- 1Department of Pediatrics, Longgang Maternity and Child Institute of Shantou University Medical College (Longgang District Maternity & Child Healthcare Hospital of Shenzhen City), Shenzhen, China
- 2Department of Computer Science, City University of Hong Kong, Hong Kong, Hong Kong SAR, China
- 3Department of Pediatrics, The People’s Hospital of Shenzhen Baoan District, Shenzhen, China
- 4School of Statistics and Data Science, NanKai University, Tianjin, China
- 5Department of Pediatrics, Affiliated Shenzhen Maternity and Child Healthcare Hospital, Southern Medical University, Shenzhen, China
Objective: To study changes in the composition and functions of the gut microbiota (GM) in children with growth hormone deficiency (GHD) using high-throughput sequencing.
Methods: Thirty-three children with GHD diagnosed in Longgang District Maternity and Child Health Hospital were included in the disease group and 24 healthy children of the same age comprised the control group. Total DNA was extracted and amplified from stool samples obtained from all subjects. High-throughput sequencing was used to analyze the GM composition and functions.
Results: The GM from the two groups of children showed significant differences in α-diversity (P < 0.05). In comparison with the control group, the abundance of the phylum Bacteroidetes was significantly higher (45.96% vs. 65.71%) while the Firmicutes count was significantly lower (47.09% vs. 25.20%). At the genus level, the abundance of Prevotella in the disease group was significantly higher (3.16% vs. 20.67%) and that of Lachnospiracea incertae sedis, Clostridium XlVa, and Megamonas was lower (6.576% vs. 1.75%; 4.51% vs. 0.80%; 5.08% vs. 2.02%, respectively). GM functions, including those involved in membrane_transport, energy_metabolism, poorly_characterized, metabolism_of_cofactors_and_vitamins, glycan_biosynthesis_and_metabolism, transcription, folding,_sorting,_and_degradation, were significantly altered in the disease group. The abundance of various GM components was correlated with endocrine hormone levels.
Conclusion: Significant alterations in the GM are seen in children with growth hormone deficiency, which may affect both energy metabolism and the levels of endocrine hormones, potentially leading to growth restriction.
1. Introduction
Short stature is defined as a height of less than two standard deviations or less than the third percentile among children of the same sex, age, or race. Growth hormone deficiency (GHD) is a growth disorder caused by reduced or absent production of growth hormone (GH). It is one of the most common causes of short stature in children, accounting for 38.6% of all causes (1). The worldwide incidence of GHD in children varies between 1/4,000 and 1/10,000 and most children show idiopathic GHD (2).
The stability of the gut microbiota (GM) is an important factor influencing the growth and development of children (3). Intestinal microorganisms and metabolites such as short-chain fatty acids (SCFAs) can regulate the production of hormones related to bone health, including sex steroids, vitamin D, and serotonin (4, 5). In addition, they mediate signal transduction via the intestinal–brain axis and affect the secretion of GH-releasing peptide, somatostatin, and leptin, all of which regulate the GH/insulin-like growth factor-1 (IGF-1) axis and modulate processes such as GH secretion, appetite regulation, and bone growth (1, 6–10). Growth hormone can not only directly promote the growth of all organs but also stimulate the production of IGF-1. The latter is an effective growth factor that plays a synergistic role with growth hormone to maintain overall growth and metabolism (11, 12). Conversely, GH or IGF-1 can also affect the composition and functions of the GM in different ways (1). Li et al. (13) reported significant changes in the GM of children with idiopathic short stature where intestinal Clostridium and Eubacterium were significantly and positively correlated with their height standard deviation score (SDS) and IGF-1 SDS. The authors believed that the decrease in IGF-1 synthesis by Clostridium and Eubacterium through SCFAs might be one of the underlying causes.
The hypothalamus–pituitary–IGF-1 axis is the main hormonal regulator of growth and development, of which GH and IGF-1 are key components (14). GHD children have reduced levels of GH and IGF-1. Imbalances in the GM can lead to endocrine hormone disorders. We speculate that children with GHD may also have GM imbalances. In this study, the intestinal composition and function of GHD children and healthy children of the same age were compared, and correlations between their GM and several hormones were analyzed to explore the characteristics of the GM of GHD children and the possible mechanism of action.
2. Materials and methods
2.1. Sample screening
We selected 33 children with GHD diagnosed at Longgang District Maternity and Child Health Hospital as the disease group, and 24 healthy children of the same age as the control group. The ages of children in the two groups ranged between 5 and 14 years, with no statistical difference seen in the comparative analysis (P > 0.05) (Table 1). All the children with GHD were diagnosed at the Department of Growth and Development, Shenzhen Longgang District Maternity and Child Health Hospital. The disease group met the diagnostic criteria for GHD in Chinese children (15): ① Below the third percentile of the height of normal healthy children of the same age and sex (−1.88 standard deviations [−1.88 SD] or minus 2 standard deviations [−2 SD]); ② Annual growth rate <5 cm/year; ③ Symmetrical dwarfism and childish face; ④ Normal intelligence development; ⑤ Bone age lagging behind actual age; ⑥ Peak values of two GH drug provocation tests of <10 µg/L; ⑦ Lower than normal level of serum IGF-1. The exclusion criteria for children in the two groups included: ① Severe liver or gastrointestinal disorders; ② Severe infection; ③ Treatment with antibiotics or probiotic preparations within one month before the test. All children provided informed consent from their guardians before enrollment.
2.2. Sample handling and species annotation
2.2.1. Collection of fecal samples for DNA extraction and sequencing from two groups of children
Approximately 5 g of the middle section of the feces was collected and immediately frozen and stored at ‒80°C. The samples were transported on dry ice to Shenzhen Micro Health Gene Technology Co., Ltd. for high-throughput sequencing. MoBio's PowerSoil® DNA Isolation Kit was used to extract bacterial DNA from fecal samples. Amplification of the V3 – V4 region of the 16S rRNA gene in DNA was performed by polymerase chain reaction (PCR). Amplified samples were sequenced using the Illumina MiSeq high-throughput sequencing platform.
2.2.2. Sequencing data analysis
Low-quality reads were filtered from the sequencing data using self-programming bioinformatics tools, and the data were spliced using FLASH software (v12.11, http://ccb.jhu.edu/software/FLASH/index.shtml). The splicing sequences were aggregated into OTUs (sortable elements) with USEARCH, which were compared with the bacterial library (Greengene V201305) to obtain the GM compositions of all samples. The bacterial abundance in the samples of both groups was analyzed only at the phylum and genus levels.
2.3. Statistical methods
The ade4 package in R (v3.3.3) software was used to perform principal component analysis (PCA) based on the composition and relative abundance of bacteria in all samples at the genus level. The overall distribution of the microbiota compositions in the two groups was plotted. Bacteria were classified to the phylum and genus levels, and different species between the two groups were investigated by the Wilcoxon method where P < 0.05 indicated a significant difference. The 16S rDNA sequencing data were used to evaluate differences in bacterial functions between the two groups of children based on the functional analysis performed by the Kyoto Encyclopedia of Genes and Genomes (KEGG) database. SPSS 22.0 software was used for general data analysis. The age, weight, height, and IGF-1 values were compared by χ2 tests or two-group independent sample t-tests.
3. Results
3.1. Comparison of differences in the composition of the GM
The GM of two groups of children showed significant differences in α-diversity (P = 0.033) (Figure 1). We used PCA to reduce the dimensionality of the GM data of the two groups, finding that there were marked differences in the GM between the two groups. The genera that contributed most to this difference included Prevotella (P < 0.001), Megamonas (P = 0.01), Bacteroides (P = 0.765), Bifidobacterium (P = 0.011), and Faecalibacterium (P = 0.094) (Figure 2).
3.2. Comparison of the dominant bacterial phyla between the two groups of children
The top five dominant bacterial phyla differed between the groups with a significant increase in the abundance of Bacteroides in the disease group (P = 0.000) together with a significant reduction in the abundance of Firmicutes (P = 0.000). In addition, there was also a significant difference between the two groups in the abundance of Fusobacteria and Actinomycetes (P < 0.05) (Table 2 and Figure 3).
3.3. Comparison of the dominant bacterial genera between the two groups of children
We selected the top 15 dominant bacterial genera in the two groups for comparison. The results showed that the abundance of Prevotella, Fusobacterium, Klebsiella, and Alistipes was significantly increased in the disease group (P < 0.05) while that of Lachnospiracea incertae sedis, Megamonas, Blautia, Clostridium XlVa, Bifidobacterium, and Eubacterium was significantly decreased (P < 0.05) (Table 3 and Figure 4).
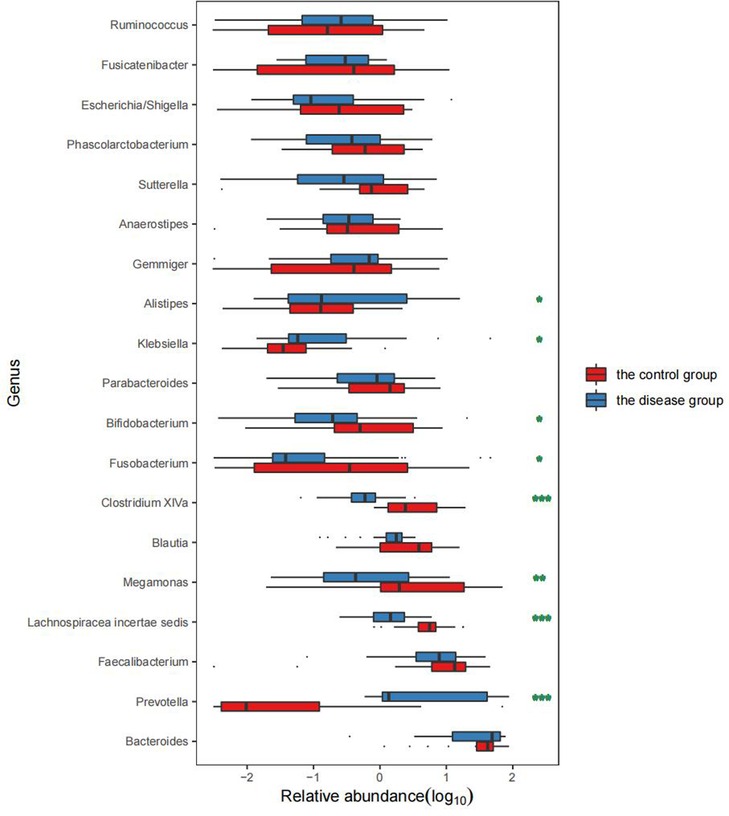
Figure 4. Comparison of the abundance of bacterial genera between the two groups. Remarks: * indicates P < 0.05, ** indicates P < 0.01, and *** indicates P < 0.001 statistically significant differences between the two groups. The higher the number of asterisks, more significant the difference.
3.4. Alterations of GM functions in the GHD children
In comparison with the healthy children, the GHD patients showed significant changes in GM functions, including the decreased “Membrane transport” (P < 0.001, FDR < 0.001), “Lipid metabolism” (P = 0.025, FDR = 0.042), and “Transcription” (P < 0.001, FDR < 0.001, Figure 5), which indicated the. In contrast, the functional categories, such as “Energy metabolism” (P < 0.001, FDR < 0.001), “Metabolism of cofactors and vitamins” (P < 0.001, FDR < 0.001), “Nucleotide metabolism” (P = 0.008, FDR = 0.016), “Glycan biosynthesis and metabolism” (P < 0.001, FDR < 0.001), and “Folding sorting and degradation” (P < 0.001, FDR < 0.001) were enriched in the GHD patients (Figure 5). These elevated GM metabolic activities in the GHD patients, especially the “Glycan biosynthesis and metabolism” function, affect the neuro-regulations in hosts and is probably related to the occurrence of GHD.
3.5. GM and clinical phenotypes
Spearman's correlation analysis was used to investigate associations between the GM of children with GHD and eight endocrine hormones. Our results showed that Bacteroides were positively correlated and Prevotella was negatively correlated with insulin, while Alistipes and Haemophilus showed a negative correlation with GH. A positive correlation was also reported between Fusicatenibacter, Fusobacterium, and Sutterella, whereas Veillonella was negatively correlated with prolactin. Faecaliterium and FSH were positively correlated (Figure 6).
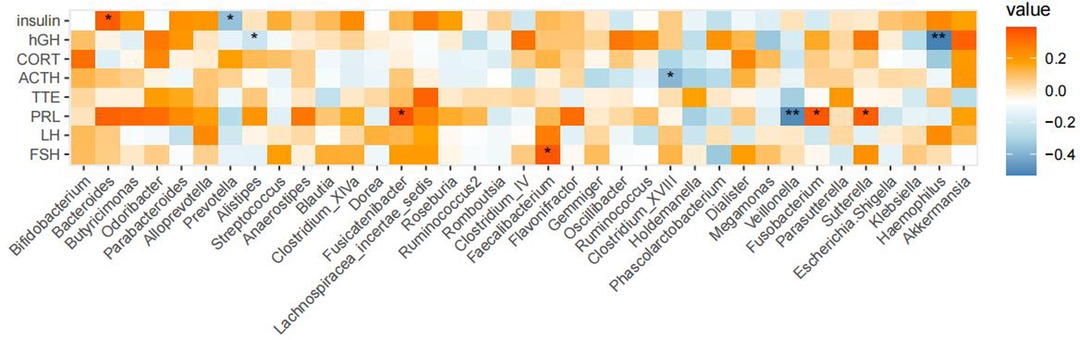
Figure 6. Correlations between GM compositions and endocrine hormones in children with GHD. Legend description: A correlation analysis was performed with eight clinical phenotypes and genera with a relative abundance of ≥0.1%. Results are shown as above where significance was expressed as *P < 0.05 and **P < 0.01.
4. Discussion
4.1. The GM composition differed markedly between the disease and control groups
Compared with the healthy controls, children in the disease group showed reduced α-diversity in the GM, consistent with results reported in malnourished children (16). The phylum Bacteroides was more abundant in children from the disease group than in those from the control group while the opposite trend was observed for Firmicutes, in contrast to findings on obese and diabetic patients (17). The abundance of Prevotella in the disease group was also significantly higher than that in the control group. Prevotella can degrade broad-spectrum plant polysaccharides (18), and carbohydrate-based diets tend to form a Prevotella-dominated “gut type.” Increased abundance of Prevotella abundance has been shown to reduce blood sugar and insulin levels, thus affecting energy absorption and promoting weight loss (19). In the disease group, the abundance of Fusobacterium, Klebsiella, Alistipes, and other genera was found to be significantly increased. Fusobacterium is present in the normal oral flora and can inhibit the immune response as well as promote the transformation of inflammation to malignancy (20). An increase in the abundance of both Klebsiella and Alistipes has been shown to be associated with intestinal inflammation (21, 22); therefore, the increase in the population of these genera can promote chronic inflammation in the intestine and disrupt the function of the intestinal barrier. This can lead to a cellular biochemical imbalance, reduced absorption capacity, and increased susceptibility to enteric pathogen infections, and consequently affect energy metabolism and nutrient absorption (23). In addition, Klebsiella and Alistipes are both associated with neurological diseases (24) and can produce neurotransmitter-related metabolites such as serotonin, dopamine, and histamine (25). These neurotransmitters enter the brain through the gut-brain axis to regulate the energy balance and function of the hypothalamus (26, 27). The hypothalamus is the highest regulatory center of thehypothalamic–pituitary–growth axis (HPA) and can reduce appetite and cause weight loss (28, 29). Lachnospiracea incertae sedis, Megamonas, Blautia, Clostridium XlVa, and Bifidobacterium were found to be significantly reduced in the intestines of the disease group, which could lead to reduced concentrations of SCFAs such as butyric acid produced by these beneficial bacteria (30). Decreased abundance of Lachnospiracea incertae sedis might also affect protein synthesis (31), disturb the intestinal energy supply, and retard growth and development. Jensen et al. (5) reported that increased Prevotella abundance together with reduced numbers of Bifidobacterium can reduce the levels of growth hormone-releasing peptide (GHRP) and leptin, thus reducing the release of GH.
4.2. Significant differences in GM function between the two groups
The enriched functional categories in the GHD group included “Replication and repair, Energy metabolism, Poorly characterized, Metabolism of cofactors and vitamins, Nucleotide metabolism, Cellular processes and signaling, Nucleotide metabolism, Glycan biosynthesis and metabolism, Transcription, Folding sorting and degradation”. Children with GHD showed dysregulation in energy metabolism, vitamin and related factor metabolism, and polysaccharide metabolism and biosynthesis. Considering that Prevotella significantly increases the catabolism of carbohydrates and that the abundance of butyric acid and other bacteria such as Lachnospiracea incertae sedis is significantly reduced in children with GHD, the GM imbalance in this population may affect the functions of the flora. This phenomenon may lead to chronic inflammation of the intestine and poor intake and absorption of nutrients such as fats and proteins, affecting both growth and development.
4.3. GM is closely related to the clinical phenotype
We conducted a correlation analysis of the GM and endocrine hormones and found that Prevotella abundance was negatively correlated with insulin. Significantly higher abundance of Prevotella can affect insulin secretion, which can not only regulate food intake (19, 32) but also modulate blood glucose levels through signaling pathways essential for maintaining energy storage, glucose metabolism, sugar production, adipogenesis, cell growth, survival, and reproduction (33). We speculate that this significant increase in Prevotella abundance may be detrimental to growth and development. We also found a variety of other intestinal bacteria related to endocrine hormones and confirmed the interaction between the GM and endocrine hormones. Maintaining the stability of the GM is conducive to the promotion of growth and development.
5. Conclusion
There was a significant reduction in the α-diversity of the intestinal microbial composition in GHD children, together with an increased abundance of Bacteroides and reduced numbers of Firmicutes. Fusobacterium, Klebsiella, Alistipes, and other genera were significantly enriched in children with GHD while the numbers of Lachnospiracea incertae sedis, Megamonas, Blautia, Clostridium XlVa, and Bifidobacterium were significantly reduced. These imbalances in the GM were predicted to affect pathways involved in energy metabolism and biosynthesis, as well as induce abnormal secretion of insulin and other endocrine hormones, which may promote the occurrence and development of GHD.
5.1. Deficiencies and next steps
There are many factors that cause insufficiency in GH secretion in children with GHD, and GM imbalance may be one of the major factors. On the one hand, GM imbalance leads to the abnormal secretion of endocrine hormones as well as an abnormal production of microbial metabolites, especially neurotransmitters, that can influence the HPA through the gut–brain axis. The sample size in the present study was small, consisting of only 33 children with GHD; hence, large-sample, multi-center research is needed to verify the associations between the GM and GHD. Studies combined with metabolomics could better clarify the mechanism of action of the GM and its metabolites in growth and development.
Data availability statement
The data presented in the study are deposited in the NCBI sequence Archive (SRA) database, accession number: PRJNA899674 .The data can be found at the following link: https://dataview.ncbi.nlm.nih. gov/object/PRJNA899674?reviewer=tu7mnej3p04c61u31c6f4v5hgo.
Ethics statement
The studies involving human participants were reviewed and approved by The Ethics Committee of Shenzhen Longgang District Maternity and Child Health Care Hospital approved the study, with the approval number of LGFYYXLL-024. Written informed consent to participate in this study was provided by the participants’ legal guardian/next of kin. Written informed consent was obtained from the minor(s)’ legal guardian/next of kin for the publication of any potentially identifiable images or data included in this article.
Author contributions
CH and HL: managed the project. DM, YL, SL, and WY: were responsible for the registration of the clinical information of the enrolled children and the collection of stool samples according to the standard configuration. CH, YL, and ZY: were responsible for DNA extraction and biological information analysis, etc. HL and ZY: were responsible for tabulation and statistical analyses. CH and DM: were responsible for interpreting various data and writing papers. All authors contributed to the article and approved the submitted version.
Funding
This research was supported by Longgang District Science and Technology Innovation Bureau (grant no. LGKCYLWS2019000186), Shenzhen Technology Bureau Foundation (grant no. JCYJ20220530155006013) and Internal project of Shenzhen Maternal and Child Health Hospital (grant no. FYB2017004).
Acknowledgments
We sincerely thank all the enrolled children and their guardians for their trust and cooperation, which enabled us to successfully complete this study. We are also very grateful to all the medical staff members of Longgang District Maternity & Child Healthcare Hospital for their efforts in this study. The authors would like to thank all the reviewers who participated in the review and MJEditor (www.mjeditor.com) for its linguistic assistance during the preparation of this manuscript.
Conflict of interest
The authors declare that the research was conducted in the absence of any commercial or financial relationships that could be construed as a potential conflict of interest.
Publisher's note
All claims expressed in this article are solely those of the authors and do not necessarily represent those of their affiliated organizations, or those of the publisher, the editors and the reviewers. Any product that may be evaluated in this article, or claim that may be made by its manufacturer, is not guaranteed or endorsed by the publisher.
References
1. Han XW, Dong ZY, Zhang WY, Ma XY, An JG, Xiao Y, et al. Analysis of the etiology and clinical characteristics of short stature. J Clin Pediatr. (2019) 37(1):39–42. doi: 10.3969/j.issn.1000-3606.2019.01.010
2. Chinoy A, Murray PG. Diagnosis of growth hormone deficiency in the paediatric and transitional age. Best Pract Res Clin Endocrinol Metab. (2016) 30(6):737–47. doi: 10.1016/j.beem.2016.11.002
3. Qi XY, Yun CY, Pang YL, Qiao J. The impact of the GM on the reproductive and metabolic endocrine system. Gut Microbes. (2021) 13(1):1–21. doi: 10.1080/19490976.2021.1894070
4. Hsu E, Pacififici R. From osteoimmunology to osteomicrobiology: how the microbiota and the immune system regulate bone. Calcif Tissue Int. (2018) 102(5):512–21. doi: 10.1007/s00223-017-0321-0
5. Jensen EA, Young JA, Mathes SC, List EO, Carroll RK, Kuhn J, et al. Crosstalk between the growth hormone/insulin-like growth factor-1 axis and the gut microbiome: a new frontier for microbial endocrinology. Growth Horm IGF Res. (2020) 53-54:101333. doi: 10.1016/j.ghir.2020.101333
6. Martel-Pelletier J, Di Battista JA, Lajeunesse D, Pelletier JP. GF/IGFBP axis in cartilage and bone in osteoarthritis pathogenesis. Inflamm Res. (1998) 47(3):90–100. doi: 10.1007/s000110050288
7. Philbrick KA, Wong CP, Branscum AJ, Turner RT, Iwaniec UT. Leptin stimulates bone formation in ob/ob mice at doses having minimal impact on energy metabolism. J Endocrinol. (2017) 232(3):461–74. doi: 10.1530/JOE-16-0484
8. Villa-Osaba A, Gahete MD, Cordoba-Chacon J, De Lecea L, Castaño JP, Luque RM. Fasting modulates GH/IGF-I axis and its regulatory systems in the mammary gland of female mice: influence of endogenous cortistatin. Mol Cell Endocrinol. (2016) 434:14–24. doi: 10.1016/j.mce.2016.06.014
9. Shamsi BH, Chatoo M, Xu XK, Xu X, Chen XQ. Versatile functions of somatostatin and somatostatin receptors in the gastrointestinal system. Front Endocrinol. (2021) 12:652363. doi: 10.3389/fendo.2021.652363
10. Schubert ML. Physiologic, pathophysiologic, and pharmacologic regulation of gastric acid secretion. Curr Opin Gastroenterol. (2017) 33(6):430–8. doi: 10.1097/MOG.0000000000000392
11. Hjelholt AJ, Lee KY, Arlien-Søborg MC, Pedersen SB, Kopchick JJ, Puri V, et al. Temporal patterns of lipolytic regulators in adipose tissue after acute growth hormone exposure in human subjects: a randomized controlled crossover trial. Mol Metab. (2019) 29:65–75. doi: 10.1016/j.molmet.2019.08.013
12. Dixit M, Poudel SB, Yakar S. Effects of GH/IGF axis on bone and cartilage. Mol Cell Endocrinol. (2021) 519:111052. doi: 10.1016/j.mce.2020.111052
13. Li L, An JJ, Wang JQ, Wang XQ, Dong ZY. The structure of gut microbiome in idiopathic short stature profiled by 16S rRNA second generation sequencing sequencing. J Diagn Concepts Pract. (2021) 20(2):149–54. doi: 10.16150/j.1671-2870.2021.02.006
14. Al-Samerria S, Radovick S. The role of Insulin-like Growth Factor-1 (IGF-1) in the control of neuroendocrine regulation of growth. Cells. (2021) 10(10):2664. doi: 10.3390/cells10102664
15. The Subspecialty Group of Endocrinologic, Hereditary and Metabolic Diseases, The Society of Pediatrics, Chinese Medical Association. Guidelines for diagnosis and treatment of children with short stature. Chin J Pediatr. (2008) 46(6):20–1.
16. Guerrant RL, DeBoer MD, Moore SR, Scharf RJ, Lima AAM. The impoverished gut–a triple burden of diarrhoea, stunting and chronic disease. Nat Rev Gastroenterol Hepatol. (2013) 10(4):220–9. doi: 10.1038/nrgastro.2012.239
17. Ley RE, Turnbaugh PJ, Klein S, Gordon JI. Microbial ecology: human gut microbes associated with obesity. Nature. (2006) 444:1022–3. doi: 10.1038/4441022a
18. Wu GD, Chen J, Hoffmann C, Bittinger K, Chen YY, Keilbaugh SA, et al. Linking long-term dietary patterns with gut microbial enterotypes. Science. (2011) 333(6052):105–8. doi: 10.1126/science.1208344
19. Hjorth MF, Blædel T, Bendtsen LQ, Lorenzen JK, Holm JB, Kiilerich P, et al. Prevotella-to-bacteroides ratio predicts body weight and fat loss success on 24-week diets varying in macronutrient composition and dietary fiber: results from a post-hoc analysis. PLoS One. (2016) 11(9):e0161211. doi: 10.1038/s41366-018-0093-2
20. Zhou Z, Chen J, Yao H, Hu H. Fusobacterium and colorectal cancer. Front Oncol. (2018) 8:371. doi: 10.3389/fonc.2018.00371
21. Atarashi K, Suda W, Luo C, Kawaguchi T, Motoo I, Narushima S, et al. Ectopic colonization of oral bacteria in the intestine drives TH1 cell induction and inflammation. Science. (2017) 358(6361):359–65. doi: 10.1126/science.aan4526
22. Saulnier DM, Riehle K, Mistretta TA, Diaz MA, Mandal D, Raza S, et al. Gastrointestinal microbiome signatures of pediatric patients with irritable bowel syndrome. Gastroenterology. (2011) 141(5):1782–91. doi: 10.1053/j.gastro.2011.06.072
23. Sharon G, Cruz NJ, Kang DW, Gandal MJ, Wang B, Kim YM, et al. Human GM from autism spectrum disorder promote behavioral symptoms in mice. Cell. (2019) 177(6):1600–1618 e17. doi: 10.1016/j.cell.2019.05.004
24. Kau AL, Ahern PP, Griffin NW, Goodman AL, Gordon JI. Human nutrition, the gut microbiome and the immune system. Nature. (2011) 474(7351):327–36. doi: 10.1038/nature10213
25. Strandwitz P. Neurotransmitter modulation by the GM. Brain Res. (2018) 1693(Pt B):128–33. doi: 10.1016/j.brainres.2018.03.015
26. Louwies T, Johnson AC, Orock A, Yuan T, Meerveld BGV. The microbiota-gut-brain axis: an emerging role for the epigenome. Exp Biol Med. (2020) 245(2):138–45. doi: 10.1177/1535370219891690
27. Heiss CN, Olofsson LE. GM-dependent modulation of energy metabolism. J Innate Immun. (2018) 10(3):163–71. doi: 10.1159/000481519
28. Georgescu T, Lyons D, Heisler LK. Role of serotonin in body weight, insulin secretion and glycaemic control. J Neuroendocrinol. (2021) 33(4):e12960. doi: 10.1111/jne.12960
29. Li H, Li Y, Zhang X, Ren G, Ren G, Wang LF, et al. The combination of Aquilaria sinensis (Lour.) Gilg and Aucklandia costus Falc. Volatile oils exerts antidepressant effects in a CUMS-induced rat model by regulating the HPA axis and levels of neurotransmitters. Front Pharmacol. (2021) 11:614413. doi: 10.3389/fphar.2020.614413
30. Zhang JD, Song LJ, Wang YJ, Liu C, Zhang L, Zhu SW, et al. Benefificial effect of butyrate-producing Lachnospiraceae on stress-induced visceral hypersensitivity in rats. J Gastroenterol Hepatol. (2019) 34(8):1368–76. doi: 10.1111/jgh.14536
31. Chen WG, Liu FL, Ling ZX, Tong XJ, Xiang CL. Human intestinal lumen and mucosa-associated microbiota in patients with colorectal cancer. PLoS One. (2012) 7(6):e39743. doi: 10.1371/journal.pone.0039743
32. Mitchell CS, Begg DP. The regulation of food intake by insulin in the central nervous system. J Neuroendocrinol. (2021) 33(4):e12952. doi: 10.1111/jne.12952
Keywords: high-throughput sequencing, growth hormone deficiency, GM, KEGG functional category, composition
Citation: Huang C, Meng D, Li Y, Lu S, Yang W, Wu B, Chen S, Yang Z and Liu H (2023) Gut microbiota composition alteration analysis and functional categorization in children with growth hormone deficiency. Front. Pediatr. 11:1133258. doi: 10.3389/fped.2023.1133258
Received: 28 December 2022; Accepted: 8 February 2023;
Published: 24 February 2023.
Edited by:
Eli Hershkovitz, Soroka Medical Center, IsraelReviewed by:
Kiran Veer Sandhu, University College Cork, IrelandSzymon Skoczen, Jagiellonian University Medical College, Poland
© 2023 Huang, Meng, Li, Lu, Yang, Wu, Chen, Yang and Liu. This is an open-access article distributed under the terms of the Creative Commons Attribution License (CC BY). The use, distribution or reproduction in other forums is permitted, provided the original author(s) and the copyright owner(s) are credited and that the original publication in this journal is cited, in accordance with accepted academic practice. No use, distribution or reproduction is permitted which does not comply with these terms.
*Correspondence: Haiying Liu liuhaiying26@163.com
†These authors have contributed equally to this work
Specialty Section: This article was submitted to Pediatric Endocrinology, a section of the journal Frontiers in Pediatrics