2.
Pooled results of two studies (18, 22) including 60 patients suggested that NPPV was also associated with the early reduced respiratory rate (MD: −12.90, 95% CI: −22.21 to −3.60, p = 0.007; I2 = 71%; Figure 3A). Besides, three studies (18, 22, 25) reported a change in symptom scores following treatment. Among them, the clinical asthma score (CAS) was reported in two studies (18, 22), which was a symptom-based severity score of acute asthma incorporating three domains, such as increased work of breathing, wheezing, and dyspnea (31). In another study (25), the pulmonary asthma score (PAS), which is a pediatric asthma severity scoring system, includes measures of respiratory rate, oxygen saturation, auscultatory findings, retractions, and symptoms of dyspnea (32). Subsequently, pooling the results of three studies (18, 22, 25) including 112 patients demonstrated that NPPV could significantly improve the symptom score (SMD: −1.85, 95% CI: −3.65 to −0.07, p = 0.04; I2 = 92%; Figure 3B) within four hours after the initiation of the treatment for children with acute asthma compared to controls with conventional treatment alone.
Figure 3. Forest plots for the meta-analysis evaluating the influences of NPPV on acute changes of RR and symptoms score in children with acute asthma; (A) Meta-analysis for the influences of NPPV on acute change of RR; and (B) meta-analysis for the influences of NPPV on acute change of symptoms score.
Four studies including 189 patients reported the outcome of LOH (19, 20, 22, 24). The meta-analysis showed that LOH was significantly decreased for pediatric patients with acute asthma who received NPPV compared to controls with conventional treatment alone (MD: −1.82 days, 95% CI: −2.32 to −1.31, p < 0.001; I2 = 0%; Figure 4).
In either of the included studies, no severe adverse events associated with NPPV were reported.
The funnel plots for the meta-analyses comparing the effects of NPPV on SaO2, PaO2, PaCO2, and LOH are shown in Figures 5A–D. The symmetry of these plots indicates a low risk of publication bias. Egger's regression tests were not performed due to the limited studies included (four or five studies for each outcome). Only two or three studies were included, making it difficult to estimate the publication biases underlying the other results.
Figure 5. Funnel plots evaluating the publication biases of the meta-analyses. (A) Funnel plots for the meta-analysis of SaO2; (B) Funnel plots for the meta-analysis of PaO2; (C) Funnel plots for the meta-analysis of PaCO2; and (D) funnel plots for the meta-analysis of LOH.
In this meta-analysis, we retrieved all available RCTs which evaluated the efficacy and safety of NPPV for children with acute asthma. The results showed that NPPV based on conventional therapy could significantly improve gas exchange within four hours after the initiation of the treatment, as evidenced by improved SaO2, increased PaO2, and decreased PaCO2 compared to conventional therapy alone. Moreover, the RR and asthma symptomatic scores were also acutely improved within four hours after the initiation of NPPV. The LOH was also significantly reduced for children who received NPPV. No severe adverse events related to NPPV were reported. These findings suggest that NPPV may be an effective and safe treatment strategy next to conventional treatment for pediatric patients with acute asthma.
To the best of our knowledge, there have not been many meta-analyses examining the function of NPPV in kids with acute asthma. In this meta-analysis, we retrieved relevant RCTs from five common English and Chinese electronic databases and summarized the current evidence regarding the efficacy and safety of NPPV for children with acute asthma. Because of the potential differences in the disease status and treatment tolerance of children and adults with acute asthma (4, 33), we carefully selected studies that included only pediatric patients. In addition, only RCTs were included, potentially minimizing the influences of confounding patients or study characteristics. Moreover, multiple outcomes were investigated, such as the acute changes of BGA parameters that reflect gas exchange, acute changes of RR and clinical symptomatic asthma scores, and the overall LOH, which all indicate a favorable role of NPPV. Finally, influencing analysis was performed, and the consistent results suggested the robustness of the finding, which was not primarily contributed by either of the included studies.
The potential benefits of NPPV for children with acute asthma may be multifactorial. As mentioned previously, using NPPV earlier in high-risk children with acute severe asthma could reduce their work of breathing, and more time could be given for the pharmacological treatment to reach maximum effect (34). This could be reflected by the acutely improved BGA parameters, reduced RR, and favorably changed symptomatic scores following NPPV in children with acute asthma in this meta-analysis. In recent large-scale retrospective studies involving both pediatric and adult patients with acute asthma exacerbation, the use of noninvasive ventilation was linked with a reduced likelihood of receiving invasive mechanical ventilation and a lower mortality rate in the hospital, suggesting that NPPV may be effective in improving the clinical outcomes (7). A recent survey with the Virtual Pediatric Systems database showed that from 2009 to 2019, a halving in the use of intubation has occurred in pediatric asthma, while a more than doubling in the use of noninvasive ventilation has occurred (35), which may also reflect the efficacy and tolerability of NPPV in real-world clinical practice. Interestingly, for pediatric outpatients with asthma, NPPV was demonstrated to reduce pulmonary inflammation and exercise-induced bronchospasm and increase asthma control and exercise durability (36, 37). To assess the long-term benefit of NPPV in children with asthma, large-scale RCTs are required.
In real-world clinical practice, determining when to initiate NPPV and which model of NPPV (BiPAP, PEP, or PEEP) to use in young children with respiratory disorders, including acute asthma, could be complicated. Since no evidence-based recommendation could be obtained as to the superiority of one model of NPPV over another in determining the initial treatment setting for NPPV, the medical team will consider hospital protocols, the availability of appropriate staff like respiratory therapists and nurses, and the degree of monitoring desired. More importantly, the choice and adjustment depend on the response and tolerability to the initial NPPV setting, jointly determined by the respiratory therapy, nursing, and medical teams. Overall, the meta-analysis results support the use of NPPV in pediatric patients with acute asthma. More studies and observations are needed to determine the optimal model and parameters of NPPV in this clinical circumstance.
On the other hand, besides NPPV, other non-invasive ventilation strategies have also shown satisfying efficacy and safety for children with acute asthma, such as HFNC (38). The mechanisms of HFNC involve delivering a high flow rate that exceeds inspiratory demand flow, providing minimal end-distending pressure, generating nasopharyngeal pressure, and reducing airway resistance (38). A pilot study showed that HFNC appears superior to conventional oxygen therapy for reducing respiratory distress within the first 2 h of treatment in children with moderate-to-severe asthma exacerbation refractory to first-line treatment (39). Another study in children with bronchiolitis suggested that FNC may be an effective and pleasant alternative to NPPV, showing similar efficacy in improving the respiratory rate, PaCO2, and symptom score (40). HFNC could significantly elevate PaO2 and RR compared to conventional therapy, making it a promising option for patients with severe bronchial asthma complicated with respiratory failure (41). Interestingly, another retrospective analysis enrolling 42 children with severe acute asthma showed that HFNC is a lower level of respiratory support than NPPV, which could potentially delay the initiation of NPPV in these patients (42). Generally, HFNC is effective and well-tolerated in pediatric patients with acute asthma, which has been well-applied in real-world clinical practice as a midway point between low-flow oxygen devices and NPPV (43). Further studies are required to determine the optimal strategy and protocol for non-invasive ventilation in pediatric patients with acute asthma.
This study also has limitations. Firstly, although we aimed to pool the current available RCTs, the total number of studies and sample sizes for each meta-analysis outcome remained small. The results need to be validated by large-scale RCTs. Moreover, heterogeneity was significant among the included studies, which may be explained by differences in patient characteristics, concurrent therapy, the severity of the disease, and modes and settings of NPPV. However, we could not determine the source of heterogeneity since limited studies are available. Studies are warranted in the future to determine if the benefits of NPPV are consistent in studies with different ventilatory modes and settings. Furthermore, future clinical studies should investigate the effects of NPPV on the clinical outcomes of pediatric patients with acute asthma. Finally, further research will be needed to determine the best NPPV model for treating children with acute asthma exacerbations.
In conclusion, the results of the meta-analysis indicate that early use of NPPV in pediatric patients with acute asthma could acutely improve the BGA parameters of gas exchange and asthma symptoms and shorten the overall LOH without any severe adverse events related to the treatment. These findings support using NPPV as an adjuvant treatment to conventional therapies for children with acute asthma exacerbation.
The original contributions presented in the study are included in the article further inquiries can be directed to the corresponding author/s.
JD: Conceptualization; Data curation; Writing—original draft. LW: Data curation. LW: Conceptualization; Investigation. FW: Formal analysis; Writing—original draft. QW: Validation; Writing—review & editing. All authors contributed to the article and approved the submitted version.
The authors declare that the research was conducted in the absence of any commercial or financial relationships that could be construed as a potential conflict of interest.
All claims expressed in this article are solely those of the authors and do not necessarily represent those of their affiliated organizations, or those of the publisher, the editors and the reviewers. Any product that may be evaluated in this article, or claim that may be made by its manufacturer, is not guaranteed or endorsed by the publisher.
1. Martin J, Townshend J, Brodlie M. Diagnosis and management of asthma in children. BMJ Paediatr Open. (2022) 6(1):e001277. doi: 10.1136/bmjpo-2021-001277
2. Editorial Board, Chinese Journal of Pediatrics; Subspecialty Group of Respiratory Diseases, the Society of Pediatrics, Chinese Medical Association; Children's Respiratory Professional Committee, the Society of Pediatrics of Chinese Medical Doctor Association. Recommendations for Diagnosis and Management of bronchial asthma in children (2020). Zhonghua Er Ke Za Zhi. (2020) 58(9):708–17. Article in Chinese. doi: 10.3760/cma.j.cn112140-20200604-00578
3. Mahesh S, Ramamurthy MB. Management of acute asthma in children. Indian J Pediatr. (2022) 89(4):366–72. doi: 10.1007/s12098-021-04051-6
4. Chavasse R, Scott S. The differences in acute management of asthma in adults and children. Front Pediatr. (2019) 7:64. doi: 10.3389/fped.2019.00064
5. Saglani S, Fleming L, Sonnappa S, Bush A. Advances in the aetiology. Management, and prevention of acute asthma attacks in children. Lancet Child Adolesc Health. (2019) 3(5):354–64. doi: S2352-4642(19)30025-2
6. Hoch HE, Houin PR, Stillwell PC. Asthma in children: a brief review for primary care providers. Pediatr Ann. (2019) 48(3):e103–e9. doi: 10.3928/19382359-20190219-01
7. Althoff MD, Holguin F, Yang F, Grunwald GK, Moss M, Vandivier RW, et al. Noninvasive ventilation use in critically ill patients with acute asthma exacerbations. Am J Respir Crit Care Med. (2020) 202(11):1520–30. doi: 10.1164/rccm.201910-2021OC
8. Soroksky A, Klinowski E, Ilgyev E, Mizrachi A, Miller A, Ben Yehuda TM, et al. Noninvasive positive pressure ventilation in acute asthmatic attack. Eur Respir Rev. (2010) 19(115):39–45. doi: 10.1183/09059180.00006109
9. Korang SK, Feinberg J, Wetterslev J, Jakobsen JC. Non-invasive positive pressure ventilation for acute asthma in children. Cochrane Database Syst Rev. (2016) 9(9):CD012067. doi: 10.1002/14651858.CD012067.pub2
10. Landry A, Foran M, Koyfman A. Does noninvasive positive-pressure ventilation improve outcomes in severe asthma exacerbations? Ann Emerg Med. (2013) 62(6):594–6. doi: 10.1016/j.annemergmed.2013.05.021
11. Scala R. Noninvasive ventilation for acute asthma. “spill-over” or “lighted windows"? Ann Am Thorac Soc. (2016) 13(7):1005–7. doi: 10.1513/AnnalsATS.201604-279ED
12. Page MJ, McKenzie JE, Bossuyt PM, Boutron I, Hoffmann TC, Mulrow CD, et al. The prisma 2020 statement: an updated guideline for reporting systematic reviews. Br Med J. (2021) 372:n71. doi: 10.1136/bmj.n71
13. Page MJ, Moher D, Bossuyt PM, Boutron I, Hoffmann TC, Mulrow CD, et al. Prisma 2020 explanation and elaboration: updated guidance and exemplars for reporting systematic reviews. Br Med J. (2021) 372:n160. doi: 10.1136/bmj.n160
14. Higgins J, Thomas J, Chandler J, Cumpston M, Li T, Page M, et al. Cochrane handbook for systematic reviews of interventions version 6.2. Chichester, UK: The Cochrane Collaboration (2021). www.training.cochrane.org/handbook
15. Ohshimo S. Oxygen administration for patients with ards. J Intensive Care. (2021) 9(1):17. doi: 10.1186/s40560-021-00532-0
16. Higgins JP, Thompson SG, Deeks JJ, Altman DG. Measuring inconsistency in meta-analyses. Br Med J. (2003) 327(7414):557–60. doi: 10.1136/bmj.327.7414.557
17. Egger M, Davey Smith G, Schneider M, Minder C. Bias in meta-analysis detected by a simple, graphical test. Br Med J. (1997) 315(7109):629–34. doi: 10.1136/bmj.315.7109.629
18. Thill PJ, McGuire JK, Baden HP, Green TP, Checchia PA. Noninvasive positive-pressure ventilation in children with lower airway obstruction. Pediatr Crit Care Med. (2004) 5(4):337–42. doi: 10.1097/01.pcc.0000128670.36435.83
19. Chen F. Study of noninvasive bi-level positive airway pressure ventilation in the treatment of severe bronchial asthma in children. Clin Lung J. (2011) 16(12):1865–6. doi: 10.3969/j.issn.1009-6663.2011.12.019
20. He HY, Xiang HJ, Li H. Clinical observation of cpap in treating pediatric asthma sustaining state. J Med Forum. (2011) 32(1):6–70. doi: 1672-3422(2011)01-0066-03
21. Yuan F, Zhang MJ, Zhang JH, Wu LX, Wu M, Xu M. Appficafion of noninvasive posifive pressnre ventilation in treatment for severe bronchial asthma in children. J Appl Clin Pediatr. (2011) 26(16):1265–7. doi: 10.3969/j.issn.1003-515X.2011.16.012
22. Basnet S, Mander G, Andoh J, Klaska H, Verhulst S, Koirala J. Safety, efficacy, and tolerability of early initiation of noninvasive positive pressure ventilation in pediatric patients admitted with Status asthmaticus: a pilot study. Pediatr Crit Care Med. (2012) 13(4):393–8. doi: 10.1097/PCC.0b013e318238b07a
23. Liu J, Tang M. Clinical analysis of non-invasive positive pressure ventilation in the treatment of acute attack of asthma in preschool children. Yunnan Med J. (2012) 33(5):446–7.
24. Yang AY. Observation on the effect of non-invasive positive pressure ventilation in children with persistent asthma. Chin J Mod Drug Appl. (2014) 8(17):113–4. doi: 10.14164/j.cnki.cn11-5581/r.2014.17.168
25. Navanandan N, Federico M, Mistry RD. Positive expiratory pressure for the treatment of acute asthma exacerbations: a randomized controlled trial. J Pediatr. (2017) 185:149–54.e2. doi: 10.1016/j.jpeds.2017.02.032
26. Gao L. Clinical effect of noninvasive positive pressure ventilation on pediatric severe bronchial asthma. Clin Med Res Pract. (2018) 3(10):90–1. doi: 10.19347/j.cnki.2096-1413.201810043
27. Jiang L, Liao LY, Ai ZH. Effect of noninvasive positive pressure ventilation in the treatment of severe bronchial asthma in children. Chin Mod Med. (2021) 28(32):116–8.
28. Boghi D, Kim KW, Kim JH, Lee SI, Kim JY, Kim KT, et al. Noninvasive ventilation for acute respiratory failure in pediatric patients: a systematic review and meta-analysis. Pediatr Crit Care Med. (2023) 24(2):123–32. doi: 10.1097/PCC.0000000000003109
29. Cammarota G, Simonte R, De Robertis E. Comfort during non-invasive ventilation. Front Med. (2022) 9:874250. doi: 10.3389/fmed.2022.874250
30. Mora Carpio AL, Mora JI. Positive End-expiratory pressure. In: StatPearls [Internet]. Treasure Island (FL): StatPearls Publishing. (2023). doi: NBK441904 [bookaccession]
31. Schuh S, Johnson DW, Callahan S, Canny G, Levison H. Efficacy of frequent nebulized ipratropium bromide added to frequent high-dose albuterol therapy in severe childhood asthma. J Pediatr. (1995) 126(4):639–45. doi: 10.1016/s0022-3476(95)70368-3
32. Ducharme FM, Chalut D, Plotnick L, Savdie C, Kudirka D, Zhang X, et al. The pediatric respiratory assessment measure: a valid clinical score for assessing acute asthma severity from toddlers to teenagers. J Pediatr. (2008) 152(4):476–80, 80 e1. doi: 10.1016/j.jpeds.2007.08.034
33. Szefler SJ, Chipps B. Challenges in the treatment of asthma in children and adolescents. Ann Allergy Asthma Immunol. (2018) 120(4):382–8. doi: 10.1016/j.anai.2018.01.003
34. Sheikh M, Tiruvoipati R, Hurley JC. Non-invasive ventilation of patients with acute asthma. Intern Med J. (2019) 49(2):262–4. doi: 10.1111/imj.14208
35. Smith MA, Dinh D, Ly NP, Ward SL, McGarry ME, Zinter MS. Changes in the use of invasive and noninvasive mechanical ventilation in pediatric asthma: 2009–2019. Ann Am Thorac Soc. (2023) 20(2):245–53. doi: 10.1513/AnnalsATS.202205-461OC
36. Schindel CS, Schiwe D, Heinzmann-Filho JP, Campos NE, Pitrez PM, Donadio MVF. Continuous positive airway pressure acutely increases exercise duration in children with severe therapy-resistant asthma: a randomized crossover trial. World J Pediatr. (2021) 17(2):189–96. doi: 10.1007/s12519-021-00425-0
37. David MMC, Gomes E, Mello MC, Costa D. Noninvasive ventilation and respiratory physical therapy reduce exercise-induced bronchospasm and pulmonary inflammation in children with asthma: randomized clinical trial. Ther Adv Respir Dis. (2018) 12:1753466618777723. doi: 10.1177/1753466618777723
38. Nolasco S, Manti S, Leonardi S, Vancheri C, Spicuzza L. High-flow nasal cannula oxygen therapy: physiological mechanisms and clinical applications in children. Front Med. (2022) 9:920549. doi: 10.3389/fmed.2022.920549
39. Ballestero Y, De Pedro J, Portillo N, Martinez-Mugica O, Arana-Arri E, Benito J. Pilot clinical trial of high-flow oxygen therapy in children with asthma in the emergency service. J Pediatr. (2018) 194:204–10.e3. doi: 10.1016/j.jpeds.2017.10.075
40. Vahlkvist S, Jurgensen L, la Cour A, Markoew S, Petersen TH, Kofoed PE. High flow nasal cannula and continuous positive airway pressure therapy in treatment of viral bronchiolitis: a randomized clinical trial. Eur J Pediatr. (2020) 179(3):513–8. doi: 10.1007/s00431-019-03533-2
41. Geng W, Batu W, You S, Tong Z, He H. High-flow nasal cannula: a promising oxygen therapy for patients with severe bronchial asthma complicated with respiratory failure. Can Respir J. (2020) 2020:2301712. doi: 10.1155/2020/2301712
42. Pilar J, Modesto IAV, Lopez-Fernandez YM, Lopez-Macias O, Garcia-Urabayen D, Amores-Hernandez I. High-flow nasal cannula therapy versus non-invasive ventilation in children with severe acute asthma exacerbation: an observational cohort study. Med Intensiva. (2017) 41(7):418–24. doi: 10.1016/j.medin.2017.01.001
Keywords: asthma, children, noninvasive positive-pressure 5entilation, efficacy, meta-analysis
Citation: Dai J, Wang L, Wang F, Wang L and Wen Q (2023) Noninvasive positive-pressure ventilation for children with acute asthma: a meta-analysis of randomized controlled trials. Front. Pediatr. 11:1167506. doi: 10.3389/fped.2023.1167506
Received: 16 February 2023; Accepted: 10 April 2023;
Published: 28 April 2023.
Edited by:
Stijn Verhulst, University of Antwerp, BelgiumReviewed by:
Aroonwan Preutthipan, Mahidol University, Thailand© 2023 Dai, Wang, Wang, Wang and Wen. This is an open-access article distributed under the terms of the Creative Commons Attribution License (CC BY). The use, distribution or reproduction in other forums is permitted, provided the original author(s) and the copyright owner(s) are credited and that the original publication in this journal is cited, in accordance with accepted academic practice. No use, distribution or reproduction is permitted which does not comply with these terms.
*Correspondence: Qingfen Wen d3FmMjUxQDE2My5jb20=
Disclaimer: All claims expressed in this article are solely those of the authors and do not necessarily represent those of their affiliated organizations, or those of the publisher, the editors and the reviewers. Any product that may be evaluated in this article or claim that may be made by its manufacturer is not guaranteed or endorsed by the publisher.
Research integrity at Frontiers
Learn more about the work of our research integrity team to safeguard the quality of each article we publish.