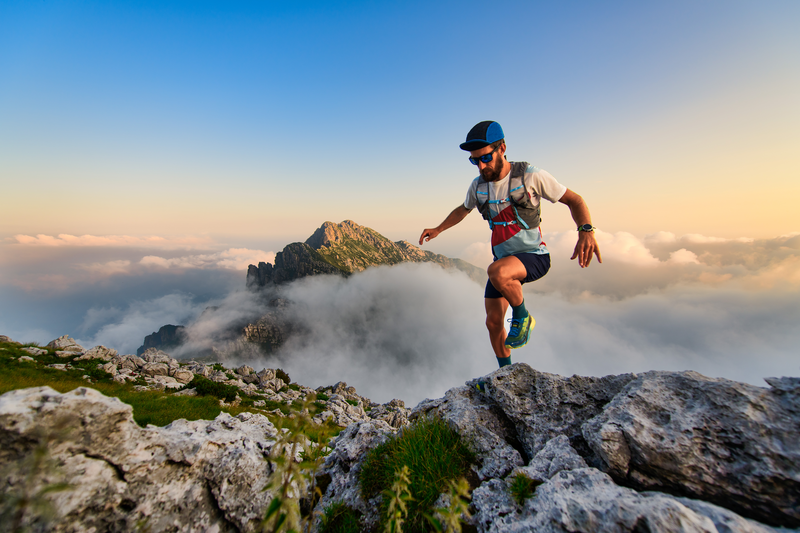
95% of researchers rate our articles as excellent or good
Learn more about the work of our research integrity team to safeguard the quality of each article we publish.
Find out more
ORIGINAL RESEARCH article
Front. Pediatr. , 24 November 2023
Sec. Neonatology
Volume 11 - 2023 | https://doi.org/10.3389/fped.2023.1151000
This article is part of the Research Topic Neonatal Sepsis: Current Insights and Challenges View all 11 articles
Introduction: Early diagnosis of infections and sepsis is essential as adequate therapy improves the outcome. Unfortunately, current diagnostics are invasive and time-consuming, making diagnosis difficult, especially in neonatology. Novel non-invasive analytical methods might be suitable to detect an infection at an early stage and might even allow identification of the pathogen. Our aim is to identify specific profiles of volatile organic compounds (VOCs) of bacterial species.
Methods: Using multicapillary column-coupled ion mobility spectrometry (MCC/IMS), we performed headspace measurements of bacterial cultures from skin and anal swabs of premature infants obtained during weekly screening for bacterial colonization according to KRINKO. We analyzed 25 Klebsiella pneumoniae (KP) cultures on MacConkey (MC) agar plates, 25 Klebsiella oxytoca (KO) cultures on MC agar and 25 bare MC agar plates as a control group.
Results: Using MCC/IMS, we identified a total of 159 VOC peaks. 85 peaks allowed discriminating KP and bare MC agar plates, and 51 peaks comparing KO and bare MC agar plates and 6 peaks between KP and KO (significance level of p < 0.05 after Bonferroni post hoc analysis), respectively. Peaks P51 (n-Decane) and P158 (Phenylethyl Alcohol), showed the best sensitivity/specificity/ positive predictive value/negative predictive value of 99.9% each (p < 0.001) for KP. P158 showed the best sensitivity/specificity/positive predictive value/negative predictive value of 99.9% each (p < 0.001) for KO. Comparing KP and KO, best differentiation was enabled using peaks P72, P97 and P16 with sensitivity/specificity/positive predictive value/negative predictive value of 76.0%, 84.0%, 82.6%, 77.8%, respectively (p < 0.05).
Discussion: We developed a method for the analysis of VOC profiles of bacteria. Using MCC/IMS, we demonstrated that VOCs derived from bacteria are clearly distinguishable from a bare agar plate. Characteristic peaks obtained by MCC/IMS are particularly suitable for the species-specific identification and differentiation of KP and KO. Thus, MCC/IMS might be a useful tool for in vitro diagnostics. Future studies must clarify whether similar patterns of VOCs can be detected in vivo in patients that are colonized or infected with KP or KO to enable rapid and accurate diagnosis of bacterial colonization.
Prematurity includes a state of immaturity of all organ systems including the immune system. Thus, preterm infants are at high risk for nosocomial infections. Therefore, weekly colonization screening is recommended by German Commission on Hospital Hygiene and Infection Protection (KRINKO) at the Robert Koch Institute (RKI) since 2013 as bloodstream infections and bacterial colonization pose a significant risk of morbidity and mortality for preterm infants (1). As infections of preterm infants often manifest with nonspecific symptoms, diagnosis is difficult and the time window to achieve adequate diagnosis and treatment is short. The existing diagnostic tests require painful procedures and are often linked to high costs and to limited sensitivity. Presently, acute inflammatory markers provide limited sensitivity, especially at the onset of an infection. Time consuming laboratory tests can delay adequate treatment for hours triggering empiric antibiotic treatment before knowing the laboratory results. A fast and real-time diagnostic tool could reduce unnecessary exposure to antibiotics in suspected, but unconfirmed sepsis. Rapid diagnosis and prompt initiation of therapy significantly improves outcome (2–4).
The analysis of volatile organic compounds (VOCs) represents an innovative approach for non-invasive diagnostics (5). VOCs can be produced by the host or by microbes and are emitted via body secretions or breath. Alterations in specific VOC profiles are linked to perinatal or neonatal diseases (6). Previous studies revealed the high potential of VOC analysis and its application for non-invasive diagnostics (6–9). We have developed a method using ion mobility spectrometer coupled to multi capillary columns (MCC/IMS) to measure VOC profiles and to assign individual VOCs to biochemical markers using a reference data set (BS-MCC/IMS-analyses database). Using MCC/IMS, characteristic VOC profiles can be detected in the incubator atmosphere of neonates (10). Moreover, using VOC analysis via MCC/IMS, we found that 5-methyl pentane as a potential biomarker for chorioamnionitis, a common cause of prematurity (11).
Bacteria can also produce VOCs; some VOCs originate exclusively from certain bacterial species, and analysis of VOCs profiles enables distinguishing between several bacterial species (9, 12, 13). Also, there are changes in VOCs profiles of neonates suffering from late-onset sepsis (14). Common healthcare-associated pathogens are Gram-negative bacteria such as Klebsiella pneumoniae (KP) and Klebsiella oxytoca (KO). The detection of KP is of the utmost importance as it can cause neonatal infections and sepsis, which may be difficult to treat due to the prevalence of multi-drug resistant strains (15–19). Similarly, KO can acquire antibiotic resistance and cause outbreaks in neonatal intensive care units (NICU) (20–22). The occurrence of KO is linked to antibiotic associated hemorrhagic colitis (23) and might be associated with necrotizing enterocolitis (24).
The aim of this study was to establish headspace measurements of KP and KO independent from ambient air for measuring and distinguishing bacterial cultures originating from routine anal/rectal swabs of preterm infants using MCC/IMS as a novel noninvasive, rapid and precise method.
This study was performed at the Department of Pediatrics, Saarland University Medical Center, Homburg (Germany) and at the Institute of Medical Microbiology and Hygiene, Saarland University, Homburg (Germany) after approval by the Ethical committee Saarland (reference 276/17) from April 2021 to October 2021. All acquired data were recorded and processed in an anonymized form.
Microbial cultures of KP and KO on MacConkey (MC) agar plates (Becton, Dickinson and Company, Franklin Lakes, New Jersey, USA) originated from rectal/anal swabs of preterm infants of the NICU of the Department of Pediatrics, Saarland University Medical Center, Homburg (Germany). The swabs were taken twice a week as part of the routine screening on bacterial colonization. Swabs were transferred in eSwab™ transport medium (Copan, Brescia, Italy). In the Institute of Medical Microbiology and Hygiene, Saarland University, Homburg (Germany), swabs were spread out onto MC agar plates. Only those agar plates with growth of KP and KO cultures were included in this study. Following a Standard operating procedure (SOP), samples were processed as follows: a part of the agar with bacterial colonies on it (size: 0.5 × 0.5 cm) was cut out and placed into a laboratory bottle.
To perform headspace measurements of VOCs derived from bacterial colonies, we used an MCC/IMS BreathDiscovery (B & S Analytik GmbH, Dortmund, Germany). It was placed on a metal cart with a laptop computer and connected to a synthetic air supply. The pre-separation was performed using an OV-5 (5% - diphenyl, 95% - dimethylpolysiloxane) multi-capillary column (MCC) (Multichrom Ltd., Novosibirsk, Russia). The device and sampling parameters are given in Supplementary Table 1. The methods for VOC analysis were published earlier. A laboratory bottle (100 ml) heated to 37°C served as a sample container. A closed system was established: A large laboratory bottle (1,000 ml, gas reservoir, Schott Duran®, DURAN Group GmbH, Wertheim /Main, Germany) was connected to the small bottle (100 ml, Schott Duran®, DURAN Group GmbH, Wertheim /Main, Germany) via a perfluoroalkoxyalkane (PFA) tube that was led through the caps. Both bottles were filled with synthetic air as carrier gas. Another tube connected the cap of the small laboratory bottle and the sampling input of MCC/IMS device (Figure 1). Data was acquired using VOCan v3.7 (B & S Analytik GmbH, Dortmund, Germany). The standard operation conditions are used as recommended by the supplier of the instrument. IMS was regularly calibrated using standardized reference mixture (“R06” calibration liquid, B & S Analytik GmbH, Dortmund, Germany, Supplementary Table 2).
Figure 1. Experimental design. We used an MCC/IMS BreathDiscovery (B & S Analytik GmbH, Dortmund, Germany) to perform headspace measurements of VOCs derived from the fecal und urine samples. (1) Laptop; (2) MCC/IMS BreathDiscovery (B & S Analytik GmbH, Dortmund, Germany); (3) Connecting tube between MCC/IMS and synthetic air supply gas bottle; (4) Connecting tube between MCC/IMS and sample container; (5) Connecting tube between gas reservoir and sample container; (6) Water bath; (7) Sample container: small laboratory bottle (100 ml); (8) Diaper sample; (9) Gas reservoir: large laboratory bottle (1,000 ml); (10) Connecting tube between synthetic air supply gas bottle and gas reservoir; (11) Synthetic air supply gas bottle; PFA tubes (green) as drift gas supply; PFA tube (grey) as sample input. Data acquisition was directed and recorded using VOCan v3.7 (B & S Analytik GmbH, Dortmund, Germany).
We evaluated the data acquired by MCC/IMS using the software VisualNow 3.7 (B & S Analytik GmbH, Dortmund, Germany). All peaks were characterized by their specific combination of retention time and drift time (corresponding 1 / K0-value, see Supplementary Table 3). The databank layer (BS-MCC/IMS-analyses database) was used for peak referencing and determination of retention times and 1/K0-values. Here, pure analytes were measured 10 times each and comparison with parallel measurements using GC/MS standard procedures was performed (25, 26). Peak intensity (in volts) was considered as an indirect measure of compound concentration. A specific threshold was calculated for each peak and comparison. Box-and-Whisker plots and a rank sum test using Mann-Whitney-U test and Bonferroni post hoc analysis correction were performed. The n-value was set at n = 25 for KP, n = 25 for KO and n = 25 for bare MC agar plates. The α-level was defined to be 0.05, the p-value (one-tailed) was determined to be <0.05. Significant peaks [p < 0.05, 95% confidential interval (CI)] were used for further evaluation with decision trees (DT).
We analyzed a total of 75 agar plates with KP (n = 25) or KO (n = 25) and bare MC agar plates (n = 25), respectively. We identified 159 signals (peaks) from each comparison such as 85 peaks between bare MC agar plates and KP, 51 peaks between bare MC agar plates and KO and 6 between KP and KO, respectively. We found 148 peaks exclusively assigned to KO or KP and 11 peaks exclusively assigned to bare MC agar plates. 17 peaks occurred in all three groups (MC/KO/KP) and did not differ significantly between the individual groups. When comparing KP and bare MC agar plates, peak P51 and P158 showed the highest sensitivity, specificity, positive and negative predictive value after Bonferroni post hoc analysis correction (sensitivity and specificity 99.9%, respectively, p < 0.001) for KP. Comparing KO and bare MC agar plates peak P158 reaching the highest sensitivity, specificity, positive and negative predictive value of 99.9% each in a significance level with p < 0.001 (Figure 2). Regarding the comparison between KO and KP, best differentiation was enabled using peaks P72, P97 and P16 with sensitivity, specificity and positive and negative predictive value of 76.0%, 84.0%, 82.6%, 77.8%, respectively in a significance level with p < 0.05 after Bonferroni post hoc analysis correction (Table 1).
Figure 2. Box-and-whisker-Plot of the peaks P158 (A) and P51 (B). After Bonferroni post hoc analysis correction, Peaks P51 and P158 showed the highest sensitivity, specificity, positive and negative predictive value with 99.9% each in a significance level with p < 0.001 for K. pneumoniae (KP) when comparing KP and bare MacConkey agar plates (MC). Comparing K. oxytoca (KO) and MC P158 reaching the highest sensitivity, specificity, positive and negative predictive value of 99.9% each in a significance level with p < 0.001.
We established a decision tree using three peaks that enabled the differentiation of KP, KO and bare MC agar plates regarding their signal intensities. The peak P158 allows to differentiate between bare MC agar plates (with a signal intensity ≤0.011 V), KP and KO (with a signal intensity >0.011 V), respectively. Regarding signal intensities >0.487 V, KP is identified via P108. The Peak P22 enables further differentiation regarding a signal intensity ≤0.487 V: a signal intensity ≤0.010 V stands for KO and a signal intensity >0.005 V for KP (Figure 3).
Figure 3. Decision tree. A total of three peaks enabled the differentiation of K. pneumoniae (KP), K. oxytoca (KO) and bare MacConkey agar plates (MC) regarding their signal intensities. With a signal intensity ≤0.011 V, P158 allows to differentiate between bare MC, KP and KO, respectively. At a signal intensitiy >0.487 V, KP is identified via P108. Peak P22 enables further differentiation regarding a signal intensity ≤0.487 V: a signal intensity ≤0.010 V represents KO and a signal intensity >0.005 V represents KP.
Using our standardized database (B. Braun Melsungen-Database/ BS-MCC/IMS-analyses database), two peaks were assigned to biochemical substances: P51 was assigned to n-Decane (CAS number 124-18-5) and P158 to Phenylethyl Alcohol (CAS number 60-12-8).
Premature babies show an increased susceptibility to nosocomial infections. Gold standard to verify bacterial bloodstream infections are blood cultures which yield results 24–48 h or even later (27–29). Rapid diagnosis and prompt initiation of therapy significantly improves outcome (2–4). In the present study we showed that KP and KO can be distinguished based on their VOC profile using MCC/IMS. As electronic nose devices detect patterns of VOC profiles, we chose MCC/IMS to precisely identify substances. In our study, we identified two signals that could be assigned to substances using a database (B. Braun Melsungen-Database/GC/MS-MCC/IMS-analyses database): n-Decane and Phenylethyl Alcohol. Phenylethyl Alcohol is considered a metabolite in the Ehrlich Pathway and might derive from Klebsiella spp. Our future aim is to enable early diagnosis of neonatal infections by means of alterations in the VOC profile of neonates suffering from blood stream infections to be able to initiate quickly a targeted antibiotic therapy. Therefore, in the first step, we created the bacteria-specific VOC profiles using MCC-IMS in agar plates deriving from routine swabs. The next step will be the analysis of skin swabs themselves without any cultivation to differentiate KO/KP swabs from commensal bacteria – later we plan to perform measurement directly inside the incubator. As bacterial colonization is a risk factor for invasive infections, it would be favorable to quickly know about the respective colonization status without performing potential stressful swabs. If this is successful, we can focus on detecting infections in future studies.
VOC detection studies showed the great potential of VOC analysis with a wide array of techniques like breath analysis, headspace measurement of bio samples and the measurement of incubators atmosphere (7, 8, 10, 30). VOC analysis can be performed by several methods, e.g., gas chromatography coupled with mass spectrometry (GC-MS), gas chromatography time of flight- mass spectrometry (GC-ToF-MS), selected ion flow tube- mass spectrometry (SIFT-MS) and ion molecule reaction mass spectrometry (IMR-MS). Obtaining time series every 15 min is an advantage of MCC/IMS, especially in comparison to different mass spectrometric methods. The moisture content (e.g., exhaled breath samples) is a major limitation for most analytical methods, but not for MCC/IMS. On the other hand, bed side and on-site applications of MCC/IMS were reported since 2017 as medical products based on MCC/IMS technology were introduced into the market. Electronic nose devices using sensor technologies are based on pattern recognition and measurements can be conducted easily and at the bedside. As those devices do not allow qualitative VOC analysis, we decided to use an ion mobility spectrometer coupled to multi capillary columns (MCC/IMS) allowing the measurement of VOC profiles and the specification of individual VOCs using a reference data set. MCC/IMS analyses provide results within a few minutes and could therefore be used for instant bedside diagnostics. Electronic noses have been used to distinguish several bacterial strains from each other in vitro (9). P. aeruginosa was detected in human sputum and in culture via gas chromatography-mass spectrometry (GC-MS) (31). Using MCC/IMS Junger et al. showed it was also possible to distinguish individual bacterial strains based on their VOCs in pure cultures (13). We conclude that treatment and diagnostics on NICU are often linked to invasive and time-consuming procedures. Establishing a noninvasive, painless method by VOC analysis would be best for vulnerable patients like preterm infants. We were the first study to use routine swabs from preterm infants and to analyze grown pathogens on agar plates. We analyzed grown agar plates, but also empty agar plates for adjustment. Incubation of inoculated agar plates took place in microbiological incubators. In preliminary work, we also incubated empty agar plates to rule out influences of the incubator storage on the VOC profile. Further strengths of our study are that our measurements can be conducted quickly within a few minutes, even bedside diagnostics are feasible (10). MCC/IMS allows to detect substances with extremely low concentrations (pg/L) (5, 32) which enables detecting weak alterations in the VOC profile. However, due to the high sensitivity of the MCC/IMS, there is a high risk that ethanol and other environmental VOCs will interfere with the detection of VOCs that would be important for detection purposes, including bacteria. Our method uses a closed system to avoid influences by ambient air and other environmental influences. For this method, especially when developed further so it can be run without the need for an overnight culture, we would not need further consumables besides the costs for the synthetic air. Thus, this approach has the potential to be cost-effective and ecologically sustainable. Nevertheless, the present study was performed under laboratory conditions which may differ from the clinical setting. An inconvenience of our study is the measurement of bacterial colonies (KP/KO) on agar plates. The cultivation of the bacteria still takes time. For this reason, additional studies are planned to allow direct analyses of standard swabs taken from preterm infants. At this point, it is unclear if we can distinguish bacterial colonization from invasive infections via VOC analysis, these conditions may differ regarding their VOC profile based on different inflammatory responses. As bacterial colonies originate from swabs, there is an uncertainty about pure cultures of bacteria. A higher number of swab samples and measurements must be considered. Klebsiella spp. are a common cause of neonatal sepsis and they frequently carry resistance genes that render them multidrug-resistant (33). Currently, antibiotic susceptibility testing using electronic nose devices is still unproven. Hence, bacterial culture linked to antibiotic susceptibility testing currently remains gold standard. Under clinical conditions, influences like oral hygiene, food habits/nutrition, age and gender were found to influence VOC profile. Nevertheless, environmental influences could be reduced following standard operating procedures.
We showed that KP and KO can be distinguished based on their VOC profile using MCC/IMS. We achieved a first step to provide a supportive tool that might quickly detect bacterial colonization on NICU.
The raw data supporting the conclusions of this article will be made available by the authors, without undue reservation.
The studies involving humans were approved by Ethics Committee at the Saarland Medical Association AZ: 276 /17. The studies were conducted in accordance with the local legislation and institutional requirements. Written informed consent for participation in this study was provided by the participants’ legal guardians/next of kin.
MB: conception and design, data analysis and interpretation, manuscript writing, final approval of manuscript. MT: collection and assembly of data, data analysis and interpretation, manuscript writing, final approval of manuscript. CP: coordination of sample collection, manuscript writing, final approval of manuscript. EK, RW: manuscript writing, final approval of manuscript. JB: data analysis and interpretation, manuscript writing, final approval of manuscript. SB: coordination of sample collection, manuscript writing, final approval of manuscript. MZ: conception and design, data analysis and interpretation, financial support, manuscript writing, final approval of manuscript. SG-F: conception and design, data analysis and interpretation, manuscript writing, final approval of manuscript. All authors contributed to the article and approved the submitted version.
Funded by a grant from the HOMFOR Foundation of Saarland University Medical School, by the Else-Kröner-Fresenius Stiftung, by the Staatskanzlei Saarbrücken, by Centre of Digital Neurotechnologies Saar, by Werner-Zeh-Stiftung and by the BMBF (PRIMAL Clinical Study FKZ: 01GL1746D).
We thank Ellen Maurer, Stefanie Lenz, Nina Bühler and Monika Schmitt for their excellent technical help.
The authors declare that the research was conducted in the absence of any commercial or financial relationships that could be construed as a potential conflict of interest.
The handling editor HN declared a past co-authorship with the author MB, EK, RW, JB, MZ, and SF.
All claims expressed in this article are solely those of the authors and do not necessarily represent those of their affiliated organizations, or those of the publisher, the editors and the reviewers. Any product that may be evaluated in this article, or claim that may be made by its manufacturer, is not guaranteed or endorsed by the publisher.
The Supplementary Material for this article can be found online at: https://www.frontiersin.org/articles/10.3389/fped.2023.1151000/full#supplementary-material
1. Graham PL, Della-Latta P, Wu F, Zhou J, Saiman L. The gastrointestinal tract serves as the reservoir for gram-negative pathogens in very low birth weight infants. Pediatr Infect Dis J. (2007) 26(12):1153–6. doi: 10.1097/INF.0b013e31814619d4
2. Flidel-Rimon O, Leibovitz E, Eventov Friedman S, Juster-Reicher A, Shinwell ES. Is lumbar puncture (LP) required in every workup for suspected late-onset sepsis in neonates? Acta Paediatr. (2011) 100(2):303–4. doi: 10.1111/j.1651-2227.2010.02012.x
3. Raju TN, Higgins RD, Stark AR, Leveno KJ. Optimizing care and outcome for late-preterm (near-term) infants: a summary of the workshop sponsored by the national institute of child health and human development. Pediatrics. (2006) 118(3):1207–14. doi: 10.1542/peds.2006-0018
4. Cohen-Wolkowiez M, Moran C, Benjamin DK, Cotten CM, Clark RH, Benjamin DK Jr, et al. Early and late onset sepsis in late preterm infants. Pediatr Infect Dis J. (2009) 28(12):1052–6. doi: 10.1097/inf.0b013e3181acf6bd
5. Cumeras R, Figueras E, Davis CE, Baumbach JI, Gràcia I. Review on ion mobility spectrometry. Part 1: current instrumentation. Analyst. (2015) 140(5):1376–90. doi: 10.1039/c4an01100g
6. Deianova N, El Manouni El Hassani S, Struijs EA, Jansen EEW, Bakkali A, van de Wiel MA, et al. Fecal amine metabolite analysis before onset of severe necrotizing enterocolitis in preterm infants: a prospective case-control study. Sci Rep. (2022) 12(1):12310. doi: 10.1038/s41598-022-16351-8
7. Darwiche K, Baumbach JI, Sommerwerck U, Teschler H, Freitag L. Bronchoscopically obtained volatile biomarkers in lung cancer. Lung. (2011) 189(6):445–52. doi: 10.1007/s00408-011-9324-1
8. Buchinger H, Kreuer S, Hellbrück R, Wolf A, Fink T, Volk T, et al. Minimal retarded propofol signals in human breath using ion mobility spectrometry. Int J Ion Mobil Spectrom. (2013) 16(3):185–90. doi: 10.1007/s12127-012-0118-9
9. Saviauk T, Kiiski JP, Nieminen MK, Tamminen NN, Roine AN, Kumpulainen PS, et al. Electronic nose in the detection of wound infection bacteria from bacterial cultures: a proof-of-principle study. Eur Surg Res. (2018) 59(1–2):1–11. doi: 10.1159/000485461
10. Steinbach J, Goedicke-Fritz S, Tutdibi E, Stutz R, Kaiser E, Meyer S, et al. Bedside measurement of volatile organic compounds in the atmosphere of neonatal incubators using ion mobility spectrometry. Front Pediatr. (2019) 7:248. doi: 10.3389/fped.2019.00248
11. Goedicke-Fritz S, Werner T, Niemarkt HJ, Wolfs TGAM, Baumbach JI, Kemp MW, et al. Detection of volatile organic compounds as potential novel biomarkers for chorioamnionitis - proof of experimental models. Front Pediatr. (2021) 9:698489. doi: 10.3389/fped.2021.698489
12. Bos LD, Sterk PJ, Schultz MJ. Volatile metabolites of pathogens: a systematic review. PLoS Pathog. (2013) 9(5):e1003311. doi: 10.1371/journal.ppat.1003311
13. Junger M, Vautz W, Kuhns M, Hofmann L, Ulbricht S, Baumbach JI, et al. Ion mobility spectrometry for microbial volatile organic compounds: a new identification tool for human pathogenic bacteria. Appl Microbiol Biotechnol. (2012) 93(6):2603–14. doi: 10.1007/s00253-012-3924-4
14. Frerichs NM, El Manouni El Hassani S, Deianova N, van Weissenbruch MM, van Kaam AH, Vijlbrief DC, et al. Fecal volatile metabolomics predict gram-negative late-onset sepsis in preterm infants: a nationwide case-control study. Microorganisms. (2023) 11(3):572. doi: 10.3390/microorganisms11030572
15. Huang W, Wang G, Sebra R, Zhuge J, Yin C, Aguero-Rosenfeld ME, et al. Emergence and evolution of multidrug-resistant Klebsiella pneumoniae with both bla(KPC) and bla(CTX-M) integrated in the chromosome. Antimicrob Agents Chemother. (2017) 61(7):e00076-17. doi: 10.1128/aac.00076-17
16. You T, Zhang H, Guo L, Ling K-R, Hu X-Y, Li L-Q. Differences in clinical characteristics of early- and late-onset neonatal sepsis caused by Klebsiella pneumoniae. Int J Immunopathol Pharmacol. (2020) 34:2058738420950586. doi: 10.1177/2058738420950586
17. Zellweger RM, Carrique-Mas J, Limmathurotsakul D, Day NPJ, Thwaites GE, Baker S, et al. A current perspective on antimicrobial resistance in Southeast Asia. J Antimicrob Chemother. (2017) 72(11):2963–72. doi: 10.1093/jac/dkx260
18. Fox-Lewis A, Takata J, Miliya T, Lubell Y, Soeng S, Sar P, et al. Antimicrobial resistance in invasive bacterial infections in hospitalized children, Cambodia, 2007-2016. Emerg Infect Dis. (2018) 24(5):841–51. doi: 10.3201/eid2405.171830
19. Crellen T, Turner P, Pol S, Baker S, Nguyen Thi Nguyen T, Stoesser N, et al. Transmission dynamics and control of multidrug-resistant Klebsiella pneumoniae in neonates in a developing country. Elife. (2019) 8:e50468. doi: 10.7554/eLife.50468
20. Herruzo R, Ruiz G, Gallego S, Diez J, Sarria A, Omeñaca F. VIM-Klebsiella oxytoca outbreak in a neonatal intensive care unit. This time it wasn't the drain. J Prev Med Hyg. (2017) 58(4):E302–7. doi: 10.15167/2421-4248/jpmh2017.58.4.692
21. Lowe C, Willey B, O'Shaughnessy A, Lee W, Lum M, Pike K, et al. Outbreak of extended-spectrum β-lactamase-producing Klebsiella oxytoca infections associated with contaminated handwashing sinks(1). Emerg Infect Dis. (2012) 18(8):1242–7. doi: 10.3201/eid1808.111268
22. Leitner E, Zarfel G, Luxner J, Herzog K, Pekard-Amenitsch S, Hoenigl M, et al. Contaminated handwashing sinks as the source of a clonal outbreak of KPC-2-producing Klebsiella oxytoca on a hematology ward. Antimicrob Agents Chemother. (2015) 59(1):714–6. doi: 10.1128/aac.04306-14
23. Högenauer C, Langner C, Beubler E, Lippe IT, Schicho R, Gorkiewicz G, et al. Klebsiella oxytoca as a causative organism of antibiotic-associated hemorrhagic colitis. N Engl J Med. (2006) 355(23):2418–26. doi: 10.1056/NEJMoa054765
24. Paveglio S, Ledala N, Rezaul K, Lin Q, Zhou Y, Provatas AA, et al. Cytotoxin-producing Klebsiella oxytoca in the preterm gut and its association with necrotizing enterocolitis. Emerg Microbes Infect. (2020) 9(1):1321–9. doi: 10.1080/22221751.2020.1773743
25. Jünger M, Bödeker B, Baumbach JI. Peak assignment in multi-capillary column–ion mobility spectrometry using comparative studies with gas chromatography–mass spectrometry for VOC analysis. Anal Bioanal Chem. (2010) 396(1):471–82. doi: 10.1007/s00216-009-3168-z
26. Perl T, Bödeker B, Jünger M, Nolte J, Vautz W. Alignment of retention time obtained from multicapillary column gas chromatography used for VOC analysis with ion mobility spectrometry. Anal Bioanal Chem. (2010) 397(6):2385–94. doi: 10.1007/s00216-010-3798-1
27. Lee A, Mirrett S, Reller LB, Weinstein MP. Detection of bloodstream infections in adults: how many blood cultures are needed? J Clin Microbiol. (2007) 45(11):3546–8. doi: 10.1128/jcm.01555-07
28. Nieman AE, Savelkoul PHM, Beishuizen A, Henrich B, Lamik B, MacKenzie CR, et al. A prospective multicenter evaluation of direct molecular detection of blood stream infection from a clinical perspective. BMC Infect Dis. (2016) 16:314. doi: 10.1186/s12879-016-1646-4
29. Kumar Y, Qunibi M, Neal TJ, Yoxall CW. Time to positivity of neonatal blood cultures. Arch Dis Child Fetal Neonatal Ed. (2001) 85(3):F182–6. doi: 10.1136/fn.85.3.f182
30. Perl T, Carstens E, Hirn A, Quintel M, Vautz W, Nolte J, et al. Determination of serum propofol concentrations by breath analysis using ion mobility spectrometry. Br J Anaesth. (2009) 103(6):822–7. doi: 10.1093/bja/aep312
31. Neerincx AH, Geurts BP, Habets MF, Booij JA, van Loon J, Jansen JJ, et al. Identification of Pseudomonas aeruginosa and Aspergillus fumigatus mono- and co-cultures based on volatile biomarker combinations. J Breath Res. (2016) 10(1):016002. doi: 10.1088/1752-7155/10/1/016002
32. Baumbach JI. Process analysis using ion mobility spectrometry. Anal Bioanal Chem. (2006) 384(5):1059–70. doi: 10.1007/s00216-005-3397-8
Keywords: volatile organic compounds, ion mobility spectrometry, premature infant, biomarkers, klebsiella pneumoniae, detection, pediatrics, non-invasive diagnostics
Citation: Bous M, Tielsch M, Papan C, Kaiser E, Weber R, Baumbach JI, Becker SL, Zemlin M and Goedicke-Fritz S (2023) Detection of volatile organic compounds in headspace of Klebsiella pneumoniae and Klebsiella oxytoca colonies. Front. Pediatr. 11:1151000. doi: 10.3389/fped.2023.1151000
Received: 25 January 2023; Accepted: 6 November 2023;
Published: 24 November 2023.
Edited by:
Hendrik Niemarkt, Máxima Medical Center, NetherlandsReviewed by:
Guido Voets, Check-Points B.V., Netherlands© 2023 Bous, Tielsch, Papan, Kaiser, Weber, Baumbach, Becker, Zemlin and Goedicke-Fritz. This is an open-access article distributed under the terms of the Creative Commons Attribution License (CC BY). The use, distribution or reproduction in other forums is permitted, provided the original author(s) and the copyright owner(s) are credited and that the original publication in this journal is cited, in accordance with accepted academic practice. No use, distribution or reproduction is permitted which does not comply with these terms.
*Correspondence: Sybelle Goedicke-Fritz c3liZWxsZS5nb2VkaWNrZS1mcml0ekB1a3MuZXU=
Abbreviations CI, confidential interval; DT, decision tree; IMS, ion mobility spectrometry; KO, Klebsiella oxytoca; KP, Klebsiella pneumoniae; MC, MacConkey; MCC, multi-capillary column; MCC/IMS, ion mobility spectrometer coupled to multi-capillary column; NICU, neonatal intensive care unit; P, peak; PFA, perfluoroalkoxyalkane; VOCs, volatile organic compounds.
Disclaimer: All claims expressed in this article are solely those of the authors and do not necessarily represent those of their affiliated organizations, or those of the publisher, the editors and the reviewers. Any product that may be evaluated in this article or claim that may be made by its manufacturer is not guaranteed or endorsed by the publisher.
Research integrity at Frontiers
Learn more about the work of our research integrity team to safeguard the quality of each article we publish.